Abstract
Ellman’s method is a standard protocol for the determination of cholinesterases activity. Though the method is ready for laboratory purposes, it has some drawbacks as well. In the current article, 2,6-dichloroindophenol acetate is performed as a chromogenic substrate suitable for acetylcholinesterase (AChE) activity examination. Michaelis constant and maximal velocity for 2,6-dichloroindophenol acetate were determined (38.0 µM and 244 pkat) and compared to the values for acetythiocholine (Km 0.18 mM; Vmax 5.1 nkat). Docking for 2,6-dichloroindophenol acetate and human AChE was done as well. In conclusion, 2,6-dichloroindophenol acetate seems to be suitable chromogenic substrate for AChE and spectrophotometry and based on this it can be easily performed whenever AChE activity should be tested.
Introduction
Acetylcholinesterase (AChE; EC 3.1.1.7) is an important enzyme responsible for termination of cholinergic neurotransmission. The enzyme is an object of interest in pharmacology research because inhibitors of AChE have wide use as drugs for, e.g. Alzheimer disease, myasthenia gravis and some other purposesCitation1. In addition to the pharmacological applications, AChE can be targeted by many harmful substances such as nerve agents in chemical warfare and pesticides such as carbofuranCitation2. Besides AChE, the second cholinesterase, butyrylcholinesterase (BChE; EC 3.1.1.8), can be found in the body. Physiological relevance of BChE is, however, lower compared to AChE and it is not unsubstitutable in regulatory processes as wellCitation3–5.
Activity of AChE is frequently measured because of new drugs testing or in an assay of neurotoxic compounds in, for example, food or environmentCitation6,Citation7. When activity of AChE assessed, Ellman’s method is typically preferred as a standard protocol. The method was discovered by Ellman et al. and used without particular changes up todayCitation8,Citation9. The method consists of two steps. In the first step, acetylthiocholine (or butyrylthiocholine when BChE assayed) is split by AChE into thiocholine and acetic acid. In the next step, thiocholine reacts with 5,5′-dithiobis(2-nitrobenzoic acid) providing yellow colored 5-thio-2-nitrobenzoic acid. The method has some drawbacks such as low stability of 5,5′-dithiobis(2-nitrobenzoic acid) and interference of hemoglobinCitation10, thiol moiety containing compounds such as cysteine, reduced glutathioneCitation11 or oximes such as obidoxime, asoximeCitation12. Because of the aforementioned disadvantages of the assay, there is a demand for a new, more reliable, assay suitable for a routine determination of cholinesterases activityCitation12,Citation13.
The current article is devoted to setup of a new protocol suitable for AChE activity measurement. Here, a new substrate, 2,6-dichlorophenolindophenol acetate, is used as a chromogenic reagent. It is hypothesized that the new substrate would be an alternative to the standard Ellman’s method and assay based on the new substrate will not have drawbacks of the Ellman’s method. Pros and cons of the new and standard methods are discussed in the article.
Materials and methods
AChE
In the assay, human recombinant AChE expressed in HEK293 cells was purchased from Sigma Aldrich (Saint Louis, MI). The AChE had activity of 1500 µmol/min per milligram of protein and was received in lyophilized form. AChE was solubilized in phosphate buffered saline (pH 7.4) and activity was adjusted up 0.67 µmol/min/µl (0.40 nkat/µl) for 1 mM acetylthiocholine chloride.
Ellman’s method
A total of 100 µl of either donepezil (Sigma Aldrich) solution or phosphate buffered saline, 200 µl of 1 mM of 5,5′-dithiobis(2-nitrobenzoic acid) in phosphate buffered saline, 100 µl of tested sample (e.g. interfering compound) or phosphate buffered saline, 490 µl of phosphate buffered saline and 10 µl of the aforementioned AChE solution was injected into disposable cuvette with maximal volume 2 ml and gently shaken. Finally, 100 µl of 10 mM acetylthiocholine chloride was given into the cuvette. Absorbance was measured at 412 nm from acetylthiocholine application for 2 min. Absorbance difference was calculated from the time interval. Enzyme activity was calculated using nitrothiobenzoate extinction coefficient ɛ = 14 150 l × mol−1 × cm−1 Citation14,Citation15.
