Abstract
The ability of flavonoids to affect multiple key pathways of glucose toxicity, as well as to attenuate inflammation has been well documented. In this study, the inhibition of rat lens aldose reductase by 3,7-di-hydroxy-2-[4-(2-chloro-1,4-naphthoquinone-3-yloxy)-3-hydroxy-phenyl]-5-hydroxy-chromen-4-one (compound 1), was studied in greater detail in comparison with the parent quercetin (compound 2). The inhibition activity of 1, characterized by IC50 in low micromolar range, surpassed that of 2. Selectivity in relation to the closely related rat kidney aldehyde reductase was evaluated. At organ level in isolated rat lenses incubated in the presence of high glucose, compound 1 significantly inhibited accumulation of sorbitol in a concentration-dependent manner, which indicated that 1 was readily taken up by the eye lens cells and interfered with cytosolic aldose reductase. In addition, compound 1 provided macroscopic protection of colonic mucosa in experimental colitis in rats. At pharmacologically active concentrations, compound 1 and one of its potential metabolite 2-chloro-3-hydroxy-[1,4]-naphthoquinone (compound 3) did not affect osmotic fragility of red blood cells.
Introduction
Aldo-keto reductases are NAD(P)H-dependent oxidoreductases, best characterized as glucose reducing agents. They have been implicated in the pathophysiology of diabetic complications. These enzymes have also been reported to metabolize lipid peroxidation products, contributing in some settings to the inflammatory response.
Aldose reductase (ALR2), apart from its involvement in diabetic complications via reducing glucose, was found to efficiently reduce lipid peroxidation-derived aldehydes and their glutathione conjugatesCitation1. Lipid peroxidation-derived lipid aldehydes, such as 4-hydroxy-trans-2-nonenal (HNE), and their glutathione conjugates (e.g. GS-HNE), were found to be efficiently reduced by ALR2 to the corresponding alcohols DHN (1,4-dihydroxy-nonene) and GS-DHN (glutathionyl-1,4-dihydroxynonene), which mediate inflammatory signalsCitation2.
ALR2 inhibitors, such as acetic acid derivatives (tolrestat, zenarestat), spiro hydantoins (sorbinil) or the succinimide class of compounds (ranirestat), have been primarily investigated for their role against diabetic complicationsCitation3–8.
The clinical data assembled on ARIs in diabetic clinics may assist in the transition of these inhibitors to treatment of inflammatory disorders. In the search for better aldose reductase inhibitors, the focus has been shifted in recent years towards novel chemotypesCitation7,Citation8.
Recently, considerable attention has been devoted to the search for phytochemical therapeutics. A variety of constituents, like vitamins, minerals, fiber and numerous phytochemicals, including flavonoids, may contribute to the health effects of fruits and vegetables. The flavonol quercetin is the most widely consumed flavonoid in the human diet and its multiple health effects have been extensively reviewedCitation9,Citation10. In plants, quercetin is present mainly in the form of O-glycosides with a sugar group, such as glucose, galactose, rhamnose, rutinose or xylose. The glycosidic structure has a large impact on the biological activity and bioavailability of quercetinCitation11,Citation12.
Patent databases present numerous semi-synthetic derivatives of quercetin with multiple biological effects such as vasodilator, antidiabetic, antihistaminic, anti-inflammatory effects, immune supporting effect and others, including their anti-cancer effect. Considering the pharmacological value of derivatives of quercetin, it is apparent that possibilities for optimal modification of quercetin have not been exhausted yet. It is important to selectively modify various hydroxyl groups of quercetin since they are not equivalent, either in chemical or bio-functional terms. Further modifications with appropriate substituents, balancing hydrophilic-lipophilic behavior and biological effect, thus provide an alternative to the previously described quercetin derivatives.
Recently 21 novel semi-synthetic derivatives of quercetin have been synthesized and screened for inhibition of ALR2 and for antioxidant actionCitation13,Citation14. Among the compounds studied, 2-chloro-1,4-naphthoquinone derivative (compound 1, ) exhibited the highest biological activities. In the present article, inhibition of aldose reductase by 1 is reported in greater detail in comparison with quercetin (compound 2), both at the level of isolated enzyme and at organ level, in the model of isolated rat eye lenses. To identify crucial interactions within the ALR2 binding site, molecular modeling studies were performed. Selectivity in relation to the closely related rat kidney aldehyde reductase (ALR1) was evaluated. Anti-inflammatory action of 1 is presented in the rat model of experimental colitis. In addition, the effect of 1 and of its potential metabolite 2-chloro-3-hydroxy-[1,4]-naphthoquinone (compound 3) on osmotic fragility of isolated red blood cells was investigated, since 1,4-naphthoquinones were reported toxic to erythrocytesCitation15,Citation16.
