Abstract
A series of novel pyrazole-based chalcones have been designed, synthesized from 1-methyl-5-(2,4,6-trimethoxyphenyl)-1H-pyrazole (6). The structures of regioisomers 6 and 7 were determined by 2D 1H–1H COSY, 1H–13C HSQC and 1H–13C HMBC experiments. The newly synthesized compounds were tested for their inhibitory activity against COX-1 and COX-2 using an in vitro cyclooxygenase (COX) inhibition assay. Moreover, they were investigated in vivo for their anti-inflammatory activities using carrageenan-induced rat paw edema model for acute inflammation and cotton pellet-induced granuloma model for chronic inflammation. All the synthesized compounds showed potential to demonstrate anti-inflammatory activities, of particular interest compounds 10i, 10e, 10f, and 10h were found to be potent anti-inflammatory agents.
Introduction
Fast and effective relief of pain and inflammation in human beings is continued to be a major challenge for medical researchers. Non-steroidal anti-inflammatory drugs (NSAIDs) are important therapeutic agents for the alleviation of pain and inflammation associated with a number of pathological conditionsCitation1. A major mechanism of action of NSAIDs is lowering prostaglandin (PG) production through the inhibition of cyclooxygenase (COX); a key enzyme in PGs biosynthesis that catalyses the conversion of arachidonic acid into PGs and thromboxanesCitation2. It was discovered that COX exists in two isoforms COX-1 and COX-2 which are regulated differently. COX-1 is a constitutive isoform; responsible for the production of cytoprotective prostaglandins in the gastrointestinal tract (GI) and proaggregatory thromboxane in blood platelets. However, COX-2 is an inducible isoform which is induced in response to the release of several proinflammatory mediatorsCitation3–5.
Most of the classical NSAIDs such as aspirin act as nonselective inhibitors of COX, inhibiting both the COX-1 and COX-2. The chronic use of NSAIDs to treat pain and inflammation is often accompanied by side effects such as gastric ulceration, bleeding and renal impairment. It is believed that the COX-2 selective inhibitors will greatly reduce these side effectsCitation6–8. Thus, the development of COX-2 selective inhibitors has led attention to improve the therapeutic potency and to reduce the classical side effects associated the use of conventional NSAIDs.
Pyrazole are an important class of compounds for new drug development that attracted much attention due to their broad spectrum of biological activities, such as anti-inflammatoryCitation9–14, antifungalCitation15, anticancerCitation16–19, and antiviral activitiesCitation20,Citation21. Pyrazole derivatives also acting as anti-angiogenic agentsCitation22, A3 adenosine receptor antagonistsCitation23, neuropeptide YY5 receptor antagonistsCitation24, kinase inhibitor for the treatment of type 2 diabetes, hyperlipidemia, obesityCitation25, and thrombopiotinmimeticsCitation26 were reported. Recently, urea derivatives of pyrazoles have been reported as potent inhibitors of p38 kinaseCitation27.
Among the highly marketed COX-2 inhibitors that comprise the pyrazole nucleus, celecoxib is the one which is treated as a safe anti-inflammatory and analgesic agent. It is considered as a typical model of the diaryl heterocyclic template that is known to selectively inhibit the COX-2 enzyme. Some other examples of pyrazole derivatives such as deracoxib, SC-558, mefobutazone, ramifenazone, famprofazone () have been reported as potent NSAIDsCitation28–31. On the other hand, the compounds with the backbone of chalcones have been reported to possess various biological activities, including anti-inflammatory, antimicrobial, antifungal, antioxidant, and anticancer activitiesCitation32,Citation33. Various methoxylated or hydroxylated chalcones, natural and synthetic, have already been tested for their possible role as anti-inflammatory agentsCitation34,Citation35. Recently, we have also reported some simple methoxylated chalcones and N-phenyl pyrazole chalcones showed significant anti-inflammatory, antioxidant and antimicrobial activitiesCitation36,Citation37.
The combination of both the active scaffolds, pyrazole and chalcone, may provide synergistic effect to improve the anti-inflammatory activity. These predictions encouraged us to synthesize hybrid compounds containing arylpyrazole combined with chalcone scaffold. Based on the earlier findings and in continuation of our interest in the synthesis of novel bioactive molecules with potential anti-inflammatory activitiesCitation38–41, a new series of pyrazolyl chalcones (10a–n) have been synthesized and evaluated for their anti-inflammatory potential.
Experimental
5-(2,4,6-Trimethoxyphenyl)-1H-pyrazole (5)
Ethyl formate (2.96 g, 3.21 ml, 30 mmol) was added to a suspension of sodium hydride (60% in oil, 0.8 g, 20 mmol) in tetrahydrofuran (3 ml) at room temperature. After stirring for 10 min, a solution of 1-(2,4,6-trimethoxyphenyl)-ethanone (3) (2.1 g, 10 mmol) in THF (15 ml) was added, and the mixture was stirred for 1 h. To the reaction mixture 1 M HCl (20 ml) was added, and extracted with diethyl ether (3 × 25 ml). Organic layer was concentrated under reduced pressure. Hydrate (5 g, 4.84 ml, 100 mmol) was added to this hydrazine, and the mixture was stirred for 30 min. The reaction mixture was made alkaline by adding 6N NaOH (20 ml) and extracted with ethyl acetate. The organic layer was washed with brine, dried over anhydrous magnesium sulfate, evaporated in vacuo, and recrystallized from ethanol to obtain colorless crystals of title compound 5.
Yield: 85%, MF/FWt: C12H14N2O3/234; m.p.: 138–140 °C; IR (KBr, cm−1): 3293 (NH), 3018, 2969, 2939 (CH), 1607, 1586, 1451 (C=C), 1204(C–O); 1H NMR (400 MHz, CDCl3): δ 3.85 (s, 3H, OCH3), 3.92 (s, 6H, OCH3), 6.22 (s, 2H, ArH), 6.82 (d, 1H, J = 1.6 Hz, C=CH), 7.6 (d, 1H, J = 1.6 Hz, N=CH), 11.38 (bs, 1H, NH); 13C NMR (100 MHz, CDCl3): 55.10 (CH3), 55.66 (CH3), 90.25 (CH), 100.38 (C), 106.98 (CH), 135.05 (C), 137.42 (CH), 158.90 (C), 162.20 (C); MS (ESI): m/e 235 (M + 1).
1-Methyl-5-(2,4,6-trimethoxyphenyl)-1H-pyrazole (6)
5-(2,4,6-Trimethoxyphenyl)-1H-pyrazole 5 (2.34 g, 10 mmol) was dissolved in dry DMF (15 ml) under N2 atmosphere. The flask was cooled in an ice bath and methyl iodide (2.84 g, 1.25 ml, 20 mmol) was added to it. To this solution, sodium hydride (60% in oil, 0.48 g, 12 mmol) was added in portions and the resulted solution was then allowed to stir at 0 °C for 15 min. The reaction mixture was poured over crushed ice and the resulted solid was filtered off, recrystallized from ethanol to afford the title compound 6 in pure form. The filtrate was then extracted three times with ethyl acetate. The combined extracts were washed with water. After drying over anhydrous MgSO4, the solvent was distilled off under reduced pressure. The resulting residue was purified by silica gel column chromatography to obtain compound 7 in pure form.
