Abstract
Metal ions, especially copper, zinc and iron, play an important role in the neurodegeneration process because they can affect protein misfolding, leading to the formation of the amyloid deposits and oxidative stress leading to reactive oxygen species (ROS). Here we report the synthesis and evaluation as antioxidant and metal chelating agents of 3,4-dihydroxybenzoic acid derivatives. Synthesized compounds were tested by the 2,2-diphenyl-2-picrylhydrazyl (DPPH) method showing a radical scavenging ability (EC50 = 0.093–0.118 μM) higher than Trolox used as reference. Furthermore, these compounds were able to bind both iron and copper, especially the iron (III), by the formation of hexa-coordinated complexes. Synthesized compounds were tested to evaluate their ability to inhibit acetyl- and butyryl-cholinesterase; the obtained results have demonstrated that they are selective inhibitors of AChE (Ki = 1.5–18.9 μM) and result weakly active versus butyrylcholinesterase (BChE).
Introduction
The events leading to various neurodegenerative diseases, e.g. Alzheimer’s (AD) or Parkinson’s (PD) disease, are still unknown. However, metal ions have long been suspected to play a key role in the pathogenesis of neurodegenerative disorders. The relationship between the development of diseases and metals is very complex, but it has become clear that alterations in metal homeostasis and their interactions with biomolecules can drive the progression of neurological diseasesCitation1.
In the central nervous system (CNS), iron, copper and zinc are required for many enzymatic activities, mitochondrial function, neurotransmission, learning and memory. The concentration of these vital metals needs to be finely tuned and any dysregulation may lead to severe disease as a consequence of cell death in specific brain areasCitation2.
High levels of Cu and Zn ions are found in amyloid plaques, commonly seen in AD brains, and in the cerebrospinal fluid of patients affected by AD or PD. High Fe ions levels are also frequently observed in PD and AD. Several transition metal ions also bind proteins associated with neurodegenerative diseases, such as β-amyloid (Aβ) and α-synuclein, and affect their aggregation processCitation2.
Moreover, it is well known that iron and copper play a well-established role as catalysts for the production of reactive oxygen species (ROS) via the Fenton reactions, so, they are a major cause of the oxidative stressCitation3. Thus, the modulation of bio-metals in the brain has been proposed as a potential therapeutic strategy for the treatment of neurodegenerative diseases, especially for ADCitation4,Citation5.
In view of the multifactorial nature of AD, more efforts are directed to develop new drugs that could be beneficial to the development and progression of symptoms by acting at different levels on the involved mechanisms. Recently, many published papers report the synthesis and the study of cholinesterase inhibitors that are at the same time anti-oxidants and / or metal chelating and / or inhibitors of amyloid aggregationCitation6–8, and the great amount of work on this topic is widely reported in numerous reviewsCitation9–11.
Protocatechuic acid (3,4-dihydroxybenzoic acid) possesses well known antioxidant and metal chelating propertiesCitation12–14 and it appears to be an interesting starting molecule which can be structurally modified by addition of further functional groups that can provide additional biological activity. In particular, keeping in mind the cholinergic hypothesis of ADCitation15 and the key role played by metal cations and ROS in the neurodegeneration correlated to AD, we designed derivatives 8–11, joining antioxidant and chelating properties of 3,4-dihydroxybenzoic acid with anticholinesterase activity due to the presence of a carbamic moiety typical of many acetylcholinesterase (AChE) inhibitorsCitation16.
Methods
Chemical synthesis
All reagents, solvents and deuterated were purchased from Sigma-Aldrich (Milano, Italia). Benzene was dried just before use by mean of Dean Stark apparatus. Acetonitrile was freshly purified by distillation over calcium hydride.
IR spectra were recorded on a FT-IR Perkin-Elmer SpectrumOne equipped with ATR system; signals are given as ν (cm−1). 1H and Citation13C NMR spectra were recorded on AVANCE400 Bruker spectrometer operating at 400 and 100 MHz, respectively; chemical shifts are given in ppm (δ) with respect to TMS as internal reference, coupling constant are given in Hz. Melting points were determined on Tottoli apparatus (Buchi) and are uncorrected. Elemental analyses were obtained by a PE 2400 (PerkinElmer, Waltham, MA) analyzer.
Synthesis of carbamates 4--7
4-Aminobutyl-diethylcarbamate (4)
4-Aminobutan-1-ol (1.0 g, 11.2 mmol) was added with 1.5 mL of aqueous 6 M HCl and 30 mL of benzene and was refluxed for 3 h in a Dean-Stark apparatus. After this time the benzene solution was cooled at room temperature (RT) and then the solvent was removed under reduced pressure to give a crude residue (4-aminobutan-1-ol hydrochloride) that was used without further purification. To the obtained residue 15.0 mL of anhydrous CH3CN and 4.6 g of diethylcarbamoyl chloride (33.7 mmol) were added and the suspension was refluxed until complete dissolution (approximately 12 h).