Methods
Chemicals
Compound 1, 3,7-dihydroxy-2-[4-(2-chloro-1,4-naphthoquinone-3-yloxy)-3-hydroxyphenyl]-5-hydroxychromen-4-one, was synthesized by the alkylation of quercetin with 2,3-dichloro-1,4-naphthoquinone in the presence of N,N-diisopropylethylamine at 100 °C as described beforeCitation13 and provided by BEL/NOVAMANN (Bratislava, Slovakia). The compound was purified by column chromatography to purity >95%, as verified by elemental analysis. Compound 3, 2-chloro-3-hydroxy-[1,4]-naphthoquinone, was obtained from Rare Chemicals GmbH (Kiel, Germany). Quercetin (≥95%), sorbitol dehydrogenase, diaphorase, β-NAD, resazurin, M-199 medium (M 3769), d,l-glyceraldehyde, sodium glucuronate, NADPH, d-glucose, β-mercaptoethanol and HClO4 were obtained from Sigma–Aldrich (St. Louis, MO). Diethylaminoethyl cellulose DEAE DE 52 was from Whatman International Ltd. (Maidstone, England). Other chemicals were purchased from local commercial sources and were of analytical grade quality.
Animals and preparation of packed erythrocytes
Male Wistar rats, 8–9 weeks old, weighing 200–250 g, were used. The animals came from the Breeding Facility of the Institute of Experimental Pharmacology, Dobra Voda (Slovak Republic). The study was approved by the Ethics Committee of the Institute and performed in accordance with the Principles of Laboratory Animal Care (NIH publication 83-25, revised 1985) and the Slovak law regulating animal experiments (Decree 289, Part 139, 9 July 2003).
The animals in light ether anesthesia were killed by exsanguinations of the carotid artery. The blood was collected in 3.8% sodium citrate (1 vol. of sodium citrate : 9 vol. of blood) and centrifuged at 500 × g for 15 min at 4 °C. Plasma and white blood cells were removed by aspiration. The retrieved erythrocytes were washed 3 times with 6 vol. of 10 mmol/l ice-cold isotonic phosphate buffer saline (PBS; pH 7.4) containing 1.9 mmol/l NaH2PO4, 8.1 mmol/l Na2HPO4 and 150 mmol/l NaCl. The entire procedure was conducted at 0–4 °C. After the last washing, the red blood cells were used for further studies. The erythrocyte suspensions used in experiments were prepared daily.
Preparation of ALR2
ALR2 from rat lens was partially purified using a procedure adapted from the work by Hayman and KinoshitaCitation17 as follows: lenses were quickly removed from rats following euthanasia and homogenized in a glass homogenizer with a teflon pestle in 5 vol. of cold distilled water. The homogenate was centrifuged at 10 000 × g at 0–4 °C for 20 min. The supernatant was precipitated with saturated ammonium sulfate at 40%, 50% and then at 75% salt saturation. The supernatant was retained after the first two precipitations. The pellet from the last step, possessing ALR2 activity, was dispersed in 75% ammonium sulfate and stored in smaller aliquots in liquid nitrogen container.
Preparation of ALR1
ALR1 from rat kidney was partially purified according to the reported procedure of Costantino et al.Citation18 as follows: kidneys were quickly removed from rats following euthanasia and homogenized in a knife homogenizer followed by processing in a glass homogenizer with a teflon pestle in 3 vol. of 10 mM sodium phosphate buffer, pH 7.2, containing 0.25 M sucrose, 2.0 mM EDTA dipotassium salt and 2.5 mmol/l β-mercaptoethanol. The homogenate was centrifuged at 16 000 × g at 0–4 °C for 30 min and the supernatant was subjected to ammonium sulfate fractional precipitation at 40%, 50% and 75% salt saturation. The pellet obtained from the last step, possessing ALR1 activity, was redissolved in 10 mM sodium phosphate buffer, pH 7.2, containing 2.0 mmol/l EDTA dipotassium salt and 2.0 mmol/l β-mercaptoethanol to achieve total protein concentration of ∼20 mg/ml. DEAE DE 52 resin was added to the solution (33 mg/ml) and after gentle mixing for 15 min removed by centrifugation. The supernatant containing ALR1 was then stored in smaller aliquots in liquid nitrogen. No appreciable contamination by ALR2 in ALR1 preparations was detected since no activity in terms of NADPH consumption was observed in the presence of glucose substrate up to 150 mmol/l.
