Abstract
2-Acetyl-4-((1R,2S,3R)-1,2,3,4-tetrahydroxybutyl)imidazole (THI) is observed as a minor contaminant in caramel food colourings (E 150c). Feeding experiments with rodents have revealed a significant lymphopenic effect that has been linked to the presence of THI in these food colourings. Pyridoxal kinase inhibition by THI has been suggested, but not demonstrated, as a mode of action as it leads to lowered levels of pyridoxal-5′-phosphate, which are known to cause lymphopenia. Recently, THI was also shown to inhibit sphingosine-1-phosphate lyase causing comparable immunosuppressive effects and derivatives of THI are being developed for the treatment of rheumatoid arthritis in humans. Interestingly, sphingosine-1-phosphate lyase activity depends on pyridoxal-5′-phosphate, which in turn is provided by pyridoxal kinase. This report shows that THI does inhibit pyridoxal kinase with competitive and mixed-type non-competitive behaviour towards its two substrates, pyridoxal and ATP, respectively. The corresponding inhibition constants are in the low millimolar range.
Introduction
Aside of its pleasant taste, the colouring properties of caramel make it a widely used food additive. Depending on their manufacturing, caramel food colourings are divided into four classes, i.e. caramel colourings of classes I, II, III and IVCitation1. Among these, caramel of colour classes III (E 150c) and IV (E 150d) are prepared by heating carbohydrates in the presence of ammonium compounds (III) and, additionally, sulfite (IV). During caramelization, these ammonium compounds serve as a source of nitrogen in the formation of a number of food contaminants including a range of imidazoles, one of them being 2-acetyl-4-((1R,2S,3R)-1,2,3,4-tetrahydroxybutyl)imidazole (THI, ). Interestingly, although ammonia is added during preparation of both classes III and IV caramel food colourings, THI is only observed in class III productsCitation2. Such caramel colourings are used in a variety of food preparations, e.g. sauces, soups, bakery or meat products, with an estimated daily mean exposure of 300 mg/kg bodyweightCitation3. Today, caramel food colourings (classes I–IV) account for >80% of all colorants added to foods and their annual global consumption exceeds 200 000 tonsCitation4,Citation5.
Figure 1. Molecular structures of THI (1, 2-acetyl-4-((1R,2S,3R)-1,2,3,4-tetrahydroxybutyl)imidazole), pyridoxal-5′-phosphate (2) and ATP (3).
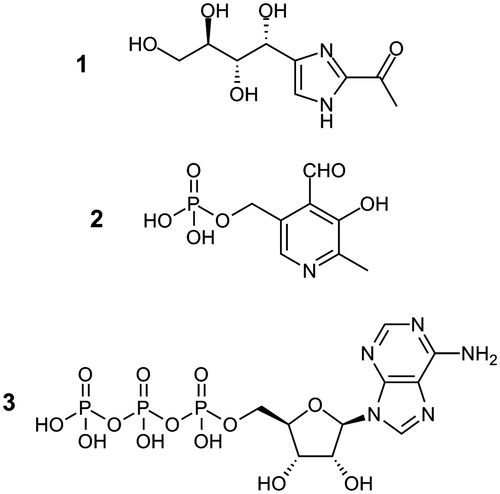
Obviously, caramel food colourings are a chemically rather ill-defined group of food additives as they are mixtures of various caramelization products. Observations of hysteria and convulsions made when feeding livestock feeds supplemented by ammoniated molasses also raised concerns about the safety of caramel food colourings, especially of those of class IIICitation6. Following a 1970 initiative by the Joint FAO/WHO Expert Committee on Food Additives (JECFA), caramel food colourings have therefore been subject of numerous toxicological investigationsCitation7. Noteworthy, rat feeding experiments carried out with high concentrations of caramel class III food colourings revealed a reduction in circulating lymphocyte countsCitation6,Citation8. This lymphopenic effect has since been the focus of extensive toxicity studies and was shown to be linked to vitamin B6 metabolism. In 1985, THI was identified as the imidazole-derived contaminant of caramel class III food colourings triggering the observed lymphopeniaCitation9–11.
