Abstract
The hypoxic areas of solid cancers represent a negative prognostic factor irrespective of which treatment modality is chosen for the patient. Still, after almost 80 years of focus on the problems created by hypoxia in solid tumours, we still largely lack methods to deal efficiently with these treatment-resistant cells. The consequences of this lack may be serious for many patients: Not only is there a negative correlation between the hypoxic fraction in tumours and the outcome of radiotherapy as well as many types of chemotherapy, a correlation has been shown between the hypoxic fraction in tumours and cancer metastasis. Thus, on a fundamental basis the great variety of problems related to hypoxia in cancer treatment has to do with the broad range of functions oxygen (and lack of oxygen) have in cells and tissues. Therefore, activation–deactivation of oxygen-regulated cascades related to metabolism or external signalling are important areas for the identification of mechanisms as potential targets for hypoxia-specific treatment. Also the chemistry related to reactive oxygen radicals (ROS) and the biological handling of ROS are part of the problem complex. The problem is further complicated by the great variety in oxygen concentrations found in tissues. For tumour hypoxia to be used as a marker for individualisation of treatment there is a need for non-invasive methods to measure oxygen routinely in patient tumours. A large-scale collaborative EU-financed project 2009–2014 denoted METOXIA has studied all the mentioned aspects of hypoxia with the aim of selecting potential targets for new hypoxia-specific therapy and develop the first stage of tests for this therapy. A new non-invasive PET-imaging method based on the 2-nitroimidazole [18F]-HX4 was found to be promising in a clinical trial on NSCLC patients. New preclinical models for testing of the metastatic potential of cells were developed, both in vitro (2D as well as 3D models) and in mice (orthotopic grafting). Low density quantitative real-time polymerase chain reaction (qPCR)-based assays were developed measuring multiple hypoxia-responsive markers in parallel to identify tumour hypoxia-related patterns of gene expression. As possible targets for new therapy two main regulatory cascades were prioritised: The hypoxia-inducible-factor (HIF)-regulated cascades operating at moderate to weak hypoxia (<1% O2), and the unfolded protein response (UPR) activated by endoplasmatic reticulum (ER) stress and operating at more severe hypoxia (<0.2%). The prioritised targets were the HIF-regulated proteins carbonic anhydrase IX (CAIX), the lactate transporter MCT4 and the PERK/eIF2α/ATF4-arm of the UPR. The METOXIA project has developed patented compounds targeting CAIX with a preclinical documented effect. Since hypoxia-specific treatments alone are not curative they will have to be combined with traditional anti-cancer therapy to eradicate the aerobic cancer cell population as well.
Introduction
For more than 20 years it has been known that there is a correlation between the ability of solid tumours to metastasise and the degree and/or amount of hypoxic tissue in the tumourCitation1–3. Since distant metastases represent a major challenge for patient survival after radical and successful treatment of the primary tumour treatment specifically attacking hypoxic cells might have great potential for saving lives. This rationale formed the basis for the call answered by collaborative EU-financed project METOXIA which has been running for 5 years since 1st February 2009. The aim of this large project has been to seek new methods for detection of hypoxic areas in tumours and to study cellular hypoxia-driven responses which could represent new potential targets for the specific killing of hypoxic cells. The most ambitious goal was to take the first steps to develop new cancer treatments based on these targets.
The present research theme raises several challenges. First of all hypoxia is by itself a complicated conceptCitation4,Citation5. The only common factor for conditions described as hypoxia is that there is less oxygen in the microenvironment than the usual amount in normal tissues. But variability is enormous: In reality hypoxia is a collective or generic term which comprises a whole range of different micro-environmental conditions affecting different molecular regulatory processes in cells. The severity of hypoxia by means of pericellular oxygen concentration is only one aspect. The duration of hypoxia, the sequencing of hypoxic versus aerobic periods (cycling) and separation by slow or quick reoxygenation are others. Production of, as well as protection against reactive oxygen species (ROS) may be pivotal for some of the cellular responses observed. Even oxygenation of normal tissues can vary by a factor of at least eight if different tissues are taken into considerationCitation6.
Changes in oxygenation activate different regulatory responses in cells, most of these studied by the METOXIA consortium and referred to in this review. Cells are said to be able to sense the change in oxygenation. Stabilisation of the hypoxia-inducible-factor (HIF) protein has been identified as the sensor of hypoxia, activated by very modest reduction in oxygenation. The role of this hypoxia sensing system in cancer therapy is however complicated by lack of cancer-specificity. HIF is usually stabilised by a small reduction of the pericellular oxygen concentration from say, about 4% (which is a normal level in most normal tissues) to about 1% O2 (i.e. from ∼40 to ∼10 µM)Citation7. Such reduction is readily experienced in various normal tissues under certain conditions, for example in muscle under exercise.
For diagnosis and treatment of cancer some mechanisms activated at lower levels of oxygenation may be more specific for the malignant tissue. It was previously for example customary to consider hypoxia as a level of oxygenation where cellular responses to radiation became significantly reduced compared to the radio-sensitivity under “normal” oxygenation. These observations were first noted as early as in the 1930sCitation8 but were intensely studied in cancer research for many decades after two papers from 1953 and 1955Citation9,Citation10, which indicated that this radiation protection may be tumour specific since severe hypoxia was tumour-specific.
The radio-resistance of hypoxic cells was later found to be related to the high electron affinity of the oxygen moleculeCitation11, which enables even small amounts of oxygen to fix radiation-induced macromolecular damage before the damaged molecule can be restored by naturally occurring radical scavengers in the cells. So, this is not an active regulation, but rather a chemical process where oxygen reacts more readily than other chemicals to bind radiation-induced radicals. It is worth recalling the great difference in oxygenation between these two extremes: The active regulatory cascades set in motion by HIF-stabilisation at an oxygen level below 1% O2 and the electron-affinic oxygen-chemical process of fixation of radical damage which was fully counteracted only for oxygen levels below about 0.01%. The level of 1% O2 was not even considered hypoxia among radiobiologists 50 years ago. It is worth noticing that the correlation between metastasis and tumour hypoxia has been shown in animal models to be just as strong for the different types of hypoxia testedCitation3. Thus, the whole range of tumour hypoxia may have a potential for the development of new specific treatment.
In the following review most cell and tissue aspects of hypoxia, therefore, have been included as they all were covered in the METOXIA project, i.e. cell metabolism, cell migratory properties by means of epithelial-mesenchymal transition (EMT), possible new biomarkers for hypoxia imaging and potential individualised treatment, angiogenesis/lymphangigenesis, radio-modifying factors, development of new preclinical models and development of new methods for direct measurement of oxygen plus other micro-environmental factors.
Biological processes regulated by hypoxia
Molecular regulation associated with low oxygen (HIF/Notch/CAIX/pH-regulation)
The presence of tumour hypoxia and/or expression of HIFα in various tumour types have been extensively reported to correlate with increased risk of metastasisCitation12. Such associations could explain the involvement of HIF-target genes in biological processes that have an impact on the metastatic spread of cancer cells such as angiogenesis, EMT, cell motility, intra/extravasation and premetastatic nicheCitation13. In spite of this, we are still far from a comprehensive picture of the hypoxia-driven changes that lead to metastasis formation. With the aim of identifying novel HIF-target genes, we developed a bioinformatics strategy based on metanalysis of gene expression profiles of hypoxic cells combined with phylogenetic foot-printing to identify HIF binding sitesCitation14. EFNA3, a member of the ephrin type A ligands, was identified as a potential novel HIF target gene with this in silico search. Ephrins are cell surface proteins that regulate diverse biological processes by modulating cellular adhesion and repulsion and increasing evidence suggest that ephrin function might be involved in multiple aspects of tumour biologyCitation15. We were able to demonstrate that hypoxia resulted in increased expression of Ephrin-A3 protein through a mechanism involving the HIF-mediated induction of a novel group of lncRNAs encoded by the EFNA3 locus. Although, to our knowledge, this is the first description of such a complex control of gene expression in response to hypoxia, it is likely to be quite prevalent since a recent study demonstrated a profound impact of HIF on the expression of lncRNAsCitation16. Indeed, we showed that the stabilisation of HIF within human tumours is associated with increased expression of lncEFNA3 and correlates with incidence of metastasis in breast cancer patientsCitation17. More importantly, animal models of metastasis revealed a causal link between EFNA3 expression and the induction of metastatic phenotype. Finally, our data demonstrate that Ephrin-A3 expression does not affect primary tumour growth rate or angiogenesis, but instead results in increased ability to intra/extravasate from blood vessels, providing an explanation for the effects on tumour metastatic abilityCitation17. As these results identify a mechanism by which hypoxia contributes to tumour metastasis, it has also been accepted that enhanced angiogenesis in response to hypoxia is part of an adaptive response aimed at achieving increased oxygen and nutrient delivery to growing tissues. This is mediated by pro-angiogenic factors such as VEGFCitation18. On the contrary, it is expected that tumour cells decrease the levels of anti-angiogenic factors in hypoxic conditions in order to favour their own survival and growth. We found that hypoxia diminishes the levels of one such angiogenesis inhibitor, the matricellular protein thrombospondin-1 (TSP-1)Citation19. TSP-1 was the first endogenous angiogenesis inhibitor identifiedCitation20, and its expression is important for the maintenance of an anti-angiogenic microenvironment; indeed, TSP-1 loss is associated with tumour metastasis and poor outcomeCitation21. The importance of TSP-1 in maintaining normal kidney angiostasis has been previously demonstratedCitation22,Citation23. In addition, our own results have shown that hypoxia-mediated decrease on TSP-1 levels in a renal carcinoma model (ccRCC) was shown to influence cell behaviour enhancing the migratory and invasive potential in in vitro assays. However, although most of the hypoxia regulated genes are specifically mediated by HIFs, TSP-1 down-regulation in these tumour cells are not due to a HIF-mediated transcriptional regulation. Instead, Akt signalling and hypoxia-mediated decrease in PHD activity contributes to the down-regulation of TSP-1 in these cells. Therefore, it seems that hypoxia stimulates multiple signals that independently help to decrease TSP-1 levels and these may contribute to the tumour outcomeCitation19.
All these results underline the importance of a precise knowledge of the control of gene expression by hypoxia through HIF-dependent or independent mechanisms in order to obtain a full picture of the cellular adaptations to hypoxia and their impact on the progression of tumours. In this regard, we have recently shown that regulation of gene expression by HIF probably requires its cooperation with a broad set of transcription factorsCitation24. This cooperation restricts the set of functional HREs among all the available RCGTG motifs within open-chromatin regions. However, it is not the only mechanism contributing to HIF-target specificity and many other genomic features including epigenetic labels and the presence of insulatorsCitation25 also restrict the number of genes that are induced in response to hypoxia.
One of the classical HIF-1 targets is carbonic anhydrase IX (CAIX), an enzyme catalysing the reversible hydration of CO2 and participating in acid–base balance. CAIX is a transmembrane protein expressed in a broad range of solid tumours, but absent from the corresponding normal tissues. Its presence is often associated with poor prognosis and poor response to therapyCitation26. Transcription of the CAIX-encoding gene is strongly activated by the HIF-1 transcription factor, which binds immediately upstream of the transcription start siteCitation27. Hypoxia also induces the activity of CAIX through the PKA-mediated phosphorylation of its intracellular tailCitation28. The extracellular enzyme's active site catalyses the conversion of pericellular CO2 to bicarbonate ions and protons. Bicarbonate ions are transported by bicarbonate transporters across the plasma membrane to the cytoplasm, where they contribute to intracellular neutralisation, which is important for cell survival and proliferationCitation29. Protons remain at the outer side of the membrane and support extracellular acidification, which facilitates cell migration and invasion. Accordingly, CAIX is functionally involved in focal adhesion dynamics and in the pH regulating cell migration machinery as part of the spatial and functional complex with the bicarbonate transporters in the lamellipodia of moving cellsCitation30,Citation31. Thereby, CAIX protects tumour cells from hypoxia and acidosis in the tumour microenvironment and contributes to their invasive and metastatic propensity. Thus, CAIX is not only a surrogate marker of hypoxia and acidosis but also a functional component of the tumour phenotypeCitation29. This offers opportunities for several anti-cancer strategies based either on immunotherapeutic approaches, or on the enzyme inhibition by the chemical compounds binding to the active site, or on blocking the molecular mechanisms of the enzyme induction and/or activationCitation32–36.
Mitochondrial reprograming induced by hypoxia
The fine regulation of mitochondrial function has proved to be an essential metabolic adaptation to fluctuations in oxygen availability. During hypoxia, cells activate an anaerobic switch that favours glycolysis and attenuates the mitochondrial activityCitation37. This switch involves the HIF-1. We have identified a HIF-1 target gene, the mitochondrial NDUFA4L2 (NADH dehydrogenase [ubiquinone] 1 alpha sub-complex, 4-like 2). Our results, obtained employing NDUFA4L2-silenced cells and NDUFA4L2 knockout murine embryonic fibroblasts, indicate that hypoxia induced NDUFA4L2 attenuates mitochondrial oxygen consumption involving inhibition of Complex I activity, which limits the intracellular ROS production under low-oxygen conditions. Thus, reducing mitochondrial Complex I activity via NDUFA4L2 appears to be an essential element in the mitochondrial reprogramming induced by HIF-1Citation38.
Cytochrome c oxidase (Complex IV) is the most oxygen-consuming enzyme within the eukaryotic cells and catalyse the transfer of electrons from reduced Cytochrome C to molecular oxygen. We have previously identified NDUFA4 as a novel component of the mitochondrial complex IVCitation39. Whereas paralog protein NDUFA4L2 is highly induced by HIF-1 under hypoxia, NDUFA4 protein levels where markedly reduced in hypoxic cells. We demonstrate that Complex IV levels are gradually reduced when oxygen supply becomes limiting. Hypoxia exerts COX sub-unit degradation as clearly evidenced by the diminished expression of NDUFA4 which leads to reduced amount of Cytochrome c oxidase. Upon this condition, relative super-complex organisation is altered, diminishing respirosome and thus becoming more resistant to oxygen deprivation. In addition, hypoxia favours the switch between COX4-1 and COX4-2, which augments relative activity of the individual enzymes. All this data clearly indicate that oxygen tensions regulate the levels of Cytochrome c oxidaseCitation40.
Hypoxia, ER-stress and the UPR
Hypoxia causes ER stress and activation of the UPR
The mechanisms influencing hypoxia tolerance and therapy resistance in tumours are only partially understoodCitation41. HIF transcription factors promote tolerance through activation of a large number of genes that influence cellular metabolism, pH regulation and angiogenesis – phenotypes classically associated with hypoxia. Stabilisation of HIF and activation of its downstream pathways occur at relatively moderate levels of hypoxia (<2% O2), which is considerably higher than that required to cause radiation resistance (below 0.2%). Work throughout the METOXIA program has shown that more severe hypoxia (<0.2%) leads to rapid activation of the unfolded protein response (UPR)Citation42–44. The UPR is an evolutionarily conserved pathway that responds to endoplasmic reticulum (ER) stress by the activation of three ER stress sensors present within the ER membrane, PERK (EIF2AK3), inositol requiring kinase 1 (IRE1/ERN1), and activating transcription factor 6 (ATF60)Citation45. They are activated through a common mechanism involving sequestration of BIP (HSPA5) by misfolded protein from the luminal domains of the sensors. Upon activation, ATF6 translocates to the Golgi apparatus and is cleaved to release an active transcription factor, while the kinase/endoribonuclease IRE-1 excises an intron from the XBP-1 transcription factor pre-mRNA. ATF6 and XBP1 induce ER chaperones and proteins involved in ER protein maturation. PERK phosphorylates the serine 51 residue of eukaryotic initiation factor 2α (eIF2α). This event inhibits translation at the initiation step, and mitigates ER stress by reducing additional ER protein load. In addition, eIF2α phosphorylation redirects translation towards a sub-set of transcripts, including the transcription factor ATF4Citation46. ATF4 induces a large number of genes, including the transcription factor CHOP. CHOP in turn induces GADD34, which directs phosphatase activity against eIF2α, setting up a negative feedback loop to fine-tune signalling through this pathway. We have shown that hypoxia causes activation of the UPR, including all its three armsCitation42,Citation47. Activation of PERK signalling occurs within 30 min of hypoxia, and is capable of responding to rapid changes in oxygenation typical of those that occur during cyclic hypoxia in tumours.
Hypoxia induces ER stress due to defects in disulphide bond formation
The UPR is a stress response primarily tailored to alleviate proteotoxic stress originating from the ER due to the presence of unfolded or misfolded proteins in this organelle. The ER serves as the first maturation compartment for proteins destined for the extracellular space, and is home to N-linked glycosylation and disulphide bond formation, as well as further glycan processing and disulphide bond rearrangements (isomerisation), all accompanied by protein folding. Unsuccessful protein folding leads to exposed hydrophobic domains that sequester chaperones and activate the UPR which regulates translation and transcription in concert to prevent further ER cargo load and to increase ER capacity. Rapid activation of the UPR during hypoxia suggested that the ER is directly sensitive to oxygen levels, potentially resulting in impaired protein folding. Work within METOXIA has led to an understanding of the mechanistic basis for hypoxia-induced ER stress and UPR activation, and revealed a novel requirement for oxygen in protein foldingCitation47. Proper folding of proteins that mature in the ER often requires formation of disulphide bonds, which are introduced both co- and post-translationally by protein disulphide isomerase (PDI)(P4HB) and family members in a redox reaction where disulphide bonds within PDI's CXXC active site are reducedCitation48. PDI must subsequently be reoxidised, a reaction catalysed by the ER oxidases Ero1α (ERO1L) and Ero1β (ERO1LB). The ERO1-bound FAD can be reoxidised by molecular oxygen in a reaction that produces stoichiometric hydrogen peroxide (H2O2)Citation49. Recently, we demonstrated the existence of two distinct phases of disulphide bond formation in living cells with differing requirements for oxygenCitation47. We showed that co-translational disulphide bond formation occurs normally without oxygen, indicating the existence of alternative oxidants under hypoxic conditions. However, post-translational disulphide rearrangement (isomerisation) is completely dependent on oxygen. Consequently, proteins that do not require disulphide isomerisation are expressed normally even under anoxia, whereas those that require disulphide isomerisation remain unfolded in the ER. Immature ER cargo proteins remain reversibly trapped in the ER during hypoxia to variable extents, perhaps depending on the complexity and/or fidelity of disulphide bond formation and rearrangement. This effect likely represents the source of ER stress during hypoxia, since the dependency on oxygen closely mirrors that of UPR activation, and a disulphide-lacking protein was processed and transported normally through the secretory pathway in the absence of oxygen.
The UPR promotes hypoxia tolerance through autophagy and ROS detoxification
Activation of the UPR, contributes to hypoxia tolerance through supporting pro-survival and detoxification mechanisms. Several reports indicate that hypoxia itself is a very powerful trigger for the induction of autophagy, a pro-survival pathway that allows recycling of cellular constituents. Hence, autophagy is primarily localised in the hypoxic fraction of the tumourCitation50–52. The widely used and accepted autophagy marker, LC3bCitation53, is partly integrated within the autophagosome causing partial degradation during autophagy. Turnover of LC3b can therefore be used as a measure for the rate of autophagy or autophagic flux. Besides its use in determining autophagy activity, LC3b is crucial in autophagy execution. LC3b coats the extending membrane allowing it to fuse and create the autophagosome. Hence, LC3b deficiency leads to impaired autophagy and increased cell deathCitation54,Citation55. During hypoxia, autophagy is rapidly activated and induces a high autophagic fluxCitation50. The degraded LC3b requires replenishment in order to maintain the high autophagic flux. This is mediated through activation of the “PERK-arm” of the UPR. Activation of PERK leads to expression of the transcription factor, ATF4, that directly binds the LC3b-promoter, and causes transcriptional up-regulation of LC3bCitation50,Citation56 and an important autophagy activator, ULK1Citation57,Citation58. Correspondingly, cells deficient in PERK-signalling fail to transcriptionally induce LC3b and become rapidly depleted of LC3b protein during hypoxia and thus fail to maintain the autophagic process.
In the context of cycling hypoxia, cells experience elevated levels of ROS-productionCitation59. The PERK-arm of the UPR is required for direct mitigation of ROS through ATF4-dependent transcription. After PERK-activation, ATF4 transcriptionally upregulates sub-units of the cysteine/glutamate antiporter xCT (SLC7A11) and 4F2hc (SLC3A2), the glycine transporter GLYT1 (SLC6A9), CTH (Gysthathione gamma-lyase), and GCLCCitation46,Citation60,Citation61. Both cysteine and glycine are required for glutathione synthesis, where cystathionine γ-lyase can also contribute by converting cystathionine to cysteine. Furthermore, GCLC is a rate-limiting enzyme in production of gluthathione. Hence cells deficient in PERK signalling and therefore ATF4 expression display compromised cysteine-uptake, reduced glutathione production and are exposed to elevated levels of ROS. The decreased glutathione levels result in cancer cells that are sensitive to oxidative stressCitation61.
Both for chronic and acute hypoxic cells, PERK-signalling is important for adaptation and response to stress. Consequently, cells deficient in PERK-signalling display lower hypoxia tolerance and increased cell death in vitro. Tumours in which PERK-signalling is targeted have a reduced fraction of hypoxic cells and are sensitised to irradiationCitation50,Citation61.
The UPR regulates metastasis
Tumour hypoxia has also been recognised as an important contributor to the distant metastasis of several human cancers, including cervixCitation62. Likewise, in vitro exposure of cancer cell lines to hypoxia increases pulmonary metastasisCitation1 and increasing tumour hypoxia in vivo increases metastasis in xenograft modelsCitation63. Experimental studies aimed at elucidating the signalling events underlying hypoxia-induced metastasis have largely focused on the HIF1 pathway. However, we have demonstrated that hypoxic activation of the UPR also drives the metastatic phenotype in an orthotopic animal model of human cervical carcinomaCitation64. Previous studies using this model have shown that subjecting animals carrying primary cervix tumours to conditions of cyclic hypoxia directly enhances metastatic spread to the local lymph nodes. We have shown that interruption of signalling through the PERK/eIF2α/ATF4-arm of the UPR in established tumours results in complete inhibition of hypoxia-driven lymph node metastasis. The changes in metastatic capacity were the result of reduced cell survival during hypoxia following disruption of the UPR. However, we also found that the PERK/ATF4 target gene LAMP3Citation65, a metastasis-associated gene, is a key mediator of hypoxia-driven lymph node metastasis. Silencing LAMP3 expression significantly inhibited lymph node metastasis in response to hypoxia, but did not affect hypoxia tolerance or tumour growth. Instead, we found evidence for a role of LAMP3 in regulating hypoxia-induced cell migration. We also investigated LAMP3 expression in human cervix tumours, one of the cancer types in which hypoxia is known to stimulate metastasis, and demonstrated that LAMP3 is regulated by both amplification as well as by hypoxia. These findings suggest that the poor prognosis of patients with hypoxic cervix cancer is due in part to PERK/eIF2α/ATF4 activation of LAMP3 and increased metastatic capacity.
The UPR as a target for therapy
All three arms of UPR have been shown to contribute to cancer cell survival while PERK and IRE1α have been the most studied and most promising as pharmacological targets. IRE1 is a highly conserved signalling arm of the UPR and, sequencing of cancer genomes revealed IRE1αCitation66 and its target, XBP1Citation67, to have higher frequency of mutations in cancer. Cells deficient in XBP1 have decreased hypoxic cell survival in vitro and a delay in tumour growth in vivoCitation68. Similarly, our group has shown that IRE1α knockdown cells have impaired proliferation under hypoxiaCitation69. However, the PERK arm of UPR appears to have the most significant role in tumour hypoxia tolerance and survival. This may be attributed to PERK's ability to transcriptionally (e.g. ATF4) and translationally (eIF2α phosphorylation) control genes essential in cell survival and homeostasis. The importance of the UPR during hypoxia, and in the survival of cancer cells subjected to ER stress, has stimulated interest from industry to develop inhibitors against both PERKCitation70,Citation71 and IRE1Citation72,Citation73 pathways. Pharmacologic targeting of IRE1α signalling exclusively focused on either its RNase domain or the ATP-binding pocket. Such RNase domain inhibitors include STF-083010Citation74 and 4 µ8CCitation72 prevent splicing of the intron in XBP1 mRNA. APY29 or sunitinib inhibit the ATP-binding pocket but allosterically activate the RNaseCitation75, while compound 3 () is an inhibitor of both active sitesCitation76. In pre-clinical in vivo studies, STF-083010 slowed the growth of human multiple myeloma xenograftsCitation74 proving potential for use of IRE1 inhibitors for the treatment of this cancer. The PERK pathway has been targeted either through inhibition of the PERK kinaseCitation77, or its target eIF2αCitation78. The optimised and orally active PERK inhibitors, GSK2656157, inhibited growth of a number of pancreatic and multiple myeloma tumour xenograftsCitation70. Our group utilised potent small-molecule inhibitors of IRE1α endonuclease activity, 4 μ8cCitation72 and PERK kinase activity, GSK compound 39Citation77, and compared the therapeutic potential of targeting these two different arms of the UPR. Surprisingly, we found that selective and potent inhibition of IRE1 splicing activity had no effect on cell proliferation or survival of cells exposed to hypoxia. This was in contrast to inactivation of PERK, which, like the genetic modelsCitation50,Citation61 substantially reduced tolerance to hypoxia and other ER stress-inducing agentsCitation50. The success of PERK inhibitors and the discovery of important downstream survival pathways regulated by PERK during ER stress such as, autophagy, anti-oxidant system and protein folding, have placed PERK at the forefront of potential UPR therapeutic targets in cancer.
The role of ROS in hypoxia
Superoxide and other ROS have been previously related to oxidative stress conditions, leading to the damage of cellular proteins, RNA, DNA, and lipids, and subsequently to the pathology of different diseases including tumour initiation and progression.
In recent years, superoxide and other ROS have been acknowledged to act as important signalling molecules in various physiological and pathophysiological conditions. Since superoxide is derived from molecular oxygen, ROS and ROS-dependent signalling appear to be connected in different ways to the pathways involved in the adaptation towards hypoxia.
One of the major pathways regulated by oxygen availability relies on the activity of HIF. Originally described to be only induced and activated under hypoxia, accumulating evidence suggests that HIFs play a more general role in the response to diverse cellular activators and stressors, many of which use ROS as signal transducers. On the other hand, the HIF pathway has also been implicated in controlling some ROS-generating systems such as NADPH oxidases. Thus, an important cross-talk exists between ROS signalling systems and the HIF pathway which appears to have implications for the pathogenesis of various disorders including cancer.