After this time, the cooled solution was added with 6.0 mL of water and 0.1 M HCl until pH 4, stirred for 15 min, added with 0.1 M Na2CO3 until pH 8 and extracted with chloroform (5 × 15.0 mL). Combined organic layers were dried over Na2SO4, then the solvent was removed under reduced pressure to give rise to 1.55 g of a colorless oily product (73.5% yield). I.R. (neat, ν, cm−1): 2934, 1685, 1273, 1172, 1071, 1004, 748. 1H NMR (CDCl3): δ ppm 3.97 (t, 2 H, J = 6.5 Hz, -CH2-O), 3.19–3.11 (m, 4 H, CH3-CH2-N), 2.65 (t, 2 H, J = 7.1 Hz, -CH2-CH2-N), 1.58–1.47 (m, 4 H, J = 7.3 Hz, -CH2-CH2-), 1.09–0.98 (m, 6 H, CH3). Citation13C NMR (CDCl3): δ ppm 155.9, 64.5, 41.5, 41.2, 40.6, 27.9, 26.4, 13.9, 13.4. Elemental analysis: Calcd for C9H20N2O2 C 57.42%, H 10.71%, N 14.88%; Found C 57.21% H 10.74%, N 14.82%.
5-aminopentyl-dimethylcarbamate (5)
Compound 5 was prepared with the same procedure described for 4 using 5-aminopentan-1-ol and dimethylcarbamoyl chloride. Pure 5 was obtained as a pale yellow waxy solid (yield 70%). I.R. (neat, ν, cm−1): 2933, 1688, 1186, 1059, 954, 770. 1H NMR (CDCl3): δ ppm 4.00 (t, 4 H, J = 6.5 Hz,-CH2-O), 2.82 (s, 6 H, -CH3), 2.57 (t, 2 H, J = 7.1 Hz, -CH2-N), 1.56–1.60 (m, 2 H, -CH2-CH2-O), 1.36–1.41 (m, 4 H, -CH2-CH2-). Citation13C NMR (CDCl3): δ ppm 156.8, 65.2, 41.9, 36.3, 35.8, 32.9, 28.9, 23.3. Elemental analysis: Calcd for C8H18N2O2 C 55.15%, H 10.41%, N 16.08%; Found C 54.99%, H 10.45%, N 16.03%.
5-aminopentyl-diethylcarbamate (6)
Compound 6 was prepared with the same procedure described for 4 using 5-aminopentan-1-ol and diethylcarbamoyl chloride. Pure 6 was obtained as a pale yellow viscous oil (yield 60%). I.R. (neat, ν, cm−1): 2935, 1676, 1275, 1174, 1072, 770. 1H NMR (CDCl3): δ ppm 3.95 (t, 2 H, J = 6.6 Hz, -CH2-O), 3.15 (s, 4 H, CH3-CH2-N), 2.75 (t, 2 H, J = 7.4 Hz, -CH2-NH2), 1.55–1.59 (m, 4 H, -CH2-CH2-O +-CH2-CH2-NH2), 1.31–1.38 (m, 2 H, -CH2-CH2-CH2-), 1.00 (t, 6 H, J = 7.1 Hz, CH3). Citation13C NMR (CDCl3): δ ppm 155.8, 64.4, 41.3, 40.1, 29.0, 28.4, 22.8, 13.4. Elemental analysis: Calcd for C10H22N2O2 C 59.37%, H 10.96%, N 13.85%; Found C 59.15%, H 10.99%, N 13.81%.
6-aminohexyl-diethylcarbamate (7)
Compound 7 was prepared with the same procedure described for 4 using 6-aminopentan-1-ol and diethylcarbamoyl chloride. Pure 7 was obtained as a pale yellow viscous oil (yield 86%). I.R. (neat, ν, cm−1): 2934, 1690, 1273, 1172, 1071, 1004, 748. 1H NMR (dimethyl sulfoxide (DMSO) + 1% C6D6): δ ppm 4.00 (t, 2 H, J = 6.5 Hz, -CH2-O), 3.23 (q, 4 H, J = 7.1 Hz, CH3-CH2-N), 2.62 (t, 2 H, J = 7.0 Hz, -CH2-NH2), 1.53–1.60 (m, 2 H, -CH2-CH2-O), 1.41–1.47 (m, 2 H, -CH2-CH2-NH2), 1.30–1.36 (m, 4 H, C-CH2-CH2-C), 1.07 (t, 6 H, J = 7.1 Hz, CH3). Citation13C NMR (CD3OD): δ ppm 156.0, 64.8, 41.5, 41.2, 40.9, 30.8, 28.9, 26.3, 25.6, 13.9. Elemental analysis: Calc for C11H24N2O2 C 61.07%, H 11.18%, N 12.95%; Found C 60.88%, H 11.19%, N 12.90%.