Measurement using 2,6-dichlorophenolindophenol acetate
In the assay, 2,6-dichloroindophenol acetate (Sigma-Aldrich, purity >97%; catalog number of the reagent 36190-10MG-F) is used as a chromogen providing blue 2,6-dichloroindophenolate as a reaction product. In physiological pH 7.4, the blue product absorbs at 606 nm with extinction coefficient ɛ = 18 800 l × mol−1 × cm−1 Citation16. A disposable cuvette was consequently filled with following: 790 µl of phosphate buffered saline, 100 µl of sample solution (tested interfering compound) or phosphate buffered saline and 10 µl of the AChE solution. Finally, 100 µl (10 mM) of 2,6-dichloroindophenol acetate solution in 5% (v/v) ethanol was added; then the absorbance was measured for 60 min. Absorbance change was calculated from the time interval. Principle of the assay is depicted in .
Computational docking
Docking studies were carried out using Autodock Vina (version 1.1.2, The Scripps Research Institute, La Jolla, CA) with default parametersCitation17,Citation18. The crystal structure of AChE was prepared using PDB structure 4YE7 as starting geometry. The ligand (donepezil) and the non-bonding water molecules were removed. Energy minimization was performed (no. of conjugate gradient steps: 100 Gradient: 0.02 RMS kcal/mol/A2) using UCSF Chimera 1.8. Grid box (search space) was set to cover entire AChE cavity. The ligand structures were prepared using PRODRG, the hydrogens were added and energy minimized. All structures receptor and ligand were consequently modified via Autodock Tools 1.5.7rc1 in order to be used by Vina. 2,6-dichloroindophenol acetate was docked using the Lamarckian genetic algorithm. Exhaustiveness of the method was increased from default 8 to 64 in order to find minimum energy pose. Minimum electrostatic potential (41.82 kcal/mol) and maximum electrostatic potential (40.09 kcal/mol) were set up. At the end of calculation, Autodock Vina performed cluster analysis. Root-mean square deviation (RMSD) for best scored pose of donepezil after redocking was 1.05 Å (predicted free binding energy of −12.1 kcal/mol).
Experimental data processing
All measurements were made five times. Origin 8 (OriginLab Corporation, Northampton, MA) software was used for the experimental data processing and plots construction. Calculation of Michaelis constant (Km) and maximal velocity (Vmax) was based on non-linear curve fitting with Hill equation. Limit of detection was calculated from confidence interval (95%) in calibration plot. ANOVA with Bonferroni test was performed in order to judge significance of differences on probability levels p = 0.05 and 0.01. In this article, enzyme activity is further expressed in katal unit: 1 kat = 1 mol/s; 1 pkat = 10−12 mol/s; 1 nkat = 10−9 mol/s.
Results and discussion
The top scored docked posse (predicted free-binding energy of −9.1 kcal/mol) shows the proximity of the ester bond of 2,6-dichloroindophenol acetate to the catalytic residues Ser203 and His447. It could be assumed that such orientation allows the ester bond cleave by AChE. This pose of 2,6-dichloroindophenol acetate within the active site is reached by interactions with hydrogen bonds Ser203 and His447 and ester oxygen (3.5 Å and 3.6 Å, respectively). Another very strong interaction is formed between Tyr133 and carbonyl oxygen in 2,6-dichloroindophenol acetate (2.2 Å). This binding orientation is stabilized by the strong hydrogen bond (2.9 Å) formed between carbonyl oxygen and Tyr124 hydroxyl. Further stabilization is facilitated by iminocyclohexanedienone ring stacking via π–π interactions with Tyr341 (3.4 Å).
The second best scored binding mode should also be taken into consideration. The free-binding energy is slightly lower compared to top scored binding mode (−9.0 kcal/mol). However, the less favorable orientation of ester with respect to catalytic triad residues suggests that cleavage of ester bond in this binding conformation is not plausible. Moreover, the phenyl part of 2,6-dichloroindophenol acetate stacks with Trp86 via π–π interactions similar to donepezil. Such binding mechanism is probably cause of 2,6-dichloroindophenol acetate mild inhibitory properties and may contribute to slower hydrolysis velocity. Localization of 2,6-dichloroindophenol acetate in active site of AChE can be learned from .
Figure 2. Localization of 2,6-dichloroindophenol acetate (top scored pose: violet = right position for uncolored printing; second top scored pose: yellow in the middle of the picture, left from the top scored pose) in active site of AChE.