ROS and antioxidants
Superoxide anion radicals ) are formed from molecular oxygen by acquisition of an electron and can further react to other ROS such as hydrogen peroxide (H2O2), hydroxyl radicals (OH•), peroxynitrite (ONOO−), hypochlorous acid (HOCl) and singlet oxygen (1O2).
is not freely diffusible, but can cross membranes via ion channelsCitation79,Citation80. H2O2 on the other hand, which is not a radical, is diffusible and has therefore been frequently considered to act as second messenger. In the presence of iron, superoxide and hydrogen peroxide can lead to the formation of highly reactive hydroxyl radicals which can damage cellular proteins, RNA, DNA, and lipids. Interaction of ROS with nitric oxide (NO) or fatty acids can lead to the formation of peroxynitrite or peroxyl radicals, respectively, which are also highly reactiveCitation81.
Antioxidant enzymes and antioxidant scavengers contribute to control the levels of ROS and to prevent oxidative stress reactions. The nuclear transcription factor Nrf2 has been considered to play an important role in regulating gene expression of antioxidant enzymesCitation82. Among the most prevalent antioxidant systems are superoxide dismutases (Cu/Zn SOD, EC SOD, and Mn SOD), catalase, glutathione peroxidases (GPX), thioredoxins (TRX) and peroxiredoxins (Prxs)Citation83. Antioxidant scavengers are predominantly of dietary origin. These biomolecules include tocopherol, ascorbic acid, carotenoids, uric acid, and polyphenols.
NADPH oxidases generate superoxide
Among the enzymatic systems which have been shown to be able to release ROS, NADPH oxidases are unique in that their sole function is to generate ROS. NADPH oxidases have been identified in leukocytes as part of the innate immune response. Subsequently, NADPH oxidases have been identified in many non-phagocytic cells including vascular cells and tumour cells (for review refer: Gorlach et al.Citation84 and Bedard & KrauseCitation85). NADPH oxidases are multi-protein enzymes which transfer an electron from NADPH to oxygen to generate . Major components of the NADPH oxidases are transmembrane NOX proteins. To date, 5 homologous NOX proteins termed NOX1 to NOX5 have been identified. Apart from NOX5, all NOX proteins form together with the protein p22phox a cyctochrome b558Citation86,Citation87.
The complex composition of the different NADPH oxidases allows them to respond to a variety of stimuli such as growth factors, cytokines, hormones, vasoactive factors and coagulation factors mainly via receptor-operated signalling pathwaysCitation88. In addition, NADPH oxidase activity can also be regulated at the level of expression, whereby transcriptional mechanisms seem to be the most prevalent pathways. This allows NADPH oxidases to respond also to changes in micro-environmental conditions including variations in oxygen availability.
Reactive oxygen species modulate hypoxia-inducible factors
Early evidence indicated that the hypoxia-inducible transcription factor HIF-1 is redox sensitive since treatment of purified HIF-1 with H2O2, diamide or N-ethyl-maleimide prevented the ability to bind DNA under hypoxic conditions, while dithiothreitol could preserve DNA binding, suggesting that HIF-1 DNA binding requires reducing conditionsCitation89. Trx has been shown to enhance HIF-1α protein levels due to interaction of the Trx effector Ref-1 with the HIF-1α TADN and TADC, a reaction which seemed to be dependent on the redox state of cysteine 800 in HIF-1α and cysteine 848 in HIF-2αCitation90,Citation91. Mutation of cysteine 800 prevented the decrease in HIF-1α TADC activity in response to hydroxyl radicals (OH•)Citation92 supporting the notion that a reducing environment is preferential for stabilisation and functioning of HIF-α proteins.
In contrast, while administration of external H2O2 or expression of MnSOD seemed to prevent hypoxic induction of HIF-1α in tumour cellsCitation90,Citation93 application of low concentrations of H2O2 under normoxic conditions or overexpression of Cu/ZnSOD or MnSOD increased the levels of HIF-1α in vascular cellsCitation94–97 but also in Hep3B cellsCitation98. Subsequent evidence was provided that HIF-α proteins are responsive to a variety of non-hypoxic stimuli in a ROS-dependent manner, including, insulinCitation99, growth factorsCitation97,Citation100,Citation101, thrombinCitation97, angiotensin-IICitation101, peptidesCitation102, TNF-alpha and cytokinesCitation103,Citation104, the “hypoxic mimetic” CoCl2Citation94,Citation98 and various other stressors. Several studies identified NADPH oxidases as important sources of ROS in the regulation of HIF-α in vascular cellsCitation95–97,Citation102,Citation105,Citation106. NOX4 was shown to control HIF-1α and HIF-2α levels in smooth muscle cellsCitation95,Citation96. NOX2 and NOX5 which are important for endothelial ROS generationCitation86 also play a role in the regulation of HIF-1αCitation102,Citation106 in these cells.
NADPH oxidases and HIF in tumour cells
Since NADPH oxidases have been shown to up-regulate angiogenic factors known to be controlled by HIF such as VEGF or PAI-1 and to promote angiogenesisCitation102,Citation107. ROS generation by NADPH oxidases might also contribute to tumour angiogenesis. In fact, xenografts deficient in NADPH oxidase activity had reduced vascularisation (Görlach et al. unpublished observation). In support, increasing evidence suggests that NADPH oxidases are also expressed in tumour cells and are important regulators of tumour growth and progressionCitation108. Since HIF transcription factors play a central role in tumour biology, a cross-talk between NADPH oxidases and HIFs may be important also in tumour biology. In line with this assumption, tumour treatment with hyperthermia was shown to enhance NOX1 and subsequently HIF-1α levels in tumour cellsCitation109. NOX1 was also shown to increase HIF-1α levels in lung tumour cellsCitation110 while NOX4 knockdown decreased HIF-1α levels in ovarian cancer cellsCitation111. Depletion of NOX4 or NOX1 reduced HIF-2α levels in VHL-deficient 786-O or RCC4 renal carcinoma cells suggesting that ROS may act via a VHL-dependent pathwayCitation112,Citation113. Interestingly, p22phox was shown to maintain HIF-2α protein levels through inactivation of tuberin and downstream activation of ribosomal protein S6 kinase 1/4E-BP1 pathwayCitation114, and to promote xenograft growth (Görlach et al. unpublished observation). These data indicate that NADPH oxidases are important sources of ROS in a variety of non-hypoxic pathways also in tumour cells.
Mechanisms of HIF regulation by reactive oxygen species and NADPH oxidases
ROS have been shown to regulate HIF-α levels by different mechanisms. H2O2 application or NOX4 overexpression increased HIF-2α TADN and TADC activity and this response was abolished upon mutation of the target prolines or asparagines, respectivelyCitation96. Similar observations were made with HIF-1α indicating that ROS can affect HIF-α stability by interfering with the PHD/pVHL pathway. In junD-deficient cells, ROS interfered with Fe(II) availability in the HIF prolyl hydroxylase catalytic site possibly by a Fenton-type reaction thus diminishing HIF-1α hydroxylation and allowing its accumulationCitation115. Similarly, NOX4-dependent ROS generation decreased the availability of Fe(II) thereby increasing HIF-1α levelsCitation96. Addition of iron, on the contrary, increased HIF-1α degradationCitation116 indicating that the balance between Fe(II) and Fe(III) is of major importance in controlling HIF-α levels. In this context, ascorbate seems to ac via maintaining Fe(II) levels in the cell thereby controlling PHD/FIH hydroxylase activityCitation117. Subsequently, provision with ascorbate decreases HIF-α levels under non-hypoxic conditionsCitation94,Citation96,Citation97,Citation116,Citation118. On the other hand, thrombin and angiotensin-II decreased cellular ascorbate levels while increasing HIF-α levelsCitation96,Citation118 further suggesting that ascorbate availability may provide an important mechanism in the regulation of HIF-α under non-hypoxic conditions. Similarly, other reducing agents such as glutathione and dithiothreitol can promote HIF hydroxylase activity further indicating that the cellular redox state is important in controlling PHD activityCitation119. Mutation of a previously recognised redox-sensitive cysteine in PHD2Citation120 increased basal hydroxylation rates and conferred resistance to oxidative damage in vitro, suggesting that this surface-accessible PHD2 cysteine residue is a target of anti-oxidative protection by vitamin C and glutathioneCitation121.
In addition to regulation of HIF-α at the level of protein stability induction of HIF-1α has been described to be regulated by a transcriptional mechanism in a ROS-dependent manner (for review, refer Gorlach, 2009Citation122). Subsequently, it was shown that HIF-1α is a direct target gene of NFκBCitation95,Citation123–127, and that ROS derived from NADPH oxidases or direct application of H2O2 regulate NFκB-dependent HIF-1α transcription Citation95,Citation106,Citation128. These findings indicate that transcriptional regulation of HIF-1α by ROS-sensitive activation of NFκB may represent an important mechanism how agonists can induce HIF-1α under non-hypoxic conditions and provide a pathophysiologically interesting link between these two important redox-sensitive transcription factors with various implications not only for inflammatory conditions, but also for cancer.
In contrast to HIF-1α, only limited data are available on the regulation of HIF-2α mRNA and the contribution of ROS. It has been shown that NOX4 depletion decreased HIF-2α mRNA levels in RCC4 cellsCitation113although the underlying mechanisms have not been elucidated. Since the NFκB binding site which mediates transcriptional regulation of HIF-1α does not seem to be conserved in the HIF-2α promoter, this may be an important factor determining non-redundant functions of HIF-1α and HIF-2α in hypoxic and non-hypoxic conditions. In hematopoietic stem cells stimulated with erythropoietin, HIF-2α was identified as a direct Stat5 target gene. Although not explicitly studied, this mechanism may also involve ROSCitation129.
Subsequently, HIF-1α protein synthesis has been considered to be regulated by cap-dependent signalling processes, mediated mainly through PI3K/Akt and mTOR in particular in response to tyrosine kinase signallingCitation130,Citation131. ROS derived from NADPH oxidases and mitochondria have been shown to be able to activate PI3K/Akt signalling in normal and malignant cellsCitation81,Citation97,Citation132,Citation133 and have been implicated in translational regulation of HIF-1α in smooth muscle cells in response to angiotensin-IICitation134. In addition it was proposed that the PI3K pathway in conjunction with NFκB may be involved in the translational regulation of HIF-1α in response to TNF-αCitation135. Recently, it was shown that HIF-2α mRNA translation was controlled by p22phox by a mechanism involving stabilisation of Rictor-associated mTORC2 complex in the absence of VHL through an eIF4E-dependent mRNA-translational mechanismCitation136. However, the relative importance of ROS-dependent HIF-α translational compared to transcriptional mechanisms and protein stabilisation has not been clarified, yet.
NADPH oxidases in the hypoxic environment
NADPH oxidases have been shown to be relevant in situations of ischaemia/reperfusion or cyclic hypoxia. In models of stroke or myocardial infarction, as well as in intermittent hypoxia simulating sleep apnoe NADPH oxidases have been described to contribute to increased ROS levelsCitation137–140. In lung tumour cells NOX1 was shown to contribute to up-regulation of HIF-1α in response to intermittent hypoxiaCitation141. NOX4 was shown to contribute not only to ROS generation in response to cyclic hypoxia in different brain tumours, but also to tumour progression and radio-resistance under these conditions, similar to HIF-1αCitation142. In hindlimb ischaemia, NOX2 mediated HIF-1α regulation in the bone marrowCitation143. In addition, NOX2 was also shown to contribute to enhanced HIF-1α levels in the carotid body and in PC cells in response to intermittent hypoxia (for review, refer Prabhakar & SemenzaCitation144).
In many cases, these observations were accompanied by increased levels of NADPH oxidase sub-units including p22phox, p47phox and different NOX proteins. Since it has been shown that several NADPH oxidase sub-units can be induced by ROS, including p22phox, NOX2, NOX4 and Rac1Citation102,Citation107,Citation145 increased ROS levels in the reoxygenation/reperfusion periods may be responsible for such an up-regulation thereby promoting sustained ROS generation under these conditions.
In addition, NADPH oxidases have also been shown to play a role in the response to sustained hypoxia. NOX2−/− mice were protected against the development of hypoxia-induced pulmonary hypertensionCitation146, and this effect was concomitant with decreased ROS levels. Similarly, hypoxia further decreased release in p47phox-deficient perfused lungsCitation147 indicating that NADPH oxidases contribute to ROS generation under hypoxia.
In fact, hypoxia can induce the levels of several NADPH oxidase sub-units, including NOX4Citation148,Citation149. Induction of NOX4 by hypoxia helps to maintain ROS generation under hypoxia, and is responsible for increased ROS generation upon reoxygenation in vascular cellsCitation148. Interestingly, hypoxic induction of NOX4 has been shown to contribute to hypoxic HIF1α upregulation in different cell types similar to the situation under normoxiaCitation148,Citation150. Conversely, NOX4 was identified a genuine HIF-1α target geneCitation148. Functional hypoxia response elements were also identified in the human NOX2 and Rac1 promoters indicating that NADPH oxidases as genuine HIF target genes are involved in the adaptive response to hypoxiaCitation102,Citation105. Experiments in mice deficient in endothelial HIF-1α confirmed the relevance of this transcription factor in the regulation of NADPH oxidasesCitation102. Although the exact importance of hypoxia and HIF-dependent up-regulation of NADPH oxidases is still not clarified, one may speculate that a certain level of ROS is important for maintaining basal cellular functions under oxygen-limited conditions and may thus help to protect against apoptosis or cell death at least at the cellular level. Furthermore, in leukocytes and other immune cells, induction of NADPH oxidases under hypoxic conditions may contribute to initiation and propagation of inflammatory conditions frequently associated with conditions of oxygen deficiency. This would also explain the protective effects of NADPH oxidase deficiency seen in different animal models of intermittent and sustained hypoxia. Moreover, recent data also indicate that NADPH oxidases might be associated with outcome to tumour therapy since they can modulate the DNA damage response (Görlach et al. unpublished observation).
In essence, there is a tight cross-talk between hypoxia and HIF signalling on the one hand, and ROS and NADPH oxidases, on the other hand, which allows tight control of HIF activity dependent on the current redox state. Since fluctuations in the redox state are commonly observed in the changing tumour environment, this cross-talk might be of particular importance in the adaptive response of tumour cells to their environment with important consequences for therapeutic sensitivity.
Technology
Standardisation of in vitro cell microenvironments
Cell culture monitoring with microsensors provides insight into the cellular metabolism as well as regulatory pathways by continuous measurements using sensors for small molecules and biosensors. The knowledge of the pericellular values of typical metabolic parameters such as dissolved oxygen, pH value, glucose and lactate is essential for standardisation of cell culture experiments. Furthermore, these parameters form the basic set of variables for control of in vitro experiments (see next Section: “Microphysiometry for drug testing and cancer research”). In 2007, we introduced the concept of the Sensing Cell Culture Flask (SCCF)Citation151,Citation152 providing a technological platform for cell culture monitoring without the need to deviate from tissue culture flasks as the conventional format for cell culturing ().
Figure 1. Sensing cell culture flask (SCCF), a microsensor chip is embedded in the bottom of a conventional tissue culture flask (A), detailed view of the transparent microsensor chip (B) with its electrical contact pads outside the flask.
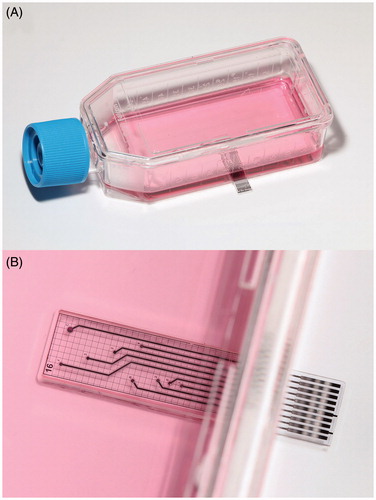
The SCCF system features a microsensor chip embedded in the bottom of a culture flask. Thus, pericellular parameters can be monitored from cells directly settling on the sensor chip. The chip itself is transparent allowing optical inspection with common phase contrast microscopes. The SCCF platform allows the integration of ideally any electrochemical or biosensor by simple adjustments during the post-processing steps of the chip fabrication. Oxygen sensors are based on platinum working electrodes covered with hydrogel acting as a diffusion limiting membrane, which were operated by chronoamperometric protocolsCitation153. An example for potentiometric sensors is the pH sensor using iridium oxide electrodes as the ion-sensitive material. Biosensors for glucose and lactate have been integrated by dispensing from a two-layer stack of hydrogels consisting of the enzyme membrane and a diffusion limiting membrane onto platinum electrodesCitation154. The enzymes used have been glucose, respectively, lactate oxidase, which convert the analyte to hydrogen peroxide, which can be subsequently oxidised at the platinum electrode. The flexibility of the SCCF technology concept also allows the integration of a specific sensor for other small molecules beyond basic metabolic parameters. The most recent enhancement of the system was the integration of sensors for superoxide.
The production of ROS, especially superoxide anions, is a common attribute of all aerobic cells. Intrinsic ROS generation is mainly linked to the mitochondrial respiration chain, whereas superoxide is produced as by-product during aerobic respiration. Other sources may be the activation of oxidoreductase enzymes and metal catalysed oxidation. The disruption of cell redox homeostasis leads to oxidative stress by decreasing ROS scavenging ability or by increased ROS production. Due to their high energy demand, cancer cells often show increased ROS production, whereas these cells are able to adapt to higher oxidative stress with the consequence of inhibited apoptosis, promoted malignant transformation and metastasis, as well as resistance to anti-cancer drugsCitation155,Citation156. Interestingly, the cell and probe handling as well as cell culture condition may attribute to measured endogenous ROS production signalsCitation157.
The measurement of superoxide levels in cell culture is often conducted by fluorescence spectroscopy or electron paramagnetic resonance (EPR) with the drawback of extensive cell culture treatment, the absence of continuous long-term monitoring ability and often selectivity issuesCitation158. Electrochemical superoxide detection by direct oxidation on modified gold electrodes offers the possibility for selective monitoring of ROS levels during in vitro cultivation. The permanent mounting of sensors directly in the culture area allows a real-time detection of ROS in the direct extracellular microenvironment without disturbing cell culture routines. The measurement principle is based on direct amperometric oxidation of superoxide anions on gold electrodes. A low oxidation potential as well as an appropriate sensor coating with a polymer layer assure a selective and sensitive radical monitoring during acute experiments.
Microphysiometry for drug testing and cancer research
In microphysiometry systems, cells are cultured on a chip, and metabolic parameters are measured non-invasively. In contrast to the SCCF (see Section “Standardisation of in vitro cell microenvironments”), medium is exchanged periodically in a stop/flow cycle. After determination of reference metabolic rates, substances can be added to the medium in order to alter the metabolism. Metabolic products and cellular behaviour upon substance exposure are then measured by the sensors. In contrast to end-point analysis, these systems allow continuous online monitoring, such as the study of pharmacodynamics and recovery effects. The measured parameters typically include extracellular acidification due to the excretion of protons and cellular adhesion to the surface. Other important parameters include oxygen consumption due to cellular respiration, or the energy metabolism, primarily glucose uptake and lactate production.
A number of different microphysiometers have been developed, most of them based on silicon chips, lacking the optical transparency for phase contrast microscopy. The Cytosensor included a light addressable potentiometric sensor (LAPS) on a silicon chip to measure pHCitation159–161. It was commercialised by Molecular Devices, and a number of modifications were developed. Amperometric biosensors were included to measure glucose and lactateCitation162,Citation163.
Cellular adhesion and morphology was measured with interdigital electrode structures (IDES), based on impedanceCitation164. Oxygen- and pH-monitoring was added by ion selective field effect transistors (ISFET) or amperometric oxygen sensing on noble metal electrodesCitation165–168. The Bionas Discovery 2500 system featured IDES for adhesion and ISFETs for pH measurement and included palladium electrodes as amperometric oxygen sensorsCitation169,Citation170. These systems were applied in pharmacological and toxicological studies or environmental monitoring.
Since optical transparency is a much needed feature, we have developed a system based on a glass chip to allow phase contrast microscopyCitation171. The basic technology and the surface on which the cells grow are shared with the SCCF. It includes electrochemical microsensors for oxygen, pH, glucose and lactate. Oxygen is measured amperometrically with platinum electrodes; pH is measured potentiometrically with iridium oxide electrodes. The medium is supplied by efficient, low volume microfluidics. The enzyme-based biosensors for glucose and lactate are integrated in the microfluidics. That allows the biosensors to be placed outside of the cell culture area to enhance biocompatibility, because the exposure of the cells to the hydrogen peroxide produced by the enzymes can be avoided.
The dimensions of the system are designed to fit the pitch of a 24-well micro-titre plate, with all sensors fully integrated on-chip (). In a first phase, cells are cultured on the glass chip at the bottom of the well for up to several days. Then, in the measurement phase, the well is sealed to form a gas-tight microfluidic system with only 10 µl total volume. The medium now needs to be exchanged periodically with a pump in stop/flow cycles. Because the medium layer above the cells is drastically reduced to only ∼100 µm, the cells' metabolism alters the medium quickly.
Figure 2. Microphysiometry chip (A). Oxygen and pH are measured in the cell culture area during the stop phase. Glucose and lactate are measured during the flow phase in the outlet microfluidic channel. Photography of microphysiometry chip with cell culture well filled for cell culturing phase (B).
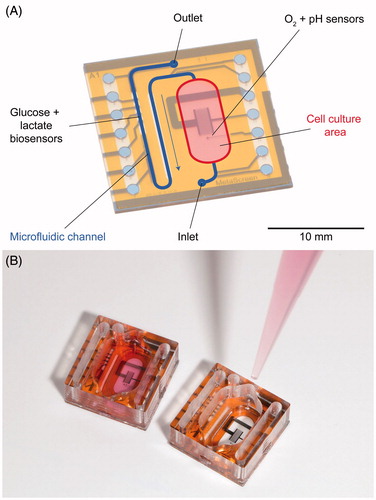
This principle gives access to cellular metabolism and measurable changes in microenvironment within a few minutes, much faster than in a large flask. Over the course of a few hours, reference metabolic rates can be determined and then altered by the addition of substance. Measurement takes place both during the stop phase, where oxygen and pH are measured in the cell chamber, and when the used medium is flushed to the biosensors in the outlet channel.
T98G human glioblastoma and T-47D breast cancer cells were cultured in the system for at least 24 h before measurements. Cell morphology can be observed at any given point due to the system's transparency. In stop/flow phases of around 5 min each, an immediate oxygen consumption and acidification was observed. In the outlet, the amount of consumed glucose and the produced lactate was quantified. The fast and efficient medium exchange by microfluidics was demonstrated. Over several hours, stable rates in a large number of cycles could be determined. These rates were altered by the addition of drugs, e.g. cytochalasin B, upon which an immediate change in cellular metabolism was determined. After supply of regular medium, the recovery of the metabolism could be observed, demonstrating the advantage of continuous monitoring in comparison to end-point tests. A low total volume per cycle of only 15 µl was achieved, reducing the consumption if the drug is available only in limited amounts.
We developed a new, transparent microphysiometry platform, including the integration of glucose and lactate biosensors and low volume microfluidics. The system allows the precise, continuous monitoring of tumour cell metabolism and the assessment of metabolic response to drug exposure.
Automation of cell culture: From routine to intelligent systems
Cell culture techniques are typically used to produce (therapeutic) antibodies and artificial tissues, provide cell material for, for example toxicity and other cell-based assays, in which mammalian cells are used as a “physiological barometer of the drug/ target interaction in HTS and early clinical profiling”Citation172.
Manual cell culture is a process that requires many hours of repetitive, painstaking operations. Furthermore, living cells require maintenance of a favourable microenvironment throughout each passage including cell growth, harvesting, reseeding, and analysisCitation173. From this point of view it is obvious, that the development of automation equipment for these repetitive steps should be a key issue for the future. This review section will give an overview of the current state of “cell nursing” in medical biotechnology with the focus on possibilities for automation.
In Germany, for instance, more than 200 biotechnology companies are working on the development of new therapies and diagnostics, thus representing the commercial successful, medical section of biotechnology, the so-called “red” biotechnology. And in this field cancer represents one of the most commonly researched diseases.
Automated high throughput cell cultivation: fully automated cell culture maintenance
In red biotechnology the highest level in automation can be found traditionally in high-throughput screening (HTS), a technology used in the early stages of drug discovery. Approximately half of the HTS assays performed are cell-based assays. That means, culturing of large amounts of mammalian cells, which are dispensed into small volumes, e.g. the mainly used multi-well micro-titre plates. This is all done in a highly standardised way, because the cells are the main “reagent” for the subsequent cell- based assays. As in manual cell culturing, the control of environmental factors is of importance and sub-cultering conditions should reliably be kept constant (relative humidity RH at 95%, 5% CO2 and a temperature of 37 °C). Robotic incubators are, beside the incubator itself, additionally equipped with tools and devices for transporting, storing and handling vessels, flasks, dishes, and bottles. Additionally media pumps and dispensing devices are needed for pipetting of media and suspended cells. The whole automatic cell culture platform is housed in hood/hoods equipped with HEPA filtersCitation174.
The “gold” standard of HTS robots is the SelecT (Sartorius, former TAP) developed for cultivation of adherent cells in flask, also in triple and HYPERflasks. A robotic arm, operating in six axes, is able to operate like a human arm. Culturing adherent cells, comprises the following processes: seeding, feeding the cells by replacing exhausted media with fresh media, and the most critical step, passaging of the cells. Passaging attached cells involves detaching enzymatically (trypsinisation) a confluent cell layer from the bottom of the vessel in several incubation and washing steps. The suspended cells are then transferred into larger or multiple vessels for further cultivation. Up to 20 different cell lines can be grown in parallel in maximal 182 flasks, all managed by the software, which also controls up to 15 media pumps. Optionally a multi-format cell dispenser for 96, 384 and 1536-well plates situated in a separate hood can be addedCitation172,Citation173,Citation175.
Both, the Freedom Evo from TecanCitation176 and the Star system from HamiltonCitation177 are based on pipetting robots. The STAR pipetting robot is designed to feed and passage adherent cells grown in multi-well plates. For cells in suspension, which need to be shaken, a small cell culture incubator add-on is available, that fits inside the STAR hood.