Yield: 82%, MF/FWt: C13H16N2O3/248; m.p.: 147–149 °C; IR (KBr, cm−1): 3064, 2962, 2943, 2841 (CH), 1612, 1584, 1549, 1473, 1458 (C=C), 1234 (C=N), 1161 (C–O); 1H NMR (500 MHz, CDCl3): δ 3.65 (s, 3H, NCH3), 3.75 (s, 6H, 2 × OCH3), 3.87 (s, 3H, OCH3), 6.20 (m, 3H, 2 × ArH, Pyr-H), 7.55 (d, 1H, Pyr-H); 13C NMR (125 MHz, CDCl3): 36.65 (CH3), 55.39 (CH3), 55.74 (CH3), 90.54 (CH), 100.68 (C), 107.44 (CH), 135.51 (C), 137.90 (CH), 159.32 (C), 162.25 (C); 135-DEPT: 36.65 (+), 55.40 (+), 55.75 (+), 90.53 (+), 107.44 (+), 137.89 (+); MS (ESI): m/e 249 (M+1).
1-Methyl-3-(2,4,6-trimethoxyphenyl)-1H-pyrazole (7)
Yield: 18%, MF/FWt: C13H16N2O3/248; m.p.: 104–106 °C; IR (KBr, cm−1): 3130, 2992, 2956, 2834 (CH), 1613, 1586, 1505, 1474 (C=C), 1224 (C=N), 1161 (C–O); 1H NMR (400 MHz, CDCl3): δ 3.76 (s, 6H, 2 × OCH3), 3.85 (s, 3H, OCH3), 3.97 (s, 3H, NCH3), 6.20 (s, 2H, 2 × ArH), 6.31 (d, J = 2.8 Hz, 1H, Pyr-H), 7.41 (d, J = 2.8 Hz, 1H, Pyr-H); 13C NMR (100 MHz, CDCl3): 38.94 (CH3), 55.25 (CH3), 55.92 (CH3), 90.92 (CH), 104.42 (C), 107.82 (CH), 130.12 (CH), 144.42 (C), 159.38 (C), 161.08 (C); 135-DEPT: 38.94 (+), 55.28 (+), 55.93 (+), 90.56 (+), 107.81 (+), 130.11 (+); MS (ESI): m/e 249 (M + 1).
1-[2-Hydroxy-4,6-dimethoxy-3-(2-methyl-2H-pyrazol-3-yl)-phenyl]-ethanone (8)
Compound 6 (2.48 g, 10 mmol) was dissolved in 25 ml of dry CH2Cl2 under N2 atmosphere and flask was cooled in an ice bath. To this solution, BF3.OEt2 (11.36 g, 10.05 ml, 80 mmol) was added followed by a dropwise addition of acetic anhydride (5.1 g, 4.72 ml, 50 mmol). The resulted solution was then allowed to stir for 24 h at room temperature. The reaction mixture was diluted with water, rendered alkaline with Na2CO3 and extracted with CH2Cl2. Removal of solvent in vacuo gave white solid that was recrystallized from methanol to obtain compound 8 in pure form.
Yield: 95%; MF/FWt: C14H16N2O4/276; m.p.: 221–223 °C; IR (KBr, cm−1): 3461 (OH), 2940, 2370 (CH), 1625 (CO), 1597, 1416 (C=C), 1277 (C=N), 1133 (C–O); 1H NMR (400 MHz, CDCl3): δ 2.69 (s, 3H, COCH3), 3.71 (s, 3H, NCH3), 3.88 (s, 3H, OCH3), 4.02 (s, 3H, OCH3), 6.08 (s, 1H, ArH), 6.26 (d, 1H, J = 1.6 Hz, C=CH), 7.59 (d, 1H, J = 1.6 Hz, N=CH); 13C NMR (100 MHz, CDCl3): 36.72 (COCH3), 38.80 (NCH3), 56.10 (OCH3), 56.45(OCH3), 90.60 (CH), 101.15 (C), 103.05 (C), 107.55 (CH), 138.22 (CH=N), 140.20 (C), 162.50 (C), 165.92 (C), 203.50 (CO); MS (ESI): m/e 277 (M + 1).
General procedure for the preparation of pyrazole-based chalcones (10a–n)
1-(2-Hydroxy-4,6-dimethoxy-3-(1-methyl-1H-pyrazol-5-yl)phenyl)ethanone (8) (0.276 g, 1 mmol) was dissolved in ethanol (10 ml) under stirring. To this NaOH (0.12 g; 3 mmol, with a minimum of water) was added and stirred for 5 min. To this reaction mixture, aromatic aldehydes 9a–n (1.2 mmol) was then added and stirring continued at room temperature for 24 h. Reaction was monitored by TLC. After completion of reaction, the mixture was poured over crushed ice and acidified with acetic acid. The separated solid was filtered and washed well with water. Crude product was dried and recrystallized from ethanol to obtain the desired product in pure form.
(E)-1-(2-hydroxy-4,6-dimethoxy-3-(1-methyl-1H-pyrazol-5-yl)phenyl)-3-phenylprop-2-en-1-one (10a)
Yield: 98%; MF/FWt: C21H20N2O4/364; m.p.: 178–180 °C; IR (KBr, cm−1): 3439 (OH), 2986, 2944, 2344 (CH), 1628 (CO), 1578, 1556 (C=C), 1335 (C=N), 799, 758; 1H NMR (400 MHz, DMSO-d6): δ 3.55 (s, 3H, NCH3), 3.89 (s, 3H, OCH3), 4.08 (s, 3H, OCH3), 6.15 (s, 1H, ArH), 6.43 (d, 1H, J = 1.6 Hz, Pyr-H), 7.41 (d, 1H, J = 1.6 Hz, Pyr-H), 7.47–7.48 (m, 3H, ArH), 7.76–7.77 (m, 3H, 2 × ArH, CH=CHCO), 7.91 (d, 1H, J = 15.6 Hz, CH=CHCO), 14.O3 (bs, 1H, OH); 13C NMR (100 MHz, CDCl3): 36.70 (NCH3), 56.32 (OCH3), 56.60 (OCH3), 91.05 (CH), 102.02 (C), 102.95 (C), 107.64 (CH), 127.50 (COCH), 127.90 (CH), 128.56 (2 × CH), 128.65 (2 × CH), 135.25 (C), 138.28 (CH=N), 140.33 (C), 145.35 (COCH=CH), 162.80 (C), 164.44 (C), 166.08 (C), 193.70 (CO); MS (ESI): m/e 365 (M + 1)
(E)-3-(4-fluorophenyl)-1-(2-hydroxy-4,6-dimethoxy-3-(1-methyl-1H-pyrazol-5-yl)phenyl)prop-2-en-1-one (10b)