Yet, until today the molecular mechanism linking THI exposure to lymphopenia has not been revealed. Taking into account that lymphopenia can occur as a result of vitamin B6 deficiency, inhibition of human pyridoxal kinase (EC 2.7.1.35), which catalyzes the random Bi Bi ATP-dependent phosphorylation of pyridoxal to pyridoxal-5′-phosphate (the active form of vitamin B6), has been considered as a possible mechanism of action ()Citation11. Human pyridoxal kinase has been shown to be inhibited by a range of substances of natural and synthetic origin, especially by small heterocyclic compounds. Some well-known drugs inhibit human pyridoxal kinase, causing a number of unwanted neurotoxic side effectsCitation12–15.
Some reports, however, question that the pyridoxal kinase inhibiting properties of class III caramel colourings actually originate from THICitation16. Recently, it was discovered that THI inhibits sphingosine-1-phosphate lyase leading to increased intracellular levels of sphingosine-1-phosphate, which in turn may also cause lymphopeniaCitation17. Details about the mode of interaction have not yet been defined, however, a binary interaction between sphingosine-1-phosphate lyase and THI has been ruled out, while a more complex interplay has been suggested. Interestingly, sphingosine-1-phosphate lyase requires pyridoxal-5′-phosphate as a cofactor to catalyze the irreversible cleavage of sphingosine-1-phosphate into hexadecanal and phosphoethanolamine. Hence, THI might affect sphingosine-1-phosphate lyase activity in two different ways, i.e. by direct inhibition and by lowering the levels of the required cofactor through pyridoxal kinase inhibition. Here, we report the results of a detailed investigation on pyridoxal kinase inhibition by THI.
Materials and methods
Chemicals
THI was prepared as reported previously at ELFI Analytik GbR (Neufahrn, Germany)Citation2. The purity of the THI sample used for the experiments was assessed by LC−MS and combustion analysis. LC−MS: purity 99%. MS (ESI+): m/z calcd for C9H14N2O5 230.1, found 231.4 [M + H]+, 253.0 [M + Na]+. Anal. Calcd for C9H14N2O5: C, 47.0; H, 6.1; N, 12.2. Found: C, 46.7; H, 6.2; N, 12.1. All other reagents were provided by Sigma–Aldrich (Milano, Italy) and were used without further purification.
Enzyme inhibition studies
A 0.5 M solution of THI in water was prepared by addition of an equimolar amount of HCl and used in this form for the assays. Wild-type human pyridoxal kinase was expressed and purified as previously publishedCitation18, except for final dialysis that was carried out overnight against 100 mM sodium N,N-bis(2-hydroxyethyl)-2-aminoethanesulfonate (BES) buffer, pH 7.3. In vitro activity of pyridoxal kinase can easily be assessed using a conventional UV spectrophotometer taking advantage of the significant bathochromic shift that pyridoxal experiences upon phosphorylation (320–388 nm). This shift occurs as the intramolecular hemiacetal form of pyridoxal, which is predominant in aqueous solutions, cannot form because of the phosphorylation of the 5′-hydroxy groupCitation19,Citation20. Briefly, initial velocity studies for the conversion of pyridoxal to pyridoxal-5′-phosphate were followed at 388 nm in an Agilent 8454 UV/Vis diode array spectrophotometer in 100 mM sodium BES buffer, pH 7.3; the final enzyme concentration was 4 µM. All assays were performed at 37 °C in a 1 cm thermostated cuvette as previously describedCitation21. Detailed kinetic studies for the determination of the inhibition mechanism were carried out in duplicate or triplicate at different THI concentrations (4.2, 7.5, 15 and 20 mM) under two experimental conditions: (i) at a constant MgATP concentration of 1.67 mM while varying the pyridoxal concentration between 20 and 300 µM and (ii) at a constant pyridoxal concentration of 300 µM while varying the MgATP concentration between 40 µM and 1.67 mM. The kinetic parameters Ki and Kii were determined by Lineweaver–Burk analysis and corresponding secondary plots of their slopes and intercepts versus THI concentrations, following the kinetic treatment proposed by Bisswanger as highlighted in the next paragraphCitation22.