In an impressive example for an extended automated cell culture protocol is givenCitation177. Confluence and trypsinisation-time can be entered through a graphical user interface GUI. The system checks the actual confluence by means of an automated microscopic cell analyser platform (Cellavista, Roche, Basel, Switzerland) and analyses the image (confluence algorithm) to decide for further cultivation or for passaging. The results of a manual high- content screening experiment were compared with those obtained from the automatic system. The experimental variability was significantly smaller by using the automatic system. The Freedom Evo System, equipped also with the above-mentioned automated microscopic cell analyser, was used for isolation and culture of human primary (disc) cells, with similar yields, viability, and phenotype compared with the manual procedureCitation176. By equipping individually the standard robotic cores with add-ons enabling them to process complex protocols, will give industry new applications and potential for innovation. But the reasonability of automating for smaller cultivation jobs was scrutinised in Hogan et al.Citation172 by defining a manual commitment time (MTC) for human intervention spent for machine versus real “handmade” cell cultures. Also, the possible overflow of cell-assay ready plates is discussed using the SelecT as an example.
Miniaturisation in biotechnology reached the market with the ambr system (Sartorius, Göttingen, Germany), a cell cultivation system which uses small volume bioreactors with integrated sensors for DO and pH, which provide individual closed loop control of these parameters. The automated pH regulation is possible through control of CO2 and liquid alkali additionCitation178. But miniaturisation requires first controlling how the assay can be adapted to the changes in the microenvironment of cells in the reduced volumeCitation179. Cell stress, for example, can be evaluated by the expression of stress markersCitation180.
From perfusion culture to the “cell-nurse”
The knowledge of optimal growth conditions for microorganisms in fermentation can often be dated back to the beginning of civilisation. But even these cultivation routines were significantly improved since analytical methods for culture monitoring have become available, including molecular genetics. While for HTS and e.g. antibody production in CHO cells, well-known and stable cell lines are used, but more often cell culture characterisation is necessary, e.g. for the development of new applications or cell lines.
Batch fermentation uses all the volume of a bioreactor, which is not exchanged during the course of cultivation. While cells are growing, a deficiency in the energy substrates glucose and glutamine will be reached and the number of viable cells decreases, while the concentration of metabolic products like lactate and ammonia increases. The product of the fermentation is extracted after the end of cultivationCitation181.
Fed-batch cultivation offers a minimum of “cell-nursing” by feeding new medium to the bioreactor, because an initial low filling level allows this procedure for a limited time. Samples are drawn from the bioreactor to find the right time for the addition of new medium. Also most cell expansion protocols for HTS follow this kind of fermentation.
Perfusion culture means, that a medium is continuously exchanged while the cells are retained in the bioreactor by means of a membrane or fibresCitation182. The advantage of the perfusion method is, that products can be harvested and metabolic waste products are removed simultaneously. Additionally, glucose, glutamine, and lactate concentrations can be measured in the perfusate, and feed additions and perfusion rate can be adjusted daily to keep the residual glucose and glutamine concentration in a favourable higher range. The Wave Bioreactor, a disposable polymer bag with a floating membrane, is moved by means of a platform, which also is used for heating. In the study of Adams et al.Citation183 and Sciences GEHLCitation184, the application of this perfusion bioreactor for protein production in CHO cells is described. In Hu et al.Citation185, all three bioreactor types were used for the same cell line and the cell cultivation qualities were compared. Cell viability is best and cultivation duration is longest in the perfusion bioreactor. The perfusate was analysed once a day on different analysers or kits. Thus, external daily concentration measurement and adjusting the perfusion rate once a day according to the lab results improves the culturing quality.
Apparently, continuous monitoring of glucose and lactate and especially of glutamine in this case, would have brought further advantages. Continuously analysing the perfusate enables for a fine-tuning of the chemical cell environment: Instead of adding medium – what means adding a fixed mixture of nutrition substances – the frequent addition of calculated amounts of concentrated stock solutions will maintain the nutrition situation of the cells at preset levels and will avoid ,on the other hand, the very high perfusion rates necessarily required at higher cell densitiesCitation186.
The technical feasibility of the on-line monitoring of these key micro-environmental parameters has been shown by Moser et al.Citation187. In the work by Moser and JobstCitation188, we reviewed the monitoring with (bio)sensors in disposable bioreactors and presented Jobst Technologies' contribution to this field: A miniaturised, disposable multi-parameter biosensor arrays for glucose, lactate, and glutamine/glutamate monitoring in a micro-flow system with few-microliter internal volume comprising micro-pumps. Various different physical formats of the devices, that are factory pre-calibrated, serving different application scenarios, can be fabricated. Also integration of the sensors during disposable bioreactor bag assembly is feasible because gamma irradiation together with the bag is possible.
shows how glucose levels can be controlled in a feedback loop using the glucose biosensor, how the full panel of analytes is controlled by the array of biosensors. An important prerequisite are the miniaturised pumps developed recently for low volume applications of this kind of applications.
Drug development
State-of-the-art small molecule targeting HIF/hypoxia signalling
Tumour hypoxia presents a major challenge to the cancer biologist from a therapeutic perspective. First, most solid tumours characteristically contain areas of hypoxia that are associated with metastatic disease and resistance to treatmentCitation189. Second, increased hypoxia occurs within the tumour microenvironment in response to treatment, providing a mechanism by which tumour cells can evade growth inhibition. There is a clear unmet need for therapeutic strategies that improve current treatment outcomes by exploiting the hypoxic response. Targeting the HIF pathway inhibits tumour progression in vivoCitation189 and can block unwanted effects of therapy-induced tumour hypoxia observed with γ-radiationCitation190,Citation191 and other therapies used clinically (e.g. bevacizumab) resulting in significantly improved efficacy of these treatments in pre-clinical modelsCitation190,Citation192.
Identifying small molecules through cell-based screening
There are several sites in the HIF pathway that are potential intervention points for inhibition by small moleculesCitation189. These include inhibition of HIF-1α stability or protein synthesis, or interference of HIF-dependent interactionsCitation189,Citation193–195. A number of small-molecule inhibitors of HIF have been described, although their exact mechanism of action remains to be elucidatedCitation189. In addition, cell-based high-throughput screens are being used to identify novel small molecule inhibitors of HIFCitation189. Generally, these systems utilise cells transfected with multiple HREs linked to a specific reporter gene construct. Cells express the reporter (e.g. luciferase or β-galactosidase) in a HIF- and hypoxia-dependent manner. This allows efficient screening of large libraries of compounds for HIF-inhibitory activity. Several small molecule agents identified from cell-based screens that indirectly inhibit HIF signalling have primarily been used to probe the HIF pathway rather than being explored as drug development candidatesCitation189.
We were one of the first groups to publish the identification and characterisation of novel small molecule inhibitors of the HIF pathway using a cell-based screen that we developedCitation191. Through this approach, we have successfully identified and mechanistically evaluated novel chemical series that exhibit desirable properties for therapeutic development (e.g. novel and chemically tractable, no attributable non-specific activity, no toxicity, good bioavailability, efficacious at inhibiting tumour growth consistent with blocking the HIF pathway in vitro and in vivo) and block the unwanted effects of treatment-induced tumour hypoxiaCitation190,Citation191. We previously identified a novel HIF pathway inhibitor, NSC-134754Citation191 which we have used extensively as a tool compound for probing the HIF pathwayCitation196,Citation197. Our chemical synthesis of NSC-134754 has led to the re-assignment of its chemical structure recentlyCitation198. Further evaluation of the mechanistic, pharmacological and biological properties of NSC-134754 has provided invaluable insight for implementing a pre-clinical development pathway for the progression of other novel inhibitors that target HIF/hypoxia signalling as potential therapeutic agents.
Targeting HIF and the p53/HDM2 pathway
The p53 tumour suppressor protein is induced and activated in response to a variety of cellular stressors. p53 is a potent negative regulator of HIF, and we have shown that pharmacological activation of p53 blocks HIF-mediated responses, tumour growth and angiogenesis in vivo, and induces significant tumour cell apoptosis in hypoxiaCitation199. Therefore understanding how cell death responses are regulated in tumour cells by HIF and p53 pathways is of particular interest to usCitation199–201. Activity of wild-type p53 is usually lost due to deregulation of HDM2, an E3-ligase and well-known target of p53. HDM2 status is therefore an important consideration when determining how tumour cells may respond to therapyCitation202. We have investigated the relationship between HDM2 and the HIF pathwayCitation197,Citation203, and the effects of HDM2 inhibitors on HIF have been described previouslyCitation204–206. Nutlin-3 stabilises p53 by targeting the p53 binding pocket on the surface of HDM2 and shows potent in vivo anti-tumour activity in xenograftsCitation204–206. We have found that up-regulated HDM2 expression positively regulates HIFCitation203, HDM2 inhibitors also block angiogenesis through p53-dependentCitation207 and p53-independent mechanismsCitation208. Nutlin-3, for example, shows greater efficacy in hypoxic cells with wild-type p53, only in combination with radiotherapyCitation204.
Most small molecule agents that have been designed to induce tumour cell death by activating p53 demonstrate poor activity in hypoxia and are often used in combination for therapeutic efficacy. Interestingly, however, we have found that the small molecule activator of p53, RITA (reactivation of p53 and induction of tumour cell apoptosis) can induce significant p53-dependent tumour cell death in normoxia and hypoxia as well as activating p53-dependent DNA damage responsesCitation199,Citation201. Furthermore, DNA damage pathways that are induced by RITA also elicit cell cycle checkpoints involved in maintaining genomic integrity in response to stressCitation209.
Close links between HIF and p53 pathways have been shown in studies involving renal cell carcinomaCitation197,Citation210. Almost 80% of renal cell carcinomas occur due to the biallelic inactivation of the von Hippel-Lindau (VHL) tumour suppressor geneCitation211,Citation212. Renal cell carcinomas and hemangioblastomas usually express wild-type p53 as well as high basal levels of HIF-α due to loss of VHL function and are highly aggressive angiogenic, and metastatic cancers that remain resistant to radiotherapy and chemotherapyCitation211,Citation212. Although loss of VHL function leads to constitutive stabilisation of HIF-α, pVHL also has HIF-independent tumour suppressor functions involving other cell cycle and apoptosis pathwaysCitation213. pVHL not only associates with HIF-1α to target it for proteosomal degradationCitation214, but has also been shown to directly associate with p53 and regulate p53 transcription in a HIF-independent mannerCitation213. By binding to p53, pVHL inhibits p53 degradation by HDM2Citation213. It seems that loss of pVHL function has a critical role in promoting renal cell carcinoma by not only up-regulating the HIF pathway, but also by affecting the p53 cell cycle and apoptotic pathways. These pathways have a central role in promoting tumourigenesis in renal cell carcinoma and other hereditary cancer syndromes whereby pVHL is deregulatedCitation211,Citation212.
Harnessing the HIF/hypoxia response via novel mitochondrial mechanisms
Rapid advances in understanding metabolic switches in cancer cells has led to the development of inhibitors that sensitise tumour cells to cell death by disrupting the energy balance within mitochondria. Signalling molecules and numerous tractable targets that are critical for tumour cell metabolism are being explored for therapeutic intervention. For example, 2-deoxy-d-glucose (2-DG) targets the dependency of cancer cells for glucose and has been shown to sensitise tumours to radiation therapy and chemotherapyCitation215,Citation216. In addition, proteins involved in mitochondrial function are also being targetedCitation217. Recently, using a functional genomics approach to identify novel regulators of HIF, we characterised the functions of the human CHCHD4 (coiled-coil helix coiled-coil helix (CHCH) domain 4) mitochondrial proteins, also known as MIA40Citation218. Modulation of CHCHD4 protein expression was shown to regulate cellular oxygen consumption rate and metabolismCitation218. Importantly, knockdown of CHCHD4 (MIA40) blocked HIF signalling in hypoxia and significantly inhibited tumour growth and angiogenesis in vivoCitation218. Furthermore, in human cancers we found that increased CHCHD4 expression significantly correlated with the hypoxia gene signature reduced patient survivalCitation218. Further studies exploring the relationship between CHCHD4, mitochondrial function and the hypoxic response in tumours are underway, with a view to identifying novel therapeutic strategies to improve the treatment of hypoxic tumours.
CAIX-inhibitors
The development of metastasis is responsible for 90% of deaths from solid tumoursCitation219, which has prompted the search for druggable targets with good anti-metastatic effects. In recent years, carbonic anhydrase (CA, EC 4.2.1.1) IX (CAIX) has been shown to be a potential candidate. In normal tissues, abundant CAIX expression is restricted to the glandular mucosa of the stomach where it is regulating the extracellular pHCitation220. In most solid tumours hypoxia (i.e. partial or complete deprivation of oxygen in tissue) is by far the most important stimulator of CAIX expressionCitation221. Clinical biopsy material and clinic-pathological data across a large selection of cancer types including those of cervical, kidney, breast and head & neck cancer origin mostly support CAIX as a poor prognostic marker in patients with metastatic cancer. The role of CAIX in pH regulation, results in acidification of the tumour microenvironment which reduces cell adhesion, increases motility and migration, induces neo-vascularisation, activates proteases and enhances other hypoxia-induced processesCitation221. While a role of CAIX in local control has been well established, it is less obvious from reports in the literature whether CAIX and acidosis also promotes metastatic dissemination.
Dual-action compounds including hypoxic radio-sensitisation
High levels of CAIX expression have been associated with poor prognosis, tumour progression and aggressivenessCitation222. Since CAIX is implicated in both extra – and intracellular pH (pHi) regulation, targeting CAIX through inhibition of its enzymatic activity using specific pharmacological inhibitors is a logical interesting approachCitation223. Previously, it has been shown that these inhibitors require not only CAIX expression but also CAIX activation, the latter dependent on the tumour oxygenation statusCitation34,Citation224–226. Several single-action compounds have shown inhibition of primary tumour growth and/or metastasis formation as single treatmentCitation34,Citation35,Citation227–229 or in combination with conventional therapiesCitation34,Citation221.
Since CAIX activation is enhanced in low oxygen conditions, specific targeting towards and sensitising of these hypoxic tumour regions is an important prerequisite for new compounds. Recently, dual-action compounds with high affinity for CAIX based on the combination of a nitroimidazole and a CAIX targeting moiety have been designedCitation230,Citation231. Nitroimidazoles have been shown to improve the radiation response in terms of loco-regional tumour control and disease-free survival both when administered in a single or fractionated radiation schedule, with the 5-nitroimidazole being less toxic compared with its 2-nitro analogueCitation232. From a series of nitroimidazole-based sulphamides, a novel nanomolar dual-action compound (N-[2-(2-methyl-5-nitro-imidazol-1-yl)ethyl]sulphamide) was identified which showed the most pronounced in vitro reduction in hypoxia-induced extracellular acidosisCitation230. Similar to single-action compounds, the dual-action compound was able to reduce tumour growth in a CAIX-dependent mannerCitation34,Citation230,Citation231. Due to the reduced extracellular acidification upon compound incubation, weak-basic chemotherapeutics have an increased potential to enter the cell, as exemplified by the sensitisation of tumours to doxorubicinCitation230. Interestingly, the dual-action compound was able to enhance the therapeutic effect of irradiation with higher sensitisation enhancement ratios compared to the well-established hypoxic radio-sensitisers misonidazole and nimorazoleCitation231. High bioavailability for oral formulations of the dual-action drug has been demonstrated, making potential clinical usability more patients convenient.
pH-regulation
Intracellular pH (pHi) regulation is a fundamental process in living organisms and particularly in rapidly growing tumours which produce excessive amounts of H+ via the lactic and carbonic acids metabolic endpoints. The hostile acidic and hypoxic tumour microenvironment is aggravated by a poor and chaotic vascularisation. These two inter-wined physiological processes, pHi regulation and energy metabolism have high potential for the development of targeted cancer therapies. The reader will find a detailed approach of the complex network of pHi regulation in recent comprehensive reviewsCitation223,Citation233,Citation234. In the context of this METOXIA project, we and others have designed experiments to validate, through genetic (shRNA, ZFN, CRISPR/Cas9-knockouts) and pharmacological approaches, the therapeutic potential of targets controlling pHi.
Targets of interest: carbonic anhydrases CAIX, CAXII and monocarboxylate transporter complexes MCT1, MCT4, Basigin/CD147
Chiche et al. first validated the hypothesis that CAIX, when highly expressed, ensures a much more alkaline pHi in cellsCitation235. This finding was independently confirmed in spheroids by the group of Adrian HarrisCitation236. We then discovered that in lung and colon carcinoma cell lines (A549, LS174), depletion of CAIX by shRNA leads to parallel up-regulation of the membrane-bound CAXII isoform which also plays a key role in pHi regulationCitation235. The mechanism behind the concomitant up-regulation of these two isoforms that in some cell types also concerns CAII is not yet resolved. Intracellular CO2-sensing via soluble form of adenylyl cyclase is among the current hypothesesCitation237. Consequently, the dual knockdown of CAIX and CAXII in some cells like the colon adenocarcinoma cell line LS174 led to the best reduction in tumour volumeCitation235. As reported in the previous section, we showed that combined knockdown of CAIX and XII radio-sensitised tumour cells by increasing intracellular acidosisCitation238.
Lactic acid export, particularly in highly glycolytic and growing tumours, is another key determinant in the control of pHi and tumour growth. Lactic acid is exported at a very low rate by simple diffusion of the uncharged acid form across the membrane and at high speed by H+/Lactate− symporters called MonoCarboxylate Transporters (MCTs). Tumour cells are equipped with the ubiquitous MCT1 isoform and often with the hypoxia-inducible form MCT4. We and othersCitation239,Citation240 have shown that tumour cells that express only the MCT1 isoform (Ras-transformed fibroblasts, Myc-induced malignancies) could be severely inhibited by the specific MCT1/2 inhibitor AZD3965Citation241, which is now being utilised in clinical trials for small cell lung cancerCitation242. In contrast, tumour cell lines (LS174, U87) expressing the MCT4 isoform that is well fitted for efficient lactic acid exportCitation243,Citation244 are fully resistant to AZD3965 or to expression of either shRNA-MCT1 or shRNA-MCT4Citation240. Furthermore, we showed that ZFN-mediated MCT4 knockout sensitised LS174 cells to MCT1 inhibitor AZD3965. These findings demonstrated that simultaneous blockade of MCT1 and MCT4 that reduced pHi by at least 1 unit (Marchiq I, Pouysségur J, in preparation) is mandatory to severely reduce growth of many human tumoursCitation240. This combined MCT1/4 targeting approach could as well be obtained by simple knockout of Basigin/CD147, an accessory protein required for appropriate folding and trafficking of MCT1/4 from ER to the plasma membraneCitation240,Citation245.
MCTs inhibition: two anti-cancer strategies
AstraZeneca has now developed an MCT4 specific inhibitor. As was expected, when we combined these two MCTs inhibitors, we strongly reduced LS174 tumour growth phenocopying the double knockout MCT1/4 (Marchiq I, Pouysségur J, in preparation). Surprisingly these double LS174 MCT1/4-KO cells grow very slowly and do not die because they are capable of re-activating OXPHOS to maintain viable levels of ATP. This first strategy that blocks lactic acid export, glycolysis and tumour growth is likely expected to amplify anti-tumour action by the re-activation of T-cell immune response suppressed by acidic tumour environmentCitation246.
The second anti-cancer strategy discussed recentlyCitation234, is to be used in a very narrow therapeutic window to limit cytotoxicity. It is based on the fact that disruption of both MCTs sensitised the cells to phenformin, a mitochondrial complex I inhibitor, inducing ATP crisis and rapid tumour cell death (Marchiq I, Pouysségur J, in preparation). These two strategies targeting pHi regulation and bioenergetics will have to be validated in mouse genetics and immune-competent models before future clinical developments.
Chemical inhibitor synthesis
Some of the interesting CA-inhibitors which showed selectivity for the inhibition of the tumour-associated isoforms CAIX and -XII are compounds 1–9 shown in . The tail present in a sulphonamide CA-inhibitor is essential for the binding of the inhibitor within the active site, and its influence on the inhibition profile against the many isoforms present in mammals is also significant. In fact, small structural changes in the ring on which the sulphonamide zinc-binding group (SBG) is appended, may also markedly influence the binding of the sulphonamide to the enzyme. This has been demonstrated in a recent workCitation247 in which the thienyl-carboxamido benzenesulphonamides 1 and 2 were crystallised bound to hCAII. Other interesting structure-based examples for obtaining isoform-selective CAIs of the sulphonamide type are compounds 3–9 which will be discussed here as tumour-associated, CAIX/XII-selective derivatives. Compound 3 incorporates tosylureido tailsCitation248. This class of derivatives has been reported earlierCitation248 but only recently an interesting selectivity ratio for inhibiting the transmembrane, tumour-associated isoforms (CAIX and -XII) over the cytosolic off-target one CAII has been observedCitation249. Indeed, 3 has KIs of 12 nM against hCAII, of 1.3 nM against hCAIX and 1.5 nM against hCAXII, whence, a selectivity ratio of 9.2 (hCA IX versus hCAII) and of 8.0 (hCA XII versus hCAII)Citation249. However, the most CAIX/XII-selective compounds reported until now are those incorporating triazinyl tails, of which 4 is an interesting exampleCitation249–251. Compound 4 has inhibition constants of 1098 nM against hCAI, of 37 nM against hCAII, of 0.75 nM against hCAIX and of 1.6 nM against hCAXII, whence selectivity ratios of 49.3 (CAIX versus CAII) and 23.1 (CAXII versus CAII)Citation249. Some congeners of 4 showed even higher such selectivity ratios (up to 700) for inhibiting the tumour-associated isoform hCAIX over hCAIICitation250,Citation251. But again it is interesting to compare this inhibition data with the X-ray crystallographic structure of compounds 3 and 4 in complex with hCAIICitation249. The benzenesulphonamide fragment of the two inhibitors binds in the expected manner (coordinating to the Zn(II) ion) and was superposable for the two compounds. However, the tosylureido and substituted-triazinyl tails of the two compounds adopted extended conformations orientated towards opposing parts of the active site cavityCitation249. This surely is reflected in the different inhibition/selectivity profiles of the two compounds, which have been mentioned above.
Figure 5. CA-inhibitors reported ultimately, which showed selectivity for the inhibition of the tumour-associated isoforms CAIX and –XII.
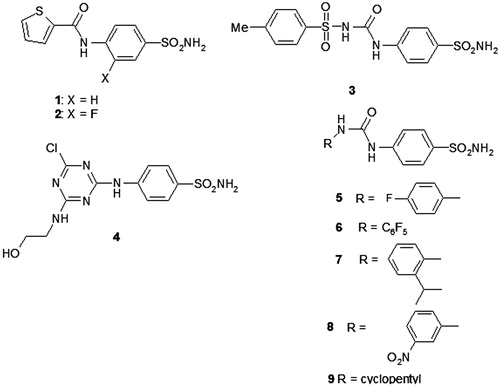
But undoubtedly, the most interesting case of CAIX-selective compounds is furnished by derivatives 5–9, which incorporate again the benzenesulphonamide head, but this time 4-aryl/alkylureido tailsCitation252,Citation253. A large series of such compounds has been reportedCitation252 and for many of them good selectivity ratios (in the range of 16–53) for inhibiting CAIX over CAII were detectedCitation252. Another interesting feature for this series of compounds was that some of its members were also excellent hCAII inhibitors (in addition to strongly inhibiting the tumour-associated isoforms hCAIX and -XII, in the low nanomolar range)Citation252,Citation253. For example, the inhibition constants of compounds 5–9 against hCAII were of: 96 nM, 50 nM, 3.3 nM, 15 nM and 226 nM, respectivelyCitation253. These parameters for the inhibition of hCAIX were of 45 nM, 5.4 nM, 0.5 nM, 0.9 nM and 7.3 nM, respectively. By reporting the X-ray crystal structures of the adducts of these five sulphonamides complexed to hCAII (), it was observed that again the benzenesulphonamide fragments of the inhibitors are quite superposable, whereas the tails adopted a variety of conformations and orientations within the enzyme active site. This variability of binding is probably made possible by the flexible ureido-linker between the benzenesulphonamide part and second moiety of the 1,3-disubstituted ureas 4–9. Indeed, some of the groups from the terminal part of these molecules were observed in the hydrophobic pocket, others in the hydrophilic one and some of them between these two binding sites. Such different orientations may explain the range of inhibitory activity against hCAII (3.3–226 nM) and obviously hCAIX, as well as the selectivity ratios for inhibiting the two isoforms. However, a very interesting feature of some of these compounds (e.g. 8) was their potent inhibition of growth of primary tumours and metastases in an animal model of breast cancer which potently over-expresses CAIXCitation252–254. In similar tumour cell lines without CAIX, no inhibition of the tumour growth has been observed, which demonstrated that the drug target of these compounds is CAIXCitation252–254.
Figure 6. hCA II complexed with the tosylureido benzenesulphonamide 3 (green) and triazinyl-substituted benzenesulphonamide 4 (blue)Citation249 (A); and with five ureido sulphonamides 5–9, compounds 5 (orange), 6 (pink), 7 (yellow), 8 (grey), and 9 (cyan) (B)Citation253.
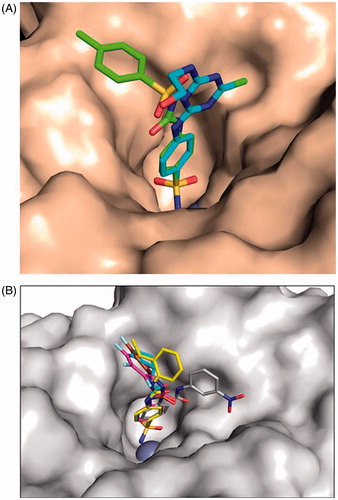
It may be observed from all data presented above that the sulphonamides dominated the drug-design landscape of CA-inhibitors for many years, However, very recently, new important chemotypes emerged. Among them, the dithiocarbamates (DTCs) are undoubtedly the most interesting onesCitation255–258. These compounds have been rationally discovered as CAIs after our report of trithiocarbonate () as an interesting (milli – micromolar) CA-inhibitor. In the X-ray crystal structure of this inorganic anion bound to CAII, it has been observed a monodentate coordination of the inhibitor via one sulphur atom to the zinc ion from the enzyme active site, and a hydrogen bond in which another sulphur and the OH of Thr199 were involved. Thus, the
was detected as a new ZBG. As DTCs incorporate this new ZBG, a rather large series of such compounds was prepared and evaluated for their inhibitory activity against several mammalian, fungal and bacterial CAsCitation255–258. Several low nanomolar and sub-nanomolar CA-inhibitors were thus detected against all these isoforms. The X-ray crystal structures were also reported for three DTCs complexed to hCAII. DTCs 10–12 inhibited hCAII with KIs of 25 nM, 41 nM and 0.95 nM, respectively, and hCAIX with KIs of 53 nM, 757 nM and 6.2 nM, respectivelyCitation256. As seen from , the binding mode of the ZBG present in 11 is identical to that of trithiocarbonate (with one sulphur coordinated to the metal ion), but the organic scaffold present in the DTC was observed to make extensive contacts with many amino acid residues from the active site, which explains the wide range of inhibitory power of these derivatives (from the sub-nanomolar to the micromolar, for the entire series of around 30 DTC reported so farCitation255,Citation256.
