Abstract
In a new group of 3-methyl-2-phenyl-1-substituted-indole derivatives (10a–f), the indomethacin analogs were prepared via the Fisher indole synthesis reaction of propiophenone with appropriately substituted phenylhydrazine hydrochloride. This is followed by the insertion of the appropriate benzyl or benzoyl fragment. All the synthesized compounds were evaluated for their anti-inflammatory (in vitro and in vivo) and analgesic activities. The methanesulphonyl derivatives 10d, e and f showed the highest anti-inflammatory (in vitro and in vivo) and analgesic activities. In addition, molecular docking studies were performed on compounds 10a–f and the results were in agreement with that obtained from the in vitro COX inhibition assays. The significant anti-inflammatory and analgesic activities exhibited by 10d and 10e warrant continued preclinical development as potential anti-inflammatory and analgesic agents.
Introduction
Non-steroidal anti-inflammatory drugs (NSAIDs) produce their activity via inhibition of cyclooxygenase (COX) catalyzed biotransformation of arachidonic acid to pro-inflammatory prostaglandins (PGs) and thromboxanes (TXs)Citation1–3. Cyclooxygenase enzyme exists in two distinct isoforms, a constitutive form (COX-1) and an inducible form (COX-2); the constitutive COX-1 is responsible for the maintenance of physiological functions, such as protection of gastric mucosa, vascular homeostasis and platelet aggregationCitation4–6. Unlike COX-1, the COX-2 isoform is induced in response to mitogenic and pro-inflammatory stimuli and it is responsible for the progression of inflammationCitation7,Citation8. Thus, selective COX-2 inhibitor drugs (coxibs) as celecoxib (1), rofecoxib (2) and valdecoxib (3) were more useful for the treatment of inflammation and inflammation-associated disorders than the non-selective traditional NSAIDs as aspirin (4), ibuprofen (5) and indomethacin (6) (). Unfortunately, some cardiovascular side effects including the increased incidences of high blood pressure and myocardial infarction accompanied the highly selective COX-2 inhibitors were attributed to the biochemical changes in the COX pathwayCitation9,Citation10. These adverse effects resulted in the withdrawal of rofecoxib and valdecoxibCitation9,Citation10 ().
Figure 1. Chemical structures of some selective cyclooxygenase-2 (COX-2) inhibitor drugs (1–3) and some traditional non-selective NSAIDs (4–6).
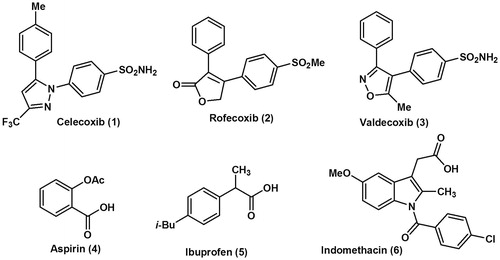
Indomethacin (6) is one of the most potent NSAIDs, which are effective against rheumatoid arthritis, ankylosing spondylitis, osteoarthritis of large joints and other types of inflammatory diseasesCitation11. At the same time, it is also one of the most ulcerogenic NSAIDs because of its high COX-1 selectivity and the acidic nature of the drugCitation12. From our point of view, there are two main approaches to prevent and/or treat gastroenteropathy associated with indomethacin as an example of traditional NSAIDs; the first comprises synthesis of prodrugs, where a nitric oxide (NO)-donating moiety is covalently attached to NSAID (indomethacin). This moiety should reduce the gastrointestinal toxicity through the protective effect of locally released NO on the gastric mucosa. In this regard, we previously reported some prodrugs of indomethacin and other NSAIDs, which showed higher safety profiles than of the parent NSAIDsCitation13,Citation14. The second strategy is based on maintaining the potency of the indomethacin by keeping the main scaffold of the drug with trials to increase COX-2 selectivity via the modifications of the side groups. Accordingly, the current work described the synthesis, molecular modeling studies, in vitro evaluation as COX-1/COX-2 inhibitors, and the in vivo anti-inflammatory (AI) activity of a new series of N-substituted indole derivatives as indomethacin analogs 10a–f in which; (i) the chlorobenzoyl moiety of indomethacin in position 1, which is important for anti-inflammatory activityCitation15, is maintained in 10b, 10e, replaced with benzoyl in 10a, 10d or replaced with benzyl in 10c, 10f, (ii) the methyl group in position 2 was replaced with the phenyl group and this replacement is expected to maximize the interaction with the hydrophobic residues within COX-2 active site and to enhance COX-2 selectivity, since the proposed compounds will be too large to fit into the smaller COX-1 active site, (iii) the –CH2COOH moiety in position 3 was replaced with a methyl group and this replacement is expected to decrease the acidity of the new compounds, which will consequently decrease the local action exerted by the direct contact of the new compounds with gastric mucosa, and (iv) the methoxy group in position 5 is replaced with H in 10a–c or with COX-2 pharmacophore methanesulfonyl moiety (SO2Me) in 10d–f to study the effect of SO2Me moiety on COX selectivity and anti-inflammatory activity ().