ALR1 and ALR2 enzyme assays
ALR1 and ALR2 activities were assayed spectrophotometrically as described beforeCitation19 by determining NADPH consumption at 340 nm and were expressed as decrease of the optical density (O.D.)/s/mg protein. To determine ALR2 activity, the reaction mixture contained 4.67 mmol/l d,l-glyceraldehyde as a substrate, 0.11 mmol/l NADPH, 0.067 M phosphate buffer, pH 6.2 and 0.05 ml of the enzyme preparation in a total volume of 1.5 ml. The reference blank contained all the above reagents except the substrate d,l-glyceraldehyde to correct for oxidation of NADPH not associated with reduction of the substrate. The enzyme reaction was initiated by addition of d,l-glyceraldehyde and was monitored for 4 min after an initial period of 1 min at 30 °C. ALR1 activity was assayed analogically using 20 mmol/l d-glucuronate as a substrate in the presence of 0.12 mmol/l NADPH in 0.1 M phosphate buffer pH 7.2 at 37 °C. Enzyme activities were adjusted by diluting the enzyme preparations with distilled water so that 0.05 ml of the preparation gave an average reaction rate for the control sample of 0.020 ± 0.005 absorbance units/min. The effect of compounds 1 and 2 on the enzyme activity was determined by including in the reaction mixture each inhibitor at required concentrations dissolved in DMSO at 1% final mixture concentration. The inhibitor at the same concentration was included in the reference blank. IC50 values (the concentration of the inhibitor required to produce 50% inhibition of the enzyme reaction) were determined from the least-square analysis of the linear portion of the semi-logarithmic inhibition curves. Each curve was generated using at least four concentrations of inhibitor causing an inhibition in the range from at least 25–75%.
Eye lenses sorbitol assay
The animals in light ether anesthesia were killed by exsanguinations of the carotid artery and the eye globes were excised. The lenses were quickly dissected and rinsed with saline. The compounds 1 or 2 dissolved in DMSO were added into the tubes containing freshly dissected eye lenses (1 lens per tube) in M-199 medium at pH 7.4, bubbled at 37 °C with pneumoxid (5% CO2, 95% O2), to the final concentrations as reported, 30 min before adding glucose. The final concentration of DMSO in all incubations was 1%. The incubation was initiated by adding glucose to the final concentration of 50 mmol/l and then continued at 37 °C with occasional (in ∼30-min intervals) bubbling of the mixture for ∼30-s periods with pneumoxid. The incubations were terminated after a 3-h period by cooling the mixtures in an ice bath, followed by washing the lenses three times with ice-cold phosphate buffered saline (1 ml). The short-term cultivations were preferred to avoid substantial permeability changes of the eye lenses. The washed lenses were kept deep-frozen for sorbitol determination which was performed as described beforeCitation20. In brief, the frozen lenses were let to melt at the ambient temperature. Then distilled water (0.2 ml/1 lens) was added. The lenses were disrupted by a glass rod. The rod was washed twice with distilled water (0.1 ml) and the suspension was ultra-sounded for 5 min. Thereafter, ice cold HClO4 (9%, 0.4 ml) was added and mixed thoroughly. The mixture was ultra-sounded for another 5 min and then kept on ice for 30 min to let proteins precipitate. The precipitated protein was spun off (15 min at 3000 rpm) at 4 °C. The supernatant was neutralized with concentrated K2CO3 (4 M). The neutralized supernatant was used for the determination of concentration of sorbitol by modified enzymatic analysisCitation21. In brief, sorbitol was oxidized to fructose by sorbitol dehydrogenase (SDH) with concomitant reduction of resazurin by diaphorase to the highly fluorescent resorufin. The final concentrations of the assay solutions were: diaphorase (11.5 U/25 ml triethanolamine buffer), NAD+ (25 mg/25 ml triethanolamine buffer), resazurin (0.025 ml 2 mmol/l resazurin solution in 25 ml of triethanolamine buffer), SDH (15.025 U/1 ml triethanolamine buffer). Reaction mixtures were incubated for 60 min at room temperature with an opaque cover. The sample fluorescence was determined at excitation 544 nm, emission 590 nm. After the appropriate blanks were subtracted from each sample, the amount of sorbitol in nmol per gram of lens wet weight in each sample was determined by comparison with a linear regression of sorbitol standards.