Figure 7. (A) Structure of DTCs 10–12. (B) Electronic density for the adduct of dithiocarbamate 11 bound within the active site of hCA II [i]. The zinc ion is shown as the central sphere and the amino acid residues involved in the binding are evidenced and numbered (hCA I numbering system)Citation255.
![Figure 7. (A) Structure of DTCs 10–12. (B) Electronic density for the adduct of dithiocarbamate 11 bound within the active site of hCA II [i]. The zinc ion is shown as the central sphere and the amino acid residues involved in the binding are evidenced and numbered (hCA I numbering system)Citation255.](/cms/asset/18892b1f-6a83-492a-ac7d-9d2f3ae76036/ienz_a_966704_f0007_b.jpg)
Preclinical models
Models for metastasis
Studying the anti-metastatic potential of anti-cancer compounds in appropriate models for metastasis as part of the preclinical drug development package is pivotal. In vitro cell spreading, migration and invasion assays are covering some of the aspects of the metastatic cascade and can be used to narrow down the search for the most potent lead compound(s). However, in vitro assays only recapitulate one or several aspects of the metastatic cascade and are by no means powerful enough to substitute for further in vivo testing of the lead compound(s). There are many different migration assays including the transwell (Boyden Chamber), scratch wound-healing, cell-exclusion zone, fence, micro-carrier-based and spheroid migration assays, which have been reviewed in great detail by Kramer et al.Citation259. In vivo models for testing anti-cancer drugs can be grouped into intravenous, ectopic and orthotopic models. On some occasions genetically engineered (GM) mouse cancer cells have been generated in which over-expression or deletion of a particular gene enhances the metastatic potential. However, these GM cancer models often have a low incidence of distant metastatic disease and are unreliable for predicting clinical outcome of anti-metastatic therapyCitation260. In intravenous models, a tail vein or intra-cardiac injection induces a systemic distribution of cancer cells. A commonly used variant of the intravenous model is the injection of colorectal carcinoma cells, e.g. HCT116 and HT-29 into the spleen. Splenic vessels which drain the spleen directly transport the cancer cells to the liver where well developed metastases are visible on magnetic resonance (MR) scans after 28 dCitation261. These “experimental metastasis” model recapitulates some of the late steps of the metastasis cascade but specifically not the earlier ones including the selection pressure in the primary tumour, local invasion of the adjacent host tissue and intravasation of blood vessels. In particular the tail vein model gives a 100% incidence of lung metastases in a temperate reproducible manner and is therefore widely used for testing anti-cancer compounds. In contrast, there are the “spontaneous metastasis” models in which the tumour cells are either growing in a donor organ ( = ectopical, usually sub-cutaneous) or in the organ of origin ( = orthotopic) allowing to study the effects of micro-environmental factors on metastatic disease. The orthotopic model provides a more clinically relevant setting than the conventional sub-cutaneous model. As an example, MDA-MB-231 injected into the mammary fat pad induces spontaneous metastases in CBA nude miceCitation229,Citation262.
The use of carbonic anhydrase inhibitors (CA-inhibitors) in enhancing local control and overall survival has been investigated for more than a decade. However until recently only broad-spectrum CAIs like acetazolamide (AZM or AZA) were available. In vitro research showed that AZM was very effective in reducing the invasiveness of renal carcinoma cellsCitation263. In addition, we showed in cell lines that highly expressed CAIX under anoxic conditions (e.g. the colorectal model HT-29 and breast cancer cells GM to over express CAIX [MDA435 CA9/18]) that AZM treatment increased the intra-tumoural pH. pH changes were accompanied by an enhanced uptake of weak-base doxorubicin which caused an increase in the doxorubicin-induced cytotoxicityCitation264. However, AZM is not very specific for the tumour-associated CAIX/XII isoforms, inhibiting also the more generally expressed off-target CAI/II isoforms. This prompted the development of more selective CAIX/XII inhibitors. In the last few years, selective CAIX inhibitors based on the sulphonamide, coumarin and sulphamate classes have shown to have promising anti-metastatic potential. Preclinical studies with orthotopic 4T1 (breast carcinoma) tumours showed that treated with ureidosulphonamide 25 and 104 and coumarin glycosyl coumarins 204 and 205 targeted 4T1 metastasis and improved metastasis-free survivalCitation35. Belonging to the sulphamate class of CA-IX inhibitors, 4-(3′-(3″,5″-dimethylphenyl)ureido)phenyl sulphamate (also named S4) reduced the spreading and migration of human MDA-MB-231 (breast carcinoma) cells and significantly reduced the metastatic burden in lung of mice with well-established orthotopic MDA-MB-231 tumoursCitation229. The significant anti-proliferative effect of similar sulphamate -based CAIX inhibitors on a range of human breast cancer cells offers the potential for additional anti-cancer leadsCitation265. Besides CAIX other hypoxia-related targets like hypoxia-inducible factor 1 alpha (Hif-1α), glucose transporter 1 (GLUT-1) and vascular endothelial growth factor (VEGF) are good approaches for anti-metastatic effectsCitation266,Citation267.
Metastasis is a complex process consisting of multiple steps of malignant cell–host cell interactions, which are tightly regulated by various signalling pathways. The metastatic cascade includes interactions between invasive tumour cells and various host cells in the tumour microenvironment, intravasation of tumour cells into the circulation, transport of tumour cells to distal tissues and organs, extravasation of tumour cells from blood or lymphatic vessels, formation of initial metastatic niches, and re-growth of metastatic tumours. Despite the advances of image analysis to detect metastatic lesions at stages, clinically detectable metastases by imaging analysis often represent the end-stage of the metastatic cascade. Visualisation of the early events of cancer metastasis is almost impossible in mammals while zebrafish provide an alternative tool to study the early events of metastasis. Given the advantages of transparent features, immunoprivilege, and genetically manipulatable zebrafish embryos, a zebrafish metastasis model has been establishedCitation268. In this model, human or mouse tumour cells can be color-coded with a fluorescent dye and their movement in the fish body can be detected at the single-cell level. Moreover, exposure of zebrafish in a hypoxic environment enhances cancer cell dissemination in this preclinical modelCitation269. Hypoxia-triggered VEGF-dependent angiogenesis is crucial for cancer cell dissemination. This model offers a unique opportunity to study the early events such as intravasation of tumour cells into the circulation and formation of metastatic niches.
Another important route of cancer spread is lymphatic metastasis by which tumour cells metastasize to regional lymph nodes. In many epithelial cell-originated tumours such as breast cancer, colorectal cancer, prostate cancer, ovarian cancer and lung cancer, lymphatic metastasis occurs more frequently than bloodstream metastasis. Despite this knowledge, appropriate animal models have been lacking to study the events and mechanisms that underpin lymphatic metastasis. In our laboratory, we have developed several tumour models in which lymphangiogenic factors are highly expressedCitation270,Citation271. Implantation of these tumour cells in mice results in lymphatic metastasis in regional lymph nodes. Consistent with lymphatic metastasis, tumour tissues contain high numbers of lymphatic vessels. It is likely that both intra-tumoural and peritumoural lymphangiogenesis contribute to lymphatic metastasis. To quantitatively assess the tumour-derived lymphangiogenic factors in promoting lymphangiogenesis, we further developed a mouse corneal lymphangiogenesis modelCitation272. This is a unique model to quantitatively study lymphangiogenesis induced by different factors either alone or in combinations, simply because cornea is an avascular tissue.
Preclinical cancer models for the assessment of novel therapeutics targeting hypoxia
The increase in knowledge of cancer pathogenesis has led to a growth in the development of anti-cancer drugs, but the successful pre-clinical assessment of novel cancer therapeutics is dependent on the use of relevant experimental models. Assessing any new drug requires methods that combine prognostic accuracy and high throughput/cost. Therefore, the models used differ at each stage of the process. It is estimated that one in 5000–10 000 potential anti-cancer agents is approved by the FDA and only 5% of those that enter Phase I clinical trialsCitation273. Even then many fail in Phase II and Phase III trialsCitation274. Therefore, there is an urgent need for superior, more clinically relevant human cancer models for drug assessment.
2D monolayer cell culture
The most practical starting point in the investigation of novel oncology therapeutics is the use of panels of cell lines that encompass the cancer of interest grown in 2D cell culture. This initial strategy allows the evaluation of drug on functional parameters such as cell proliferation, cell death and effects on invasive potential to be measured, using standard protocols and IC50 values to be obtained. This is exemplified by studies identifying CAIX inhibitors active against breast and ovarian cancer cell linesCitation265,Citation275. Possible additive and synergistic interactions with standard treatments can also be studied. Because cancer is an extremely heterogeneous condition, the size of the cell panel used in preliminary work can be crucialCitation276 and should include cell lines that reflect various sub-types of the cancer. The major drawback of many cancer cell lines is that a highly passaged line may no longer fully represent the initial primary cancer they were derived from, since selection pressures tend to allow less differentiated cells to survive, leading to loss of some biological differencesCitation277. These problems can be overcome by the development of cell lines de novo, from cancer tissuesCitation278.
2D cell culture of cell lines is an extremely simple model system, and bears little resemblance to any in vivo physiological environment, and it cannot recapitulate the heterogeneity between or within human tumours. Single-cell sequencing shows that tumours contain individual clones with many different mutationsCitation279. Culture media maintain neutral pH, and therefore do not reflect the increased acidity of the tumour microenvironment which can drop to pH 6.0Citation280. Further, most cells are cultured at oxygen levels of approximately 21%, when oxygen tension in normal tissue is 7% on average, and much lower in tumours, with the mean value of 1.5%Citation281. Moreover, cells in 2D culture show considerable variation in morphology, metabolism, gene expression and signalling in comparison with cells in tumour tissueCitation282–285.
In solid tumours, cells adapt to survive in conditions where pH and O2 gradients are the norm, glucose and other nutrients are limited, and waste metabolites concentrated. Therefore, cellular responses to drugs can differ markedly when cells are cultured using in vitro 3D methods rather than 2D cultureCitation286,Citation287. However, the advantages of using 2D culture at early stages outweigh any disadvantages in that these models offer a fast, reliable and reproducible test bed and any results can be quickly investigated for specificity and potential mechanisms of action.
3D culture
While differences between 2- and 3D models are important in any anti-cancer drug study, they are crucial when the main strategy under investigation is the exploitation of survival strategies in hostile micro-environmental conditions, such as interference with pH homeostasis by CAIX-inhibition. Much current research considers the interaction of tumour cells with the microenvironment and how tumour-driven changes in the microenvironment then influence the activities of tumour cells. Therefore, relevant 3D tumour culture techniques are the next logical research step. These methods can be divided into in vitro or in vivo systems.
The development of 3D in vitro models, using spheroids grown from cell lines, or in matrix scaffolds, allows a better understanding of drug responses and intracellular signalling in a more relevant milieu that mirrors some aspects of tumour physiology and show better correlation with results from in vivo drug studiesCitation282,Citation287–291. The methodology for 3D growth models is well documented using suspension approaches or ECM component systems, which allow a more physiological model of invasionCitation292,Citation293. The matrix can be designed to include components that mirror the stroma of the cancer under study, for example, breast cancer extracellular matrix has a high proportion of collagen I which has been shown to promote invasion and metastasisCitation294,Citation295. As spheroids become larger, areas of hypoxia and acidosis develop reproducing oxygen and pH gradients similar to those found in micro-metastases and avascular tumours, and causing the development of a necrotic core and perinecrotic hypoxic areaCitation296,Citation297. The role for CAIX in regulating extracellular and pHi in several cancer types has been demonstrated by use of 3D spheroidsCitation298,Citation299.
Xenograft and genetically engineered mouse models
Xenograft models allow tumour growth, drug efficacy, toxicity and a range of pharmacokinetic measurements to be made in an in vivo situation. Xenografts are however formed from the same cell lines used in 2D studies, and grown in immune-deficient mice to allow rapid tumour growth. While these growths can recapitulate the oxygen and pH gradients of tumours, the stromal elements are murine and therefore may not accurately reproduce the stromal–tumour interactions found in human tumours and xenografts from a cell line will not encompass the complete genetic and epigenetic heterogeneity of an actual tumourCitation300. However xenografts allow the investigation of therapy using known histological cancer sub-typesCitation301, but metastatic models are limited and duplication of the different tumour sub-types found in patients can be difficult, as this is dependent on the cell lines availableCitation302,Citation303. However, reduction of breast cancer metastases to the lung by CAIX inhibition has been demonstrated using both the human MDA-MB-231 xenograft modelCitation229 and the murine 4T1 mammary modelCitation35.
Combination strategies can also be assessed pre-clinically such as the interaction between CAIX inhibition and either radiotherapyCitation231,Citation238 or angiogenesis inhibitorsCitation299. The translation of xenograft studies into the clinic has had variable success, although better selection and characterisation of the model has led to better success in more selective targeted therapiesCitation304,Citation305. Mechanistic insights can also be derived using xenografts, for example, to study the consequences of silencing CAIX or CAXII and demonstrating modified tumour growthCitation235.
Murine tumours can be induced in immune-competent mice; some occur spontaneously, or can be induced using carcinogens. The other strategy is to use GM mouse models (GEMMs) in which specific mutations are introduced which can be tissue specific, or temporally controlledCitation306. GEMMs, allow the growth of primary cancers in immune competent mice within a murine tumour microenvironmentCitation307; this may more accurately replicate the stroma component and microenvironment of human cancer than xenografts, but they are not of human originCitation308–310. These models have been demonstrated to replicate closely the results of clinical trials for human cancersCitation311,Citation312. This system still has limitations and no animal model is feasible for HTSCitation287,Citation313. However, models such as the TRACK renal cancer mouse model which express a constitutively active HIF1α in kidney proximal tubule cells should provide enhanced insight into the oncogenic functions of HIF1α in renal cancerCitation314.
Ex vivo tumour tissue explants/grafts
One way of modelling cancer cells in situ is the use of ex vivo tumour material, known as tumour graft models or patient-derived xenograftsCitation315. These xenografts are formed by digesting the patient's tumour tissue and then transferring cells from it into an immune-deficient mouse. Tumours are then directly passaged from mouse to mouse.
The advantages of this system have been shown using tissue grafts from breast cancer patients, which have been shown to reproduce tumour growth, metastatic capacities and pathology of the main sub-types of human breast cancer in an accurate mannerCitation316. Studies indicate that they also preserve histological indicators such as receptor positivity and proliferation markers, suggesting that this methodology can sustain the original pathophysiology of the tumourCitation303. This could be a relevant model for anti-cancer therapeutics that exploits micro-environmental changes. The major disadvantage of the system is that more than one animal is needed to capture the variability of each tumour and therefore multiple transfers are needed even for one tumourCitation317; and while the tumour cells preserve many of the feature of the original tumours, there are alterations and losses of the human stromal elements of the tumourCitation318–320. Other disadvantages are the substantial cost, since these grafts must be sustained in a murine host, and therefore passaging techniques require more skill, and also there can be a long growth period for a tumour graft to formCitation321.
Cultured ex vivo tumour explants
Primary tumour material can also be cultured ex vivo in a similar manner to 3D tumour spheroidsCitation322,Citation323. This use of primary tumour tissue allows analysis of heterogeneous material and tumour sub-types. With this approach, there is no digestion of tissue so that these explants maintain the stromal structures associated with the original tumourCitation324. This is a particularly useful system if tissue can be obtained pre-treatment from biopsy material.
Our own studies indicated that changes to breast cancer explants can be seen and monitored within 5 d in most instances. This system could define cases that will respond to a specific therapy and be used as part of the route towards personalised medicine. It may also provide insights into tumour response comparable to neoadjuvant trialsCitation324; since investigation of co-treatment strategies or scheduling of treatments may be examined. We have found that these explants can be maintained in culture for at least a monthCitation323.
More research is currently needed into metastatic disease. In vivo metastatic research utilises injection of tumour cells and monitoring distal sites for metastatic formationCitation325. These are time consuming assays that are expensive and do not allow observation of the early phases of metastasisCitation325,Citation326. Ex vivo explant methodology also allows monitoring of invasive changes in an appropriate tumour microenvironment which could be assessed in high throughput formats. Changes in matrix composition and the effect of stromal cells can also be assessed. The advantages of this system are that explant growth/response can be continuously monitored and tissue can be harvested and investigated when changes are actually taking place. Tissue can be lysed, or fixed for later analysis. The disadvantages are that pharmacokinetic changes cannot be monitored and analysis is time consuming. However, the use of patient-derived human tumour tissue for appropriate preclinical research is increasingCitation327.
Summary – Section “Preclinical cancer models for the assessment of novel therapeutics targeting hypoxia”
Although there have been many recent advances in cancer treatments, the number of novel therapeutics that fail during randomised phase III clinical trials of novel oncology therapeutic, even after successful completion of Phase I and II is extremely highCitation300,Citation321,Citation328. This suggests that pre-clinical models of this disease either do not accurately reproduce authentic tumour physiology or fail to reflect the actual heterogeneity of tumours. One reason for this is a failure to explore the effects of new drugs in the conditions found in the tumour microenvironment, which strongly influences the growth and survival of tumour cells. The advantages and disadvantages of each model system suggest that novel therapies must be tested using several appropriate methods.
In the aspect of hosts, genetically identical mice are commonly used in both treated and non-treated groups. In humans, however, the genetic information in each individual patient is different from others. Additionally, the same age and gender mice are used for experimentation and human patients often are different in these parameters as well. For tumours, development of a clinically detectable tumour in human patients often takes years while growing a mouse tumour of proportionally the same size often needs a few weeks. The different growth rates could affect the cellular and molecular composition in the tumour microenvironment and eventually lead to different responses to the same drug. Also, in experimental tumour models established tumour cell lines are often used and they may lose their identity of the tissues and organs from which they originated. Another concern for the mouse tumour model is that tumours are often implanted sub-cutaneously and this location is not directly relevant to human patients. As for treatment regimen, treatment with drugs is often initiated shortly after tumour implantation in mice and the same drugs are often given to human cancer patients with advanced and metastatic cancer disease. Finally, assessment of therapeutic efficacy in human cancer patients and mouse tumour models are different. In human patients, survival especially overall survival improvement is the endpoint for drug approval. In mice, tumour size has been used as a surrogate marker for therapeutic efficacy. Tumour sizes do not always correlate with survival advantages. Within the context of radiotherapy it is often more relevant to use TCD50 experiments, although these are very expensive. Although various genetic mouse models have been established to reproduce the clinical situation, these genetically manipulated tumour models are often driven by a particular oncogene or mutations of tumour suppressors and are thus less relevant to the clinical situation.
A novel strategy has been proposed called “co-clinical trials”Citation329, which synchronises preclinical and clinical trials by correlating human patients and mouse models in parallel. A model using ex-vivo patient derived explant models either in a murine host or in ex-vivo culture could realistically offer a more pre-clinically appropriate testing bed for clinical trials or in concert with co-clinical trialsCitation276. Furthermore for drugs targeting tumour vasculatures, it is important that orthotopic cancer models should be considered since blood vessels in various tissues are intrinsically different from each other. An alternative is also spontaneous models arising from transgenic animals.
Clinical testing
Diagnostics: hypoxia detection and imaging
Radiotherapy combined with chemotherapy is currently the preferred treatment modality for patients with solid tumours. Treatment effectiveness however is dependent on tumour micro-environmental characteristics, such as hypoxia. Hypoxia results in poor prognosis since it promotes resistance to radiotherapy and chemotherapy and it increases tumour aggressiveness, angiogenesis and metastatic potential. Detection and quantification of hypoxia could therefore help selecting patients who might benefit from treatment adaptation counteracting hypoxia.
Since tumour hypoxia is distributed very heterogeneously, biopsy-based assessment of hypoxia may be prone to sampling errors. Furthermore, for some treatments, like selective dose escalation to hypoxic tumour sub-volumes, gene expression is clearly not appropriate to guide treatment. Therefore, PET-based assessment of tumour oxygenation in hypoxic tissue which allows assessment of the whole tumour and 3D mapping of the distribution of hypoxia, is attractive and has received immense interest.
In the context of radiotherapy treatment planning, PET imaging of the selective binding and retention of 2-nitroimidazoles prior to and during treatment is well-suited for displaying the spatial distribution of hypoxiaCitation330. As an increased radiation dose to radio-resistant hypoxic areas may increase local controlCitation331, accurate identification and stable detection of intra-tumoural hypoxic sub-regions is utmost importantCitation332.
Some inherent weaknesses in PET in general (low resolution of several mm) and hypoxia PET in particular (slow tracer retention and slow clearance of unbound hypoxia-unrelated tracer) leads to limited inter-tissue and intra-tumoural contrast even hours after tracer administration, which may compromise the quantitative accuracy of hypoxia PET. Specifically problematic is the risk of overlooking areas where viable hypoxic cells are intermixed with necrosis with little tracer at a spatial scale that is too small to resolve on PET. Interestingly, although PET hypoxia imaging is able to identify patients with poor prognosis, the 15-gene hypoxia gene signature described below ranks patients differently than PET imaging, suggesting that they do not provide identical informationCitation333. In the METOXIA project, significant efforts to develop hypoxia tracers with improved pharmacokinetics has been undertaken, resulting in the development and testing of two new tracers. Pimonidazole, a 2-nitroimidazole, has excellent pharmacokinetic characteristics and is often used as the “gold standard” to mark hypoxia in tissue following tissue removal, sectioning and immunohistological labelling. However, since radioactive labelling of pimonidazole has not been attempted previously, a method for labelling of pimonidazole was developed under the framework of the METOXIA projectCitation334. Initial in vitro testing in cell lines revealed that [18F] labelled pimonidazole ([18F]FPIMO) was strongly hypoxia-driven with slight superiority to the established hypoxia tracer [18F]FAZA. [18F]FPIMO also accumulated in experimental tumours compared to non-hypoxic reference tissue and its intra-tumoural distribution (autoradiography) was similar to the distribution of unlabelled pimonidazole (immunohistochemistry). Nonetheless, [18F]FPIMO proved inferior to [18F]FAZA in vivo, since absolute tumour signal and intra-tumoural contrast was low, thus compromising the quantitative accuracy of PET imaging. Low tumour signal was coupled to extensive tracer accumulation in liver and kidneys, and very rapid degradation of [18F]FPIMO in the blood. On-going work focuses on the possibility of labelling pimonidazole in different positions with [18F] to improve tracer stability in vivo.
A recently developed 2-nitroimidazole nucleoside analogue [Citation18F]-HX4 with preferred pharmacokinetic properties, having high water solubility and fast clearance from non-hypoxic tissues, has shown to be a promising and non-toxic marker to visualise tumour hypoxiaCitation335–337. Preclinical studies in a rat rhabdomyosarcoma model demonstrated increasing [18F]-HX4 contrast over time, reaching a plateau 4 h after injectionCitation335. A recent clinical trial in NSCLC patients confirmed this optimal imaging time point. Tumour hypoxia, defined as a tumour-to-blood ratio (TBR) > 1.4, was observed in 80% of the primary tumours and 60% of the involved lymph node regions increased significantly from 2 h to 4 h after injection both for the primary tumour and the lymph node regionsCitation338. Additionally, it was found that the minimal acquisition time without affecting TBR and hypoxic fraction equals 10 min, reducing influence on image acquisition due to patient movementCitation338.
Recently, it has been pointed out that a systemic examination of several 2-nitroimidazole based PET hypoxia markers within the same animal model or patient group is needed to assess whether one tracer is superior to anotherCitation339. Several of these studies are currently on-going and focus on optimal imaging time point, TBR, spatial reproducibility and oxygen sensitivity as endpoints. Especially for future patient dose painting studies it is important to acquire insights in the spatio-temporal stability of the PET marker, which would imply image acquisition in treatment position in order to reduce possible registration errors.
Assessment of tumour hypoxia using hypoxic metagenes
Generally the use of gene expression profiling is a powerful diagnostic approach to identify and quantify tumour hypoxia and provides a number of distinct advantages over the other techniques described. Gene expression profiling is done by extracting RNA from clinical samples and simultaneously determining gene expression of multiple transcripts. Traditionally, this was achieved by using a gene expression microarray platform. Although the very large datasets generated using this approach have been necessary for deriving and validating hypoxia signatures, microarrays provide too much data and are not a GCP validated format for routine diagnostic use. Therefore, recent efforts to apply and validate hypoxia-associated gene signatures (or metagenes) have utilised low density quantitative real-time polymerase chain reaction (qPCR)-based assays to interrogate the expression level of fewer genes.
The main strength of this approach is the ability to measure multiple hypoxia responsive markers in parallel, providing a more robust measure of hypoxia than determining the expression of a single gene that is hypoxia-inducible, e.g. CA9. This property means that metagene analysis produces hypoxia measurements with lower levels of intra-tumour heterogeneityCitation340.
Using Affymetrix U133plus2 GeneChips expression data from 59 head and neck squamous cell cancers (HNSCC) our Oxford partner group initially developed a 99-gene hypoxia signatureCitation341. The signature was derived using a seed clustering approach, where a hypoxia gene sub-network is formed by selecting gene transcripts whose expression is highly correlated with the expression of 10 well-known hypoxia-regulated genes (the initial “seeds” of the network). High hypoxia score (HS), i.e. high summary expression of the hypoxia signature, was able to predict worse outcome in an independent cohort of 60 HNSCC cases. Further validation using a published series of 295 breast cancer samples also confirmed the ability of the hypoxia metagene to significantly predict disease-specific survival.
A more compact 51-gene hypoxia signature was developed extending the above approach to a meta-analysis context where genes were selected in a common hypoxia signature if they were highly correlated with the expression of the initial seeds and this correlation was consistent across cancer datasets and typesCitation342. More than 1000 cancer samples were used for this analysis and the resultant metagene was able to better predict outcome in several large independent data sets than the initial 99-gene signature and other published signatures. Specificity of the signature for hypoxia has also been demonstrated using cell lines exposed to hypoxia.
Recently, we have applied this signature to the Metabric cohort of 2000 breast cancers ()Citation343. This work demonstrated that basal-like and HER2 + breast cancers have increased hypoxia scores compared with luminal A, luminal B, normal-like breast cancer sub-types and normal breast tissue. Consistently, the basal-like sub-type of breast cancers has been reported to have high HIF-1α activityCitation344. In this dataset the hypoxia signature was highly prognostic.