Results and discussion
Chemistry
The synthesis of the target 1-substituted-2-phenyl-3-methyl indole derivatives (10a–f) was accomplished in accordance with the sequence of reactions depicted in . Reaction of propiophenone (7) with either phenylhydrazine hydrochloride (8a) or 4-methylsulfonylphenylhydrazine hydrochloride (8b) in ethanol under the Fisher indole synthesis reaction conditions afforded the respective 2-phenyl-3-methylindole derivative 9a (79%) or 9b (82%). Replacement of the chlorine atom present in benzoyl chloride, 4-chlorobenzoyl chloride or benzyl chloride by the indole derivatives (9a–b) in DMF under basic conditions (NaH) afforded the target products (10a–f) in moderate yield (42–58%). All the prepared compounds have been characterized by IR, 1H NMR, 13C NMR, mass spectra and elemental analyses.
Biological evaluation
Anti-inflammatory activity
In vitro cyclooxygenase inhibition assay
In vitro structure–activity relationships acquired by this group of indomethacin derivatives (10a–f) showed that they are relatively weak inhibitors of COX-1 (IC50 = 7.78–42.36 µM) when compared with the reference drug indomethacin (IC50 = 0.63 µM). However, they are moderately-to-highly potent inhibitors of COX-2 (IC50 = 1.65 to 8.58 µM range) in comparison with indomethacin (IC50 = 11.63 µM) (). Compounds having a SO2Me moiety (COX-2 pharmacophore) were generally more potent inhibitors of COX-2 than the corresponding analogs free of SO2Me (10e > 10b; 10f > 10c; but 10a and 10d were approximately of the same potency). In general, all compounds were more selective to COX-2 enzyme with selectivity index (S.I.) = 0.90 to 25.67 compared to indomethacin (COX-2 S.I. = 0.055). The selectivity could be attributed to the size of compounds (10a–f), which is too large to fit into the smaller COX-1 active site. Moreover, the presence of COX-2 pharmacophore in some analogs (10d–f) increased the selectivity of COX-2 isozyme. The most potent COX-2 inhibitor (10e) with IC50 = 1.65 µM and S.I. = 25.65 had indomethacin as a chlorobenzoyl moiety in addition to SO2Me moiety (COX-2 pharmacophore) which was 467 times more COX-2 selective than indomethacin (COX-2 IC50 = 11.36 µM, S.I. = 0.055).
Table 1. In vitro COX-1 and COX-2 inhibition of compounds 10a–f, and reference drug (indomethacin).
In vivo anti-inflammatory activity
The anti-inflammatory activity of the test compounds compared to indomethacin was tested using the carrageen-induced rat paw edema test, which is widely used as a primary test for the screening of new anti-inflammatory agents. Results indicated that after 1 h, indomethacin derivatives 10a, b and c showed percentage anti-inflammatory activity of about 5.8–26.9%, while indomethacin derivatives with SO2Me 10d, e and f showed higher percentage of anti-inflammatory activity (67.9–73.5%). After 3 h, compounds 10a, b and c showed percentage anti-inflammatory activity of 8.8–17.4% and compounds 10d, e and f showed percentage anti-inflammatory activity of 66.8–80.1%. Similarly after 6 h, compounds 10a, b and c showed percentage activity of 6.1–21.2%, while compounds 10d, e and f showed percentage anti-inflammatory activity of 57.9–78.8%. 1-Benzyl-3-methyl-2-phenyl-1H-indole (10c) displayed the lowest anti-inflammatory activity at all-time intervals (5.8–8.8%), while (4-chlorophenyl)-(5-methanesulfonyl-3-methyl-2-phenyl-indol-1-yl)-methanone (10e) showed the highest anti-inflammatory activity (73.5%) at one-hour interval (more than indomethacin, 71.2%). The benzoyl derivative (10d) showed good anti-inflammatory activity at three- and six-hour intervals (80.1 and 78.8%, respectively) comparable to that of indomethacin (86.3 and 88.4%, respectively) and it was the most potent among the six compounds 10a–f at these time intervals. These data indicate that; (i) presence of methanesulfonyl (SO2Me) moiety (in compounds 10d, e and f) increases the anti-inflammatory activity for this class of compounds, and (ii) maintaining chlorobenzoyl moiety of indomethacin as in compound 10e or its replacement with benzoyl moiety (compound 10d) maintains or increases the anti-inflammatory activity, while its replacement with benzyl moiety (compound 10f) sharply decreased the anti-inflammatory activity ().
Table 2. Anti-inflammatory activities of compounds 10a–f, and reference drug (indomethacin) in carrageenan-induced rat paw edema assay.