Experimental ulcerative colitis in rats
The 24-h fasted rats were anesthetized with thiopental (65 mg/kg i.p.). A rubber cannula (8 cm long) was inserted into the colon through anus. Acetic acid (4% solution in water, 1.5 ml) was instilled and the cannula was left in the colon for 30 s and then the fluid was withdrawnCitation22. Two hours and then 1 h before the acetic acid rectal administration, the rats were administered orally saline (untreated colitis group), pure DMSO (DMSO-treated group), or DMSO solution of compound 1 (2 × 50 mg/kg; DMSO + 1 group), or DMSO solution of quercetin (compound 2, 2 × 50 mg/kg; DMSO + 2 group). The drugs were dissolved in concentrated DMSO and the volume administered did not exceed 1 ml. After 48 h, the animals were sacrificed by exsanguination in thiopental (65 mg/kg i.p.) anesthesia. The colonic segments, rinsed with cold saline, were placed on an ice-cold plate, cleaned of fat and mesentery, and blotted on filter paper. The colon was cut longitudinally and scored for visible damage according to Nosaľova et al.Citation23 by an observer unaware of the treatment. The following criteria were used for scoring colonic damage: 0 – normal appearance; 1 – hyperemia; 2 – hemorrhage; 3 – one ulcer; 4 – two or more sites of ulceration; 5 – ulceration exceeding >1 cm along the length of the colon; 6 and further – damage exceeding >2 cm along the colon length, and with each additional centimeter of involvement the score was increased by one point. All results are expressed as mean ± SD. Differences among groups were tested for statistical significance the Mann–Whitney U-test. Statistical significance was set at p < 0.05.
Osmotic fragility of red blood cells
The osmotic fragility was determined by the degree of hemolysis induced by the changes of osmotic pressure using a step-down protocol with decreasing concentrations of NaCl. The erythrocytes were suspended (hematocrit 0.4%) in isotonic PBS containing increasing concentrations of NaCl from 0 to 150 mmol/l in the absence/presence of 1 or 3 in the final concentration of 25 µmol/l. The total hemolysis (100%) was obtained by incubation of control erythrocytes in 10 mmol/l hypotonic PBS, pH 7.4 (1.9 mmol/l NaH2PO4, 8.1 mmol/l Na2HPO4). The samples were incubated for 1 h at 37 °C in a shaking water bath. After incubation, the erythrocyte suspensions were centrifuged at 700 × g for 15 min at 4 °C. The hemolysis degree was determined by spectrophotometry of the hemoglobin released into the supernatant, as described by WinterbournCitation24.
Computational methods
Low energy conformations (starting geometries) of the compounds studied were obtained by Monte Carlo equilibrium conformer search (MMFF94) and subsequent optimization in DFT B3LYP 6-31G* method, all performed in program SPARTAN’08 (Wavefunction, Inc., Irvine, CA, 2009)Citation25. To search for the most probable ionic form of quercetin and compound 1, we performed the calculations for all possible dissociated forms of both molecules. Anions with minimum energy (7-O− for both quercetin and 1, in vacuum and water) were chosen for further studies.
For modeling the enzyme-ligand interaction, the PDB structure of aldose reductase complexed with NADP+ and lidorestat was taken from Protein Data Bank (http://www.rcsb.org, structure 1z3n, representing the aldo-keto reductase class AKR1B1). The structure of the enzyme was treated to correct the bonds and hydrogens by means of the software YASARACitation26. First, the individual ligands (1, 2 and zopolrestat) were immersed in original (unoptimized) complex instead of lidorestat and docking procedure according to the local docking protocol of YASARA (with 250 runs and RMSDmin = 5.0 Å) was performed. The first 10 clusters were then searched for the minimum value of Ebin within the optimization protocol em_run.mcr, which enables to take into account the surrounding water molecules. After optimization, the water molecules were deleted and the value of Ebin coming from the pure interaction between the ligand and enzyme–coenzyme complex was calculated.
Results
As shown in , compound 1 was found to be a more efficient inhibitor of ALR2 in comparison with the parent quercetin. No inhibition of the aldose reductase was recorded for compound 3. Non-competitive type of inhibition of ALR2 by 1 and for d,l-glyceraldehyde as a substrate was observed (), with the corresponding inhibition constant Ki(1) = 1.15 ± 0.37 μmol/l.
Figure 2. Inhibitory effect of compound 1 (2-chloro-1,4-naphthoquinone derivative of quercetin) on rat lens aldose reductase. Typical double reciprocal plot of the initial enzyme velocity versus the concentration of substrate (d,l-glyceraldehyde) in the presence or absence of inhibitor: (•) no inhibitor; (○) 2 μmol/l compound 1 (non-competitive type of inhibition).