Briefly, the random Bi Bi, two-substrate rapid-equilibrium mechanism proposed for human pyridoxal kinase follows the general pathway illustrated in , in which A and B represent the two substrates. In case of a competitive inhibitor towards substrate A (pyridoxal in our case), inhibitor I (THI) would participate at every step in the kinetic mechanism in which the substrate A normally binds. Thus, the following interactions of the inhibitor with the enzyme are expected (ATP representing substrate B):
Figure 2. Schematic representation of the pathways describing the interaction of pyridoxal kinase (E) with its two substrates pyridoxal (A) and ATP (B) and their corresponding products pyridoxal-5′-phosphate (P) and ADP (Q).
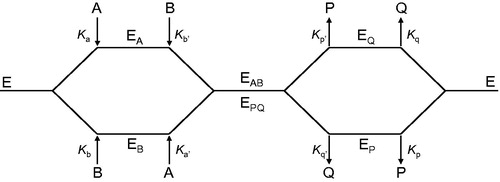
When deriving the velocity equation for the effect of the competitive inhibitor on substrate A, the following rate relationship is obtained:
(1)
When double reciprocal plots of as a function of
are prepared at different fixed concentrations of the inhibitor, only the slope term of the rate expression is altered, i.e.:
(2)
while the ordinate intercept is constant, being
When analyzing the secondary plot of slope values against [I] (middle graph of ), the abscissa intercept will result, from EquationEquation (2)(2) :
and rearranging
At quasi-saturating concentrations of substrate B, for which [B] >> Kb′ the following simplification can be made:
and, consequently
On the other hand, when B is the variable substrate, double reciprocal plots at different fixed concentrations of the inhibitor show increases in both slopes and ordinate intercepts, i.e.:
From secondary plots of slopes and ordinate intercepts versus [I] (, middle and bottom, respectively), the values of Ki and Kii can be determined. Furthermore, EquationEquation (1)(1) predicts that following a random Bi Bi mechanism a dead end competitive inhibitor for substrate A must be a non-competitive inhibitor of substrate B, and vice versaCitation22.
Figure 4. Kinetic analysis of pyridoxal kinase inhibition by THI with respect to the cosubstrate ATP shows that THI acts as a non-competitive inhibitor. Lineweaver–Burk (top) and secondary plots (middle, bottom) reveal the inhibition constants Ki = 5.3 mM and Kii = 23.0 mM. Mean values with standard errors are shown from duplicate or triplicate measurements; THI concentrations: • 0 mM, ▪ 4.2 mM, ▴ 7.5 mM, ▾ 15.0 mM and ♦ 20.0 mM.
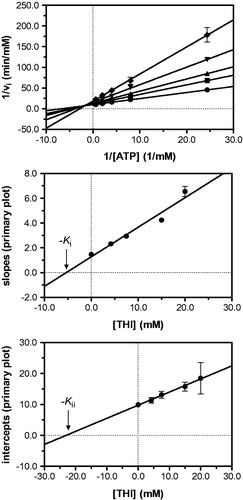
Results and discussion
Pyridoxal-5′-phosphate represents the active form of vitamin B6, a term that refers to the three vitamers pyridoxal, pyridoxine and pyridoxamine, as well as to their phosphorylated forms. As pyridoxal-5′-phosphate, it acts as a cofactor in more than 140 vital enzyme reactions and consequently a deficiency in vitamin B6 may cause a variety of neurological and non-neurological disorders, e.g. seizures, headache, agitation, tremors or hallucinationCitation23. Therefore, intracellular pyridoxal-5′-phosphate levels are constantly replenished by the catalytic action of either pyridoxal kinase or pyridoxine-5′-phosphate oxidase. While the latter catalyzes the FMN-dependent oxidation of pyridoxine or pyridoxamine phosphate, pyridoxal kinase leads to the ATP-dependent phosphorylation of the pyridoxal 5′-hydroxymethyl group. In this respect, pyridoxal kinase is of paramount importance as it is responsible for pyridoxal phosphorylation once pyridoxal is imported into the cellCitation24.