Synthesis of carbamates 8--11
4-[(3,4-Dihydroxybenzoyl)amino]butyl-diethylcarbamate (8)
3,4-Dihydroxybenzoic acid (0.2 g, 1.29 mmol) 15.0 mL of anhydrous CH3CN was heated at 40 °C until complete dissolution. N,N'-Bis(4-methylphenyl)carbodiimide (0.29 g, 1.30 mmol) was added and the suspension was stirred 24 h at 40 °C. After this time, 5.0 mL of anhydrous CH3CN, containing 0.25 g (1.33 mmol) of carbamate 4, were added and the suspension was stirred for additional 24 h at 40 °C.
The suspension was cooled, the white precipitate was collected by centrifugation and washed with cold MeOH (4 × 1.5 mL); the obtained white precipitate was discarded and the combined methanolic solutions were evaporated under reduced pressure. The obtained crude residue was further washed with cold methanol (4 × 1.0 mL), each time discarding the precipitate, and the combined methanolic solutions was then evaporated under reduced pressure to provide a white residue that was crystallized from 2-propanol to give pure 8 as a pale yellow hygroscopic solid (yield 48%). Melting point (mp) 56–60 °C. I.R. (neat, ν, cm−1): 2971, 1666, 1600, 1276, 1174, 1007, 947, 770. 1H NMR (CD3OD): δ ppm 7.48 (d, 1 H, J = 2.0 Hz, Ar), 7.41 (dd, 1 H, J = 2.0 and 8.2 Hz, Ar), 6.78 (d, 1 H, J = 8.2 Hz, Ar), 4.09–4.14 (m, 2 H, -CH2-O), 3.29 (q, 4 H, J = 7.1 Hz, CH3-CH2-N), 2.90–2.96 (m, 2 H, -CH2-NH-), 1.69–1.75 (m, 4 H, -CH2-CH2-), 1.13 (t, 6 H, J = 7.1 Hz, CH3-CH2-). Citation13C NMR (CD3OD): δ ppm 174.2, 156.3, 147.8, 144.1, 128.8, 121.6, 116.4, 113.9, 64.3, 41.6, 41.2, 39.1, 25.8; 24.3, 12.9, 12.4. Elemental analysis: Calcd for C16H24N2O5 C 59.24%, H 7.46%, N 8.64%; Found C 59.01%, H 7.49%, N 8.60%.
5-[(3,4-Dihydroxybenzoyl)amino]pentyl dimethylcarbamate (9)
Compound 9 was prepared with the same procedure described for 8; carbamate 5 was used as reactant and the reaction mixture was heated 72 h at 40°C. Pure 9 was obtained as a light yellow hygroscopic solid (yield 60%). I.R. (neat, ν, cm−1): 2936, 1672, 1600, 946, 893, 769. 1H NMR (CD3OD): δ ppm 7.70 (s, 1 H, Ar), 7.39 (d, 1 H, J = 8.2 Hz, Ar), 6.79 (d, 1 H, J = 8.2 Hz, Ar), 4.03 (t, 2 H, J = 6.4 Hz, -CH2-O), 2.92 (t, 2 H, J = 7.6 Hz, -CH2-NH-), 2.88 (s, 6 H, CH3), 1.60–1.67 (m, 4 H, -CH2-CH2-), 1.38–1.44 (m, 2 H, -CH2-CH2-CH2-). Citation13C NMR (CD3OD): δ ppm 176.1, 159.3, 146.4, 123.8, 118.7, 116.3, 67.1, 41.4, 37.0, 30.4, 29.1, 24.8. Elemental analysis: Calcd for C15H22N2O5 C 58.05%, H 7.15%, N 9.03%; Found C 57.81%, H 7.19%, N 9.01%.