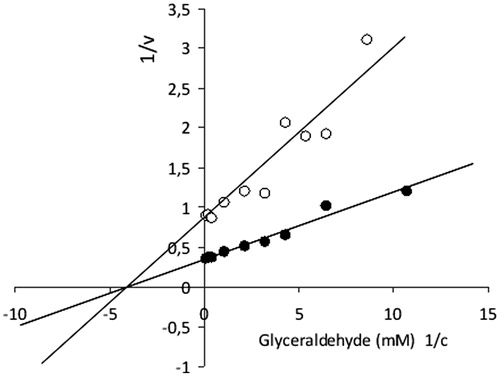
Table 1. Inhibitory effect of compound 1 on rat ALR2 and ALR1 in comparison with quercetin (2).
The IC50 value of compound 1 for its inhibition of glucuronide substrate reduction by partially purified aldehyde reductase (ALR1) from rat kidney was found to be ∼5 times higher in comparison with the IC50 value for ALR2 ().
The interaction energies Ebin of 1 and 2 versus enzyme–NADP+ complexes, in comparison with reference zopolrestat, calculated by computer aided molecular modeling for the best scored docked geometries by total optimization of the inhibitor–ALR2–NADP+ ternary complex (), were 351.46 kJ/mol for 1, 348.12 kJ/mol for 2 and 537.96 kJ/mol for standard zopolrestat.
As shown in , significantly increased sorbitol levels were recorded in the lenses incubated with glucose in comparison with control incubations without glucose. Sorbitol production was inhibited both by compounds 1 and 2 in a concentration-dependent manner. At 20 μmol/l concentration, slightly higher inhibition was recorded for 1 in comparison with 2.
Table 2. Effect of compound 1 on sorbitol accumulation in isolated rat lenses cultivated with high glucose* in comparison with quercetin (2).
In rats, colonic instillation of acetic acid induced a pronounced intestinal injury of various intensities, ranging from hyperemia to severe hemorrhagic necrosis. As summarized in , the damage score was 7.4 ± 1.2. Administration of DMSO vehicle worsened the acetic-acid-induced colonic injury (score 9.4 ± 1.0), yet non-significantly. Equally, quercetin (2 × 50 mg/kg) had no effect on macroscopic injury (score 9.50 ± 0.50). Compound 1, at the same dose as quercetin, significantly protected the rat colon against acetic-acid-induced injury with the score of 3.4 ± 1.0.
Table 3. Effect of compound 1 and quercetin (2) on acute experimental colitis induced in rats by acetic acid*.
For compounds 1 and 3, their effect on osmotic fragility of the erythrocyte membrane was assessed. As shown in , at concentration 25 μmol/l, neither of the compounds did affect significantly the course of osmotic hemolysis of erythrocytes.
Figure 4. Effect of compounds 1 and 3 on osmotic fragility of rat erythrocytes. Erythrocyte suspensions (0.4% haematocrit) in 10 mmol/l phosphate buffer containing increasing concentrations of NaCl were incubated at 37 °C for 1 h in the absence (•) or presence of compound 1 (○, 25 μmol/l) or compound 3 (▴, 25 μmol/l). Experimental points represent mean values ± SD from at least three independent experiments.
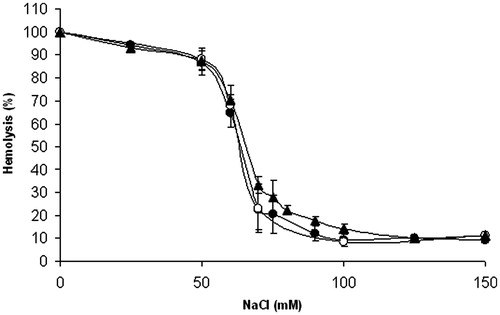
Discussion
Chemistry
As part of our ongoing interest in developing new aldose reductase inhibitors, we recently reported the synthesis of 21 novel derivatives of quercetin and the results of their screening for inhibition of aldose reductase and antioxidant activity actionCitation13,Citation14. Among the novel compounds studied, the most promising results were obtained for the 2-chloro-1,4-naphthoquinone derivative of quercetin (compound 1, ).
Enzyme inhibition
In the present study, compound 1 was evaluated for its ability to inhibit the in vitro reduction of d,l-glyceraldehyde by partially purified ALR2 from rat lenses, in relation to the parent quercetin (2) as reference. For 1, the inhibitory activity in low micromolar range of IC50 values was recorded to surpass that of 2 (). The reported IC50 values for quercetin inhibition of aldose reductase vary from 2.2 to 12.7 μmol/l both for rat lens ALR2 and human recombinant AKR1B1Citation27–31.