Interestingly, human pyridoxal kinase is inhibited by a range of substances of natural and synthetic origin, especially by small heterocyclic compounds, e.g. ginkgotoxin or theophyllineCitation12–15. Hence, inhibition of pyridoxal kinase by THI does not appear unlikely and we considered a detailed investigation worthwhile. As every other kinase, also pyridoxal kinase relies on a nucleotide cofactor supplying the phosphate moiety to be transferred, i.e. ATP. Pyridoxal phosphorylation by pyridoxal kinase further depends on the presence of catalytic magnesium ions, which is why MgATP is generally used in corresponding enzyme assays, although human pyridoxal kinase activity may also be regulated by monovalent cationsCitation18,Citation25. While the intracellular ATP concentration in human cells is typically in the 1–10 mM rangeCitation26, the concentration of free pyridoxal-5′-phosphate in eukaryotic cells is maintained rather low, ∼1 µM, as it is a very reactive moleculeCitation23. By now, no data on the concentration of free pyridoxal in eukaryotic cells has been reported. In E. coli cells, the concentration of pyridoxal is ∼15% of pyridoxal-5′-phosphate and pyridoxal is mainly bound to proteinsCitation27. The reported catalytic parameters of human pyridoxal kinaseCitation21 are Km (MgATP) =420 µM, Km (pyridoxal) = 30 µM and kcat = 45 min−1.
In a first series of experiments, inhibition of pyridoxal kinase with respect to the substrate pyridoxal was investigated at a constant, quasi-saturating MgATP concentration. Pyridoxal-5′-phosphate accumulation was followed spectrophotometrically in the presence of increasing THI concentrations and initial velocities of product formation were obtained by linear regression. Lineweaver–Burk analysis revealed a series of straight lines intersecting in one point on the ordinate, as it is the case with a competitive mode of inhibition indicating that THI and pyridoxal (PL) bind to the same pocket on the enzyme active site (, top). To corroborate this observation, secondary plots were prepared relating the slopes and intercepts derived from linear regression analysis of the Lineweaver–Burk data to their corresponding inhibitor concentrationsCitation22. Hereby, the nominal abscissa intercept of a line passing through the slope values reveals an apparent inhibition constant (Kii) of 11.2 mM, while the secondary graph of intercepts confirms that THI competes with pyridoxal for binding to the enzyme active site (, middle/bottom). Similar experiments were carried out at a quasi-saturating pyridoxal concentration to evaluate the inhibitory effect of THI with respect to MgATP. This time, the Lineweaver–Burk analysis produced a series of straight lines intersecting in the second quadrant to the left of the ordinate, indicating a mixed-type non-competitive mode of inhibition (, top). A corresponding set of secondary plots confirmed this finding, as slope and intercept plots provided apparent inhibition constants of 5.3 mM (Ki) and 23.0 mM (Kii), respectively (, middle/bottom).
Figure 3. Kinetic analysis of pyridoxal kinase inhibition by THI with respect to the substrate pyridoxal (PL) shows that THI acts as a competitive inhibitor. Lineweaver–Burk (top) and secondary plots (middle, bottom) reveal a Kii inhibition constant of 11.2 mM. Mean values with standard errors are shown from duplicate or triplicate measurements; THI concentrations: • 0 mM, ▪ 4.2 mM, ▴ 7.5 mM, ▾ 15.0 mM and ♦ 20.0 mM.