5-[(3,4-Dihydroxybenzoyl)amino]pentyl diethylcarbamate (10)
Compound 10 was prepared with the same procedure described for 8; carbamate 6 was used as reactant and the reaction mixture was heated 72 h at 40°C. Pure 10 was obtained as a white hygroscopic solid (yield 45%). I.R. (neat, ν, cm−1): 2937, 1666, 1640, 1280, 1181, 1093, 771. 1H NMR (DMSO): δ ppm 7.36 (d, 1 H, J = 2.0 Hz, Ar), 7.29 (dd, 1 H, J = 8.2 and 2.0 Hz, Ar), 6.77 (d, 1 H, J = 8.2 Hz, Ar), 4.00 (t, 2 H, J = 6.6 Hz, -CH2-O), 3.23 (q, 4 H, J = 7.1 Hz, CH3-CH2-N), 2.76 (t, 2 H, J = 7.2 Hz, -CH2-NH-), 1.53–1.61 (m, 4 H, -CH2-CH2-O +-CH2-CH2-NH-), 1.32–1.40 (m, 2 H, CH2-CH2-CH2), 1.08 (t, 6 H, J = 7.1 Hz, CH3-CH2-). Citation13C NMR (CD3OD): δ ppm 169.4, 154.9, 147.7, 127.4, 114.9, 112.7, 63.0, 39.8, 37.8, 26.8, 25.4, 21.1, 11.0. Elemental analysis: Calcd for C17H26N2O5 C 60.34%, H 7.74%, N 8.28%; Found C 60.13%, H 7.76%, N 8.26%.
5-[(3,4-Dihydroxybenzoyl)amino]hexyl diethylcarbamate (11)
Compound 11 was prepared with the same procedure described for 8; carbamate 7 was used as reactant and the reaction mixture was heated 24 h at 40 °C. Pure 11 was obtained as a white solid (yield 62%). mp 155–159 °C. I.R. (neat, ν, cm−1): 3038, 2936, 1665, 1603 1278, 1172, 1071, 1004, 748. 1H NMR (CD3OD): δ ppm 7.46 (d, 1 H, J = 2.1 Hz, Ar), 7.39 (dd, 1 H, J = 8.2 and 2.1 Hz, Ar), 6.78 (d, 1 H, J = 8.2 Hz, Ar), 4.10 (t, 2 H, J = 7.2 Hz, -CH2-O), 3.30 (q, 4 H, J = 7.5 Hz, CH3-CH2-N), 2.93 (t, 2 H, J = 6.6 Hz, -CH2-NH-), 1.65–1.77 (m, 4 H, -CH2-CH2-O +-CH2-CH2-NH-), 1.40–1.51 (m, 4 H, CH2-CH2-CH2-CH2), 1.13 (t, 6 H, J = 7.5 Hz, CH3-CH2-N). Citation13C-NMR (CD3OD): δ ppm 173.5, 156.5, 147.9, 144.1, 128.1, 121.7, 116.4, 113.9, 64.8, 41.3, 39.3, 28.5, 27.2, 25.6, 25.1, 12.7. Elemental analysis: Calcd for C18H28N2O5 C 61.34%, H 8.01%, N 7.95%; Found C 61.13%, H 8.03%, N 7.93%.
Antioxidant activity tests
The antioxidant activity was evaluated using the spectrophotometric DPPH method according to Brand-Williams et al..based on the ability of antioxidant compounds to react with the stable radical 2,2-diphenyl-2-picrylhydrazyl (DPPH·) characterized by an absorption maxima at 490 nm. An UV-Vis lambda 40 Perkin Elmer spectrophotometer, optical polystyrene cuvettes and methanol as solvent were used; each measure was repeated at least in triplicate.
A calibration equation was obtained (r2 = 0.996) by measuring the absorbance of DPPH solutions at concentrations ranging between 25 and 150 µM. To assess the antioxidant activity of compounds (8–11) 3.0 mL of 100 µM DPPH were placed in a cuvette and the absorbance at 490 nm was read in order to determine the initial concentration of DPPH radical; therefore, the opportune amount of methanolic solution of each carbamate was added to obtain cuvette concentrations ranging from 5 to 30 µM. For each cuvette the absorbance was read each 20 min until a plateau was reached. For each carbamate concentration, the residual plateau percentage of DPPH radical was calculated from residual absorbance using the calibration equation and the obtained values were plotted versus the molar ratio [carbamate]/[DPPH] to graphically extrapolate the EC50 values (efficient concentration = EC50 expressed as the moles of antioxidant per moles of DPPH needed to reduce to 50% the initial DPPH).
Complexation studies
The ability of carbamates 8–11 to complex the metal ions Fe+3, Fe+2, Cu+2 and Zn+2 was achieved using the continuous variation method of Job, based on the UV-Vis absorption maxima intensity changes due to the complex formation. This method requires to mix, in adequate proportions, equimolar solutions of metal ion and ligand, recording the UV spectra and plotting the difference in the maximum absorbance versus the molar ratio (X) of the ligand; in this way the stoichiometric coefficient of the complex can be obtained.