Yield: 96%; MF/FWt: C21H19N2O4F/382; m.p.: 200–202 °C; IR (KBr, cm−1): 3447 (OH), 2944, 2372 (CH), 1635 (CO), 1600, 1553, 1479 (C=C), 1237 (C=N), 1126 (C–O); 1H NMR (400 MHz, DMSO-d6): δ 3.54 (s, 3H, NCH3), 3.86 (s, 3H, OCH3), 4.07 (s, 3H, OCH3), 6.16 (s, 1H, Ar-H), 6.41 (d, J = 1.6 Hz, 1H, Pyr-H), 7.25–7.30 (m, 2H, Ar-H), 7.41 (d, J = 1.6 Hz, Pyr-H), 7.76 (d, J = 16 Hz, 1H, CH=CHCO), 7.80–7.88 (m, 3H, 2 × Ar-H, CH=CHCO), 14.01 (bs, 1H, OH); 13C NMR (100 MHz, CDCl3): 36.65 (NCH3), 56.02 (OCH3), 56.58 (OCH3), 91.00 (CH), 102.05 (C–CO), 102.96 (C), 107.67 (CH), 117.58 (2 × CH), 127.60 (COCH), 130.84 (2 × CH), 130.95 (C), 138.12 (CH=N), 140.04 (C), 146.43 (COCH=CH), 147.23 (C), 162.78(C), 164.23 (C), 166.15 (C), 193.76 (CO); MS (ESI): m/e 383 (M + 1)
(E)-3-(2,4-difluorophenyl)-1-(2-hydroxy-4,6-dimethoxy-3-(1-methyl-1H-pyrazol-5-yl)phenyl)prop-2-en-1-one (10c)
Yield: 95%; MF/FWt: C21H18N2O4F2/400; m.p.: 198–200 °C; IR (KBr, cm−1): 3684 (OH), 3020, 2977, 2400 (CH), 1615 (CO), 1587, 1566, 1501 (C=C), 1215 (C=N), 767, 669; 1H NMR (400 MHz, DMSO-d6): δ 3.55 (s, 3H, NCH3), 3.89 (s, 3H, OCH3), 4.01 (s, 3H, OCH3), 6.15 (s, 1H, ArH), 6.43 (d, 1H, J = 1.7 Hz, Pyr-H), 7.23 (d, 1H, J = 1.7 Hz, Pyr-H), 7.42–7.44 (m, 2H, ArH, CH=CHCO), 7.73 (d, 1H, J = 15.7 Hz, CH=CHCO), 7.93–7.99 (m, 2H, ArH), 13.91 (bs,1H, OH); 13C NMR (100 MHz, CDCl3): 36.68 (NCH3), 56.05 (OCH3), 56.68 (OCH3), 91.01 (CH), 102.05 (C), 102.97 (C), 107.65 (CH), 113.0 (CH), 115.40 (CH), 118.7 (C), 122.30 (COCH), 130.08 (CH), 138.47 (CH=N), 140.66 (C), 145.28 (COCH=CH), 148.45 (C), 149.33 (C), 162.81 (C), 164.39 (C), 166.35 (C), 193.75 (CO); MS (ESI): m/e 400 (M+).
(E)-3-(2,4-dichlorophenyl)-1-(2-hydroxy-4,6-dimethoxy-3-(1-methyl-1H-pyrazol-5-yl)phenyl)prop-2-en-1-one (10d)
Yield: 97%; MF/FWt: C21H18N2O4Cl2/433; m.p.: 215–217 °C; IR (KBr, cm−1): 3446 (OH), 2925, 2344 (CH), 1623 (CO), 1583, 1565, 1469(C=C), 1327 (C=N), 1123 (C–O), 870; 1H NMR (400 MHz, DMSO-d6): δ 3.55 (s, 3H, NCH3), 3.86 (s, 3H, OCH3), 4.01 (s, 3H, OCH3), 6.11 (s, 1H, ArH), 6.33 (d, 1H, J = 1.7 Hz, Pyr-H), 7.38 (s, 1H, ArH), 7.52 (d, 1H, J = 8.4 Hz, ArH), 7.76 (d, 1H, J = 1.7 Hz, Pyr-H), 7.83–7.86 (m, 2H, CH=CHCO), 7.95 (d, 1H, J = 8.4 Hz, ArH); 13C NMR (100 MHz, CDCl3):06 (OCH3), 56.61 (OCH3), 91.02 (CH), 102.11 (C), 102.85 (C), 107.66 (CH), 121.88 (COCH), 125.08 (C), 126.80 (CH), 129.11 (CH), 130.06 (CH), 131.18 (C), 137.28 (C), 138.64 (CH=N), 140.70 (C), 146.01 (COCH=CH), 162.0 (C), 164.46 (C), 166.83 (C), 193.80 (CO); MS (ESI): m/e 433 (M+).
(E)-3-(3,4-dimethoxyphenyl)-1-(2-hydroxy-4,6-dimethoxy-3-(1-methyl-1H-pyrazol-5-yl)phenyl)prop-2-en-1-one (10e)
Yield: 98%; MF/FWt: C23H24N2O6/424; m.p.: 220–222 °C; IR (KBr, cm−1): 3685 (OH), 3020, 2974, 2400 (CH), 1618 (CO), 1587, 1560, 1511 (C=C), 1215 (C=N), 758, 669; 1H NMR (400 MHz, DMSO-d6): δ 3.55 (s, 3H, NCH3), 3.82 (s, 6H, 2 × OCH3), 3.86 (s, 3H, OCH3), 4.04 (s, 3H, OCH3), 6.14 (s, 1H, ArH),6.41 (d, 1H, J = 1.7 Hz, Pyr-H), 7.05 (d, 1H, J = 8.7 Hz, ArH), 7.32–7.40 (m, 2H, Pyr-H, ArH), 7.51 (s, 1H, ArH), 7.71 (d, 1H, J = 16 Hz, CH=CHCO), 7.80 (d, 1H, J = 16 Hz, CH=CHCO), 14.14 (bs, 1H, OH); 13C NMR (100 MHz, CDCl3): 36.66 (NCH3), 56.05 (OCH3), 56.15 (OCH3), 56.18 (OCH3), 56.60 (OCH3), 90.89 (CH), 102.12 (C), 102.81 (C), 107.64 (CH), 110.67 (CH), 110.92 (CH), 122.55 (CH), 127.33 (CH), 127.67 (COCH), 138.15 (CH=N), 140.52 (C), 145.30 (COCH=CH), 150.13 (C), 150.78 (C), 162.68 (C), 164.40 (C), 166.30 (C), 193.80 (CO); MS (ESI): m/e 425 (M + 1).
(E)-3-(3,4-difluorophenyl)-1-(2-hydroxy-4,6-dimethoxy-3-(1-methyl-1H-pyrazol-5-yl)phenyl)prop-2-en-1-one (10f)
Yield: 94%; MF/FWt: C21H18N2O4F2/400; m.p.: 198–200 °C; IR (KBr, cm−1): 3685 (OH), 3020, 2400 (CH), 1615 (CO), 1587, 1515 (C=C), 1215 (C=N), 770, 669; 1H NMR (300 MHz, CDCl3): δ 3.72 (s, 3H, NCH3), 3.90 (s, 3H, OCH3), 4.10 (s, 3H, OCH3), 6.12 (s, 1H, ArH), 6.28 (s, 1H, Pyr-H), 7.20 (dd, 1H, J = 8 Hz, ArH), 7.30–7.35 (d, 1H, J = 8 Hz, ArH), 7.43 (t, 1H, J = 8 Hz, ArH), 7.58 (s, 1H, Pyr-H), 7.69 (d, 1H, J = 16 Hz, CH=CHCO), 7.79 (d, 1H, J = 16 Hz, CH=CHCO), 14.25 (bs, 1H, OH); 13C NMR (100 MHz, CDCl3): 36.66 (NCH3), 56.05 (OCH3), 56.59 (OCH3), 91.01 (CH), 102.06 (C), 102.76 (C), 107.65 (CH), 113.81 (CH), 125.01 (CH), 126.20 (CH), 127.78 (COCH), 133.40 (C), 138.16 (CH=N), 140.50 (C), 145.18 (COCH=CH), 145.92 (C), 146.75 (C), 162.58 (C),164.02 (C), 165.99 (C), 193.62 (CO); MS (ESI): m/e 401 (M + 1).