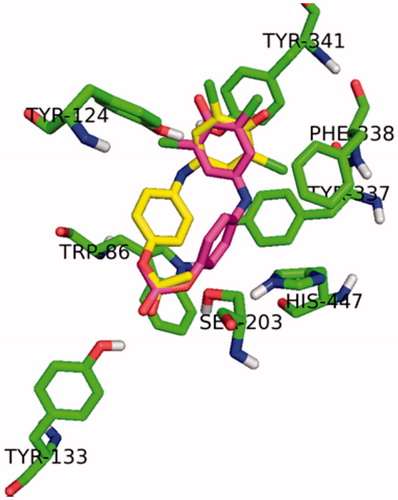
When tested acetylthiocholine as a substrate (), Km = 0.18 mM and Vmax = 5.1 nkat were found. The constant were reached when concentration of acetylthiocholine up to 1 mM taken into consideration. Acetylthiocholine caused inhibition of AChE when presented in excessive concentration. The fact is known from quoted papersCitation19,Citation20. In this experiment with acetylthiocholine, inhibition of AChE by excess of substrate was perceived when it reached concentration 5 mM. The finding is not surprising because it is known from literatureCitation21–23. The Km and Vmax values are in a good correspondence with literature as wellCitation24–26.
Figure 3. Saturation for human AChE and acetylthiocholine as a substrate. Error bars indicate standard deviations for n = 5.
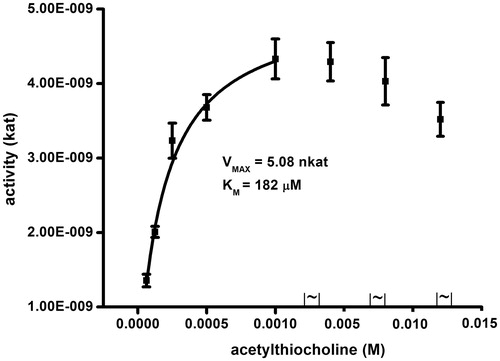
Saturation curve for 2,6-dichlorophenolindophenol acetate is depicted as . 2,6-dichlorophenolindophenol acetate caused inhibition by excess of substrate like the acetylthiocholine do. The inhibition was obvious when 2,6-dichlorophenolindophenol acetate was presented in concentration 0.25 mM or higher. When concentration of 2,6-dichlorophenolindophenol acetate up to 0.13 mM was taken into consideration, Km 38.0 µM and Vmax 244 pkat were found. The found values and the finding about inhibition by substrate are original one. On the other hand, the fact that 2,6-dichlorophenolindophenol acetate can serve as a chromogenic substrate was mentioned by other scientist as wellCitation27.
Figure 4. Saturation for human AChE and 2,6-dichlorophenolindophenol acetate as a substrate. Error bars indicate standard deviations for n = 5.
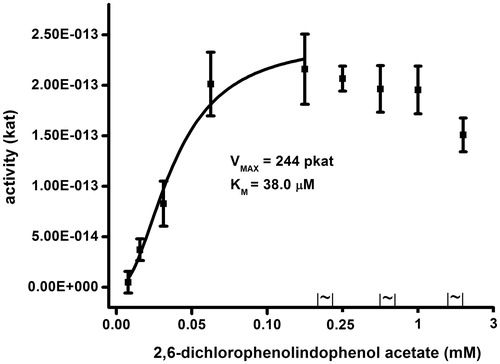
We tested interference for the assay as well. First, compounds typically interfering in Ellman’s method: reduced glutathione and albumin were chosen and interference was assessed. The substances did not cause any significant alteration in absorbance in a 100-µl solution taken in a cuvette in concentration 5 g/l for albumin and 5 µM reduced glutathione. The values covered expected range in a plasma sampleCitation28–30. Principle of the reaction is depicted as . It should be emphasized that the assay was developed for physiological pH. In acidic pH, phenolic moiety in 2,6-dichlorophenolindophenol is not dissociated and coloration is differentCitation27. Ascorbic acid can act as another interfering compound because it can react with created 2,6-dichlorophenolindophenol. In the reaction, 2,6-dichlorophenolindophenol is reduced to colorless formCitation31. Concentration of ascorbic acid in human plasma up to level 100 µM can be expectedCitation32. A 100-µl sized sample containing just ascorbic acid 100 µM was chosen for the reason. No significant interference was found when the sample was analyzed, which can be a result of the fact that pH of the reaction mixture was slightly alkaline (7.4).
Figure 5. Principle of redox change of 2,6-dichlorphenoindophenol color: blue color (form in the left part) is changed to colorless form because of reduction by, for example, ascorbic acid.
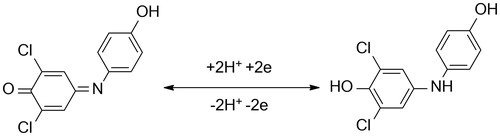
When compared with acetylcholine and 2,6-dichlorophenolindophenol acetate as substrates for AChE activity measurement, some pros and cons were discovered. While the standard Ellman’s method can be easily done because of high turnover rate, 2,6-dichlorophenolindophenol acetate has much lower turnover rate. In this experiment, the assay lasted 1 h which is much more than for the Ellman’s method and it represent some disadvantage. On the other hand, assay based on 2,6-dichlorophenolindophenol acetate is less sensitive to interferences and the color change is more contrasts (intensive blue) when compared to the Ellman’s method (light yellow). Because of higher wavelength, assay based on 2,6-dichlorophenolindophenol acetate does not overlap with compounds such as hemoglobin. It is similar to Ellman’s assay, where absorbance peak has similar position like peak of hemoglobinCitation10. The both methods correlates well one to each other as can be learned from . In this measurement, samples of AChE were assayed by the both methods and correlation coefficient r = 0.993 was found.