FeCl3·6H2O, FeSO4·7H2O, CuSO4·6H2O and ZnSO4·7H2O were purchased from CARLO ERBA REAGENT (Milano, Italia); UV-Vis lambda 40 Perkin Elmer spectrophotometer and quartz HELLMA 10 mm cuvette were used. Each measure was triplicate and data were elaborated using UV-WIN Lab Version 2.0, Perkin Elmer Corporation and Microsoft Excel 2010.
The same procedure was followed for all carbamates towards each metal cations. Briefly, 4 × 10−4 M solutions of each carbamate in 1:1 MeOH/water and inorganic salts 4 × 10−4 M in water were prepared. The solutions of each carbamate and each metal ion were mixed in order to obtain an increasing molar ration of carbamate (from 0.1 to 0.95) and maintaining unchanged the total concentration of carbamate + metal ion (4 × 10−4 M). Each solution was placed in cuvette and the UV-Vis spectra were recorded between 200 and 400 nm using an equimolar carbamate solution as blank; from these spectra was algebraically subtracted that of an equimolar solution of the same cation in order to obtain the exclusive spectral changes due to the complex formation.
The molar ratio (X) that causes the maximum ΔA can be obtained plotting the differences in absorbance measured at two wavelength (usually absorption maxima) versus molar ratio of ligand. The stoichiometry of the complex can be easily calculated with the following equation:
where n is the number of ligand molecules per cation present in the complex.
Enzymatic inhibition studies
Electric eel AChE (EC 3.1.1.7), equine BChE (EC 3.1.1.8), acetylthiocholine iodide, butyrylthiocholine iodide and 5,5′-dithio-bis-(2-nitrobenzoic acid) (DTNB) were purchased from Sigma-Aldrich (Milano, Italy). All other chemical and biological reagents and solvents used were of the highest analytical, commercially available grade. Enzymatic inhibition of carbamates 8–11 were assessed by means of Ellman method, as previously describedCitation25, using a double beam UV-Vis lambda 40 Perkin Elmer spectrophotometer and optical polystyrene cuvettes (10 × 10 × 45 mm, 340–800 nm optical transparency); each measure was repeated at least in triplicate. AChE and BChE were daily tested to evaluate the effective enzymatic activity.
Enzymatic inhibition tests were carried out by mixing in a polystyrene cuvette 3.0 mL of 0.1 M (pH 7.4) phosphate buffer containing DTNB (0.25 mM) and AChE (0.028 U mL−1) or BChE (0.08 U mL−1) with each carbamate derivatives 8–11 (final concentration range 1.0–10.0 µM). The reaction was started by the addition of acetylthiocholine iodide or butyrylthiocholine iodide (200–600 μM) and the changes in the absorbance at 412 nm were recorded at 25 °C between 0.5 and 1.5 min after reagent addition. The data were analyzed with the enzyme kinetic module of SigmaPlot, version 8.02 a, in order to find the best fitting model of inhibition, as indicated by calculated linear regression coefficient r2. Ki values were obtained according to Dixon’s method, reporting the reciprocal of the hydrolysis rate versus the carbamate concentrations.
Molecular docking studies
Molecular docking study was performed in order to suggest a possible binding mode of all synthesized compounds with AChE and BChE.
The crystal complex (code: 1EVE, resolution of 2.50 Å) of Torpedo californica AChE (TcAChE) with donepezil and the crystal (code: 1P0I, resolution of 2.00 Å) of hBChE were downloaded from PDB (http://www.rcsb.org).
Prior to performing molecular docking, the protein PDB file was prepared using the Dock Prep tool available in the free software package UCSF Chimera 1.7. This involved the addition of hydrogen atoms, removal of solvent (water) molecules and assigning partial charges (using the AMBER99 force field).
Docking calculations were carried out using SwissDock, an online server that docks ligand to protein using EADock DSS software. Docking runs were performed using the “Accurate” parameters option, which is the most exhaustive in terms of the number of binding modes sampled.
Output clusters were obtained after each docking run and were ranked according to the FullFitness (FF) scoring function specified by the SwissDock algorithm (cluster 0 being the cluster with the best FF score). A greater negative FF score indicates a more favorable binding mode with a better fit. Within each cluster, the individual binding poses were further arranged and ranked based on their FF score.
The EADock DSS software was able to identify the native binding mode of donepezil as the best FF score binding conformation.
Results and discussion
Chemistry
The general synthesis of 8–11 is illustrated in Scheme 1. The amino-alcohols 1–3 were treated with aqueous 6 M HCl and benzene and dried by Dean-Stark distillation; the corresponding hydrochlorides formed were subsequently suspended in dry acetonitrile and treated with dimethylcarbamic or diethylcarbamic chloride to furnish the aminocarbamates 4–7. Afterwards, intermediates 4–7 were added to the 3,4-dihydroxybenzoic acid, pre-activated by 12 h treatment with N,N′-bis(4-methylphenyl)carbodiimide in dry acetonitrile at 40 °C; the suspension was stirred at 40 °C for additional 24–72 h. The solvent was then removed and the residue was extracted with 3–4 small portions of cold methanol to furnish pure carbamates 8–11 as solids. The IR, 1H and Citation13C NMR spectroscopic data of compounds 4–11 are in agreement with proposed structures.