(E)-3-(benzo[d][1,3]dioxol-5-yl)-1-(2-hydroxy-4,6-dimethoxy-3-(1-methyl-1H-pyrazol-5-yl)phenyl)prop-2-en-1-one (10 g)
Yield: 92%; MF/FWt: C22H20N2O6/408; MP: 178–179 °C; IR (KBr, cm−1): 3430 (OH), 2919 (CH), 1626 (CO), 1599, 1554, 1498, 1448 (C=C), 1248 (C=N), 1035 (C–O); 1H NMR (400 MHz, DMSO-d6): δ 3.54 (s, 3H, NCH3), 3.88 (s, 3H, OCH3), 4.06 (s, 3H, OCH3), 6.12 (s, 2H, O–CH2–O), 6.14 (s, 1H, ArH), 6.41 (d, 1H, J = 1.7 Hz, Pyr-H), 7.01 (d, 1H, J = 8.2 Hz, ArH), 7.28 (d, 1H, J = 8 Hz, ArH), 7.39–7.40 (m, 2H, Pyr-H, ArH), 7.71 (d, 1H, J = 15.5 Hz, CH=CHCO), 7.76 (d, 1H, J = 15.5 Hz, CH=CHCO); 13C NMR (100 MHz, CDCl3): 36.65 (NCH3), 56.03 (OCH3), 56.07 (OCH3), 91.02 (CH), 100.2 (OCH2O), 102.15 (C), 102.78 (C), 107.64 (CH), 108.0 (CH), 110.06 (CH), 122.53 (CH), 127.35 (C), 127.68 (COCH), 138.15 (CH=N), 140.52 (C), 145.32 (COCH=CH), 150.03 (C), 150.48 (C), 162.56 (C), 164.40 (C), 166.30 (C), 193.69 (CO); MS (ESI): m/e 409 (M + 1).
(E)-1-(2-hydroxy-4,6-dimethoxy-3-(1-methyl-1H-pyrazol-5-yl)phenyl)-3-(3-nitrophenyl)prop-2-en-1-one (10 h)
Yield: 98%; MF/FWt: C21H19N3O6/409; MP: 176–178 °C; IR (KBr, cm−1): 3683 (OH), 3020, 2946,2400 (CH), 1618 (CO), 1587, 1532(C=C), 1215 (C=N), 1128 (C–O), 757, 668; 1H NMR (400 MHz, DMSO-d6): δ 3.55 (s, 3H, NCH3), 3.87 (s, 3H, OCH3), 4.03 (s, 3H, OCH3), 6.13 (s, 1H, ArH), 6.37 (d,1H, J = 1.6 Hz, Pyr-H), 7.40 (d, 1H, J = 1.6 Hz, Pyr-H), 7.73–7.77 (m, 2H, ArH, CH=CHCO), 7.95 (d, 1H, J = 15 Hz, CH=CHCO), 8.22 (d, 1H, J = 7.3 Hz, ArH), 8.26 (d, 1H, J = 8.2 Hz, ArH), 8.53 (s, 1H, ArH), 14.1 (bs, 1H, OH); 13C NMR (100 MHz, CDCl3): 36.68 (NCH3), 56.11 (OCH3), 56.23 (OCH3), 91.17 (CH), 102.15 (C), 102.80 (C), 107.68 (CH), 125.07 (CH), 126.10 (CH), 127.81 (COCH), 130.26 (CH), 135.66 (CH), 138.0 (C), 138.20 (CH=N), 140.60 (C), 150.08 (C), 162.61 (C), 164.45 (C), 166.32 (C), 193.84 (CO); MS (ESI): m/e 410 (M + 1).
(E)-3-(furan-2-yl)-1-(2-hydroxy-4,6-dimethoxy-3-(1-methyl-1H-pyrazol-5-yl)phenyl)prop-2-en-1-one (10i)
Yield: 93%; MF/FWt: C19H18N2O5/354; MP: 220–222 °C; IR (KBr, cm−1): 3683 (OH), 3019, 2400 (CH), 1618 (CO), 1551, 1471, 1413 (C=C), 1216 (C=N), 768, 669; 1H NMR (400 MHz, DMSO-d6): δ 3.54 (s 3H, NCH3), 3.88 (s, 3H, OCH3), 4.05 (s, 3H, OCH3), 6.14 (s, 1H, Ar-H), 6.42 (d, 1H, J = 1.6 Hz, Pyr-H), 6.70 (s, 1H, Furan-H), 7.07 (s, 1H, Furan-H), 7.41 (d, 1H, J = 1.6 Hz, Pyr-H), 7.62 (d, 1H, J = 15.4 Hz, CH=CHCO), 7.70 (d, 1H, J = 15.4 Hz, CH=CHCO), 7.93 (s, 1H, Furan-H), 14.23 (bs, 1H, OH); 13C NMR (100 MHz, CDCl3): 36.65 (NCH3), 56.02 (OCH3), 56.09 (OCH3), 90.96 (CH), 102.10 (C), 102.95 (C), 107.64 (CH), 113.70 (CH), 115.08 (CH), 122.91 (COCH=CH), 127.63 (COCH), 138.12 (CH), 140.48 (C), 145.07 (CH), 152.58 (C), 162.48 (C), 164.04 (C), 166.0 (C), 193.63 (CO); MS (ESI): m/e 355 (M + 1).
(E)-1-(2-hydroxy-4,6-dimethoxy-3-(1-methyl-1H-pyrazol-5-yl)phenyl)-3-(thiophen-2-yl)prop-2-en-1-one (10j)
Yield: 94%; MF/FWt: C19H18N2O4S/370; MP: 192–194 °C; IR (KBr, cm−1): 3684 (OH), 3020, 2400 (CH), 1619 (CO), 1586, 1561, 1422 (C=C), 1215 (C=N), 770, 669; 1H NMR (400 MHz, DMSO-d6): δ 3.55 (s, 3H, NCH3), 3.88 (s, 3H, OCH3), 4.06 (s, 3H, OCH3), 6.14 (s, 1H, Ar-H), 6.42 (d, 1H, J = 1.54 Hz, Pyr-H), 7.20 (dd, 1H, J = 4.6, 3.8 Hz, Thiophene-H), 7.41 (d, 1H, J = 1.54 Hz, Pyr-H), 7.62–7.68 (m, 2H, CH=CHCO, Thiophene-H), 7.79 (d, 1H, J = 4.6 Hz, Thiophene-H), 7.95 (d, 1H, J = 15.3 Hz, CH=CHCO), 14.16 (bs, 1H, OH); 13C NMR (100 MHz, CDCl3): 36.65 (NCH3), 56.01 (OCH3), 56.06 (OCH3), 90.95 (CH), 102.08 (C), 102.90 (C), 107.64 (CH), 127.68 (COCH), 128.93 (CH), 129.55 (CH), 131.01 (CH), 136.72 (COCH=CH), 138.03 (CH=N), 140.30 (C), 140.58 (CH), 162.45 (C), 163.97 (C), 165.88 (C), 193.60 (CO); MS (ESI): m/e 371 (M + 1).