The non-competitive type of ALR2 inhibition () indicates that the glucose substrate would not compete with the inhibitor for the enzyme. Thus under hyperglycemia conditions in diabetics, excessive glucose would presumably not decrease the inhibitory efficacy of the drug. The experimentally obtained Km value for the aldose reductase and glyceraldehyde substrate, (Km)Glyceraldehyde = 0.21 ± 0.04 mmol/l, was in the range of those determined by other authors for rat lens ALR2Citation32–34.
The value of IC50 = 13.6 ± 3.6 μmol/l for inhibition of ALR1 by 1 points to a medium selectivity of 1 in inhibition of ALR2 relative to ALR1, which is involved in detoxification of diverse aldehydes and dicarbonyl compounds.
Molecular modeling
To relate the inhibitory efficiency of compounds 1 and 2 to their structural features and to identify crucial interactions within the enzyme binding site, we performed several computational studies. The calculated results of Ebin obtained for the anionic species, 351.46 kJ/mol for 1, 348.12 kJ/mol for 2 and 537.96 kJ/mol for standard zopolrestat, agreed with the order of the inhibitory activities, considering the previously reportedCitation19 IC50 value for zopolrestat to be 0.005 ± 0.001 μmol/l.
Based on the geometric parameters of the minimized complex, interaction with Thr113 seems to be crucial for the inhibitory activity of compound 1, along with binding to Trp20, Tyr48, Leu300 (H-bond) and Trp111 (hydrophobic and pi–pi interactions). In addition, hydrophobic interaction of the chloronaphthoquinone moiety with Phe122 was identified. The mutual distance between the phenolic oxygen (7-O−) of the inhibitor and the hydrogen of Thr113 was found to be 1.7 Å ().
Inhibitory activity on rat lens sorbitol accumulation
The eye lens represents a body tissue with the highest content of aldose reductase, the first enzyme of the polyol pathway, which is believed to have a key role in the development of diabetic cataract. On this basis, we studied the effect of 1, in comparison with 2, on glucose-induced sorbitol accumulation in isolated rat lenses incubated in the presence of high glucose in vitro. Significantly increased sorbitol levels recorded in the lenses incubated with glucose, in comparison with control incubations without glucose, reflect increased flux of glucose through the polyol pathway. Similarly, other authorsCitation35 observed a more than 10-fold increase of sorbitol level in the eye lenses incubated with glucose under comparable conditions (50 mmol/l glucose, 4-h incubation). Inhibition of sorbitol production by both compounds 1 and 2 indicates that the compounds are readily taken up by eye lens cells and within the lens they interfere with cytosolic ALR2.
Effect on acute experimental colitis in rats
The highly significant inhibition of acetic-acid-induced colonic injury indicates that 1 or its metabolic product(s) reaches the colon after oral administration in pharmacologically effective concentrations. On the other hand, the inability of quercetin to attenuate colon injury in animal colitis models can be explained by its absorption profileCitation36--39. Quercetin is rapidly absorbed in the upper intestinal portion which may prevent its ability to reach pharmacologically effective concentrations at the site of required action, i.e. the inflamed colonCitation40,Citation41. Our results, pointing to the difference in bioavailability of quercetin and its synthetic derivative 1, are in agreement with the well-documented variance in bioavailabilities of natural derivatives of quercetinCitation10–12.
Effect on osmotic hemolysis
Since 1,4-naphthoquinones were reported toxic to erythrocytesCitation15,Citation16, we investigated the effect of 1 and its potential metabolite 2-chloro-3-hydroxy-[1,4]-naphthoquinone (compound 3) on osmotic fragility of isolated red blood cells. At the concentrations studied, no significant hemolytic effect of the compounds was observed ().
Conclusions
Compound 1 (i) inhibited ALR2 isolated from rat lenses with an activity in the low micromolar range of IC50 values; structurally related ALR1 was inhibited less efficiently, with an ∼5 times higher IC50; key interactions of 1 with amino acid residues in the inhibitor binding site of ALR2 were identified by molecular modeling; (ii) attenuated sorbitol accumulation in isolated rat eye lenses as a result of its inhibition of glucose flux through the polyol pathway, which indicates that 1 is readily taken up by eye lens cells and interferes with cytosolic ALR2; (iii) provided macroscopic protection of colonic mucosa in acetic-acid-induced experimental colitis in rats when administered orally before induction of colitis, which indicates availability of 1 and/or its metabolite(s) at the site of the colon and (iv) compound 1 and one of its potential metabolites, 2-chloro-3-hydroxy-[1,4]-naphthoquinone (compound 3), at pharmacologically active concentrations, did not affect osmotic fragility of red blood cells.