Scheme 1. Reagents and conditions: (a): 6 M HCl, benzene, Dean-Stark distillation; (b): R2NCOCl, CH3CN, reflux, 12 h; (c): N,N′-bis(4-methylphenyl)carbodiimide, CH3CN, 40 °C, 12 h; (d): 4–7, CH3CN, 40 °C, 24–72 h.
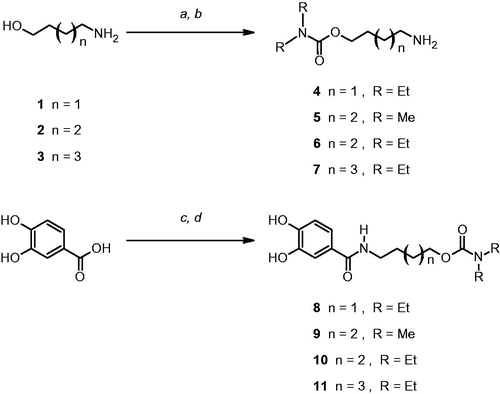
The antioxidant activity of carbamates 8–11 was evaluated using the DPPH method that allows to assess the ability of the compounds to scavenge the stable DPPH radicalCitation17; this capability is expressed as EC50 (moles of antioxidant per mole of DPPH needed to reduce to 50% the initial DPPH); lower EC50 values indicate better antioxidant properties. The obtained data are summarized in .
Table 1. Antioxidant EC50 values of compounds 8--11 obtained with DPPH method.
The obtained EC50 values range from 0.093 for compound 8 to 0.118 for compound 11 and are lower than the hydrophylic tocopherol derivative Trolox (EC50 = 0.150), thus indicating the good radical scavenging and antioxidant properties of catecholic derivatives 8–11.
The chelation abilities of 8–11 towards bio-metal cations such as Fe+3, Fe+2, Cu+2 and Zn+2 were investigated by Job’s methodCitation18,Citation19 based on the changes in the UV-Vis absorption spectra due to the complex formation. The results of complexation studies are summarized in and indicate that all compounds are able to form metal complex with Fe+3, Fe+2 and Cu+2 (Figures S1–S12 Supplementary material). The major changes in the UV-Vis spectra of carbamates 8–11 were observed in presence of Fe3+, which leads to the highest values. By the Job’s plot (ΔA versus molar ratio of ligand) the stoichiometry of the complex can be derived (); in some cases three molecules of catechol derivative are needed per each Fe3+ cation, thus indicating that a single molecule of carbamate can form a bidentate coordination with the iron (III). Otherwise, reduced changes were recorded for Fe2+ and Cu2+ complex and some differences in complex stoichiometry were also observed. Compound 10 produced a noticeable turbidity in presence of Fe+3, Fe+2 and Cu+2, probably due to the formation of insoluble complexes, that could explain the lower ΔA values observed in the complexation studies of this compound. Negligible or no changes in the absorption spectra were recorded in the UV-Vis spectra of compounds 8–11 in presence of Zn2+.
Figure 1. (a) UV-Vis spectral changes obtained for Fe3+ in presence of increasing amounts of carbamate 11; (b) Job’s plot of ΔA observed at 269 and 309 nm versus the molar ratio of the carbamate.
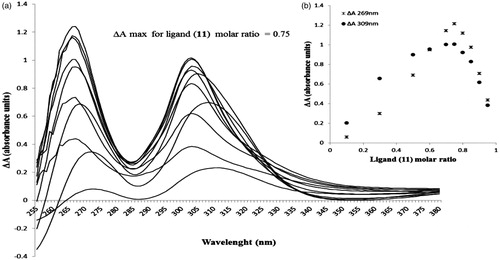
Table 2. Complexation of Fe+3, Fe+2, Cu+2 and Zn+2with carbamates 8--11.
Ellman spectrophotometric methodCitation20 was used to assess the ability of carbamates 8–11 to inhibit AChE and butyrylcholinesterase (BChE).