(E)-3-(4-bromophenyl)-1-(2-hydroxy-4,6-dimethoxy-3-(1-methyl-1H-pyrazol-5-yl)phenyl)prop-2-en-1-one (10k)
Yield: 97%; MF/FWt: C21H19N2O4Br/443; MP: 196–198 °C; IR (KBr, cm−1): 3446 (OH), 2925 (CH), 1623(CO), 1583, 1565, 1469 (C=C), 1327 (C=N), 1123 (C–O); 1H NMR (400 MHz, DMSO-d6): δ 3.70 (s, 3H, NCH3), 3.88 (s, 3H, OCH3), 4.05 (s, 3H, OCH3), 6.10 (s, 1H, ArH), 6.25 (d, J = 2 Hz, 1H, Pyr-H), 7.45 (d, J = 2 Hz, 1H, Pyr-H), 7.49–7.58 (m, 4H, ArH), 7.72 (d, J = 16 Hz, 1H, COCH=CH), 7.87 (d, J = 16 Hz, 1H, COCH=CH), 14.26 (s, 1H, OH); 13C NMR (100 MHz, CDCl3): 36.65 (NCH3), 55.95 (OCH3), 56.26 (OCH3), 90.87 (CH), 102.02 (C), 102.90 (C), 107.65 (CH), 123.23 (C), 127.56 (COCH), 128.95 (CH), 132.52 (CH), 135.20 (C), 138.08 (CH=N), 140.06 (C), 146.35 (COCH=CH), 162.67 (C), 163.95(C), 165.99 (C), 193.80 (CO); MS (ESI): m/e 444 (M + 1).
(E)-3 -(2,6-dichlorophenyl)-1-(2-hydroxy-4,6-dimethoxy-3-(1-methyl-1H-pyrazol-5-yl)phenyl)prop-2-en-1-one (10l)
Yield: 90%; MF/FWt: C21H18N2O4Cl2/433; MP: 212–214 °C; IR (KBr, cm−1): 3446 (OH), 2944 (CH), 1623 (CO), 1582, 1567, 1466 (C=C), 1208 (C=N), 1123 (C–O), 770; 1H NMR (400 MHz, DMSO-d6): δ 3.55 (s, 3H, NCH3), 3.83 (s, 3H, OCH3), 4.01 (s, 3H, OCH3), 6.15 (s, 1H, ArH), 6.41 (d, 1H, J = 1.7 Hz, Pyr-H), 7.36–7.46 (m, 2H, ArH, Pyr-H), 7.61 (d, 2H, J = 8 Hz, ArH), 7.76 (d, 1H, J = 16.4 Hz, CH=CHCO), 8.00 (d, 1H, J = 16.4 Hz, CH=CHCO), 13.82 (bs, 1H, OH); 13C NMR (100 MHz, CDCl3): 36.66 (NCH3), 56.12 (OCH3), 56.66 (OCH3), 91.08 (CH), 102.15 (CH), 102.86 (CH), 107.68 (CH=N), 122.50 (COCH), 126.84 (CH), 130.02 (2 × CH), 135.25 (2 × CH), 137.81 (COCH=CH), 138.11 (CH=N), 140.10 (CH), 145.37 (COCH=CH), 162.78 (C), 164.09 (C), 166.21 (C), 193.78 (CO); MS (ESI): m/e 433 (M+)
(E)-3-(2,6-difluorophenyl)-1-(2-hydroxy-4,6-dimethoxy-3-(1-methyl-1H-pyrazol-5-yl)phenyl)prop-2-en-1-one (10m)
Yield: 91%; MF/FWt: C21H18N2O4F2/400; MP: 194–196 °C; IR (KBr, cm−1): 3018, 2979, 2945 (CH), 1619 (CO), 1588, 1469, 1412 (C=C), 1214 (C=N), 1131 (C–O), 992, 788; 1H NMR (300 MHz, CDCl3): δ 3.72 (s, 3H, NCH3), 3.90 (s, 3H, OCH3), 4.08 (s, 3H, OCH3), 6.12 (s, 1H, ArH), 6.24 (s, 1H, Pyr-H), 6.88 (t, 1H, J = 8 Hz, ArH), 7.28–34 (m, 2H, ArH), 7.60 (s, 1H, Pyr-H), 7.85 (d, 1H, J = 16 Hz, CH=CHCO), 8.20 (d, 1H, J = 16 Hz, CH=CHCO), 14.25 (bs, 1H, OH); 13C NMR (100 MHz, CDCl3): 36.65 (NCH3), 56.06 (OCH3), 56.73 (OCH3), 91.09 (CH), 102.18 (C), 102.92 (C), 107.70 (CH), 112.96 (CH), 114.48 (CH), 122.48 (COCH), 130.10 (CH), 138.12 (CH=N), 140.16 (C), 145.63 (COCH=CH), 160.42 (C), 162.75 (C), 165.89 (C), 193.81 (CO); MS (ESI): m/e 401 (M + 1).
(E)-1-(2-hydroxy-4,6-dimethoxy-3-(1-methyl-1H-pyrazol-5-yl)phenyl)-3-(2,4,6-trimethoxyphenyl)prop-2-en-1-one (10n)
Yield: 90%; MF/FWt: C24H26N2O7/454; MP: 234–236 °C; IR (KBr, cm−1): 3684 (OH), 3019, 2942(CH), 1617(CO), 1551, 1467 (C=C), 1216 (C=N), 1128 (C–O), 769, 669; 1H NMR (400 MHz, DMSO-d6): δ 3.54 (s, 3H, NCH3), 3.87 (s, 6H, 2 × OCH3), 3.92 (s, 6H, 2 × OCH3), 4.06 (s, 3H, OCH3), 6.13 (d, 1H, J = 1.6 Hz, Pyr-H), 6.33 (s, 2H, ArH), 6.39 (s, 1H, ArH), 7.40 (d, 1H, J = 1.6 Hz, Pyr-H), 8.20 (d, 1H, J = 15.8 Hz, CH=CHCO), 8.25 (d, 1H, J = 15.8 Hz, CH=CHCO), 14.72 (bs, 1H, OH); 13C NMR (100 MHz, CDCl3): 36.63 (NCH3), 56.06 (OCH3), 56.37 (OCH3), 56.68 (OCH3), 56.73 (OCH3), 90.88 (CH), 92.75 (CH), 102.22 (C), 102.89 (C), 107.06 (CH), 107.66 (C), 122.50 (COCH), 123.42 (COCH=CH), 138.02 (CH=N), 140.0 (C), 158.01 (C), 159.69 (C), 162.60 (C), 163.87 (C), 165.92 (C), 193.52 (CO); MS (ESI): m/e 455 (M + 1).