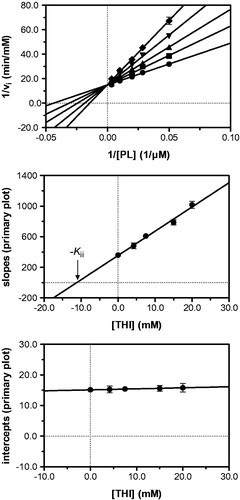
This inhibition pattern is consistent with a random Bi Bi rapid equilibrium system, in which THI is a competitive inhibitor of pyridoxal and binds with different affinities to either free enzyme or the enzyme–ATP complex. provides a schematic overview of the equilibria underlying pyridoxal kinase inhibition by THI. The white side of the cube represents the two pathways leading to a productive ternary complex (E–ATP–PL), where the free enzyme may bind both substrates following random sequential kinetics as it has been shown to be the case for pyridoxal kinaseCitation28–32. As THI and pyridoxal share the same binding site, a competitive inhibition is observed for the equilibria describing the front face of the cube (dark grey), which is why no ternary E–PL–THI complex exists. Non-competitive inhibition however characterizes the interaction of pyridoxal kinase with respect to THI and ATP that is depicted on the top face of the cube (light grey). Here, a ternary E–ATP–THI complex can be formed following two pathways, as it is the case with the productive equilibria. Following the notation described by FrommCitation33, the equilibrium of THI binding to the binary E–ATP complex is thus governed by Kii, whereas binding of THI to the free enzyme is described by Ki (). According to the inhibition pattern of a random Bi Bi rapid equilibrium system, it may be assumed that the inhibition constant obtained from the secondary plot of the slopes at constant and quasi-saturating ATP concentrations (, middle) approximately corresponds to Kii, since under these conditions most of the enzyme is present in complex with ATP. The inhibition constant found from the secondary plot of the ordinate intercepts in the mixed-type inhibition (, bottom) also corresponds to the binding of THI to the enzyme–ATP complex. This is in good agreement with the experimental values of 11.2 and 23.0 mM, which are of the same magnitude. On the other hand, the inhibition constant derived from the secondary plot of the slopes in the mixed-type inhibition (5.3 mM; , middle) corresponds to Ki, i.e. to the binding of the inhibitor to free enzyme. These results also suggest that THI binds better to the free enzyme than to the enzyme–ATP complex (Ki is smaller than Kii).
Figure 5. Schematic overview of competing equilibria describing the inhibition of pyridoxal kinase (E) by THI in the presence of both substrates pyridoxal (PL) and ATP (adapted from Asante-Appiah and ChanCitation34). Ki and Kii refer to the inhibition constants describing a random Bi Bi rapid equilibrium system in which PL and THI compete for the same enzyme binding site.
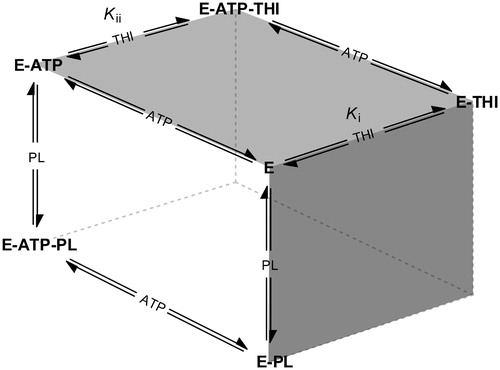
Conclusions
In summary, THI acts as an inhibitor of pyridoxal kinase being competitive with respect to pyridoxal with low to moderate millimolar affinity. In view of maximum allowed THI levels of 10 mg/kg in caramel food colourings and a daily mean exposure of 300 mg/kg bodyweight, no relevant pyridoxal kinase inhibition is expected as at 80 kg bodyweight a maximum daily uptake of 240 µg THI results. Assuming a mean body water content of 60% and a complete resorption of THI with even distribution throughout the whole body, this would provide a local THI concentration of ∼20 nM.