All synthesized compounds were found to inhibit AChE at low μM concentration (, Figures S13–S16 Supplementary material), in particular carbamate 9–11 showed mixed inhibition with Ki values, respectively, of 6.6, 18.9 and 1.5 μM, suggesting that these compounds could interact with peripheral anionic site (PAS) and the deepest catalytic site. Differently, the carbamate 8, endowed with an alkyl linker of four methylene units, showed a non-competitive inhibition with a Ki value of 5.8 μM suggesting that it could interact exclusively with PAS. All the studied compounds resulted weak inhibitors of BChE, underlining their high selectivity towards the AChE.
Table 3. Anticholinesterase activity of carbamates 8–11.
To support the experimental results obtained by enzymatic inhibition, we carried out a molecular docking study using SwissDock freely available on Swiss Institute of Bioinformatics WebsiteCitation21,Citation22. Three-dimensional crystal structures of Torpedo californica AChE (TcAChE) complexed with donepezil and of human BChE (hBChE) were obtained by RCSB Protein Data Bank (PDB codes: 1EVE for AChE; 1P0I for BChE). The protein preparation and the molecular docking results visualization were carried out using the UCSF Chimera packageCitation23.
The complex 1EVE has been chosen for the high degree of homology with Electric eel AChE used in the enzymatic inhibition tests. The results of the TcAChE docking study led to the hypothesis that the carbamates 8–11 could bind the enzyme active site; the binding conformations resulted within the two highest FF score clusters. The binding poses were found very similar for all compounds (, Figures S18–S21 Supplementary material): the catechol moieties were found in the enzyme active site, interacting mainly with Trp84 and with Ser200 or Glu199; the 4--6 methylene alkyl chains are close to aromatic aminoacid side chains of the enzyme gorge (Phe330 and 331); finally, the carbamic function was found in proximity of the PAS aminoacids (Trp279, Tyr70 and Tyr121).
Figure 2. Docking conformations of carbamates 8 (yellow), 9 (blue), 10 (red) and 11 (green) in the active site of TcAChE (1EVE). The selected aminoacids are dark cyan colored.
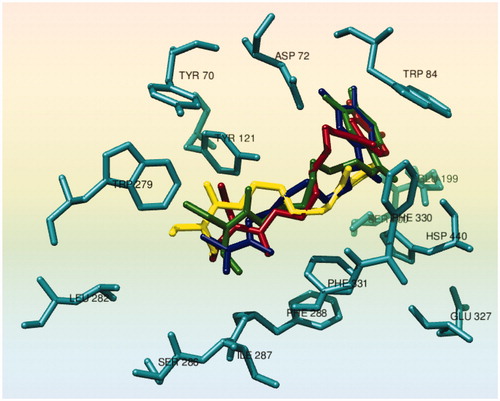
In the case of BChE, the docking study is more difficult, indeed most of the crystal structures available in the PDB were complexes with irreversible inhibitors, such as organophosphorus compounds. Then, we decided to use the crystal 1P0I on the basis of informations from the literatureCitation24. All compounds formed π–π interactions with Trp82 and/or hydrogen bond with Glu197. The conformations of 8–11 are slightly bent, not linear, and the fit of these molecules is not satisfying as in the case of AChE, confirming the high selectivity against AChE demonstrated by the enzymatic assay. In particular, all compounds do not interact with the PAS residues (Trp 231, Ala 277, Gln 119, Gln 71 -- Figures S22--S25 Supplementary material).
In conclusion, we have designed and synthesized the carbamates 8–11 possessing good antioxidant and radical scavenging properties, as showed by EC50 values lower than trolox. These compounds are also able to chelate the bio-metal cations Cu (II), Fe (II) and especially Fe (III). Interestingly, 8–11 also resulted highly selective non-competitive or mixed inhibitors of AChE, characterized by low μM Ki values. Based on the molecular docking study, the carbamates 8–11 could inhibit the AChE by interaction with active catalytic site, access gorge and PAS. Moreover, the reduced activity towards BChE could be explained by the weak interactions with PAS aminoacid residues.
For these reasons, carbamates 8–11 can be considered interesting lead compounds in the development of new multifactorial agents potentially useful in the treatment of neurodegenerative disorders that typically possess a complex multifactorial etiology.
Supplementary material available online
Supplementary Figures S1–S25.
supplemental_material_866657.docx
Download MS Word (4.5 MB)Declaration of interest
The authors report no declarations of interest.
This research was financially supported by grants from Sapienza, Università di Roma – Progetti di Ricerca di Ateneo, and by Ministero dell’Istruzione, dell’Università e della Ricerca (MIUR).