Biological evaluation
In vitro COX inhibition assay
The assay was performed by using Colorimetric COX (ovine) inhibitor Screening assay kit (Catalogue No. 760111, Cayman Chemicals Co., Ann Arbor, MI)Citation42. Briefly, the reaction mixture contains, 150 µl of assay buffer, 10 µl of heme, 10 µl of enzyme (either COX-1 or COX-2), and 50 µl of sample (0.1 mM). The assay utilizes the peroxidase component of the COX catalytic domain. The peroxidase activity can be assayed colorimetrically by monitoring the appearance of oxidized N,N,N,N′-tetramethyl-p-phenylenediamine (TMPD) at 590 nm. Indomethacin (0.1 mM) was used as a standard drug. The percent COX inhibition was calculated using following equation:
where T is the absorbance of the inhibitor well at 590 nm, C is the absorbance of the 100% initial activity without inhibitor well at 590 nm.
Animals
Animals were assigned into several groups randomly and each group consists of six animals. The animals were kept in appropriate cages at temperature controlled (25 ± 2 °C) rooms, under a 12 h light and dark cycle and they were fed standard rodent pellet. All the animals were acclimatized for a week before the experiment. Experimental protocols were reviewed and approved by the Institutional Animal Ethics Committee (SIPS/IAEC/2011-12/17) and conform to the Indian National Science Academy Guidelines for the use and care of experimental animals in research. The animal house registration number with Government of India is 962/c/06/CPCSEA.
Carrageenan induced hind paw edema assay
Dose–response study
Rats were divided into various groups (n = 6) and allowed for free access to water ad libitum. Different groups of rats administered with indomethacin (100 mg/kg, b.w.) and various test compounds (100 mg/kg, v) orally. One group of rats served as a control and administered with gum acacia (1% (w/v); 10 ml/kg, b.w., p.o.). One hour after the drug administration, to all rats, hind paw edema was induced by the method of Winter et al.Citation43 by injecting 0.1 ml of 1% (w/v) solution of carrageenan subcutaneously into the subplanter region of hind paw.
Time course study
The hind paw edema volume was measured by volume displacement method using plethysmometer (UGO, Besile 7140, Italy) by immersing the paw till the level lateral malleolus at various time intervals (1, 3 and 6 h) after carrageenan injection.
Cotton pellets induced granuloma assay
Two sterilized cotton pellets (10 mg) were implanted on ventral regions of rats, procedure described by winter and porterCitation44 and divided into various four groups (six rats/group). Different groups of rats administered with indomethacin (100 mg/kg), compound 10e, 10f, 10h, and 10i (100 mg/kg) orally respectively for the duration of 8 d. Control group received gum acacia (2%, 10 ml/kg, p.o.). On the day 9, rats were sacrificed with excess ether anesthesia. The cotton pellets were removed and freed from extraneous tissue and used for granular tissue formation by recording wet (immediately) and dry weight of pellets. The granular tissue formation was studied by drying cotton pellets at 55 °C for 6 h or till the weight of pellets remains constant. The dry weight was calculated after deducting cotton pellet weight and taken as a granular tissue formation.
Results and discussion
Chemistry
Synthesis of target compounds 10a–n was achieved by the Claisen–Schmidt condensation of 1-(2-hydroxy-4,6-dimethoxy-3-(1-methyl-1H-pyrazol-5-yl)phenyl)ethanone 8 with various aromatic/heteroaromatic aldehydes 9a–n in the presence of NaOH in ethanol at room temperature in good to excellent yields (Scheme 1). 1-(2-Hydroxy-4,6-dimethoxy-3-(1-methyl-1H-pyrazol-5-yl)phenyl)ethanone 8 was readily prepared by the Friedel–Craft acetylation of key intermediate 1-methyl-5-(2,4,6-trimethoxyphenyl)-1H-pyrazole 6 by using acetic anhydride in the presence of boron trifluoride etherate under nitrogen atmosphere.
The regioselective synthesis of key intermediate 1-methyl-5-(2,4,6-trimethoxyphenyl)-1H-pyrazole 6 was carried out using the synthetic strategies illustrated in Scheme 2. Xanthoxyline 2 was synthesized by the Friedel–Craft acetylation of 1,3,5-trimethoxybenzene 1 by adopting the literature precedentCitation45, and successive O-methylation of the resulting xanthoxyline 2 using dimethyl sulphate and flame-dried potassium carbonate in acetone under reflux condition gave 1-(2,4,6-trimethoxyphenyl)ethanone 3 in excellent yield. 2-Formylation of acetophenone 3 with ethyl formate and sodium hydride gave 3-oxo-3-(2,4,6-trimethoxyphenyl)propanal 4, and without further purification subsequent treatment of 4 with hydrazine hydrate afforded the corresponding 5-(2,4,6-trimethoxyphenyl)-1H-pyrazole 5 in 96% yield. Treatment of compound 5 with methyl iodide and sodium hydride in dry DMF under nitrogen atmosphere afforded a mixture of 1-methyl-5-(2,4,6-trimethoxyphenyl)-1H-pyrazole 6 and 1-methyl-3-(2,4,6-trimethoxyphenyl)-1H-pyrazole 7, which were easily seperated by silica gel column chromatography.
Scheme 2. Reagents and conditions: (a) CH3COCl, AlCl3 (anhydrous), Et2O, N2, 0 °C - rt; (b) Me2SO4, K2CO3, acetone, reflux, 12 h; (c) HCOOEt, NaH, THF, N2, rt; (d) NH2NH2.H2O, rt; (e) MeI, NaH, DMF, N2, 0 °C.
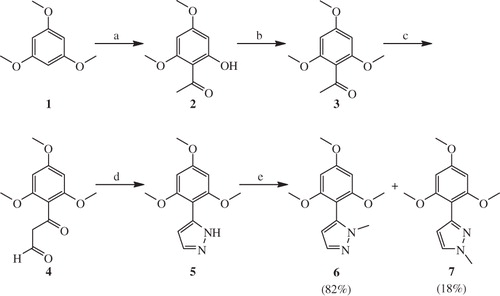
The structures of the regioisomers 6 and 7 were determined using 1D 1H–13C NMR spectra, 135-DEPT and 2D NMR experiments (1H–1H COSY, 1H–13C HSQC and 1H–13C HMBC and NOESY), which were scanned on Bruker AV-400 and 500 MHz instrument (Supporting Data). These two regioisomers differed only in the position of methyl group on pyrazole nucleus, therefore, the 1H and 13C NMR chemical shifts were found to be almost similar. Thus, the structures of these two regioisomers only assigned with the help of HMBC, HSQC and COSY correlations. The most important HMBC and COSY correlations are summarized in and the complete chemical shift assignment is shown in and . According to a Heteronuclear Multiple Bond Correlation (HMBC) experiment performed with regioisomer 6, the HMBC correlation observed between H-13 (δH 3.65) and C-3 (δC 135.5) and with regioisomer 7, the HMBC correlation observed between H-13 (δH 3.97) and C-1 (δC 130.12) allowing to unambiguous identification of the regioisomer 6 and 7 ( and ). Additionally, heteronuclear single quantum coherence (HSQC) experiment was used to assign proton signals to the corresponding carbon signals. There is a strong 1H–1H COSY correlation between H-1 and H-2.
Table 1. 1H, 13C NMR and 135-DEPT spectral data and 1H-1H COSY, HSQC and HMBC correlations in compound 6.
Table 2. 1H, 13C NMR and 135-DEPT spectral data and 1H-1H COSY, HSQC and HMBC correlations in compound 7.