Figure 8. Validation of the common hypoxia signatureCitation342 in different breast cancer subtypes and prognostic utility. (A) Box-whisker plots showing median, upper and lower quartiles and 95% range of hypoxia signature. Breast cancer subtypes were classified by hormonal receptor status (ER−/PgR−/HER2−, HER2−, HER2+, ER−, ER+) or PAM50 (Basal, Her2, LuminalB, LuminalA, Normal-like). (B) Kaplan–Meier analysis of the Metabric cohort demonstrating poorer overall survival for breast cancer patients with relatively higher hypoxia signature score. Patients were divided into quartiles from low (Q1) to high (Q4) hypoxia groups based on cumulative hypoxia score.
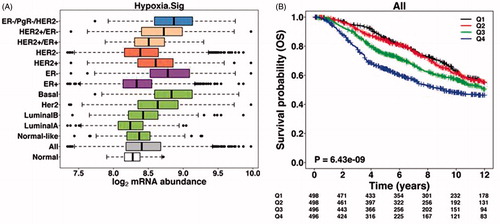
The prognostic information provided by hypoxia signatures could allow clinicians and patients to make better informed decisions when planning treatment strategies and this information may be particularly useful in cases where routine histological analysis is unable to provide prognostic valueCitation345. However, a more powerful application for hypoxia signatures is their use to predict which patients will respond to hypoxia-modifying or hypoxia-targeted treatments.
A 26-gene hypoxia signature was able to retrospectively identify laryngeal cancer patients that benefited from hypoxia modifying carbogen and nicotinamide (CON) treatment in combination with radiotherapyCitation346. Patients with low hypoxia scores did not benefit from addition of CON to the standard accelerated radiotherapy treatment. When the same analysis was conducted using samples from a bladder cancer trial there was no benefit of addition of CON treatment in patients with hypoxic tumours. There are several potential reasons for this lack of benefit. One possibility is that while the hypoxia signature used may accurately detect hypoxic cells in laryngeal cancer, it may not be accurately detecting “hypoxic” cells in bladder cancer, i.e. bladder cells may respond to hypoxia by inducing a different set of genes. Another possibility is that the actual radio-resistant fraction of cells in the tumour is not proportional to the hypoxia score.
The amount of hypoxia in tumours is expected to decrease in response to anti-proliferative agents as oxygen demand is reduced. Indeed, a reduction in the hypoxia metagene score was observed in breast cancers when patients were treated with an aromatase inhibitor and this change correlated significantly with a reduction in a marker of cellular proliferation (Ki67)Citation347. This increase in tumour oxygenation may improve the efficacy of subsequently administered treatments, e.g. radiotherapy or may reduce the efficacy of agents that require hypoxia for their activation, e.g. hypoxia-activated prodrugs.
An alternative marker for assessment of hypoxia is microRNA-210 (mir210). Expression of mir210 is strongly induced in cells exposed to hypoxia in a HIF-dependent mannerCitation348,Citation349. mir210 has been demonstrated to target several transcripts including the mitochondrial iron sulphur scaffold protein ISCUCitation350,Citation351. The down-regulation of ISCU by mir210 during hypoxia was shown to repress mitochondrial respiration, providing an adaptive response to the hypoxic stress.
Levels of mir210 expression in 219 breast cancer cases correlated strongly with the 99-gene hypoxia metagene suggesting it is a useful alternative for measuring tumour hypoxiaCitation348. Consequently, high levels of mir210 correlated with poor prognosis in breast cancerCitation352. This finding was confirmed in an independent study of breast cancer cases, with mir210 providing prognostic performance equivalent to that of many multiple genes signaturesCitation353. In contrast, high levels of mir-210 expression correlated with better prognosis in renal cell carcinoma reflecting differences in the tumour biology of breast and renal cancersCitation349.
In cervical cancer, a combined analysis of DCE MRI and gene expression profiles was performed to generate a 31 hypoxia gene signature with prognostic impact in a cohort of patients referred to chemo-radiotherapyCitation354. Its specificity for hypoxia was validated in analysis with cervical cancer-specific hypoxia gene sets derived from cell lines. The signature predicted outcome in an independent cohort and showed significance in multi-variate analysis with conventional clinical markers. Its relationship to a prognostic DCE MRI parameter suggests a potential of combining MRI with the hypoxia gene expression signature in treatment planning of this disease.
In prostate cancer, the possibility of generating a gene expression signature of HIF1 targets for monitoring changes in hypoxia during androgen-deprivation therapy (ADT) was investigated. ADT improves outcome of intermediate and high-risk patients when combined with radiotherapy in a neoadjuvant setting, and this has been attributed to increased oxygenation during the neoadjuvant periodCitation355. Still, a significant proportion of patients relapses, and a hypoxia biomarker could be useful for planning radiotherapy initiation and a possible need for concurrent hypoxia targeted therapy. In a xenograft model system, we demonstrated ADT-driven down-regulation of HIF1 target genes without changes in the hypoxic fractionCitation356. The down-regulation was probably a consequence of androgen withdrawal per se, since AR activation by androgens can contribute to HIF1 activation in a reversible mannerCitation357,Citation358. A HIF1 target gene signature is therefore not a reliable hypoxia biomarker in this context. However, since HIF1 signalling seemed to play a major role in the regressive phase of the tumours, the signature might be useful for monitoring ADT effect independent of oxygen status.
In conclusion our experience is that tumours classified as having a greater proportion of hypoxia using these signatures correlated with poorer prognosis in several cancer sites including head and neck, breast, lung and ovarian cancer, in agreement with other methods for identifying hypoxia. The prognostic information provided by hypoxia signatures could allow clinicians and patients to make better informed decisions when planning treatment strategies and this information may be particularly useful in cases where routine histological analysis is unable to provide prognostic valueCitation345. However, a more powerful application for hypoxia signatures is their use to predict which patients will respond to hypoxia-modifying or hypoxia-targeted treatments (see also Section “Tumour kinase profiling technology” below).
Predicting response to hypoxia targeted therapies
The development of a tumour hypoxia gene signature which allows classification of patients for treatment individualisation is a multi-step process. To identify possible candidate genes for a hypoxia gene signature, ideally, hypoxia-responsiveness should initially be verified in vitro under simplified and standardised conditions of varying pO2 conditions. Other micro-environmental conditions, typically co-existing with hypoxia (e.g. low pH), may modify hypoxia-driven changes in gene expression and needs to be taken into account. Given that not all micro-environmental conditions can be mimicked appropriately in vitro, additional studies verifying the in vivo hypoxia specificity of such candidate genes should also be performed. Finally, clinical testing of the gene-signature in patients should ultimately establish its prognostic value, and importantly, also whether the efficacy of hypoxic intervention may be predicted based on the gene signature. Proteins are the effectors of biological function, but biopsy-based mRNAs are easier to quantify objectively and routinely making them more attractive as clinical biomarkers. In accordance, most gene signatures are RNA-based.
Studies have shown that treatment with nimorazole to sensitise hypoxic cells to radiotherapy improved the disease outcome for head and neck cancer patientsCitation359. Importantly, the benefit from nimorazole was restricted to patients with relatively high levels of plasma osteopontin, a possible marker of tumour hypoxia Likewise, hypoxic tumours identified by [18F]MISO-PET were better controlled in patients assigned a hypoxia-targeted radio-chemotherapy regimen containing the hypoxic cytotoxin (tirapazamine) than patients assigned to standard radio-chemotherapyCitation360. These studies demonstrate the principle that identification of hypoxia and consequential tailoring of treatment strategy could benefit patients with hypoxic tumours. However, another implication highlighted in both of these studies is that patients with low levels of hypoxia did not receive any benefit from the hypoxia modifying/hypoxia targeted intervention, emphasising the importance of sparing patients more intensive and lesser tolerated treatments when these are not warranted. Several retrospective studies have shown how hypoxia metagenes can be used to predict treatment response.
Recently, a prognostic and predictive hypoxia gene signature for head and neck cancer patients was developed by Toustrup et al.Citation361,Citation362. Initially, a selection of robustly hypoxia-induced genes with little pH-dependency were identified from cell culture studies, using a panel of squamous cell carcinomas cell lines. Interestingly, this initial testing revealed that the hypoxia-driven expression of CAIX, a classical endogenous hypoxia marker, was highly suppressed under low pH conditions, making it questionable as a reliable marker for tissue hypoxia. Next, the in vivo hypoxia-specificity of selected pH-independent genes were verified by analysing gene expression profiles in hypoxic versus non-hypoxic tumour tissue dissected from xenograft tumours based on [18F]-FAZA (PET hypoxia tracer) autoradiography of frozen tissue sections. Using a training set of tumour tissue material derived from 58 patients with known hypoxia status, a 15-gene mRNA-based hypoxia classifier was developed. Finally, this classifier was validated in paraffin-embedded biopsy-material from 323 patients with HNSCC randomised for hypoxic modification or placebo in combination with radiotherapy, the DAHANCA 5 studyCitation232. Tumours categorised as “more hypoxic” on the basis of the classifier were associated with a significantly poorer clinical outcome than “less hypoxic” tumours. Importantly, outcome in patients with hypoxic tumours was improved and equalised to patients with “less hypoxic” tumours by addition of hypoxic modification with the radio-sensitiser nimorazole. The gene signature also revealed that not all patients with hypoxic tumours benefits from this intervention. Specifically, it was demonstrated that tumour hypoxia, as assessed by genetic analysis, were equally distributed among patients with HPV-driven disease and patients with tumours of other etiologies, but that patients with hypoxic HPV-positive tumours did not benefit from hypoxic intervention. This probably relates to the fact that HPV-positive tumour cells are much more sensitive to radiationCitation363, which may explain their relatively good prognosis and may reduce the potential added value of hypoxia-targeting in this sub-group of cancers. Some of the clinical data are summarised in .
Figure 9. Predictive impact of the 15-gene hypoxia gene signature stratified by HPV status (immunohistochemical detection of p16) on loco-regional control. HPV-negative / “more” hypoxic tumours (A), HPV-negative / “less” hypoxic tumours (B), HPV-positive / “more” hypoxic tumours (C), and HPV-positive / “less” hypoxic tumours (D). Loco-regional tumour control is described with the Kaplan–Meier method, compared using the log-rank test, and also expressed as adjusted hazard ratios (HR) using a multi-variate Cox proportional hazards model with the following parameters included: tumour and nodal classification, gender, and age. Modified from Toustrup et al.Citation362.
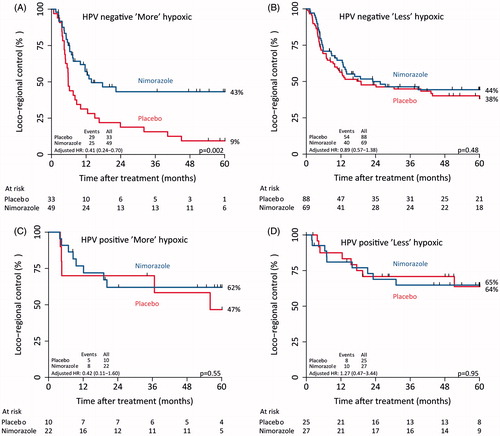
This example shows that proper patient characterisation prior to individualised treatment relies on both tumour microenvironment assessment and additional biological testing. In the DAHANCA 5 study, patients only received radiotherapy; however, current state-of-the-art treatment includes chemotherapy. In accordance, as preparation before a potential clinical implementation of the 15-gene hypoxia classifier, current efforts are put into a further validation of the classifier on more cohorts of HNSCC patients treated with radiotherapy/chemoradiotherapy.
Clinical and preclinical studies exploring the value of the 15-gene signature in other cancer types are currently being conducted, and to that end, recently published data by Winther et al.Citation364 suggests that the gene signature may also be useful for patient stratification in esophagus cancer. On-going preclinical work, focus on evaluation of the gene profile as a reliable marker of hypoxia (and a reliable predictor of the efficacy of hypoxic intervention) in other cancer types including colon and prostate.
Tumour kinase profiling technology
At the molecular level, tumour hypoxia promotes angiogenesis, metastasis, and therapy resistance through the alteration of oxygen-sensitive regulatory mechanismsCitation41,Citation365. The adaptive responses to hypoxic stress involve intrinsic activation of a range of signalling pathways mediated by receptor tyrosine kinases such as EGFR, VEGFR and PDGFR family members, collectively contributing to the continuous augmentation of the malignant phenotypeCitation366. Hence, receptor tyrosine kinase-governed signalling pathways are increasingly recognised as potential biomarkers for stratification of patients to molecularly individualised therapies.
In selecting patients for molecularly targeted agents, both as single-agent therapy and for optimisation of multi-modality cancer treatment, the prevailing gold standards for biomarkers are mainly based on detection of tumour gene aberrationsCitation367. However, such biomarkers may not be sufficient for the purpose since multiple gene aberrations, which in solid tumours often are the case rather than a single driving gene modification, will affect a wide range of components of the signalling network. Of further note, the plasticity of micro-environmental changes in hypoxic tumours will also contribute to the diversity in signalling activity. Consequently, methodologies comprising the resultant condition of interacting signalling effects may be particularly advantageous to identify functional biomarkers of molecularly targeted therapeutics. Within this frame of reference, kinase substrate array technologies are tools for global profiling of kinase activities in tissue samples without prior knowledge of which signalling pathways are activated, theoretically portraying the state of composite information flow through signalling cascades.
The Tyrosine Kinase PamChip® Array technology (PamGene International B.V., ‘s-Hertogenbosch, The Netherlands), which our Oslo University Hospital group has applied in studies of tumour biopsies from patients with rectal and prostate cancer and malignant melanomaCitation368–372, is an array containing 144 peptides, representing 100 different proteins. Each of these kinase targets consists of 13 or 14 amino acids with tyrosine residues for phosphorylation. Substrate phosphorylation levels are measured as signals from a fluorescent anti-phosphotyrosine antibody bound to the phosphorylated peptides. To provide additional information of specific signalling pathways that mechanistically may be important for the biological process of investigation, the tissue lysate can be incubated on the array also in the presence of a selected small-molecular kinase inhibitor for measurement of specific alterations in phosphorylation levels of array substrates. In this manner, the ex vivo substrate specificity of the kinase inhibiting agent may also indicate signalling mechanisms that potentially may be actionable tumour targets in patient treatment.
Future prospects on hypoxia detection and imaging
Well-designed studies will need to be conducted to demonstrate the value of hypoxia signatures for patient stratification in a prospective setting. These studies require clear standardisation guidelines for classifying hypoxia scores into high and low groups. In future, hypoxia signatures may become more sophisticated and this will help to derive additional information about tumour biology. For example, signatures that differentiate between acute and chronic hypoxia may be used to determine the contribution of each of these features to specific outcomes including metastasis and treatment response. Another interesting offshoot of this work has been the use of hypoxia signatures to identification novel co-expressed hypoxia-regulated genes. Investigating the role of these newly identified genes in hypoxia biology may reveal new drug targets for modulating the hypoxic responseCitation373.
New therapeutic anti-metastasis treatment
Stereotactic ablative body radiotherapy
Oligometastatic disease is cancer that has spread, but only to one or a small number of sites (classically ≤ 5 metastases in ≤ 3 organs). Although diseases arise in nature, their diagnostic categories are generated by man in ways that are useful to us. Oligometastatic disease is getting more attention because of advances in radiation dose delivery such as by stereotactic body radiation therapy (SBRT) where the radiation dose is delivered with high precision to the tumour.
Stereotactic ablative body radiotherapy (SABR) is a form of high-precision radiotherapy delivering extremely high ablative doses of radiation, usually in 3–8 fractions, combining reproducible patient immobilisation, tumour motion tracking and steep dose gradients, resulting in reduced normal tissue toxicity. SABR achieves excellent local control rates in patients with stage I/II non-small cell lung cancer (NSCLC) and liver metastases of colorectal cancer (CRC)Citation374. Nowadays, these favourable results of SABR are being transferred to patients with limited sites of metastatic disease originating from solid tumours (e.g. breast, NSCLC, head and neck, renal cell carcinoma, melanoma, CRC), both at primary diagnosis (synchronous) and during the course of diseaseCitation375–382. Tree et al. reports favourable local control rates of approximately 80% using SABR with few treatment-related side effectsCitation382.
Recently, the MAASTRO group found NSCLC patients with synchronous oligometastases to have a median progression-free survival (PFS) of 12.1 months when treated radically to all known metastatic sitesCitation383. However, in the vast majority of patients, disease-progression at a distance from the treated site occurs ultimately leading to extensive metastatic disease and cancer-related death. There is a great opportunity to combined modern systemic treatment such as hypoxia targeting drugs with SABR in patients with oligometastasis.
Locally advanced rectal cancer
Despite the introduction of multi-modal therapy for locally advanced rectal cancer (LARC), primarily the combination of surgery and radiation that frequently results in high rates of local control, a substantial number of patients will experience metastatic progression. A rational integration of molecularly targeted agents in combined-modality treatment regimens might cause both improvement of local control in poor-responding patients and reduction in metastasis risk. This strategy, however, will require a clear definition of functional biomarkers for treatment stratification.
Recognising that tumour hypoxia is a common determinant of resistance to cytotoxic therapies and metastatic behaviour, the prospective non-randomised study Locally Advanced Rectal Cancer—Radiation Response Prediction of neoadjuvant radiotherapy with concomitant chemotherapy followed by surgery and no further treatment in LARC (ClinicalTrials.gov NCT00278694) offered a unique opportunity to explore this intriguing concept in a defined clinical context. Utilising the Tyrosine Kinase PamChip® Array technology to analyse study patients' tumour samples, we hypothesised that the study might enable identification of potentially actionable therapeutic targets implicated in hypoxic tumour signalling.
At the time of diagnosis (baseline), tumour biopsy specimens were sampled at rigid proctoscopy from the study patients. Phosphorylation levels of array peptides generated by the tumour samples were correlated to histologic tumour response following neoadjuvant treatment. Essentially, patients with poor response had significantly elevated baseline tumour kinase activity, representing signalling mediated by VEGFR, EGFR and phosphatidylinositol-3-kinase (PI3K), compared to good-responding patientsCitation368. Next, it appeared that phosphorylation of array peptides representing PDGFR in particular was strongly inhibited by addition of the anti-angiogenic agent sunitinib to the tumour samples from patients without detectable tumour cells in the bone marrow at the time of diagnosisCitation369. The recognition that PDGFR-mediated signalling is central for maturation of pericytes during angiogenesisCitation366 makes it tempting to speculate that intact tumour angiogenic signalling of pericytes is associated with low likelihood of early systemic tumour dissemination in LARC. Of note, in a hypoxic tumour stroma, the vasculature is leaky because of a poorly functioning pericyte layer surrounding the endothelial cellsCitation384,Citation385, which further is associated with metastasis developmentCitation386.
Finally, unsupervised clustering analysis of the array phosphosubstrate data separated the tumour samples into two phenotypic populations. The smaller of the groups, displaying high tumour activities within the PI3K signalling network, showed a particularly aggressive disease course as almost a half of cases had developed metastatic disease by less than 1 year of follow-up. Hence, the study indicated that high tumour PI3K-mediated signalling is a biomarker for rapid failure of metastatic disease control in LARC patients following radical treatment of the pelvic cavityCitation370. Currently, no consensus exists to whether systemic therapy may reduce the risk of metastatic failureCitation387,Citation388, which may partly be explained by the paucity of biomarkers for risk assessment and treatment stratification. Given our findings that PI3K may be a key signalling orchestrator both of poor local tumour response to neoadjuvant treatment and importantly, of metastasis development, therapeutic targeting of components of the PI3K complex might be rational to integrate into combined-modality treatment regimens in LARC.
Need for combination treatment
It is was quite clear that to cure a cancer we need to kill several logs of cells and this can best be done by a combination of treatment classically: surgery, radiotherapy, chemotherapy, hormone therapy, targeted drugs and the recent emerging immunotherapy. Interestingly, hypoxia can be an obstacle for all of these six types of treatment. Indeed accumulating evidence implicates the biological responses to hypoxia and the alterations in these pathways in cancer as important contributors to tumourigenesis and treatment efficacy. This has recently prompted several investigations into the possibility of targeting treatment at the biological responses to hypoxia. We then need to rethink strategies to evaluate hypoxia targeting treatment: The classical “Response Evaluation Criteria In Solid Tumours” (RECIST) approach would not work because hypoxic cells are proliferating more slowly than well oxygenated cells. Their contribution to tumour growth or in case of specific targeting to tumour regression is limited in the short term.
An interesting approach is the “window-of-opportunity” clinical trial, to determine whether this trial design offers a valuable alternative to detect activity of the new therapeutic approaches (). The aim is to obtain knowledge about anti-tumour activity of the new therapeutic approaches in a disease state that is not disturbed by previous or simultaneous treatments. The end-point of the window-of-opportunity trial is a clinical end-point, or better an early biomarker of responseCitation389,Citation390.
Figure 10. Approach and design of the “window-of-opportunity” phase 2 trial with a hypoxia targeting drug combined with a conventional treatment.
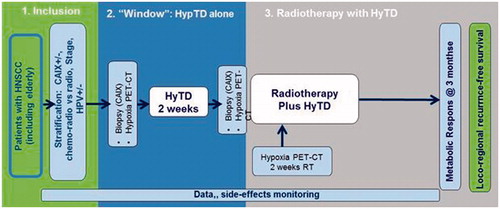
An example would be to do a hypoxia scan in head and neck cancer followed by a hypoxia targeted drug followed by a second hypoxia scan to see whether the hypoxic fraction is decreased then continue with the classical treatment, e.g. chemo-radiotherapy, with the new agent ().
Over the past decade, we have witnessed enormous advances in healthcare. As a result, the delivery of care for oncological patients has been greatly complicated by the rapid expansion of new diagnostic methods and treatment modalitiesCitation391. This evolution has created new challenges such as how to reach evidence level I in view of the ever-diminishing number of seemingly homogenous patients and the explosion of disease and patient parametersCitation392. The emergence of individualised medicine contrasts to a certain extent with well-established evidence-based medicine, where randomised trials are designed for selective populationCitation393.
Despite this complexity, individualised cancer treatment is a realistic goal. There is dramatic geneticCitation394, transcriptomicCitation395, histologicalCitation396 and micro-environmentalCitation397 heterogeneity within individual tumours, and even greater heterogeneity between patientsCitation398. But if personalised medicine is challenging and necessary, then new techniques and tools are urgently required to aid in clinical decision-making.
The central difficulty is deciding how to integrate diverse, multi-modal information (i.e. clinical, imaging and molecular data) in a transparent and quantitative manner to provide specific clinical predictions that accurately and robustly predict patient outcomes (i.e. generalisable for different patient populations).
Now accurate, externally validated prediction models are being rapidly developed, where multiple features related to the patient's disease are combined into an integrated prediction. The key, however, is standardisation, mainly in data acquisition in all areas, including molecular- and imaging-based assays, patients' preferences and possible treatments. This requires harmonised clinical guidelines, standardised image acquisition and analysis, validated biomarker assay criteria and data-sharing using the identical ontologies. But assessing clinical usefulness is just as important as standardising the development of prediction models with high-quality data, preferably by standardising the design of clinical trialsCitation398. These crucial steps are the basis of validated decision support systems, which in turn will allow the next steps of “shared decision making”.
Conclusions (and main results of the consortium)
Specific treatment of hypoxic cells in patients has four fundamental problems which need to be solved. The METOXIA consortium included expertise within all these areas and solutions to these problems have been addressed:
(a) The need of new methods to easily measure oxygenation and/or visualise hypoxic areas both in our pre-clinical models and in patient tumours before and during therapy.
A solution was developed for in vitro oxygen monitoring (see Section “Automated high throughput cell cultivation: fully automated cell culture maintenance”) where the oxygen sensor is moulded into the bottom of the disposable flask so that monitoring of pericellular oxygen for cells attached to the sensor area can be done on line. In animal models and in patients non-invasive methods, such as PET-imaging of the oxygenation status of the tumour have been developed. The most promising new development in this area is the new 2-nitroimidazole nucleoside analogue [18F]-HX4 (see Section “Diagnostics: hypoxia detection and imaging”). A recent clinical trial in NSCLC patients confirmed that the optimal contrast was obtained 4 h after administration. Research on the use of [18F]FPIMO is on-going with focus on the possibility of labelling pimonidazole in different positions with [18F] to improve tracer stability in vivo.
(b) The need of new and more clinically relevant models for pre-clinical testing, particularly models relevant for metastasis.
Both 3D-models using alginate as a scaffold material and 2D methods based on ex vivo tumour tissue explants were studied (see Section “Preclinical cancer models for the assessment of novel therapeutics targeting hypoxia”). Cell migration studies were used as an indicator for ability to metastasize and a first screening of patented compounds were performed using these methods. Animal studies of metastatic capability have been done using an orthotopic model of MDA-MB-231 injected into the mammary fat pad inducing spontaneous metastases in CBA nude mice (see Section “Models for metastasis”).
(c) The need to identify relevant hypoxia-related patterns of gene expression to improve individualisation of patient treatment.
Low density quantitative real-time polymerase chain reaction (qPCR)-based assays have been developed measuring multiple hypoxia-responsive markers in parallel to identify tumour hypoxia-related patterns of gene expression (hypoxia metagenes) (see Section “Assessment of tumour hypoxia using hypoxic metagenes”) for classification of patients for treatment individualisation. These methods can even distinguish between acute and protracted (chronic) hypoxia, which are factors of utmost importance regarding response to treatment as well as the ability to metastasize. The strength of the method was demonstrated in a cohort of 2000 breast cancers (see ).
(d) The need to identify and validate further hypoxia-specific targets essential for tumour growth/metastasis and to synthesise compounds and select potential drugs.
A wide variety of hypoxia-related cell-regulatory processes have been studied. Most emphasis was put on HIF-regulated cascades operating at moderate to weak hypoxia (<1% O2), and the UPR activated by endoplasmatic reticulum (ER) stress and operating at more severe hypoxia (<0.2%). Both pathways influence expression of several potential targets for drug development, but during the METOXIA project the prioritised targets were the HIF-regulated proteins CAIXCitation399,Citation400, the lactate transporter MCT4 and the PERK/eIF2α/ATF4-arm of the UPR. Specific inhibition of CAIX and the UPR pathway has been shown to halt tumour metastasis. There are in particular two compound patents which are being followed-up after the end of METOXIA, both relating to inhibition of CAIX. One of these represents compounds able to inactivate CAIX, the other represents compounds with a dual action: These compounds can both inactivate CAIX and act as hypoxic cell radio-sensitisers. For both these types of compounds the development will face the challenge which is common for all treatments specific for hypoxic cells: the compound is insufficient alone to inactivate a tumour. Treatment with the compound will have to be combined with conventional anti-cancer therapies eradicating the aerobic cancer cell population.