Figure 6. Correlation of AChE activity examination using 2,6-dichlorophenolindophenol acetate and Ellman's method. Error bars indicate standard deviations for n = 5.
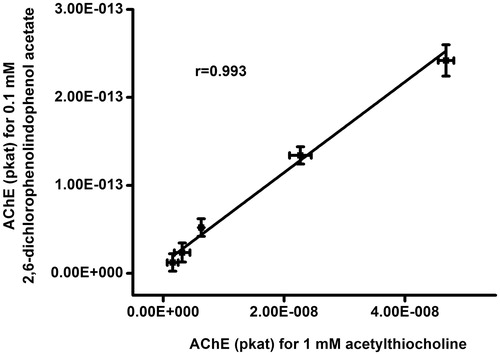
When compared with 2,6-dichlorophenolindophenol acetate, the product of hydrolysis, 2,6-dichlorophenolindophenol, is used as an redox indicator. It can be, for example, used for determining antioxidant capacityCitation33 or study of electron transfer during metabolismCitation34. Hence, interference in this way can be expected. However, it did not arise in this experiment probably because of the 2,6-dichlorophenolindophenol low concentration.
Conclusions
In this article, 2,6-dichlorophenolindophenol acetate was performed as an chromogenic substrate for AChE and compared to the standard Ellman’s method. The finding presented here encourage authors to claim that 2,6-dichlorophenolindophenol acetate is suitable for routine assay where AChE activity should be performed. Assay based on 2,6-dichlorophenolindophenol acetate is not sensitive to interferences under the conditions tested. Moreover, the assay uses long wavelength for absorbance measurement, hence typical interferents such as hemoglobin do not overlap with the assay comparing to the Ellman's method. Lower turnover rate when compared to acetylthiocholine is major disadvantage of the method and it is necessary to plan long time intervals for coloration arising.
Acknowledgements
The European Union is gratefully acknowledged for project TEAB; CZ.1.07/2.3.00/20.0235. A long-term organization development plan 1011 (Faculty of Military Health Sciences, University of Defence, Czech Republic) is acknowledged as well.
Declaration of interest
The authors declare no conflicts of interests. The authors alone are responsible for the content and writing of this article.
References
- Pohanka M. Acetylcholinesterase inhibitors: a patent review (2008 - present). Expert Opin Ther Pat 2012;22:871–86
- Pohanka M. Cholinesterases, a target of pharmacology and toxicology. Biomed Pap 2011;155:219–29
- de los Rios C. Cholinesterase inhibitors: a patent review (2007–2011). Expert Opin Ther Pat 2012;22:853–69
- Li B, Duysen EG, Carlson M, Lockridge O. The butyrylcholinesterase knockout mouse as a model for human butyrylcholinesterase deficiency. J Pharmacol Exp Ther 2008;324:1146–54
- Pohanka M. Butyrylcholinesterase as a biochemical marker, a review. Brat Med J 2013;114:726–34
- Pohanka M. Spectrophotomeric assay of aflatoxin B1 using acetylcholinesterase immobilized on standard microplates. Anal Lett 2013;46:1306–15
- Pohanka M. Acetylcholinesterase based dipsticks with indoxylacetate as a substrate for assay of organophosphates and carbamates. Anal Lett 2012;45:367–74
- Ellman GL, Courtney KD, Andres V Jr., Feather-Stone RM. A new and rapid colorimetric determination of acetylcholinesterase activity. Biochem Pharmacol 1961;7:88–95
- Arduini F, Errico I, Amine A, et al. Enzymatic spectrophotometric method for aflatoxin B detection based on acetylcholinesterase inhibition. Anal Chem 2007;79:3409–15
- Vasudevan G, McDonald MJ. Wavelength-dependent spectral changes accompany CN-hemin binding to human apohemoglobin. J Prot Chem 2000;19:583–90
- Pohanka M. Cholinesterases in biorecognition and biosensor construction, a review. Anal Lett 2013;46:1849–68
- Sinko G, Calic M, Bosak A, Kovarik Z. Limitation of the Ellman method: cholinesterase activity measurement in the presence of oximes. Anal Biochem 2007;370:223–7
- McGarry KG, Bartlett RA, Machesky NJ, et al. Evaluation of HemogloBind™ treatment for preparation of samples for cholinesterase analysis. Adv Biosci Biotechnol 2013;4:1020–3