However, research investigating the effects of THI derivatives on sphingosine-1-phosphate lyase activity and related lymphopenia outcome should still consider pyridoxal kinase inhibition as a possible cause. In fact, the very delicate equilibrium of pyridoxal-5′-phosphate cellular availability may be perturbed even by a moderate inhibition of pyridoxal kinase, which can lead to a significant lowering of pyridoxal-5′-phosphate levels. Furthermore, when considering pyridoxal-5′-phosphate homeostasis one has to bear in mind that a local imbalance of active vitamin B6 concentrations as a result of varying local THI distribution is of uttermost importance.
Declaration of interest
The authors declare no conflict of interest.
Acknowledgements
We thank Caterina Nardella for helping with the kinetic measurements. This work was partly supported by Finanziamento Progetti di Ricerca 2011–2012 of Sapienza University of Rome.
References
- Joint FAO/WHO Expert Committee on Food Additives. Caramel colours (ammonia process). Toxicological evaluation of some enzymes, modified starches, and certain other substances 1972. No. 240
- Elsinghorst PW, Raters M, Dingel A, et al. Synthesis and application of 13C-labeled 2-acetyl-4-((1R,2S,3R)-1,2,3,4-tetrahydroxybutyl)imidazole (THI), an immunosuppressant observed in caramel food colorings. J Agric Food Chem 2013;61:7494–9
- Aguilar F, Dusemund B, Galtier P, et al. Scientific opinion on the re-evaluation of caramel colours (E 150 a,b,c,d) as food additives. EFSA J 2011;9:2004
- Kamuf W, Nixon A, Parker O, Barnum GC. Overview of caramel colors. Cereal Foods World 2003;48:64–9
- Sengar G, Sharma HK. Food caramels: a review. J Food Sci Technol 2012;49:1–11
- Gaunt IF, Lloyd AG, Grasso P, et al. Short-term study in the rat on two caramels produced by variations of the “Ammonia process”. Food Cosmet Toxicol 1977;15:509–21
- Joint FAO/WHO Expert Committee on Food Additives. Caramel colours. Thirteenth report. Geneva: WHO; 1970:10–12
- Evans JG, Butterworth KR, Gaunt IF, Grasso P. Long-term toxicity study in the rat on a caramel produced by the “half open-half closed pan” ammonia process. Food Cosmet Toxicol 1977;15:523–31
- Kröplien U, Rosdorfer J, van der Greef J, et al. 2-Acetyl-4(5)-(1,2,3,4-tetrahydroxybutyl)imidazole: detection in commercial caramel color III and preparation by a model browning reaction. J Org Chem 1985;50:1131–3
- Sinkeldam EJ, de Groot AP, van den Berg H, Chappel CI. The effect of pyridoxine on the number of lymphocytes in the blood of rats fed caramel colour (III). Food Chem Toxicol 1988;26:195–203
- Spector R, Huntoon S. Effects of caramel color (ammonia process) on mammalian vitamin B6 metabolism. Toxicol Appl Pharmacol 1982;62:172–8
- Kästner U, Hallmen C, Wiese M, et al. The human pyridoxal kinase, a plausible target for ginkgotoxin from Ginkgo biloba. FEBS J 2007;274:1036–45
- Ubbink JB, Bissbort S, Vermaak WJ, Delport R. Inhibition of pyridoxal kinase by methylxanthines. Enzyme 1990;43:72–9
- Gandhi AK, Desai JV, Ghatge MS, et al. Crystal structures of human pyridoxal kinase in complex with the neurotoxins, ginkgotoxin and theophylline: insights into pyridoxal kinase inhibition. PLoS One 2012;7:e40954