Declaration of interest
The authors report no conflicts of interest. The METOXIA project was an EC-financed collaborative project of FP7 (HEALTH-F2-2009-222741) named “Metastatic tumours facilitated by hypoxic tumour micro-environments” which ended by 31 July 2014.
References
- Young SD, Marshall RS, Hill RP. Hypoxia induces DNA overreplication and enhances metastatic potential of murine tumor cells. Proc Natl Acad Sci USA 1988;85:9533–7
- Young SD, Hill RP. Effects of reoxygenation on cells from hypoxic regions of solid tumors: analysis of transplanted murine tumors for evidence of DNA overreplication. Cancer Res 1990;50:5031–8
- Rofstad EK, Galappathi K, Mathiesen B, Ruud EB. Fluctuating and diffusion-limited hypoxia in hypoxia-induced metastasis. Clin Cancer Res 2007;13:1971–8
- McKeown SR. Defining normoxia, physoxia and hypoxia in tumours-implications for treatment response. Br J Radiol 2014;87:20130676
- Connett RJ, Honig CR, Gayeski TE, Brooks GA. Defining hypoxia: a systems view of VO2, glycolysis, energetics, and intracellular PO2. J Appl Physiol (Bethesda, MD) 1990;68:833–42
- Cairns RA, Kalliomaki T, Hill RP. Acute (cyclic) hypoxia enhances spontaneous metastasis of KHT murine tumors. Cancer Res 2001;61:8903–8
- Jiang BH, Semenza GL, Bauer C, Marti HH. Hypoxia-inducible factor 1 levels vary exponentially over a physiologically relevant range of O2 tension. Am J Physiol 1996;271:C1172–80
- Mottram MC. Factor of importance in radiosensitivity of tumours. Br J Radiol 1936;9:606–14
- Gray LH, Conger AD, Ebert M, et al. The concentration of oxygen dissolved in tissues at the time of irradiation as a factor in radiotherapy. Br J Radiol 1953;26:638–48
- Thomlinson RH, Gray LH. The histological structure of some human lung cancers and the possible implications for radiotherapy. Br J Cancer 1955;9:539–49
- Adams GE, Dewey DL. Hydrated electrons and radiobiological sensitisation. Biochem Biophys Res Commun 1963;12:473–7
- Vaupel P, Mayer A. Hypoxia in cancer: significance and impact on clinical outcome. Cancer Metastasis Rev 2007;26:225–39
- Lu X, Kang Y. Hypoxia and hypoxia-inducible factors: master regulators of metastasis. Clin Cancer Res 2010;16:5928–35
- Ortiz-Barahona A, Villar D, Pescador N, et al. Genome-wide identification of hypoxia-inducible factor binding sites and target genes by a probabilistic model integrating transcription-profiling data and in silico binding site prediction. Nucleic Acids Res 2010;38:2332–45
- Chen J. Regulation of tumor initiation and metastatic progression by Eph receptor tyrosine kinases. Adv Cancer Res 2012;114:1–20
- Choudhry H, Schodel J, Oikonomopoulos S, et al. Extensive regulation of the non-coding transcriptome by hypoxia: role of HIF in releasing paused RNApol2. EMBO Rep 2014;15:70–6
- Gómez-Maldonado L, Tiana M, Roche O, et al. EFNA3 long non-coding RNAs induced by hypoxia promote metastatic dissemination. Oncogene 2014. [Epub ahead of print]. doi: 10.1038/onc.2014.200
- Shweiki D, Neeman M, Itin A, Keshet E. Induction of vascular endothelial growth factor expression by hypoxia and by glucose deficiency in multicell spheroids: implications for tumor angiogenesis. Proc Natl Acad Sci USA 1995;92:768–72
- Bienes-Martinez R, Ordonez A, Feijoo-Cuaresma M, et al. Autocrine stimulation of clear-cell renal carcinoma cell migration in hypoxia via HIF-independent suppression of thrombospondin-1. Sci Rep 2012;2:788
- Good DJ, Polverini PJ, Rastinejad F, et al. A tumor suppressor-dependent inhibitor of angiogenesis is immunologically and functionally indistinguishable from a fragment of thrombospondin. Proc Natl Acad Sci USA 1990;87:6624–8
- Henkin J, Volpert OV. Therapies using anti-angiogenic peptide mimetics of thrombospondin-1. Expert Opin Therapeut Targets 2011;15:1369–86
- Veliceasa D, Ivanovic M, Hoepfner FT, et al. Transient potential receptor channel 4 controls thrombospondin-1 secretion and angiogenesis in renal cell carcinoma. FEBS J 2007;274:6365–77
- Zubac DP, Bostad L, Kihl B, et al. The expression of thrombospondin-1 and p53 in clear cell renal cell carcinoma: its relationship to angiogenesis, cell proliferation and cancer specific survival. J Urol 2009;182:2144–9
- Villar D, Ortiz-Barahona A, Gomez-Maldonado L, et al. Cooperativity of stress-responsive transcription factors in core hypoxia-inducible factor binding regions. PLoS One 2012;7:e45708
- Tiana M, Villar D, Perez-Guijarro E, et al. A role for insulator elements in the regulation of gene expression response to hypoxia. Nucleic Acids Res 2012;40:1916–27
- Benej M, Pastorekova S, Pastorek J. Carbonic anhydrase IX: regulation and role in cancer. Sub-Cellular Biochem 2014;75:199–219
- Wykoff CC, Beasley NJ, Watson PH, et al. Hypoxia-inducible expression of tumor-associated carbonic anhydrases. Cancer Res 2000;60:7075–83
- Ditte P, Dequiedt F, Svastova E, et al. Phosphorylation of carbonic anhydrase IX controls its ability to mediate extracellular acidification in hypoxic tumors. Cancer Res 2011;71:7558–67
- Sedlakova O, Svastova E, Takacova M, et al. Carbonic anhydrase IX, a hypoxia-induced catalytic component of the pH regulating machinery in tumors. Frontiers Physiol 2014;4:400
- Svastova E, Witarski W, Csaderova L, et al. Carbonic anhydrase IX interacts with bicarbonate transporters in lamellipodia and increases cell migration via its catalytic domain. J Biol Chem 2012;287:3392–402
- Csaderova L, Debreova M, Radvak P, et al. The effect of carbonic anhydrase IX on focal contacts during cell spreading and migration. Frontier Physiol 2013;4:271
- Zatovicova M, Jelenska L, Hulikova A, et al. Carbonic anhydrase IX as an anticancer therapy target: preclinical evaluation of internalizing monoclonal antibody directed to catalytic domain. Curr Pharm Des 2010;16:3255–63
- Winum JY, Scozzafava A, Montero JL, Supuran CT. Inhibition of carbonic anhydrase IX: a new strategy against cancer. Anti-Cancer Agents Med Chem 2009;9:693–702
- Dubois L, Peeters S, Lieuwes NG, et al. Specific inhibition of carbonic anhydrase IX activity enhances the in vivo therapeutic effect of tumor irradiation. Radiother Oncol 2011;99:424–31
- Lou Y, McDonald PC, Oloumi A, et al. Targeting tumor hypoxia: suppression of breast tumor growth and metastasis by novel carbonic anhydrase IX inhibitors. Cancer Res 2011;71:3364–76
- Ditte Z, Ditte P, Labudova M, et al. Carnosine inhibits carbonic anhydrase IX-mediated extracellular acidosis and suppresses growth of HeLa tumor xenografts. BMC Cancer 2014;14:358
- Fukuda R, Zhang H, Kim JW, et al. HIF-1 regulates cytochrome oxidase subunits to optimize efficiency of respiration in hypoxic cells. Cell 2007;129:111–22
- Tello D, Balsa E, Acosta-Iborra B, et al. Induction of the mitochondrial NDUFA4L2 protein by HIF-1alpha decreases oxygen consumption by inhibiting complex I activity. Cell Metabol 2011;14:768–79
- Balsa E, Marco R, Perales-Clemente E, et al. NDUFA4 is a subunit of complex IV of the mammalian electron transport chain. Cell Metabol 2012;16:378–86
- Balsa E, Acosta-Iborra B, Tello D, et al. HIF-dependent vs. independent mechanisms that regulate Complex IV and oxygen consumption in hypoxia. 2014; submitted for publication
- Wouters BG, Koritzinsky M. Hypoxia signalling through mTOR and the unfolded protein response in cancer. Nat Rev Cancer 2008;8:851–64
- Koritzinsky M, Magagnin MG, van den Beucken T, et al. Gene expression during acute and prolonged hypoxia is regulated by distinct mechanisms of translational control. Embo J 2006;25:1114–25
- Koritzinsky M, Seigneuric R, Magagnin MG, et al. The hypoxic proteome is influenced by gene-specific changes in mRNA translation. Radiother Oncol 2005;76:177–86
- Bi M, Naczki C, Koritzinsky M, et al. ER stress-regulated translation increases tolerance to extreme hypoxia and promotes tumor growth. Embo J 2005;24:3470–81
- Walter P, Ron D. The unfolded protein response: from stress pathway to homeostatic regulation. Science (New York) 2011;334:1081–6
- Harding HP, Zhang Y, Zeng H, et al. An integrated stress response regulates amino acid metabolism and resistance to oxidative stress. Mol Cell 2003;11:619–33
- Koritzinsky M, Levitin F, van den Beucken T, et al. Two phases of disulfide bond formation have differing requirements for oxygen. J Cell Biol 2013;203:615–27
- Braakman I, Bulleid NJ. Protein folding and modification in the mammalian endoplasmic reticulum. Ann Rev Biochem 2011;80:71–99
- Tu BP, Weissman JS. The FAD- and O(2)-dependent reaction cycle of Ero1-mediated oxidative protein folding in the endoplasmic reticulum. Mol Cell 2002;10:983–94
- Rouschop KMA, van den Beucken T, Dubois L, et al. The unfolded protein response protects human tumor cells during hypoxia through regulation of the autophagy genes MAP1LC3B and ATG5. J Clin Invest 2010;120:127–41
- Zhang HF, Bosch-Marce M, Shimoda LA, et al. Mitochondrial autophagy is an HIF-1-dependent adaptive metabolic response to hypoxia. J Biol Chem 2008;283:10892–903
- Papandreou I, Lim AL, Laderoute K, Denko NC. Hypoxia signals autophagy in tumor cells via AMPK activity, independent of HIF-1, BNIP3, and BNIP3L. Cell Death Differ 2008;15:1572–81
- Klionsky DJ, Abeliovich H, Agostinis P, et al. Guidelines for the use and interpretation of assays for monitoring autophagy in higher eukaryotes. Autophagy 2008;4:151–75
- Ding WX, Ni HM, Gao WT, et al. Linking of autophagy to ubiquitin-proteasome system is important for the regulation of endoplasmic reticulum stress and cell viability. Am J Pathol 2007;171:513–24
- Iwata A, Christianson JC, Bucci M, et al. Increased susceptibility of cytoplasmic over nuclear polyglutamine aggregates to autophagic degradation. Proc Natl Acad Sci USA 2005;102:13135–40
- Rzymski T, Milani M, Pike L, et al. Regulation of autophagy by ATF4 in response to severe hypoxia. Oncogene 2010;29:4424–35
- Schaaf MB, Cojocari D, Keulers TG, et al. The autophagy associated gene, ULK1, promotes tolerance to chronic and acute hypoxia. Radiother Oncol 2013;108:529–34
- Pike LR, Singleton DC, Buffa F, et al. Transcriptional up-regulation of ULK1 by ATF4 contributes to cancer cell survival. Biochem J 2013;449:389–400
- Rouschop KMA, Ramaekers CHMA, Schaaf MBE, et al. Autophagy is required during cycling hypoxia to lower production of reactive oxygen species. Radiother Oncol 2009;92:411–16
- Dickhout JG, Carlisle RE, Jerome DE, et al. Integrated stress response modulates cellular redox state via induction of cystathionine gamma-lyase cross-talk between integrated stress response and thiol metabolism. J Biol Chem 2012;287:7603–14
- Rouschop KM, Dubois Lj Fau, Keulers TG, et al. PERK/eIF2alpha signaling protects therapy resistant hypoxic cells through induction of glutathione synthesis and protection against ROS. Proc Natl Acad Sci USA 2013;110:4622–7
- Fyles A, Milosevic M, Hedley D, et al. Tumor hypoxia has independent predictor impact only in patients with node-negative cervix cancer. J Clin Oncol 2002;20:680–7
- Cairns RA, Hill RP. Acute hypoxia enhances spontaneous lymph node metastasis in an orthotopic murine model of human cervical carcinoma. Cancer Res 2004;64:2054–61
- Mujcic H, Nagelkerke A Fau, Rouschop KMA, et al. Hypoxic activation of the PERK/eIF2alpha arm of the unfolded protein response promotes metastasis through induction of LAMP3. Clin Cancer Res 2013;19:6126–37
- Mujcic H, Rzymski T, Rouschop KM, et al. Hypoxic activation of the unfolded protein response (UPR) induces expression of the metastasis-associated gene LAMP3. Radiother Oncol 2009;92:450–9
- Greenman C, Stephens P, Smith R, et al. Patterns of somatic mutation in human cancer genomes. Nature 2007;446:153–8
- Cancer Genome Atlas N. Comprehensive molecular portraits of human breast tumours. Nature 2012;490:61–70
- Romero-Ramirez L, Cao HB, Nelson D, et al. XBP1 is essential for survival under hypoxic conditions and is required for tumor growth. Cancer Res 2004;64:5943–7
- Cojocari D, Vellanki RN, Sit B, et al. New small molecule inhibitors of UPR activation demonstrate that PERK, but not IRE1 alpha signaling is essential for promoting adaptation and survival to hypoxia. Radiother Oncol 2013;108:541–7
- Atkins C, Liu Q, Minthorn E, et al. Characterization of a novel PERK kinase inhibitor with antitumor and antiangiogenic activity. Cancer Res 2013;73:1993–2002
- Wang H, Blais J, Ron D, Cardozo T. Structural determinants of PERK inhibitor potency and selectivity. Chem Biol Drug Design 2010;76:480–95
- Cross BC, Bond PJ, Sadowski PG, et al. The molecular basis for selective inhibition of unconventional mRNA splicing by an IRE1-binding small molecule. Proc Natl Acad Sci USA 2012;109:E869–78
- Fribley AM, Cruz PG, Miller JR, et al. Complementary cell-based high-throughput screens identify novel modulators of the unfolded protein response. J Biomol Screen 2011;16:825–35
- Papandreou I, Denko NC, Olson M, et al. Identification of an Ire1alpha endonuclease specific inhibitor with cytotoxic activity against human multiple myeloma. Blood 2011;117:1311–14
- Korennykh AV, Egea PF, Korostelev AA, et al. The unfolded protein response signals through high-order assembly of Ire1. Nature 2009;457:687–93
- Wang L, Perera Bg Fau, Hari SB, et al. Divergent allosteric control of the IRE1alpha endoribonuclease using kinase inhibitors. Nat Chem Biol 2012;8:982–9
- Axten JM, MedinaJr Fau, Feng Y, et al. Discovery of 7-methyl-5-(1-{[3-(trifluoromethyl)phenyl]acetyl}-2,3-dihydro-1H-indol-5-yl)-7H-p yrrolo[2,3-d]pyrimidin-4-amine (GSK2606414), a potent and selective first-in-class inhibitor of protein kinase R (PKR)-like endoplasmic reticulum kinase (PERK). J Med Chem 2012;55:7193–207
- Sidrauski C, Acosta-Alvear D, Khoutorsky A, et al. Pharmacological brake-release of mRNA translation enhances cognitive memory. eLife 2013;2:e00498. doi: 10.7554/eLife.00498
- Hawkins BJ, Madesh M, Kirkpatrick CJ, Fisher AB. Superoxide flux in endothelial cells via the chloride channel-3 mediates intracellular signaling. Mol Biol Cell 2007;18:2002–12
- Han D, Antunes F, Canali R, et al. Voltage-dependent anion channels control the release of the superoxide anion from mitochondria to cytosol. J Biol Chem 2003;278:5557–63
- Gorlach A, Kietzmann T. Superoxide and derived reactive oxygen species in the regulation of hypoxia-inducible factors. Methods Enzymol 2007;435:421–46
- Hybertson BM, Gao B, Bose SK, McCord JM. Oxidative stress in health and disease: the therapeutic potential of Nrf2 activation. Mol Aspects Med 2011;32:234–46
- Muller FL, Lustgarten MS, Jang Y, et al. Trends in oxidative aging theories. Free Radic Biol Med 2007;43:477–503
- Gorlach A, Kietzmann T, Hess J. Redox signaling through NADPH oxidases: involvement in vascular proliferation and coagulation. Ann New York Acad Sci 2002;973:505–7
- Bedard K, Krause KH. The NOX family of ROS-generating NADPH oxidases: physiology and pathophysiology. Physiol Rev 2007;87:245–313
- BelAiba RS, Djordjevic T, Petry A, et al. NOX5 variants are functionally active in endothelial cells. Free Radic Biol Med 2007;42:446–59
- Lambeth JD, Kawahara T, Diebold B. Regulation of Nox and Duox enzymatic activity and expression. Free Radic Biol Med 2007;43:319–31
- Petry A, Weitnauer M, Gorlach A. Receptor activation of NADPH oxidases. Antioxid Redox Signal 2010;13:467–87
- Wang GL, Jiang BH, Semenza GL. Effect of altered redox states on expression and DNA-binding activity of hypoxia-inducible factor 1. Biochem Biophys Res Commun 1995;212:550–6
- Huang LE, Arany Z, Livingston DM, Bunn HF. Activation of hypoxia-inducible transcription factor depends primarily upon redox-sensitive stabilization of its alpha subunit. J Biol Chem 1996;271:32253–9
- Welsh SJ, Bellamy WT, Briehl MM, Powis G. The redox protein thioredoxin-1 (Trx-1) increases hypoxia-inducible factor 1alpha protein expression: Trx-1 overexpression results in increased vascular endothelial growth factor production and enhanced tumor angiogenesis. Cancer Res 2002;62:5089–95
- Liu Q, Berchner-Pfannschmidt U, Moller U, et al. A Fenton reaction at the endoplasmic reticulum is involved in the redox control of hypoxia-inducible gene expression. Proc Natl Acad Sci USA 2004;101:4302–7
- Wang M, Kirk JS, Venkataraman S, et al. Manganese superoxide dismutase suppresses hypoxic induction of hypoxia-inducible factor-1alpha and vascular endothelial growth factor. Oncogene 2005;24:8154–66
- BelAiba RS, Djordjevic T, Bonello S, et al. Redox-sensitive regulation of the HIF pathway under non-hypoxic conditions in pulmonary artery smooth muscle cells. Biol Chem 2004;385:249–57
- Bonello S, Zahringer C, BelAiba RS, et al. Reactive oxygen species activate the HIF-1alpha promoter via a functional NFkappaB site. Arterioscler Thromb Vasc Biol 2007;27:755–61
- Diebold I, Flugel D, Becht S, et al. The hypoxia-inducible factor-2alpha is stabilized by oxidative stress involving NOX4. Antioxidants Redox Signal 2010;13:425–36
- Gorlach A, Diebold I, Schini-Kerth VB, et al. Thrombin activates the hypoxia-inducible factor-1 signaling pathway in vascular smooth muscle cells: role of the p22(phox)-containing NADPH oxidase. Circulation Res 2001;89:47–54
- Chandel NS, McClintock DS, Feliciano CE, et al. Reactive oxygen species generated at mitochondrial complex III stabilize hypoxia-inducible factor-1alpha during hypoxia: a mechanism of O2 sensing. J Biol Chem 2000;275:25130–8
- Kietzmann T, Samoylenko A, Roth U, Jungermann K. Hypoxia-inducible factor-1 and hypoxia response elements mediate the induction of plasminogen activator inhibitor-1 gene expression by insulin in primary rat hepatocytes. Blood 2003;101:907–14
- Tacchini L, De Ponti C, Matteucci E, et al. Hepatocyte growth factor-activated NF-kappaB regulates HIF-1 activity and ODC expression, implicated in survival, differently in different carcinoma cell lines. Carcinogenesis 2004;25:2089–100
- Richard DE, Berra E, Pouyssegur J. Nonhypoxic pathway mediates the induction of hypoxia-inducible factor 1alpha in vascular smooth muscle cells. J Biol Chem 2000;275:26765–71
- Diebold I, Petry A, Sabrane K, et al. The HIF1 target gene NOX2 promotes angiogenesis through urotensin-II. J Cell Sci 2012;125:956–64
- Stiehl DP, Jelkmann W, Wenger RH, Hellwig-Burgel T. Normoxic induction of the hypoxia-inducible factor 1alpha by insulin and interleukin-1beta involves the phosphatidylinositol 3-kinase pathway. FEBS Lett 2002;512:157–62
- Haddad JJ, Land SC. A non-hypoxic, ROS-sensitive pathway mediates TNF-alpha-dependent regulation of HIF-1alpha. FEBS Lett 2001;505:269–74
- Diebold I, Djordjevic T, Hess J, Gorlach A. Rac-1 promotes pulmonary artery smooth muscle cell proliferation by upregulation of plasminogen activator inhibitor-1: role of NFkappaB-dependent hypoxia-inducible factor-1alpha transcription. Thromb Haemost 2008;100:1021–8
- Petry A, BelAiba RS, Weitnauer M, Gorlach A. Inhibition of endothelial nitric oxyde synthase increases capillary formation via Rac1-dependent induction of hypoxia-inducible factor-1alpha and plasminogen activator inhibitor-1. Thromb Haemost 2012;108:849–62
- Diebold I, Djordjevic T, Petry A, et al. Phosphodiesterase 2 mediates redox-sensitive endothelial cell proliferation and angiogenesis by thrombin via Rac1 and NADPH oxidase 2. Circ Res 2009;104:1169–77
- Block K, Gorin Y. Aiding and abetting roles of NOX oxidases in cellular transformation. Nat Rev Cancer 2012;12:627–37
- Moon EJ, Sonveaux P, Porporato PE, et al. NADPH oxidase-mediated reactive oxygen species production activates hypoxia-inducible factor-1 (HIF-1) via the ERK pathway after hyperthermia treatment. Proc Natl Acad Sci USA 2010;107:20477–82
- Goyal P, Weissmann N, Grimminger F, et al. Upregulation of NAD(P)H oxidase 1 in hypoxia activates hypoxia-inducible factor 1 via increase in reactive oxygen species. Free Radic Biol Med 2004;36:1279–88
- Xia C, Meng Q, Liu LZ, et al. Reactive oxygen species regulate angiogenesis and tumor growth through vascular endothelial growth factor. Cancer Res 2007;67:10823–30
- Block K, Gorin Y, Hoover P, et al. NAD(P)H oxidases regulate HIF-2alpha protein expression. J Biol Chem 2007;282:8019–26
- Maranchie JK, Zhan Y. NOX4 is critical for hypoxia-inducible factor 2-alpha transcriptional activity in von Hippel–Lindau-deficient renal cell carcinoma. Cancer Res 2005;65:9190–3
- Block K, Gorin Y, New DD, et al. The NADPH oxidase subunit p22phox inhibits the function of the tumor suppressor protein tuberin. Am J Pathol 2010;176:2447–55
- Gerald D, Berra E, Frapart YM, et al. JunD reduces tumor angiogenesis by protecting cells from oxidative stress. Cell 2004;118:781–94
- Knowles HJ, Raval RR, Harris AL, Ratcliffe PJ. Effect of ascorbate on the activity of hypoxia-inducible factor in cancer cells. Cancer Res 2003;63:1764–8
- Epstein AC, Gleadle JM, McNeill LA, et al. C. elegans EGL-9 and mammalian homologs define a family of dioxygenases that regulate HIF by prolyl hydroxylation. Cell 2001;107:43–54
- Page EL, Chan DA, Giaccia AJ, et al. Hypoxia-inducible factor-1alpha stabilization in nonhypoxic conditions: role of oxidation and intracellular ascorbate depletion. Mol Biol Cell 2008;19:86–94
- Flashman E, Davies SL, Yeoh KK, Schofield CJ. Investigating the dependence of the hypoxia-inducible factor hydroxylases (factor inhibiting HIF and prolyl hydroxylase domain 2) on ascorbate and other reducing agents. Biochem J 2010;427:135–42
- Mecinovic J, Chowdhury R, Flashman E, Schofield CJ. Use of mass spectrometry to probe the nucleophilicity of cysteinyl residues of prolyl hydroxylase domain 2. Anal Biochem 2009;393:215–21
- Nytko KJ, Maeda N, Schlafli P, et al. Vitamin C is dispensable for oxygen sensing in vivo. Blood 2011;117:5485–93
- Gorlach A. Regulation of HIF-1 at the transcriptional level. Curr Pharm Des 2009;15:3844–52
- Rius J, Guma M, Schachtrup C, et al. NF-kappaB links innate immunity to the hypoxic response through transcriptional regulation of HIF-1alpha. Nature 2008;453:807–11
- van Uden P, Kenneth NS, Rocha S. Regulation of hypoxia-inducible factor-1alpha by NF-kappaB. Biochem J 2008;412:477–84
- Gorlach A, Bonello S. The cross-talk between NF-kappaB and HIF-1: further evidence for a significant liaison. Biochem J 2008;412:e17–19
- Belaiba RS, Bonello S, Zahringer C, et al. Hypoxia up-regulates hypoxia-inducible factor-1alpha transcription by involving phosphatidylinositol 3-kinase and nuclear factor kappaB in pulmonary artery smooth muscle cells. Mol Biol Cell 2007;18:4691–7
- Kracun D, Riess F, Kanchev I, et al. The beta3-integrin binding protein beta3-endonexin is a novel negative regulator of hypoxia-inducible factor-1. Antioxidant Redox Signal 2014;20:1964–76
- Diebold I, Petry A, Djordjevic T, et al. Reciprocal regulation of Rac1 and PAK-1 by HIF-1alpha: a positive-feedback loop promoting pulmonary vascular remodeling. Antioxidant Redox Signal 2010;13:399–412
- Fatrai S, Wierenga AT, Daenen SM, et al. Identification of HIF2alpha as an important STAT5 target gene in human hematopoietic stem cells. Blood 2011;117:3320–30
- Zhong H, Chiles K, Feldser D, et al. Modulation of hypoxia-inducible factor 1alpha expression by the epidermal growth factor/phosphatidylinositol 3-kinase/PTEN/AKT/FRAP pathway in human prostate cancer cells: implications for tumor angiogenesis and therapeutics. Cancer Res 2000;60:1541–5