References
- Myhre O, Utkilen H, Duale N, et al. Metal dyshomeostasis and inflammation in Alzheimer's and Parkinson's diseases: possible impact of environmental exposures. Oxid Med Cell Longev 2013; 2013:art. no. 726954
- Kozlowski H, Luczkowski M, Remelli M, Valensin D. Copper, zinc and iron in neurodegenerative diseases (Alzheimer's, Parkinson's and prion diseases). Coordin Chem Rev 2012;256:2129–41
- Jomova K, Vondrakova D, Lawson M, Valko M. Metals, oxidative stress and neurodegenerative disorders. Mol Cell Biochem 2010;345:91–104
- Molina-Holgado F, Hider RC, Gaeta A, et al. Metals ions and neurodegeneration. Biometals 2007;20:639–54
- Ward RJ, Dexter DT, Crichton RR. Chelating agents for neurodegenerative diseases. Curr Med Chem 2012;19:2760–72
- Mao F, Huang L, Luo Z, et al. O-Hydroxyl- or o-amino benzylamine-tacrine hybrids: multifunctional biometals chelators, antioxidants, and inhibitors of cholinesterase activity and amyloid-β aggregation. Bioorg Med Chem 2012;20:5884–92
- Fernandez-Bachiller MI, Perez C, Campillo NE, et al. Tacrine–melatonin hybrids as multifunctional agents for Alzheimer’s disease, with cholinergic, antioxidant, and neuroprotective properties. ChemMedChem 2009;4:828–41
- Fernández-Bachiller MI, Pérez C, Monjas L, et al. New tacrine-4-Oxo-4 H-chromene hybrids as multifunctional agents for the treatment of Alzheimer’s disease, with cholinergic, antioxidant, and β-amyloid-reducing properties. J Med Chem 2012;55:1303–17
- Leon R, Garcia AG, Marco-Contelles J. Recent advances in the multitarget-directed ligands approach for the treatment of Alzheimer’s disease. Med Res Rev 2013;33:139--89
- Van der Schyf CJ. The use of multi-target drugs in the treatment of neurodegenerative diseases. Expert Rev Clin Pharmacol 2011;4:293–8
- Youdim MBH, Buccafusco JJ. CNS targets for multi-functional drugs in the treatment of Alzheimer’s and Parkinson’s diseases. J Neural Transm 2005;112:519–37
- Ramassamy C. Emerging role of polyphenolic compounds in the treatment of neurodegenerative diseases: a review of their intracellular targets. Eur J Pharmacol 2006;545:51–64
- Ordoudi SA, Tsimidou MZ, Vafiadis AP, Bakalbassis EG. Structure-DPPH• scavenging activity relationships: parallel study of catechol and guaiacol acid derivatives. J Agric Food Chem 2006;54:5763–8
- Mandel S, Amit T, Bar-Am O, Youdim MBH. Iron dysregulation in Alzheimer's disease: multimodal brain permeable iron chelating drugs, possessing neuroprotective-neurorescue and amyloid precursor protein-processing regulatory activities as therapeutic agents. Prog Neurobiol 2007;82:348–60
- Schliebs R, Arendt T. The cholinergic system in aging and neuronal degeneration. Behav Brain Res 2011;221:555–63
- Anand P, Singh B. A review on cholinesterase inhibitors for Alzheimer's disease. Arch Pharm Res 2013;36:375–99
- Brand-Williams W, Cuvelier ME, Berset C. Use of a free radical method to evaluate antioxidant activity. LWT Food Sci Technol 1995;28:25–30
- Vosburgh WC, Cooper GR. Complex ions. I. The identification of complex ions in solution by spectrophotometric measurements. J Am Chem Soc 1941;63:437–42
- Foley RT, Anderson RC. Spectrophotometric studies on complex formation with sulfosalicylic acid. I. With iron III. J Am Chem Soc 1948;70:1195–7
- Ellman GL, Courtney KD, Andres V, Featherstone RM. A new and rapid colorimetric determination of acetylcholinesterase activity. Biochem Pharmacol 1961;7:88–90
- Grosdidier A, Zoete V, Michielin O. SwissDock, a protein-small molecule docking web service based on EADock DSS. Nucleic Acids Res 2011;39:W270–7
- Zoete V, Cuendet MA, Grosdidier A, Michielin O. SwissParam: a fast force field generation tool for small organic molecules. J Comput Chem 2011;32:2359–68
- Pettersen EF, Goddard TD, Huang CC, et al. UCSF Chimera – a visualization system for exploratory research and analysis. J Comput Chem 2004;25:1605–12
- Cappelli A, Gallelli A, Manini M, et al. Further studies on the interaction of the 5-hydroxytryptamine3 (5-HT3) receptor with arylpiperazine ligands. Development of a new 5-HT3 receptor ligand showing potent acetylcholinesterase inhibitory properties. J Med Chem 2005;48:3564–75
- Alcaro S, Arcone R, Costa G, et al. Simple choline esters as potential anti-alzheimer agents. Curr Pharm Des 2010;16:692–7