- Lainé-Cessac P, Cailleux A, Allain P. Mechanisms of the inhibition of human erythrocyte pyridoxal kinase by drugs. Biochem Pharmacol 1997;54:863–70
- Houben GF, van den Berg H, Kuijpers MH, et al. Effects of the color additive caramel color III and 2-acetyl-4(5)-tetrahydroxybutylimidazole (THI) on the immune system of rats. Toxicol Appl Pharmacol 1992;113:43–54
- Bagdanoff JT, Donoviel MS, Nouraldeen A, et al. Inhibition of sphingosine-1-phosphate lyase for the treatment of autoimmune disorders. J Med Chem 2009;52:3941–53
- Musayev FN, di Salvo ML, Ko T-P, et al. Crystal structure of human pyridoxal kinase: structural basis of M+ and M2+ activation. Protein Sci 2007;16:2184–94
- Metzler DE, Snell EE. Spectra and ionization constants of the vitamin B6 group and related 3-hydroxypyridine derivatives. J Am Chem Soc 1955;77:2431–7
- Nakamoto K, Martell AE. Pyridoxine and pyridoxal analogs. IV. Ultraviolet spectra and solution equilibria of 3-methoxypyridine-2(and 4-)-aldehydes and of 3-hydroxypyridine-2(and 4-)-aldehydes. J Am Chem Soc 1959;81:5863–9
- Di Salvo ML, Hunt S, Schirch V. Expression, purification, and kinetic constants for human and Escherichia coli pyridoxal kinases. Protein Expr Purif 2004;36:300–6
- Bisswanger H. Enzyme kinetics: principles and methods. Weinheim, Germany: Wiley-VCH Verlag GmbH & Co. KGaA; 2008
- Di Salvo ML, Safo MK, Contestabile R. Biomedical aspects of pyridoxal 5′-phosphate availability. Front Biosci 2012;4:897–913
- Di Salvo ML, Contestabile R, Safo MK. Vitamin B6 salvage enzymes: mechanism, structure and regulation. Biochim Biophys Acta 2011;1814:1597–608
- Lainé-Cessac P, Allain P. Kinetic studies of the effects of K+, Na+ and Li+ on the catalytic activity of human erythrocyte pyridoxal kinase. Enzyme Protein 1996;49:291–304
- Beis I, Newsholme EA. The contents of adenine nucleotides, phosphagens and some glycolytic intermediates in resting muscles from vertebrates and invertebrates. Biochem J 1975;152:23–32
- Fu T-F, di Salvo ML, Schirch V. Distribution of B6 vitamers in Escherichia coli as determined by enzymatic assay. Anal Biochem 2001;298:314–21
- Churchich JE, Wu C. Brain pyridoxal kinase. Mechanism of substrate addition, binding of ATP, and rotational mobility of the inhibitor pyridoxaloxime. J Biol Chem 1981;256:780–4
- Gandhi AK, Ghatge MS, Musayev FN, et al. Kinetic and structural studies of the role of the active site residue Asp235 of human pyridoxal kinase. Biochem Biophys Res Commun 2009;381:12–15
- Safo MK, Musayev FN, di Salvo ML, et al. Crystal structure of pyridoxal kinase from the Escherichia coli pdxK gene: implications for the classification of pyridoxal kinases. J Bacteriol 2006;188:4542–52
- Safo MK, Musayev FN, Hunt S, et al. Crystal structure of the PdxY Protein from Escherichia coli. J Bacteriol 2004;186:8074–82
- Li M-H, Kwok F, Chang W-R, et al. Crystal structure of brain pyridoxal kinase, a novel member of the ribokinase superfamily. J Biol Chem 2002;277:46385–90
- Fromm HJ. Initial rate enzyme kinetics. London: Springer; 2012
- Asante-Appiah E, Chan WW. Analysis of the interactions between an enzyme and multiple inhibitors using combination plots. Biochem J 1996;320:17–26