- Laughner E, Taghavi P, Chiles K, et al. HER2 (neu) signaling increases the rate of hypoxia-inducible factor 1alpha (HIF-1alpha) synthesis: novel mechanism for HIF-1-mediated vascular endothelial growth factor expression. Mol Cell Biol 2001;21:3995–4004
- Djordjevic T, Pogrebniak A, BelAiba RS, et al. The expression of the NADPH oxidase subunit p22phox is regulated by a redox-sensitive pathway in endothelial cells. Free Radic Biol Med 2005;38:616–30
- Wenner CE. Targeting mitochondria as a therapeutic target in cancer. J Cell Physiol 2012;227:450–6
- Page EL, Robitaille GA, Pouyssegur J, Richard DE. Induction of hypoxia-inducible factor-1alpha by transcriptional and translational mechanisms. J Biol Chem 2002;277:48403–9
- Zhou J, Callapina M, Goodall GJ, Brune B. Functional integrity of nuclear factor kappaB, phosphatidylinositol 3′-kinase, and mitogen-activated protein kinase signaling allows tumor necrosis factor alpha-evoked Bcl-2 expression to provoke internal ribosome entry site-dependent translation of hypoxia-inducible factor 1alpha. Cancer Res 2004;64:9041–8
- Nayak BK, Feliers D, Sudarshan S, et al. Stabilization of HIF-2alpha through redox regulation of mTORC2 activation and initiation of mRNA translation. Oncogene 2012;32:3147–55
- Doerries C, Grote K, Hilfiker-Kleiner D, et al. Critical role of the NAD(P)H oxidase subunit p47phox for left ventricular remodeling/dysfunction and survival after myocardial infarction. Circulation Res 2007;100:894–903
- Murdoch CE, Grieve DJ, Cave AC, et al. NADPH oxidase and heart failure. Curr Opin Pharmacol 2006;6:148–53
- Kleikers PW, Wingler K, Hermans JJ, et al. NADPH oxidases as a source of oxidative stress and molecular target in ischemia/reperfusion injury. J Mol Med 2012;90:1391–406
- Nair D, Dayyat EA, Zhang SX, et al. Intermittent hypoxia-induced cognitive deficits are mediated by NADPH oxidase activity in a murine model of sleep apnea. PLoS One 2011;6:e19847
- Malec V, Gottschald OR, Li S, et al. HIF-1 alpha signaling is augmented during intermittent hypoxia by induction of the Nrf2 pathway in NOX1-expressing adenocarcinoma A549 cells. Free Radic Biol Med 2010;48:1626–35
- Hsieh CH, Wu CP, Lee HT, et al. NADPH oxidase subunit 4 mediates cycling hypoxia-promoted radiation resistance in glioblastoma multiforme. Free Radic Biol Med 2012;53:649–58
- Urao N, McKinney RD, Fukai T, Ushio-Fukai M. NADPH oxidase 2 regulates bone marrow microenvironment following hindlimb ischemia: role in reparative mobilization of progenitor cells. Stem Cells 2012;30:923–34
- Prabhakar NR, Semenza GL. Adaptive and maladaptive cardiorespiratory responses to continuous and intermittent hypoxia mediated by hypoxia-inducible factors 1 and 2. Physiol Rev 2012;92:967–1003
- Djordjevic T, BelAiba RS, Bonello S, et al. Human urotensin II is a novel activator of NADPH oxidase in human pulmonary artery smooth muscle cells. Arterioscler Thromb Vasc Biol 2005;25:519–25
- Liu JQ, Zelko IN, Erbynn EM, et al. Hypoxic pulmonary hypertension: role of superoxide and NADPH oxidase (gp91phox). Am J Physiol Lung Cell Mol Physiol 2006;290:L2–10
- Weissmann N, Zeller S, Schafer RU, et al. Impact of mitochondria and NADPH oxidases on acute and sustained hypoxic pulmonary vasoconstriction. Am J Respir Cell Mol Biol 2006;34:505–13
- Diebold I, Petry A, Hess J, Gorlach A. The NADPH oxidase subunit NOX4 is a new target gene of the hypoxia-inducible factor-1. Mol Biol Cell 2010;21:2087–96
- Mittal M, Roth M, Konig P, et al. Hypoxia-dependent regulation of nonphagocytic NADPH oxidase subunit NOX4 in the pulmonary vasculature. Circulation Res 2007;101:258–67
- Li J, Wang JJ, Yu Q, et al. Inhibition of reactive oxygen species by Lovastatin downregulates vascular endothelial growth factor expression and ameliorates blood-retinal barrier breakdown in db/db mice: role of NADPH oxidase 4. Diabetes 2010;59:1528–38
- Kieninger J DA, Aravindalochanan K, Jobst G, et al. Amperometric oxygen sensor array with novel chronoamperometric protocols for hypoxic tumor cell cultures. TRANSDUCERS and EUROSENSORS ‘07 – 4th International Conference on Solid-State Sensors, Actuators and Microsystems 4300531; Lyon; pp. 1907–10
- Ebbesen P, Pettersen EO, Gorr TA, et al. Taking advantage of tumor cell adaptations to hypoxia for developing new tumor markers and treatment strategies. J Enzyme Inhib Med Chem 2009;24:1–39
- Kieninger J, Aravindalochanan K, Sandvik JA, et al. Pericellular oxygen monitoring with integrated sensor chips for reproducible cell culture experiments. Cell Prolif 2014;47:180–8
- Jobst G, Moser I, Varahram M, et al. Thin-film microbiosensors for glucose-lactate monitoring. Analy Chem 1996;68:3173–9
- Schneider BL, Kulesz-Martin M. Destructive cycles: the role of genomic instability and adaptation in carcinogenesis. Carcinogenesis 2004;25:2033–44
- Chen EI, Hewel J, Krueger JS, et al. Adaptation of energy metabolism in breast cancer brain metastases. Cancer Res 2007;67:1472–86
- Halliwell B. Oxidative stress and cancer: have we moved forward? Biochem J 2007;401:1–11
- Swartz HM, Gutierrez PL. Free radical increases in cancer: evidence that there is not a real increase. Science (New York) 1977;198:936–8
- Hafeman DG, Parce JW, McConnell HM. Light-addressable potentiometric sensor for biochemical systems. Science (New York) 1988;240:1182–5
- Owicki JC, Parce JW. Biosensors based on the energy metabolism of living cells: the physical chemistry and cell biology of extracellular acidification. Biosens Bioelectron 1992;7:255–72
- Hafner F. Cytosensor Microphysiometer: technology and recent applications. Biosens Bioelectron 2000;15:149–58
- Eklund SE TD, Kozlov E, Prokop A, Cliffel DE. A microphysiometer for simultaneous measurement of changes in extracellular glucose, lactate, oxygen, and acidification rate. Anal Chem 2004;76:519–27
- Eklund SE SR, Wikswo J, Baudenbacher F, Prokop A. Multianalyte microphysiometry as a tool in metabolomics and systems biology. J Electroanal Chem 2006;587:333–9
- Ehret R, Baumann W, Brischwein M, et al. Monitoring of cellular behaviour by impedance measurements on interdigitated electrode structures. Biosens Bioelectron 1997;12:29–41
- Lehmann M, Baumann W, Brischwein M, et al. Non-invasive measurement of cell membrane associated proton gradients by ion-sensitive field effect transistor arrays for microphysiological and bioelectronical applications. Biosens Bioelectron 2000;15:117–24
- Lehmann M, Baumann W, Brischwein M, et al. Simultaneous measurement of cellular respiration and acidification with a single CMOS ISFET. Biosens Bioelectron 2001;16:195–203
- Wolf B, Brischwein M, Baumann W, et al. Monitoring of cellular signalling and metabolism with modular sensor-technique: the PhysioControl-Microsystem (PCM). Biosens Bioelectron 1998;13:501–9
- Brischwein M, Motrescu ER, Cabala E, et al. Functional cellular assays with multiparametric silicon sensor chips. Lab Chip 2003;3:234–40
- Thedinga E, Kob A, Holst H, et al. Online monitoring of cell metabolism for studying pharmacodynamic effects. Toxicol Appl Pharmacol 2007;220:33–44
- Mestres P, Morguet A. The Bionas technology for anticancer drug screening. Expert Opin Drug Discov 2009;4:785–97
- Weltin A, Slotwinski K, Kieninger J, et al. Cell culture monitoring for drug screening and cancer research: a transparent, microfluidic, multi-sensor microsystem. Lab Chip 2014;14:138–46
- Hogan C, Simons S, Zhang H, Burdick D. Living with irresolute cell lines in an automated world. J Assoc Lab Automat 2008;13:159–67
- Kempner ME, Felder RA. A review of cell culture automation. J Assoc Lab Automat 2002;7:56–62
- Triaud F, Clenet DH, Cariou Y, et al. Evaluation of automated cell culture incubators. J Assoc Lab Automat 2003;8:82–6
- Thomas RJ, Chandra A, Hourd PC, Williams DJ. Cell culture automation and quality engineering: a necessary partnership to develop optimized manufacturing processes for cell-based therapies. JALA - J Assoc Lab Automat 2008;13:152–8
- Franscini N, Wuertz K, Patocchi-Tenzer I, et al. Development of a novel automated cell isolation, expansion, and characterization platform. J Lab Automat 2011;16:204–13
- Jain S, Sondervan D, Rizzu P, et al. The complete automation of cell culture: improvements for high-throughput and high-content screening. J Biomol Screen 2011;16:932–9
- Ngibuini M. Reducing biomanufacturing bottlenecks. Genetic Eng Biotechnol News 2013;33:34–5
- Maddox CB, Rasmussen L, White EL. Adapting cell-based assays to the high-throughput screening platform: problems encountered and lessons learned. J Assoc Lab Automat 2008;13:168–73
- Su X, Theberge AB, January CT, Beebe DJ. Effect of microculture on cell metabolism and biochemistry: do cells get stressed in microchannels? Analy Chem 2013;85:1562–70
- Kuwae S, Ohda T, Tamashima H, et al. Development of a fed-batch culture process for enhanced production of recombinant human antithrombin by Chinese hamster ovary cells. J Biosci Bioeng 2005;100:502–10
- Yeo D, Kiparissides A, Cha JM, et al. Improving embryonic stem cell expansion through the combination of perfusion and bioprocess model design. PLoS ONE 2013;8
- Adams T, Noack U, Frick T, et al. Increasing efficiency in protein supply and cell production by combining single-use bioreactor technology and perfusion: an off-the-shelf, single-use perfusion system enables high-density cell culture with high viability and high protein yield. BioPharm Int 2011;24:s4–11
- Sciences GEHL. Application note 29-0051-80 AA and 28-9606-57 AC. Uppsala: GE Healthcare Life Sciences; 2012
- Hu S, Deng L, Wang H, et al. Bioprocess development for the production of mouse-human chimeric anti-epidermal growth factor receptor vIII antibody C12 by suspension culture of recombinant Chinese hamster ovary cells. Cytotechnology 2011;63:247–58
- Kleman GL, Chalmers JJ, Luli GW, Strohl WR. Glucose-stat, a glucose-controlled continuous culture. Appl Environ Microbiol 1991;57:918–23
- Moser I, Jobst G, Urban GA. Biosensor arrays for simultaneous measurement of glucose, lactate, glutamate, and glutamine. Biosens Bioelectron 2002;17:297–302
- Moser I, Jobst G. Pre-calibrated biosensors for single-use applications. Chemie-Ingenieur-Technik 2013;85:172–8
- Poon E, Harris AL, Ashcroft M. Targeting the hypoxia-inducible factor (HIF) pathway in cancer. Expert Rev Mol Med 2009;11:e26. doi: 10.1017/S1462399409001173
- Kioi M, Vogel H, Schultz G, et al. Inhibition of vasculogenesis, but not angiogenesis, prevents the recurrence of glioblastoma after irradiation in mice. J Clin Invest 2010;120:694–705
- Chau NM, Rogers P, Aherne W, et al. Identification of novel small molecule inhibitors of hypoxia-inducible factor-1 that differentially block hypoxia-inducible factor-1 activity and hypoxia-inducible factor-1alpha induction in response to hypoxic stress and growth factors. Cancer Res 2005;65:4918–28
- Rapisarda A, Hollingshead M, Uranchimeg B, et al. Increased antitumor activity of bevacizumab in combination with hypoxia inducible factor-1 inhibition. Mol Cancer Ther 2009;8:1867–77
- Rogers JL, Bayeh L, Scheuermann TH, et al. Development of inhibitors of the PAS-B domain of the HIF-2alpha transcription factor. J Med Chem 2013;56:1739–47
- Kung AL, Zabludoff SD, France DS, et al. Small molecule blockade of transcriptional coactivation of the hypoxia-inducible factor pathway. Cancer Cell 2004;6:33–43
- Miranda E, Nordgren IK, Male AL, et al. A cyclic peptide inhibitor of HIF-1 heterodimerization that inhibits hypoxia signaling in cancer cells. J Am Chem Soc 2013;135:10418–25
- Baker LC, Boult JK, Walker-Samuel S, et al. The HIF-pathway inhibitor NSC-134754 induces metabolic changes and anti-tumour activity while maintaining vascular function. Br J Cancer 2012;106:1638–47
- Carroll VA, Ashcroft M. Regulation of angiogenic factors by HDM2 in renal cell carcinoma. Cancer Res 2008;68:545–52
- Hickin JA, Ahmed A, Fucke K, et al. The synthesis and structure revision of NSC-134754. Chem Commun (Camb) 2014;50:1238–40
- Yang J, Ahmed A, Poon E, et al. Small-molecule activation of p53 blocks hypoxia-inducible factor 1alpha and vascular endothelial growth factor expression in vivo and leads to tumor cell apoptosis in normoxia and hypoxia. Mol Cell Biol 2009;29:2243–53
- Ahmed A, Yang J, Maya-Mendoza A, et al. Pharmacological activation of a novel p53-dependent S-phase checkpoint involving CHK-1. Cell Death Dis 2011;2:e160. doi:10.1038/cddis.2011.42
- Yang J, Ahmed A, Ashcroft M. Activation of a unique p53-dependent DNA damage response. Cell Cycle 2009;8:1630–2
- Vousden KH, Lu X. Live or let die: the cell's response to p53. Nat Rev Cancer 2002;2:594–604
- Bardos JI, Chau NM, Ashcroft M. Growth factor-mediated induction of HDM2 positively regulates hypoxia-inducible factor 1alpha expression. Mol Cell Biol 2004;24:2905–14
- Supiot S, Hill RP, Bristow RG. Nutlin-3 radiosensitizes hypoxic prostate cancer cells independent of p53. Mol Cancer Ther 2008;7:993–9
- Vassilev LT. MDM2 inhibitors for cancer therapy. Trends Mol Med 2007;13:23–31
- Vassilev LT, Vu BT, Graves B, et al. In vivo activation of the p53 pathway by small-molecule antagonists of MDM2. Science 2004;303:844–8
- Secchiero P, Corallini F, Gonelli A, et al. Antiangiogenic activity of the MDM2 antagonist nutlin-3. Circ Res 2007;100:61–9
- LaRusch GA, Jackson MW, Dunbar JD, et al. Nutlin3 blocks vascular endothelial growth factor induction by preventing the interaction between hypoxia inducible factor 1alpha and Hdm2. Cancer Res 2007;67:450–4
- Ahmed A, Yang J, Maya-Mendoza A, et al. Pharmacological activation of a novel p53-dependent S-phase checkpoint involving CHK-1. Cell Death Dis 2011;2:e160. doi:10.1038/cddis.2011.42
- Selvarajah J, Nathawat K, Moumen A, et al. Chemotherapy-mediated p53-dependent DNA damage response in clear cell renal cell carcinoma: role of the mTORC1/2 and hypoxia-inducible factor pathways. Cell Death Dis 2013;4:e865. doi: 10.1038/cddis.2013.395
- Kaelin WG Jr. Treatment of kidney cancer: insights provided by the VHL tumor-suppressor protein. Cancer 2009;115:2262–72
- Shen C, Kaelin WG Jr. The VHL/HIF axis in clear cell renal carcinoma. Semin Cancer Biol 2013;23:18–25
- Roe JS, Kim H, Lee SM, et al. p53 stabilization and transactivation by a von Hippel-Lindau protein. Mol Cell 2006;22:395–405
- Maxwell PH, Wiesener MS, Chang GW, et al. The tumour suppressor protein VHL targets hypoxia-inducible factors for oxygen-dependent proteolysis. Nature 1999;399:271–5
- Pelicano H, Martin DS, Xu RH, Huang P. Glycolysis inhibition for anticancer treatment. Oncogene 2006;25:4633–46
- Maity A, Tuttle SW. 2-Deoxyglucose and radiosensitization: teaching an old DOG new tricks? Cancer Biol Ther 2006;5:824–6
- Ellinghaus P, Heisler I, Unterschemmann K, et al. BAY 87-2243, a highly potent and selective inhibitor of hypoxia-induced gene activation has antitumor activities by inhibition of mitochondrial complex I. Cancer Med 2013;2:611–24
- Yang J, Staples O, Thomas LW, et al. Human CHCHD4 mitochondrial proteins regulate cellular oxygen consumption rate and metabolism and provide a critical role in hypoxia signaling and tumor progression. J Clin Invest 2012;122:600–11
- Gupta GP, Massague J. Cancer metastasis: building a framework. Cell 2006;127:679–95
- Pastorekova S, Parkkila S, Parkkila AK, et al. Carbonic anhydrase IX, MN/CA IX: analysis of stomach complementary DNA sequence and expression in human and rat alimentary tracts. Gastroenterology 1997;112:398–408
- Gieling RG, Parker CA, De Costa LA, et al. Inhibition of carbonic anhydrase activity modifies the toxicity of doxorubicin and melphalan in tumour cells in vitro. J Enzym Inhib Med Chem 2013;28:360–9
- Potter CP, Harris AL. Diagnostic, prognostic and therapeutic implications of carbonic anhydrases in cancer. Br J Cancer 2003;89:2–7
- Neri D, Supuran CT. Interfering with pH regulation in tumours as a therapeutic strategy. Nat Rev Drug Discov 2011;10:767–77
- Svastova E, Hulikova A, Rafajova M, et al. Hypoxia activates the capacity of tumor-associated carbonic anhydrase IX to acidify extracellular pH. FEBS Lett 2004;577:439–45
- Dubois L, Douma K, Supuran CT, et al. Imaging the hypoxia surrogate marker CA IX requires expression and catalytic activity for binding fluorescent sulfonamide inhibitors. Radiother Oncol: journal of the European Society for Therapeutic Radiology and Oncology 2007;83:367–73
- Dubois L, Lieuwes NG, Maresca A, et al. Imaging of CA IX with fluorescent labelled sulfonamides distinguishes hypoxic and (re)-oxygenated cells in a xenograft tumour model. Radiother Oncol 2009;92:423–8
- Ahlskog JK, Dumelin CE, Trussel S, et al. In vivo targeting of tumor-associated carbonic anhydrases using acetazolamide derivatives. Bioorg Med Chem Lett 2009;19:4851–6
- Buller F, Steiner M, Frey K, et al. Selection of carbonic anhydrase IX inhibitors from one million DNA-encoded compounds. ACS Chem Biol 2011;6:336–44
- Gieling RG, Babur M, Mamnani L, et al. Antimetastatic effect of sulfamate carbonic anhydrase IX inhibitors in breast carcinoma xenografts. J Med Chem 2012;55:5591–600
- Rami M, Dubois L, Parvathaneni NK, et al. Hypoxia-targeting carbonic anhydrase IX inhibitors by a new series of nitroimidazole-sulfonamides/sulfamides/sulfamates. J Med Chem 2013;56:8512–20
- Dubois L, Peeters SG, van Kuijk SJ, et al. Targeting carbonic anhydrase IX by nitroimidazole based sulfamides enhances the therapeutic effect of tumor irradiation: a new concept of dual targeting drugs. Radiother Oncol 2013;108:523–8
- Overgaard J, Hansen HS, Overgaard M, et al. A randomized double-blind phase III study of nimorazole as a hypoxic radiosensitizer of primary radiotherapy in supraglottic larynx and pharynx carcinoma. Results of the Danish Head and Neck Cancer Study (DAHANCA) Protocol 5-85. Radiother Oncol 1998;46:135–46
- Casey JR, Grinstein S, Orlowski J. Sensors and regulators of intracellular pH. Nat Rev Mol Cell Biol 2010;11:50–61
- Parks SK, Chiche J, Pouyssegur J. Disrupting proton dynamics and energy metabolism for cancer therapy. Nat Rev Cancer 2013;13:611–23
- Chiche J, Ilc K, Laferriere J, et al. Hypoxia-inducible carbonic anhydrase IX and XII promote tumor cell growth by counteracting acidosis through the regulation of the intracellular pH. Cancer Res 2009;69:358–68
- Swietach P, Hulikova A, Vaughan-Jones RD, Harris AL. New insights into the physiological role of carbonic anhydrase IX in tumour pH regulation. Oncogene 2010;29:6509–21
- Cummins EP, Selfridge AC, Sporn PH, et al. Carbon dioxide-sensing in organisms and its implications for human disease. Cell Mol Life Sci 2014;71:831–45
- Doyen J, Parks SK, Marcie S, et al. Knock-down of hypoxia-induced carbonic anhydrases IX and XII radiosensitizes tumor cells by increasing intracellular acidosis. Frontier Oncol 2012;2:199. doi: 10.3389/fonc.2012.00199. eCollection 2012
- Doherty JR, Yang C, Scott KE, et al. Blocking lactate export by inhibiting the Myc target MCT1 disables glycolysis and glutathione synthesis. Cancer Res 2014;74:908–20
- Le Floch R, Chiche J, Marchiq I, et al. CD147 subunit of lactate/H + symporters MCT1 and hypoxia-inducible MCT4 is critical for energetics and growth of glycolytic tumors. Proc Natl Acad Sci USA 2011;108:16663–8
- Murray CM, Hutchinson R, Bantick JR, et al. Monocarboxylate transporter MCT1 is a target for immunosuppression. Nat Chem Biol 2005;1:371–6
- Polanski R, Hodgkinson CL, Fusi A, et al. Activity of the monocarboxylate transporter 1 inhibitor AZD3965 in small cell lung cancer. Clin Cancer Res 2014;20:926–37
- Chiche J, Le Fur Y, Vilmen C, et al. In vivo pH in metabolic-defective Ras-transformed fibroblast tumors: key role of the monocarboxylate transporter, MCT4, for inducing an alkaline intracellular pH. Int J Cancer 2012;130:1511–20
- Lutz NW, Le Fur Y, Chiche J, et al. Quantitative in vivo characterization of intracellular and extracellular pH profiles in heterogeneous tumors: a novel method enabling multiparametric pH analysis. Cancer Res 2013;73:4616–28
- Halestrap AP. Monocarboxylic acid transport. Compreh Physiol 2013;3:1611–43
- Gottfried E, Kunz-Schughart LA, Ebner S, et al. Tumor-derived lactic acid modulates dendritic cell activation and antigen expression. Blood 2006;107:2013–21
- Biswas S, Aggarwal M, Guzel O, et al. Conformational variability of different sulfonamide inhibitors with thienyl-acetamido moieties attributes to differential binding in the active site of cytosolic human carbonic anhydrase isoforms. Bioorg Med Chem 2011;19:3732–8
- Scozzafava A, Supuran CT. Carbonic anhydrase inhibitors. Arylsulfonylureido- and arylureido-substituted aromatic and heterocyclic sulfonamides: towards selective inhibitors of carbonic anhydrase isozyme I. J Enzym Inhib 1999;14:343–63
- Carta F, Garaj V, Maresca A, et al. Sulfonamides incorporating 1,3,5-triazine moieties selectively and potently inhibit carbonic anhydrase transmembrane isoforms IX, XII and XIV over cytosolic isoforms I and II: solution and X-ray crystallographic studies. Bioorg Med Chem 2011;19:3105–19
- Garaj V, Puccetti L, Fasolis G, et al. Carbonic anhydrase inhibitors: synthesis and inhibition of cytosolic/tumor-associated carbonic anhydrase isozymes I, II, and IX with sulfonamides incorporating 1,2,4-triazine moieties. Bioorg Med Chem Lett 2004;14:5427–33
- Garaj V, Puccetti L, Fasolis G, et al. Carbonic anhydrase inhibitors: novel sulfonamides incorporating 1,3,5-triazine moieties as inhibitors of the cytosolic and tumour-associated carbonic anhydrase isozymes I, II and IX. Bioorg Med Chem Lett 2005;15:3102–8
- Pacchiano F, Carta F, McDonald PC, et al. Ureido-substituted benzenesulfonamides potently inhibit carbonic anhydrase IX and show antimetastatic activity in a model of breast cancer metastasis. J Med Chem 2011;54:1896–902
- Pacchiano F, Aggarwal M, Avvaru BS, et al. Selective hydrophobic pocket binding observed within the carbonic anhydrase II active site accommodate different 4-substituted-ureido-benzenesulfonamides and correlate to inhibitor potency. Chem Commun (Camb) 2010;46:8371–3
- McDonald PC, Winum JY, Supuran CT, Dedhar S. Recent developments in targeting carbonic anhydrase IX for cancer therapeutics. Oncotarget 2012;3:84–97
- Carta F, Aggarwal M, Maresca A, et al. Dithiocarbamates: a new class of carbonic anhydrase inhibitors. Crystallographic and kinetic investigations. Chem Commun (Camb) 2012;48:1868–70
- Carta F, Aggarwal M, Maresca A, et al. Dithiocarbamates strongly inhibit carbonic anhydrases and show antiglaucoma action in vivo. J Med Chem 2012;55:1721–30
- Maresca A, Carta F, Vullo D, Supuran CT. Dithiocarbamates strongly inhibit the beta-class carbonic anhydrases from Mycobacterium tuberculosis. J Enzym Inhib Med Chem 2013;28:407–11
- Monti SM, Maresca A, Viparelli F, et al. Dithiocarbamates are strong inhibitors of the beta-class fungal carbonic anhydrases from Cryptococcus neoformans, Candida albicans and Candida glabrata. Bioorg Med Chem Lett 2012;22:859–62
- Kramer N, Walzl A, Unger C, et al. In vitro cell migration and invasion assays. Mutat Res 2013;752:10–24
- Francia G, Cruz-Munoz W, Man S, et al. Mouse models of advanced spontaneous metastasis for experimental therapeutics. Nat Rev Cancer 2011;11:135–41
- Cawthorne C, Burrows N, Gieling RG, et al. [18F]-FLT positron emission tomography can be used to image the response of sensitive tumors to PI3-kinase inhibition with the novel agent GDC-0941. Mol Cancer Ther 2013;12:819–28
- Iorns E, Drews-Elger K, Ward TM, et al. A new mouse model for the study of human breast cancer metastasis. PLoS One 2012;7:e47995