Recently, antioxidant activity of 1 was proved in a DPPH test in vitroCitation14. Based on the initial velocity of DPPH decolorization, the antiradical activity of 1 was found higher than that of quercetin and comparable with that of trolox. Under the experimental conditions used, one molecule of l was found to quench 2.6 ± 0.1 DPPH radicals. Antioxidant action, in addition to the efficient inhibition of aldose reductase, makes compound 1 interesting from the point of its potential pharmacological use for indications like diabetic complications and inflammatory disorders. It is a question of further studies to reveal exact molecular mechanisms involved in the observed protective effects of 1.
Declaration of interest
The authors have declared no conflicts of interest. This work was supported by VEGA 2/0067/11, VEGA 2/0030/11, EU Structural Funds (ITMS 26240220040) and the joint research project between the Scientific and Technical Research Council of Turkey (TÜBİTAK) and the Slovak Academy of Sciences 2013–2015 (project number 113S006).
References
- Ramana K-V. Aldose reductase: new insights for an old enzyme. Biomol Concepts 2011;2:103–14
- Chatzopoulou M, Pegklidou K, Papastavrou N, Demopoulos V-J. Development of aldose reductase inhibitors for the treatment of inflammatory disorders. Expert Opin Drug Discov 2013;8:1365–80
- Hotta N. New approaches for treatment in diabetes: aldose reductase inhibitors. Biomed Pharmacother 1995;5:244–50
- Costantino L, Rastelli G, Gamberini M-C, Barlocco D. Pharmacological approaches to the treatment of diabetic complications. Expert Opin Ther Patents 2000;10:1245–62
- Miyamoto S. Recent advances in aldose reductase inhibitors: potential agents for the treatment of diabetic complications. Expert Opin Ther Patents 2002;12:621–31
- Srivastava S-K, Ramana K-V, Bhatnagar A. Role of aldose reductase and oxidative damage in diabetes and the consequent potential for therapeutic options. Endocr Rev 2005;26:380–92
- Alexiou P, Pegklidou K, Chatzopoulou M, et al. Aldose reductase enzyme and its implication to major health problems of the 21(st) century. Curr Med Chem 2009;16:734–52
- Chatzopoulou M, Alexiou P, Kotsampasakou E, Demopoulos V-J. Novel aldose reductase inhibitors: a patent survey (2006-present). Expert Opin Ther Pat 2012;11:1303–23
- Boots A-W, Haenen GRMM, Bast A. Health effects of quercetin: from antioxidant to nutraceutical. Eur J Pharm 2008;585:325–37
- Stefek M, Karasu C. Eye lens in aging and diabetes: effect of quercetin. Rejuvenation Res 2011;14:525–34
- Arts IC, Sesink A-L, Faassen-Peters M, Hollman P-C. The type of sugar moiety is a major determinant of the small intestinal uptake and subsequent biliary excretion of dietary quercetin glycosides. Br J Nutr 2004;91:841–7
- Crozier A, Del Rio D, Clifford M-N. Bioavailability of dietary flavonoids and phenolic compounds. Mol Aspects Med 2010;31:446–67
- Veverka M, Gallovic J, Svajdlenka E, et al. Novel quercetin derivatives: synthesis and screening for antioxidant activity and aldose reductase inhibition. Chem Papers 2013;67:76–83
- Milackova I, Kovacikova L, Veverka M, et al. Screening for antiradical efficiency of 21 semi-synthetic derivatives of quercetin in a DPPH assay. Interdiscip Toxicol 2013;6:13--17
- Munday R, Fowke E-A, Smith B-L, Munday C-M. Comparative toxicity of alkyl-1,4-naphthoquinones in rats: relationship to free radical production in vitro. Free Radic Biol Med 1994;16:725–31
- Munday R, Smith B-L, Munday C-M. Structure-activity relationships in the haemolytic activity and nephrotoxicity of derivatives of 1,2- and 1,4-naphthoquinone. J Appl Toxicol 2007;27:262–9
- Hayman S, Kinoshita J. Isolation and properties of lens aldose reductase. J Biol Chem 1965;240:877–82
- Stefek M, Snirc V, Djoubissie P-O, et al. Carboxymethylated pyridoindole antioxidants as aldose reductase inhibitors: synthesis, activity, partitioning, and molecular modelling. Bioorg Med Chem 2008;16:4908–20
- Costantino L, Rastelli G, Gamberini M-C, et al. 1-Benzopyran-4-one antioxidants as aldose reductase inhibitors. J Med Chem 1999;42:1881–93