Anti-inflammatory activity
In vitro COX inhibition
All the synthesized compounds (10a–n) were screened for their inhibitory potential against COX-1 and COX-2 enzymes at 100 μM using colorimetric COX (ovine) inhibitor screening assay kit. Indomethacin was used as a reference compound. The results were shown in . The results showed that most of the synthesized compounds had an inhibitory profile against both COX-1 and COX-2 enzymes, some were found to be selective against COX-2 (10i, 10f, 10d, 10h and 10e) by a small percentage of inhibition. Compound 10b, 10l and 10c had an inhibitory profile against both COX-1 and COX-2. However, all the other compounds showed weak inhibitory activity against both the isoforms of COX (). The structure–activity relationship (SAR) study revealed that, compound carrying furyl heterocycle by replacing phenyl ring, 10i, appeared as the most active compound in this series. Moreover, final compounds with –F, –NO2, and –OCH3 substituents (10f, 10h and 10e) at the meta position of phenyl ring showed selective inhibitory activity against COX-2 enzyme.
Table 3. In vitro COX-1 and COX-2 enzyme inhibitory activities of pyrazole containing chalcones (10a–n).
Carrageenan-induced rat paw edema bioassay
Compounds showing significant COX-2 inhibition were selected for further anti-inflammatory evaluation by using carrageenan-induced hind paw edema in rats using indomethacin as a reference standard. The results of all the tested compounds were shown in . The results revealed that among the all tested compounds, compounds 10i, 10e, 10f, and 10h inhibited the edema formation significantly (p < 0.05) at 3 h and 6 h than other compounds when compared to carrageenan control rats. Edema formation due to carrageenan is a biphasic event. The first phase begins immediately after injection and diminishes in 1 h and the second phase begins after 1 h. The initial phase of edema is attributed to the release of histamine and serotonin and second phase is due to the release of prostaglandins. All the tested compounds (10i, 10e, 10f, and 10h) showed greater inhibition of paw edema formation at 6 h due to the inhibition of prostaglandins release, which are known to be released at 4–6 h after the carrageenan injection.
Table 4. Anti-inflammatory activity of the target compounds against the carrageenan-induced paw edema in rats with different time intervals.
Cotton pellet-induced granuloma bioassay
Cotton pellet implantation is the most suitable method for studying the efficacy of drugs against proliferative phase of inflammation. Compounds showing promising anti-inflammatory activity in the carrageenan-induced rat paw edema bioassay were further evaluated for their in vivo cotton pellet-induced granuloma bioassay in rats using indomethacin as a reference standard. In this assay, test compounds 10i, 10e, 10f, and 10h inhibited both the exudatory and granulatory phases of inflammation (). During the repair process of inflammation, there is a proliferation of macrophages, neutrophils, fibroblasts and multiplication of small blood vessels, which are the basic sources of forming a highly vascularized radish mass called as granular tissue (granular tissue formation). Inhibition of this granular phase of inflammation by 10i, 10e, 10f, and 10h suggested significant anti-inflammatory property was observed in the experimental animal models.
Table 5. Effects of test compounds on cotton pellet induced granuloma in rats.
Acute toxicity study
There were no significant behavioral changes observed with all the employed doses of various test compounds. To all the mice, neither toxic reaction nor mortality was observed. Therefore, 2000 mg/kg, body weight (b.w.), dose was considered as maximum tolerated dose. Based on acute toxicity study, we have selected 100 mg/kg for anti-inflammatory activity.
Conclusion
In conclusion, we herein report the synthesis, spectral studies, and biological evaluation of a novel series of pyrazole-based chalcones. The structures of the two regioisomers 6 and 7 were assigned with the help of HMBC, HSQC and COSY correlations. All the newly synthesized compounds (10a–n) were evaluated for in vitro COX-1/COX-2 inhibition and in vivo anti-inflammatory activity. Compounds which showed significant COX-2 inhibition were subjected to anti-inflammatory studies. Some of the synthesized compounds might constitute initial leads for the design of new more potent therapeutics against inflammation. The present study revealed that the compounds 10i, 10e, 10f, and 10h were effective against both carrageenan-induced paw edema and cotton pellet-induced granuloma models. This revealed that these compounds were potent inhibitors of exudative and proliferative phases of inflammation. Thus, these compounds constitute an interesting template for the evaluation of new anti-inflammatory agents and may be helpful for the design of new therapeutic tools against inflammation.
Supplementary material available online
supplementary_material_873037.pdf
Download PDF (19.1 MB)Declaration of interest
The authors report no conflicts of interest. The authors alone are responsible for the content and writing of this article. Author Hemant V. Chavan is thankful to the Council of Scientific and Industrial Research (CSIR), New Delhi, Govt. of India, for financial support in the form of SRF.
References
- Bekhit AA, Fahmy HTY, Rostom SAF, Baraka AM. Design and synthesis of some substituted 1H-pyrazolyl-thiazolo[4,5-d]pyrimidines as anti-inflammatory-antimicrobial agents. Eur J Med Chem 2003;38:27–36
- Khanna IK, Yu Y, Huff RM, et al. Selective Cyclooxygenase-2 inhibitors: heteroaryl modified 1,2-diarylimidazoles are potent, orally active antiinflammatory agents. J Med Chem 2000;43:3168–85
- Frank MM, Fries LF. The role of complement in inflammation and phagocytosis. Immunol Today 1991;12:322–6
- Collier HOJ. Prostaglandins and aspirin. Nature 1971;232:17–19
- Cryer B, Feldman M. Effects of nonsteroidal anti-inflammatory drugs on endogenous gastrointestinal prostaglandins and therapeutic strategies for prevention and treatment of nonsteroidal anti-inflammatory drug-induced damage. Arch Intern Med 1992;152:1145–55
- Clive DM, Stoff JS. Renal syndromes associated with nonsteroidal anti-inflammatory drugs. N Eng J Med 1984;310:563–72
- Allison MC, Howatson AG, Torrance CJ, et al. Gastrointestinal damage associated with the use of nonsteroidal antiinflammatory drugs. N Eng J Med 1992;327:749–54
- Griswold DE, Adams JL. Constitutive cyclooxygenase (COX-1) and inducible cyclooxygenase (COX-2): rationale for selective inhibition and progress to date. Med Res Rev 1996;16:181–206