- Parkkila S, Rajaniemi H, Parkkila AK, et al. Carbonic anhydrase inhibitor suppresses invasion of renal cancer cells in vitro. Proc Natl Acad Sci USA 2000;97:2220–4
- Gieling RG, Williams KJ. Carbonic anhydrase IX as a target for metastatic disease. Bioorg Med Chem 2013;21:1470–6
- Winum JY, Carta F, Ward C, et al. Ureido-substituted sulfamates show potent carbonic anhydrase IX inhibitory and antiproliferative activities against breast cancer cell lines. Bioorg Med Chem Lett 2012;22:4681–5
- Rademakers SE, Span PN, Kaanders JH, et al. Molecular aspects of tumour hypoxia. Mol Oncol 2008;2:41–53
- Burrows N, Resch J, Cowen RL, et al. Expression of hypoxia-inducible factor 1 alpha in thyroid carcinomas. Endocrine-Relat Cancer 2010;17:61–72
- Lee SL, Rouhi P, Dahl Jensen L, et al. Hypoxia-induced pathological angiogenesis mediates tumor cell dissemination, invasion, and metastasis in a zebrafish tumor model. Proc Natl Acad Sci USA 2009;106:19485–90
- Rouhi P, Jensen LD, Cao Z, et al. Hypoxia-induced metastasis model in embryonic zebrafish. Nat Protocol 2010;5:1911–18
- Cao R, Ji H, Feng N, et al. Collaborative interplay between FGF-2 and VEGF-C promotes lymphangiogenesis and metastasis. Proc Natl Acad Sci USA 2012;109:15894–9
- Cao R, Bjorndahl MA, Religa P, et al. PDGF-BB induces intratumoral lymphangiogenesis and promotes lymphatic metastasis. Cancer Cell 2004;6:333–45
- Cao R, Lim S, Ji H, et al. Mouse corneal lymphangiogenesis model. Nat Protocol 2011;6:817–26
- Zamboni WC, Torchilin V, Patri AK, et al. Best practices in cancer nanotechnology: perspective from NCI nanotechnology alliance. Clin Cancer Res 2012;18:3229–41
- Petsko GA. When failure should be the option. BMC Biol 2010;8:61
- Meehan J WC, Supuran C, Langdon SP, Kunkler IH. The effects of novel carbonic anhydrase inhibitors on the proliferation and invasion of breast and ovarian cancer cells. J Pathol 2013;231:36–45
- Huang M, Shen A, Ding J, Geng M. Molecularly targeted cancer therapy: some lessons from the past decade. Trends Pharmacol Sci 2014;35:41–50
- Daniel VC, Marchionni L, Hierman JS, et al. A primary xenograft model of small-cell lung cancer reveals irreversible changes in gene expression imposed by culture in vitro. Cancer Res 2009;69:3364–73
- Langdon SP. Isolation and culture of ovarian cancer cell lines. Method Mol Med 2004;88:133–9
- Navin N, Kendall J, Troge J, et al. Tumour evolution inferred by single-cell sequencing. Nature 2011;472:90–4
- Tannock IF, Rotin D. Acid pH in tumors and its potential for therapeutic exploitation. Cancer Res 1989;49:4373–84
- Harrison DK, Vaupel P. Heterogeneity in tissue oxygenation: from physiological variability in normal tissues to pathophysiological chaos in malignant tumours. Adv Exp Med Biol 2014;812:25–31
- Debnath J, Brugge JS. Modelling glandular epithelial cancers in three-dimensional cultures. Nat Rev Cancer 2005;5:675–88
- Weigelt B, Bissell MJ. Unraveling the microenvironmental influences on the normal mammary gland and breast cancer. Semin Cancer Biol 2008;18:311–21
- Gillet JP, Calcagno AM, Varma S, et al. Redefining the relevance of established cancer cell lines to the study of mechanisms of clinical anti-cancer drug resistance. Proc Natl Acad Sci USA 2011;108:18708–13
- Mehta JP, O'Driscoll L, Barron N, et al. A microarray approach to translational medicine in breast cancer: how representative are cell line models of clinical conditions? Anticancer Res 2007;27:1295–300
- dit Faute MA, Laurent L, Ploton D, et al. Distinctive alterations of invasiveness, drug resistance and cell-cell organization in 3D-cultures of MCF-7, a human breast cancer cell line, and its multidrug resistant variant. Clin Exp Metastasis 2002;19:161–8
- Yamada KM, Cukierman E. Modeling tissue morphogenesis and cancer in 3D. Cell 2007;130:601–10
- Pampaloni F, Reynaud EG, Stelzer EH. The third dimension bridges the gap between cell culture and live tissue. Nat Rev Mol Biol 2007;8:839–45
- Schmeichel KL, Bissell MJ. Modeling tissue-specific signaling and organ function in three dimensions. J Cell Sci 2003;116:2377–88
- Kumar HR, Zhong X, Hoelz DJ, et al. Three-dimensional neuroblastoma cell culture: proteomic analysis between monolayer and multicellular tumor spheroids. Pediatr Surg Int 2008;24:1229–34
- Griffith LG, Swartz MA. Capturing complex 3D tissue physiology in vitro. Nat Rev Mol Cell Biol 2006;7:211–24
- Hirschhaeuser F, Menne H, Dittfeld C, et al. Multicellular tumor spheroids: an underestimated tool is catching up again. J Biotechnol 2010;148:3–15
- Lin RZ, Chang HY. Recent advances in three-dimensional multicellular spheroid culture for biomedical research. Biotechnol J 2008;3:1172–84
- Provenzano PP, Inman DR, Eliceiri KW, et al. Collagen density promotes mammary tumor initiation and progression. BMC Med 2008;6:11
- Ramaswamy S, Ross KN, Lander ES, Golub TR. A molecular signature of metastasis in primary solid tumors. Nat Genet 2003;33:49–54
- Friedrich J, Ebner R, Kunz-Schughart LA. Experimental anti-tumor therapy in 3-D: spheroids--old hat or new challenge? Int J Radiat Biol 2007;83:849–71
- Minchinton AI, Tannock IF. Drug penetration in solid tumours. Nat Rev Cancer 2006;6:583–92
- Swietach P, Patiar S, Supuran CT, et al. The role of carbonic anhydrase 9 in regulating extracellular and intracellular ph in three-dimensional tumor cell growths. J Biol Chem 2009;284:20299–310
- McIntyre A, Patiar S, Wigfield S, et al. Carbonic anhydrase IX promotes tumor growth and necrosis in vivo and inhibition enhances anti-VEGF therapy. Clin Cancer Res 2012;18:3100–11
- Kamb A. What's wrong with our cancer models? Nat Rev Drug Discov 2005;4:161–5
- Harrington KJ, Billingham LJ, Brunner TB, et al. Guidelines for preclinical and early phase clinical assessment of novel radiosensitisers. Br J Cancer 2011;105:628–39
- Vargo-Gogola T, Rosen JM. Modelling breast cancer: one size does not fit all. Nat Rev Cancer 2007;7:659–72
- Dean JL, McClendon AK, Hickey TE, et al. Therapeutic response to CDK4/6 inhibition in breast cancer defined by ex vivo analyses of human tumors. Cell Cycle (Georgetown, Tex) 2012;11:2756–61
- Sausville EA, Burger AM. Contributions of human tumor xenografts to anticancer drug development. Cancer Res 2006;66:3351–4, discussion 4
- Boedigheimer MJ, Freeman DJ, Kiaei P, et al. Gene expression profiles can predict panitumumab monotherapy responsiveness in human tumor xenograft models. Neoplasia (New York) 2013;15:125–32
- Politi K, Pao W. How genetically engineered mouse tumor models provide insights into human cancers. J Clin Oncol 2011;29:2273–81
- Sharpless NE, Depinho RA. The mighty mouse: genetically engineered mouse models in cancer drug development. Nat Rev Drug Discov 2006;5:741–54
- Olive KP, Jacobetz MA, Davidson CJ, et al. Inhibition of Hedgehog signaling enhances delivery of chemotherapy in a mouse model of pancreatic cancer. Science (New York) 2009;324:1457–61
- Graves EE, Vilalta M, Cecic IK, et al. Hypoxia in models of lung cancer: implications for targeted therapeutics. Clin Cancer Res 2010;16:4843–52
- Maity A, Koumenis C. Location, location, location-makes all the difference for hypoxia in lung tumors. Clin Cancer Res 2010;16:4685–7
- Singh M, Lima A, Molina R, et al. Assessing therapeutic responses in Kras mutant cancers using genetically engineered mouse models. Nat Biotech 2010;28:585–93
- Chen Z, Cheng K, Walton Z, et al. A murine lung cancer co-clinical trial identifies genetic modifiers of therapeutic response. Nature 2012;483:613–17
- Moding EJ, Kastan MB, Kirsch DG. Strategies for optimizing the response of cancer and normal tissues to radiation. Nat Rev Drug Discov 2013;12:526–42
- Fu L, Wang G, Shevchuk MM, et al. Generation of a mouse model of Von Hippel-Lindau kidney disease leading to renal cancers by expression of a constitutively active mutant of HIF1alpha. Cancer Res 2011;71:6848–56
- Morgan MA, Parsels LA, Zhao L, et al. Mechanism of radiosensitization by the Chk1/2 inhibitor AZD7762 involves abrogation of the G2 checkpoint and inhibition of homologous recombinational DNA repair. Cancer Res 2010;70:4972–81
- DeRose YS, Wang G, Lin YC, et al. Tumor grafts derived from women with breast cancer authentically reflect tumor pathology, growth, metastasis and disease outcomes. Nat Med 2011;17:1514–20
- Whiteford CC, Bilke S, Greer BT, et al. Credentialing preclinical pediatric xenograft models using gene expression and tissue microarray analysis. Cancer Res 2007;67:32–40
- Zhao X, Liu Z, Yu L, et al. Global gene expression profiling confirms the molecular fidelity of primary tumor-based orthotopic xenograft mouse models of medulloblastoma. Neuro-Oncology 2012;14:574–83
- Reyal F, Guyader C, Decraene C, et al. Molecular profiling of patient-derived breast cancer xenografts. Breast Cancer Res 2012;14:R11
- Ding L, Ellis MJ, Li S, et al. Genome remodelling in a basal-like breast cancer metastasis and xenograft. Nature 2010;464:999–1005
- Siolas D, Hannon GJ. Patient-derived tumor xenografts: transforming clinical samples into mouse models. Cancer Res 2013;73:5315–19
- Katz E, Sims AH, Sproul D, et al. Targeting of Rac GTPases blocks the spread of intact human breast cancer. Oncotarget 2012;3:608–19
- Ward C MJ, Harrison DJ, Kunkler IH, Langdon SP. An ex-vivo tumour model using core biopsy explants validate carbonic anhydrase IX (CAIX) as a therapeutic target in breast cancer. J Pathol 2013;231:24–30
- van der Kuip H, Murdter TE, Sonnenberg M, et al. Short term culture of breast cancer tissues to study the activity of the anticancer drug taxol in an intact tumor environment. BMC Cancer 2006;6:86
- Steeg PS. Tumor metastasis: mechanistic insights and clinical challenges. Nat Med 2006;12:895–904
- MacDonald IC, Groom AC, Chambers AF. Cancer spread and micrometastasis development: quantitative approaches for in vivo models. BioEssays 2002;24:885–93
- Tentler JJ, Tan AC, Weekes CD, et al. Patient-derived tumour xenografts as models for oncology drug development. Nat Rev Clin Oncol 2012;9:338–50
- Singh M, Ferrara N. Modeling and predicting clinical efficacy for drugs targeting the tumor milieu. Nat Biotechnol 2012;30:648–57
- Nardella C, Lunardi A, Patnaik A, et al. The APL paradigm and the “co-clinical trial” project. Cancer Discov 2011;1:108–16
- Lambin P, Petit SF, Aerts HJ, et al. The ESTRO Breur Lecture 2009. From population to voxel-based radiotherapy: exploiting intra-tumour and intra-organ heterogeneity for advanced treatment of non-small cell lung cancer. Radiother Oncol 2010;96:145–52
- Thorwarth D, Eschmann SM, Paulsen F, Alber M. Hypoxia dose painting by numbers: a planning study. Int J Radiat Oncol Biol Phys 2007;68:291–300
- Bollineni VR, Wiegman EM, Pruim J, et al. Hypoxia imaging using positron emission tomography in non-small cell lung cancer: implications for radiotherapy. Cancer Treat Rev 2012;38:1027–32
- Mortensen LS, Johansen J, Kallehauge J, et al. FAZA PET/CT hypoxia imaging in patients with squamous cell carcinoma of the head and neck treated with radiotherapy: results from the DAHANCA 24 trial. Radiother Oncol 2012;105:14–20
- Busk M, Jakobsen S, Horsman MR, et al. PET imaging of tumor hypoxia using 18F-labeled pimonidazole. Acta Oncol (Stockholm, Sweden) 2013;52:1300–7
- Dubois LJ, Lieuwes NG, Janssen MH, et al. Preclinical evaluation and validation of [18F]HX4, a promising hypoxia marker for PET imaging. Proc Natl Acad Sci USA 2011;108:14620–5
- van Loon J, Janssen MH, Ollers M, et al. PET imaging of hypoxia using [18F]HX4: a phase I trial. Eur J Nucl Med Mol Imag 2010;37:1663–8
- Doss M, Zhang JJ, Belanger MJ, et al. Biodistribution and radiation dosimetry of the hypoxia marker 18F-HX4 in monkeys and humans determined by using whole-body PET/CT. Nucl Med Commun 2010;31:1016–24
- Zegers CM, van Elmpt W, Wierts R, et al. Hypoxia imaging with [(1)(8)F]HX4 PET in NSCLC patients: defining optimal imaging parameters. Radiother Oncol 2013;109:58–64
- Horsman MR, Mortensen LS, Petersen JB, Busk M, Overgaard J. Imaging hypoxia to improve radiotherapy outcome. Nat Rev Clin Oncol 2012;9:674–87
- Betts GN, Eustace A, Patiar S, et al. Prospective technical validation and assessment of intra-tumour heterogeneity of a low density array hypoxia gene profile in head and neck squamous cell carcinoma. Eur J Cancer 2013;49:156–65
- Winter SC, Buffa FM, Silva P, et al. Relation of a hypoxia metagene derived from head and neck cancer to prognosis of multiple cancers. Cancer Res 2007;67:3441–9
- Buffa FM, Harris AL, West CM, Miller CJ. Large meta-analysis of multiple cancers reveals a common, compact and highly prognostic hypoxia metagene. Br J Cancer 2010;102:428–35
- Curtis C, Shah SP, Chin SF, et al. The genomic and transcriptomic architecture of 2,000 breast tumours reveals novel subgroups. Nature 2012;486:346–52
- TCGA. Comprehensive molecular portraits of human breast tumours. Nature 2012;490:61–70
- Jubb AM, Buffa FM, Harris AL. Assessment of tumour hypoxia for prediction of response to therapy and cancer prognosis. J Cell Mol Med 2010;14:18–29
- Eustace A, Mani N, Span PN, et al. A 26-gene hypoxia signature predicts benefit from hypoxia-modifying therapy in laryngeal cancer but not bladder cancer. Clin Cancer Res 2013;19:4879–88
- Ghazoui Z, Buffa FM, Dunbier AK, et al. Close and stable relationship between proliferation and a hypoxia metagene in aromatase inhibitor-treated ER-positive breast cancer. Clin Cancer Res 2011;17:3005–12
- Camps C, Buffa FM, Colella S, et al. hsa-miR-210 Is induced by hypoxia and is an independent prognostic factor in breast cancer. Clin Cancer Res 2008;14:1340–8
- McCormick RI, Blick C, Ragoussis J, et al. miR-210 is a target of hypoxia-inducible factors 1 and 2 in renal cancer, regulates ISCU and correlates with good prognosis. Br J Cancer 2013;108:1133–42
- Chan SY, Zhang YY, Hemann C, et al. MicroRNA-210 controls mitochondrial metabolism during hypoxia by repressing the iron-sulfur cluster assembly proteins ISCU1/2. Cell Metabol 2009;10:273–84
- Favaro E, Ramachandran A, McCormick R, et al. MicroRNA-210 regulates mitochondrial free radical response to hypoxia and krebs cycle in cancer cells by targeting iron sulfur cluster protein ISCU. PLoS One 2010;5:e10345
- Buffa FM, Camps C, Winchester L, et al. microRNA-associated progression pathways and potential therapeutic targets identified by integrated mRNA and microRNA expression profiling in breast cancer. Cancer Res 2011;71:5635–45
- Rothe F, Ignatiadis M, Chaboteaux C, et al. Global microRNA expression profiling identifies MiR-210 associated with tumor proliferation, invasion and poor clinical outcome in breast cancer. PLoS One 2011;6:e20980
- Halle C, Andersen E, Lando M, et al. Hypoxia-induced gene expression in chemoradioresistant cervical cancer revealed by dynamic contrast-enhanced MRI. Cancer Res 2012;72:5285–95
- Milosevic M, Warde P, Menard C, et al. Tumor hypoxia predicts biochemical failure following radiotherapy for clinically localized prostate cancer. Clin Cancer Res 2012;18:2108–14
- Ragnum HB, Roe K, Holm R, et al. Hypoxia-independent downregulation of hypoxia-inducible factor 1 targets by androgen deprivation therapy in prostate cancer. Int J Radiat Oncol Biol Phys 2013;87:753–60
- Mabjeesh NJ, Willard MT, Frederickson CE, et al. Androgens stimulate hypoxia-inducible factor 1 activation via autocrine loop of tyrosine kinase receptor/phosphatidylinositol 3′-kinase/protein kinase B in prostate cancer cells. Clin Cancer Res 2003;9:2416–25
- Horii K, Suzuki Y, Kondo Y, et al. Androgen-dependent gene expression of prostate-specific antigen is enhanced synergistically by hypoxia in human prostate cancer cells. Mol Cancer Res 2007;5:383–91
- Overgaard J, Eriksen JG, Nordsmark M, Group DHaNC. Plasma osteopontin, hypoxia, and response to the hypoxia sensitiser nimorazole in radiotherapy of head and neck cancer: results from the DAHANCA 5 randomised double-blind placebo-controlled trial. Lancet Oncol 2005;6:757–64
- Rischin D, Hicks RJ, Fisher R, et al. Prognostic significance of [18F]-misonidazole positron emission tomography-detected tumor hypoxia in patients with advanced head and neck cancer randomly assigned to chemoradiation with or without tirapazamine: a substudy of Trans-Tasman Radiation Oncology Group Study 98.02. J Clin Oncol 2006;24:2098–104
- Toustrup K, Sorensen BS, Nordsmark M, et al. Development of a hypoxia gene expression classifier with predictive impact for hypoxic modification of radiotherapy in head and neck cancer. Cancer Res 2011;71:5923–31
- Toustrup K, Sorensen BS, Lassen P, et al. Gene expression classifier predicts for hypoxic modification of radiotherapy with nimorazole in squamous cell carcinomas of the head and neck. Radiother Oncol 2012;102:122–9
- Sorensen BS, Busk M, Olthof N, et al. Radiosensitivity and effect of hypoxia in HPV positive head and neck cancer cells. Radiother Oncol 2013;108:500–5
- Winther M, Alsner J, Tramm T, Nordsmark M. Hypoxia-regulated gene expression and prognosis in loco-regional gastroesophageal cancer. Acta Oncol (Stockholm, Sweden) 2013;52:1327–35
- Bertout JA, Patel SA, Simon MC. The impact of O2 availability on human cancer. Nat Rev Cancer 2008;8:967–75
- Casazza A, Di Conza G, Wenes M, et al. Tumor stroma: a complexity dictated by the hypoxic tumor microenvironment. Oncogene 2014;33:1743–54
- Martini M, Vecchione L, Siena S, et al. Targeted therapies: how personal should we go? Nat Rev Clin Oncol 2012;9:87–97
- Folkvord S, Flatmark K, Dueland S, et al. Prediction of response to preoperative chemoradiotherapy in rectal cancer by multiplex kinase activity profiling. Int J Radiat Oncol Biol Phys 2010;78:555–62
- Saelen MG, Flatmark K, Folkvord S, et al. Tumor kinase activity in locally advanced rectal cancer: angiogenic signaling and early systemic dissemination. Angiogenesis 2011;14:481–9
- Ree AH, Kristensen AT, Saelen MG, et al. Tumor phosphatidylinositol-3-kinase signaling and development of metastatic disease in locally advanced rectal cancer. PLoS One 2012;7:e50806
- Roe K, Bratland A, Vlatkovic L, et al. Hypoxic tumor kinase signaling mediated by STAT5A in development of castration-resistant prostate cancer. PLoS One 2013;8:e63723
- Tahiri A, Roe K, Ree AH, et al. Differential inhibition of ex-vivo tumor kinase activity by vemurafenib in BRAF(V600E) and BRAF wild-type metastatic malignant melanoma. PLoS One 2013;8:e72692
- Ramachandran A, Betts G, Bhana S, et al. An in vivo hypoxia metagene identifies the novel hypoxia inducible factor target gene SLCO1B3. Eur J Cancer 2013;49:1741–51
- van Baardwijk A, Tome WA, van Elmpt W, et al. Is high-dose stereotactic body radiotherapy (SBRT) for stage I non-small cell lung cancer (NSCLC) overkill? A systematic review. Radiother Oncol 2012;105:145–9
- Ashworth A, Rodrigues G, Boldt G, Palma D. Is there an oligometastatic state in non-small cell lung cancer? A systematic review of the literature. Lung Cancer (Amsterdam, Netherlands) 2013;82:197–203
- Cheruvu P, Metcalfe SK, Metcalfe J, et al. Comparison of outcomes in patients with stage III versus limited stage IV non-small cell lung cancer. Radiat Oncol (London, England) 2011;6:80. doi:10.1186/1748-717X-6-80
- Dahele M, Senan S. The role of stereotactic ablative radiotherapy for early-stage and oligometastatic non-small cell lung cancer: evidence for changing paradigms. Cancer Res Treat 2011;43:75–82
- Hasselle MD, Haraf DJ, Rusthoven KE, et al. Hypofractionated image-guided radiation therapy for patients with limited volume metastatic non-small cell lung cancer. J Thorac Oncol 2012;7:376–81
- Higginson DS, Chen RC, Tracton G, et al. The impact of local and regional disease extent on overall survival in patients with advanced stage IIIB/IV non-small cell lung carcinoma. Int J Radiat Oncol Biol Phys 2012;84:e385–92
- Marks LB, Saynak M, Christodouleas JP. Stage III vs. stage IV lung cancer: “crossing a great divide”. Lung Cancer (Amsterdam, Netherlands) 2010;67:1–3
- Milano MT, Katz AW, Zhang H, Okunieff P. Oligometastases treated with stereotactic body radiotherapy: long-term follow-up of prospective study. Int J Radiat Oncol Biol Phys 2012;83:878–86
- Tree AC, Khoo VS, Eeles RA, et al. Stereotactic body radiotherapy for oligometastases. Lancet Oncol 2013;14:e28–37
- De Ruysscher D, Wanders R, van Baardwijk A, et al. Radical treatment of non-small-cell lung cancer patients with synchronous oligometastases: long-term results of a prospective phase II trial (Nct01282450). J Thorac Oncol 2012;7:1547–55
- De Bock K, Mazzone M, Carmeliet P. Antiangiogenic therapy, hypoxia, and metastasis: risky liaisons, or not? Nat Rev Clin Oncol 2011;8:393–404
- Weis SM, Cheresh DA. Tumor angiogenesis: molecular pathways and therapeutic targets. Nat Med 2011;17:1359–70
- Cooke VG, LeBleu VS, Keskin D, et al. Pericyte depletion results in hypoxia-associated epithelial-to-mesenchymal transition and metastasis mediated by met signaling pathway. Cancer Cell 2012;21:66–81
- Petersen SH, Harling H, Kirkeby LT, et al. Postoperative adjuvant chemotherapy in rectal cancer operated for cure. Cochrane Database Syst Rev 2012;3:CD004078
- Bosset JF, Calais G, Mineur L, et al. Fluorouracil-based adjuvant chemotherapy after preoperative chemoradiotherapy in rectal cancer: long-term results of the EORTC 22921 randomised study. Lancet Oncol 2014;15:184–90
- Fass L. Imaging and cancer: a review. Mol Oncol 2008;2:115–52
- Orloff J, Douglas F, Pinheiro J, et al. The future of drug development: advancing clinical trial design. Nat Rev Drug Discov 2009;8:949–57
- Vogelzang NJ, Benowitz SI, Adams S, et al. Clinical cancer advances 2011: Annual report on progress against cancer from the American society of clinical oncology. J Clin Oncol 2012;30:88–109
- Lambin P, Roelofs E, Reymen B, et al. ‘Rapid Learning health care in oncology’ – an approach towards decision support systems enabling customised radiotherapy'. Radiother Oncol 2013;109:159–64
- Maitland ML, Schilsky RL. Clinical trials in the era of personalized oncology. Cancer J Clin 2011;61:365–81
- Gerlinger M, Rowan AJ, Horswell S, et al. Intratumor heterogeneity and branched evolution revealed by multiregion sequencing. New Engl J Med 2012;366:883–92
- Bachtiary B, Boutros PC, Pintilie M, et al. Gene expression profiling in cervical cancer: an exploration of intratumor heterogeneity. Clin Cancer Res 2006;12:5632–40
- Boyd CA, Benarroch-Gampel J, Sheffield KM, et al. 415 patients with adenosquamous carcinoma of the pancreas: a population-based analysis of prognosis and survival. J Surg Res 2012;174:12–9
- Milosevic MF, Fyles AW, Wong R, et al. Interstitial fluid pressure in cervical carcinoma: within tumor heterogeneity, and relation to oxygen tension. Cancer 1998;82:2418–26
- Lambin P, van Stiphout RG, Starmans MH, et al. Predicting outcomes in radiation oncology – multifactorial decision support systems. Nat Rev Clin Oncol 2013;10:27–40
- (a) Supuran CT. Inhibition of carbonic anhydrase IX as a novel anticancer mechanism. World J Clin Oncol 2012;3:98–103. (b) Supuran CT. Structure-based drug discovery of carbonic anhydrase inhibitors. J Enzyme Inhib Med Chem 2012;27;759–72
- Supuran CT. Carbonic anhydrases: from biomedical applications of the inhibitors and activators to biotechnological use for CO(2) capture. J Enzyme Inhib Med Chem 2013;28;229–32