- Juskova M, Snirc V, Gajdosikova A, et al. Carboxymethylated tetrahydropyridoindoles as aldose reductase inhibitors: in vitro selectivity study in intact rat erythrocytes in relation to glycolytic pathway. Gen Physiol Biophys 2009;28:325–30
- Mylari B-L, Armento S-J, Beebe D-A, et al. A highly selective, non-hydantoin, non-carboxylic acid inhibitor of aldose reductase with potent oral activity in diabetic rat models: 6-(5-chloro-3-methylbenzofuran-2-sulfonyl)-2-H-pyridazin-3-one. J Med Chem 2003;46:2283–6
- Fabia R, Willen R, Ar’Rajab A, et al. Acetic acid-induced colitis in the rat: a reproducible experimental model for acute ulcerative colitis. Eur Surg Res 1992;24:211–25
- Nosalova V, Zeman M, Cerna S, et al. Protective effect of melatonin in acetic acid induced colitis in rats. J Pineal Res 2007;42:364–70
- Winterbourn C-C. Oxidative reactions of hemoglobin. Meth Enzymol 1990;186:265–72
- Shao Y, Molnar L-F, Jung Y, et al. Advances in methods and algorithms in a modern quantum chemistry program package. Phys Chem Chem Phys 2006;8:3172–91
- Krieger E, Koraimann G, Vriend G. Increasing the precision of comparative models with YASARA NOVA – a self-parameterizing force field. Proteins [Online] 2002;47:393–402
- Lee Y-S, Kim S-H, Jung S-H, et al. Aldose reductase inhibitory compounds from Glycyrrhiza uralensis. Biol Pharm Bull 2010;33:917–21
- Jung H-A, Jung Y-J, Yoon N-Y, et al. Inhibitory effects of Nelumbo nucifera leaves on rat lens aldose reductase, advanced glycation endproducts formation, and oxidative stress. Food Chem Toxicol 2008;46:3818–26
- Jung H-A, Yoon N-Y, Kang S-S, et al. Inhibitory activities of prenylated flavonoids from Sophora flavescens against aldose reductase and generation of advanced glycation endproducts. J Pharm Pharmacol 2008;60:1227–36
- Wirasathien L, Pengsuparp T, Suttisri R, et al. Inhibitors of aldose reductase and advanced glycation end-products formation from the leaves of Stelechocarpus cauliflorus R.E. Fr. Phytomedicine 2007;14:546–50
- Matsuda H, Morikawa T, Toguchida I, Yoshikawa M. Structural requirements of flavonoids and related compounds for aldose reductase inhibitory activity. Chem Pharm Bull (Tokyo) 2002;50:788–95
- DeRuiter J, Borne R-F, Mayfield C-A. N- and 2-substituted N-(phenylsulfonyl)glycines as inhibitors of rat lens aldose reductase. J Med Chem 1989;32:145–51
- DeRuiter J, Mayfield C-A. Inhibitory activity and mechanism of inhibition of the N-[[(4-benzoylamino)phenyl]sulfonyl]amino acid aldose reductase inhibitors. Biochem Pharmacol 1990;40:2219–26
- Haraguchi H, Hayashi R, Ishizu T, Yagi A. A flavone from Manilkara indica as a specific inhibitor against aldose reductase in vitro. Planta Med 2003;69:853–5
- Terashima H, Hama K, Yamamoto R, et al. Effects of a new aldose reductase inhibitor on various tissues in vitro. J Pharmacol Exp Ther 1984;229:226–30
- Camuesco D, Comalada M, Rodríguez-Cabezas M-E, et al. The intestinal anti-inflammatory effect of quercitrin is associated with an inhibition in iNOS expression. Br J Pharmacol 2004;143:908–18
- Kwon H-K, Murakami A, Tanaka T, Ohigashi H. Dietary rutin, but not its aglycone quercetin, ameliorates dextran sulfate sodium-induced experimental colitis in mice: attenuation of pro-inflammatory gene expression. Biochem Pharmacol 2005;69:395–406
- Comalada M, Camuesco D, Sierra S, et al. In vivo quercitrin anti-inflammatory effect involves release of quercetin, which inhibits inflammation through down-regulation of the NF-kappaB pathway. Eur J Immunol 2005;35:584–92
- Guazelli C-F, Fattori V, Colombo B-B, et al. Quercetin-loaded microcapsules ameliorate experimental colitis in mice by anti-inflammatory and antioxidant mechanisms. J Nat Prod 2013;76:200–8
- Crespy V, Morand C, Besson C, et al. Quercetin, but not its glycosides, is absorbed from the rat stomach. J Agric Food Chem 2002;50:618–21
- Manach C, Scalbert A, Morand C, et al. Polyphenols: food sources and bioavailability. Am J Clin Nutr 2004;79:727–47