- Nakamura T, Sato M, Kakinuma H, et al. Pyrazole and isoxazole derivatives as new, potent, and selective 20-hydroxy-5,8,11,14-eicosatetraenoic acid synthase inhibitors. J Med Chem 2003;46:5416–27
- Cheng H, DeMello KML, Li J, et al. Synthesis and SAR of heteroaryl-phenyl-substituted pyrazole derivatives as highly selective and potent canine COX-2 inhibitors. Bioorg Med Chem Lett 2006;16:2076–80
- Bekhit AA, Ashour HMA, Ghany YSA, et al. Synthesis and biological evaluation of some thiazolyl and thiadiazolyl derivatives of 1H-pyrazole as anti-inflammatory antimicrobial agents. Eur J Med Chem 2008;43:456–63
- El-Sayed MA-A, Abdel-Aziz NI, Abdel-Aziz AA-M, et al. Design, synthesis, and biological evaluation of substituted hydrazone and pyrazole derivatives as selective COX-2 inhibitors: molecular docking study. Bioorg Med Chem 2011;19:3416–24
- Nagarapu L, Materi J, Gaikwad HK, et al. Synthesis and anti-inflammatory activity of some novel 3-phenyl-N-[3-(4-phenylpiperazin-1yl)propyl]-1H-pyrazole-5-carboxamide derivatives. Bioorg Med Chem Lett 2011;21:4138–40
- Ahlstrom MM, Ridderstrom M, Zamora I, Luthman K. CYP2C9 Structure-metabolism relationships: optimizing the metabolic stability of COX-2 inhibitors. J Med Chem 2007;50:4444–52
- Prakash O, Kumar R, Parkash V. Synthesis and antifungal activity of some new 3-hydroxy-2-(1-phenyl-3-aryl-4-pyrazolyl) chromones. Eur J Med Chem 2008;43:435–40
- Brough PA, Aherne W, Barril X, et al. 4,5-Diarylisoxazole Hsp90 chaperone inhibitors: potential therapeutic agents for the treatment of cancer. J Med Chem 2008;51:196–218
- Vera-DiVaio MAF, Freitas ACC, Castro HCA, et al. Synthesis, antichagasic in vitro evaluation, cytotoxicity assays, molecular modeling and SAR/QSAR studies of a 2-phenyl-3-(1-phenyl-1H-pyrazol-4-yl)-acrylic acid benzylidene-carbohydrazide series. Bioorg Med Chem 2009;17:295–302
- Singh P, Paul K, Holzer W. Synthesis of pyrazole-based hybrid molecules: search for potent multidrug resistance modulators. Bioorg Med Chem 2006;14:5061–71
- Hsu TC, Robins RK, Cheng CC. Studies on 4APP: antineoplastic action in vitro. Science 1956;13:848–68
- Storer R, Ashton CJ, Baxter AD, et al. The synthesis and antiviral activity of 4-fluoro-1-β-d-ribofuranosyl-1h-pyrazole-3-carboxamide. Nucleosides Nucleotides Nucleic Acids 1999;18:203–16
- Genin MJ, Biles C, Keiser BJ, et al. Novel 1,5-diphenylpyrazole nonnucleoside hiv-1 reverse transcriptase inhibitors with enhanced activity versus the delavirdine-resistant P236L mutant: lead identification and SAR of 3- and 4-substituted derivatives. J Med Chem 2000;43:1034–40
- Qiao JX, Pinto DJ, Orwat MJ, et al. Preparation of 1,1-disubstituted cycloalkyl derivatives as factor Xa inhibitors for treating a thromboembolic disorder. PCT Int Appl WO 03 99, 276 (Chem Abstr 2004;140:16722g)
- Baraldi PG, Bovero A, Fruttarolo F, et al. New strategies for the synthesis of A3 adenosine receptor antagonists. Bioorg Med Chem 2003;11:4161–9
- Stamford AW, Wu Y. Preparation of N-(phenyl)pyrazolyl-N′-piperidinylureas as neuropeptide Y Y5 receptor antagonists. PCT Int Appl WO 2004, 5262 (Chem. Abstr. 2004;140:11141p)
- Brown ML, Cheung M, Dickerson SH, et al. Preparation of pyrazolopyrimidines as kinase inhibitors for the treatment of type 2 diabetes. PCT Int Appl WO 2004, 9596 (Chem. Abstr. 2004;140:128436y)
- Heerding DA. Preparation of arylazopyrazoles as thrombopoietin mimetics. PCT Int Appl WO 03 103686 (Chem. Abstr. 2004;140: 42170v)
- Duma J, Hatoum-Mokdad H, Sibley R, et al. 1-Phenyl-5-pyrazolyl ureas: potent and selective p38 kinase inhibitors. Bioorg Med Chem Lett 2000;10:2051–4
- Reynold JEF, ed. The extra pharmacopia, 30th ed. Martindale, London: Pharmaceutical Press; 1993. 1 p
- Amir M, Kumar S. Synthesis and anti-inflammatory, analgesic, ulcerogenic and lipid peroxidation activities of 3,5-dimethyl pyrazoles, 3-methyl pyrazol-5-ones and 3,5-disubstituted pyrazolines. Indian J Chem Sec B 2005;44B:2532–7
- Gursoy A, Demirayak S, Capan G, et al. Synthesis and preliminary evaluation of new 5-pyrazolinone derivatives as analgesic agents. Eur J Med Chem 2000;35:359–64
- Kumar A, Sharma S, Bajaj K, et al. Synthesis and anti-inflammatory, analgesic, ulcerogenic and cyclooxegenase activity of novel quinazolinyl-Δ2-pyrazolines. Indian J Chem Sec B 2003;42B:1979–84
- Dimmock JR, Elias DW, Beazely MA, Kandepu NM. Bioactivities of chalcones. Curr Med Chem 1999;6:1125–49
- Go ML, Wu X, Liu XL. Chalcones: an update on cytotoxic and chemoprotective properties. Curr Med Chem 2005;12:483–99
- Kontogiorgis C, Mantzanidou M, Hadjipavlou-Litina D. Chalcones and their potential role in inflammation. Mini Rev Med Chem 2008;8:1224–42
- Nowakowska Z. A review of anti-infective and anti-inflammatory chalcones. Eur J Med Chem 2007;42:125–37
- Bandgar BP, Gawande SS, Bodade RG, et al. Synthesis and biological evaluation of a novel series of pyrazole chalcones as anti-inflammatory, antioxidant and antimicrobial agents. Bioorg Med Chem 2009;17:8168–73
- Bandgar BP, Gawande SS, Bodade RG, et al. Synthesis and biological evaluation of simple methoxylated chalcones as anticancer, anti-inflammatory and antioxidant agents. Bioorg Med Chem 2010;18:1364–70
- Chavan HV, Bandgar BP, Adsul LK, et al. Design, synthesis, characterization and anti-inflammatory evaluation of novel pyrazole amalgamated flavones. Bioorg Med Chem Lett 2013;23:1315–21
- Bandgar BP, Chavan HV, Adsul LK, et al. Design, synthesis, characterization and biological evaluation of novel pyrazole integrated benzophenones. Bioorg Med Chem Lett 2013;23:912–16
- Bandgar BP, Adsul LK, Chavan HV, et al. Synthesis, biological evaluation, and molecular docking of N-{3-[3-(9-methyl-9H-carbazol-3-yl)-acryloyl]-phenyl}-benzamide/amide derivatives as xanthine oxidase and tyrosinase inhibitors. Bioorg Med Chem 2012;20:5649–57
- Bandgar BP, Patil SA, Totre JV, et al. Synthesis and biological evaluation of nitrogen-containing benzophenone analogues as TNF-α and IL-6 inhibitors with antioxidant activity. Bioorg Med Chem Lett 2010;20:2292–6
- Maurias M, Handler N, Erker T, et al. Resveratrol analogues as selective cyclooxygenase-2 inhibitors: synthesis and structure–activity relationship. Bioorg Med Chem 2004;12:5571–8
- Winter CA, Risley EA, Nuss GW. Carrageenin-induced edema in hind paw of the rat as an assay for antiinflammatory drugs. Proc Soc Exp Biol Med 1962;111:544--74
- Winter CA, Portar CC. Effect of alterations in the side chain upon anti-inflammatory and liver glycogen activities of hydrocortisone esters. J Am Pharm Assoc Sci Educ 1957;46:515–19
- Ahluwalia VK. Intermediates for organic synthesis. New Delhi, India: I. K. International Publisher; 2005. 140 p