- Collier HB. Letter: a note on the molar absorptivity of reduced Ellman’s reagent, 3-carboxylato-4-nitrothiophenolate. Anal Biochem 1973;56:310–11
- Riddles PW, Blakeley RL, Zerner B. Reassessment of Ellman’s reagent. Meth Enzymol 1983;91:49–60
- Bissbort SH, Vermaak WJH, Elias J, et al. Novel test and its automation for the determination of erythrocyte acetylcholinesterase and its application to organophosphate exposure. Clin Chim Acta 2001;303:139–45
- Trott O, Olson AJ. AutoDock Vina: improving the speed and accuracy of docking with a new scoring function, efficient optimization, and multithreading. J Comput Chem 2010;31:455–61
- Schuttelkopf AW, van Aalten DM. PRODRG: a tool for high-throughput crystallography of protein-ligand complexes. Acta Cristallographica D Biological Crystallogra 2004;60:1355–63
- Radic Z, Pickering NA, Vellom DC, et al. Three distinct domains in the cholinesterase molecule confer selectivity for acetyl- and butyrylcholinesterase inhibitors. Biochemistry 1993;32:12074–84
- Nair HK, Seravalli J, Arbuckle T, Quinn DM. Molecular recognition in acetylcholinesterase catalysis: free-energy correlations for substrate turnover and inhibition by trifluoro ketone transition-state analogs. Biochemistry 1994;33:8566–76
- Masson P, Schopfer LM, Bartels CF, et al. Substrate activation in acetylcholinesterase induced by low pH or mutation in the pi-cation subsite. Biochim Biophys Acta-Protein Struct Molec Enzym 2002;1594:313–24
- Masson P, Nachon F, Bartels CF, et al. High activity of human butyrylcholinesterase at low pH in the presence of excess butyrylthiocholine. Eur J Biochem 2003;270:315–24
- Visciarelli EC, Chopa CS, Picollo MI, Ferrero AA. Cholinesterase activity during embryonic development in the blood-feeding bug Triatoma patagonica. Med Vet Entomol 2011;25:297–301
- Ciliv G, Ozand PT. Human erythrocyte acetylcholinesterase purification, properties and kinetic behavior. Biochim Biophys Acta 1972;284:136–56
- Walsh SB, Dolden TA, Moores GD, et al. Identification and characterization of mutations in housefly (Musca domestica) acetylcholinesterase involved in insecticide resistance. Biochem J 2001;359:175–81
- Shi J, Boyd AE, Radic Z, Taylor P. Reversibly bound and covalently attached ligands induce conformational changes in the omega loop, Cys69-Cys96, of mouse acetylcholinesterase. J Biol Chem 2001;276:42196–204
- Miao Y, He N, Zhu JJ. History and new developments of assay for cholinesterase activity and inhibition. Chem Rev 2010;110:5216–34
- Michelet F, Gueguen R, Leroy P, et al. Blood and plasma glutathione measured in healthy subjects by HPLC: relation to sex, aging, biological variables, and life habits. Clin Chem 1995;41:1509–17
- Taravati A, Ardestani SK, Soroush MR, et al. Serum albumin and paraoxonase activity in Iranian veterans 20 years after sulfur mustard exposure. Immunopharmacol Immunotoxicol 2012;34:706–13
- Bhonsle HS, Korwar AM, Kote SS, et al. Low plasma albumin levels are associated with increased plasma protein glycation and HbA1c in diabetes. J Proteome Res 2012;11:1391–6
- Vanderjagt DJ, Garry PJ, Hunt WC. Ascorbate in plasma as measured by liquid-chromatography and by dichlorophenolindophenol colorimetry. Clin Chem 1986;32:1004–6
- Chung WY, Chung JKO, Szeto YT, et al. Plasma ascorbic acid: measurement, stability and clinical utility revisited. Clin Biochem 2001;34:623–7
- Merola ET, Catherman AD, Yehl JB, Strein TG. Determination of total antioxidant capacity of commercial beverage samples by capillary electrophoresis via in-line reaction with 2,6 – dichlorophenolindophenol. J Agric Food Chem 2009;57:6518–23
- Azarkina N, Konstantinov AA. Stimulation of menaquinone-dependent electron transfer in the respiratory chain of Bacillus subtilis by membrane energization. J Bacteriol 2002;184:5339–47