Analgesic activity
The analgesic activity of the target compounds (10a–f) and indomethacin as a reference drug was also investigated using acetic acid-induced writhing test. Acetic acid-induced writhing was significantly reduced in mice receiving SO2Me containing compounds 10d, e and f, and the degree of inhibition of the writhing response by these compounds was 49.1, 65.1 and 59.3%, respectively, compared to the vehicle-treated control group. The analgesic activity of SO2Me free compounds (10a, b, and c) was relatively low (33.0, 30.2 and 18.9%, respectively) (). From these results, the analgesic activities of these compounds are clearly parallel to their anti-inflammatory activities.
Figure 3. Analgesic effect of test compounds 10a–f compared to indomethacin using acetic acid-induced writhing in mice. Data represent the mean value ± SD of four mice per group. Statistical comparisons between basal and post-drug values were analyzed for statistical significance using the one-way ANOVA followed by Dunnett’s test and denoted by *p < 0.05, #p < 0.01.
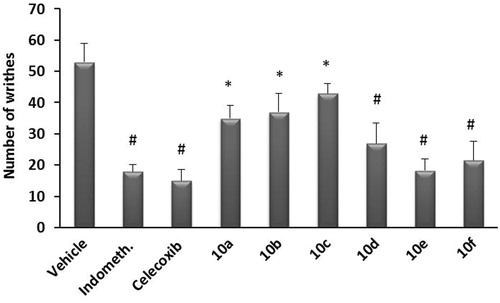
Molecular modeling
To know the plausible mode of interactions of the prepared compounds 10a–f within the COX-1 and COX-2 isozymes, molecular docking experiments were performed using X-ray, crystal structure data for COX-1 and COX-2 were obtained from the protein data bankCitation16,Citation17. Indomethacin (6) – as a selective COX-1 inhibitor – was used as a ligand for COX-1 isoform while, valdecoxib (3) was used as a ligand for COX-2 isoform. The main interactions of indomethacin with COX-1 resulted in two main residues Tyr385 and Arg120 amino acids, sequentially forming two hydrogen bonding interactions with –N and –CO of indomethacin at a distance equal to 2.96 and 2.76 Å, respectively. On the other hand, docking valdecoxib into COX-2 isozyme afforded three hydrogen bonding interactions; (i) NH2 with His94 (3.28 Å), (ii) NH2 with Thr199 (2.73 Å), and (iii) SO2 with Thr199 (2.80 Å).
The docking results including the energy associated with intermolecular interactions (affinity in kcal/mol) obtained upon computational docking for all compounds (10a–f, indomethacin and valdecoxib) within COX-1 and COX-2 active sites and the hydrogen bonding interactions between the amino acid residues and functional groups of the compounds are summarized in . For COX-1, all six compounds 10a–f indicated lower binding interactions (affinity in kcal/mol ranges from −5.5167 to −12.1998 with no or one hydrogen bonding interaction) than indomethacin (−17.0967 with two hydrogen bonding interactions). In case of COX-2, while compounds 10a–c showed appreciable binding interactions (affinity in kcal/mol ranges from −11.4647 to −15.6805 with one or two hydrogen bonding interactions), compounds 10d–f (have SO2Me as COX-2 pharmacophore) showed excellent binding interactions (affinity in kcal/mol ranges from −20.7351 to −21.7493 with two or three hydrogen bonding interactions) in comparison with valdecoxib (−17.0929 with three hydrogen bonding interactions). All the docked compounds were in response to COX-2 rather than COX-1 and that is consistent with their experimentally observed potent COX-2 inhibition ().
Figure 4. Binding of the most active compounds 10d and e inside COX-2 active site. (A) The proposed binding mode inside the active site of COX-2 resulting from docking, the most important amino acids are shown together with their respective numbers. (B) 2D interaction.
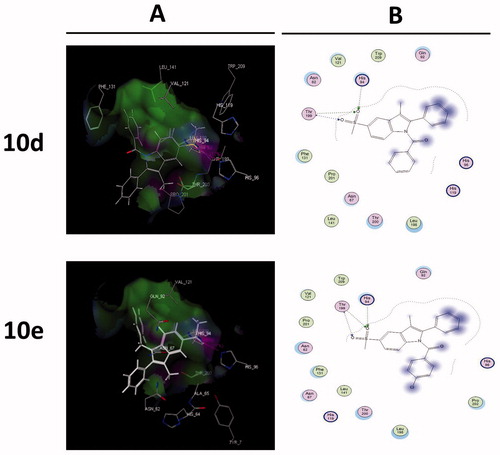
Table 3. Molecular modeling data for compounds 10a–f, indomethacin and valdecoxib during docking in the COX-1 (PDB:ID 4COX) and COX-2 (PDB:ID 2AW1) active sites.
Conclusion
Six new compounds 10a–f, as indomethacin derivatives, were synthesized for biological evaluation as anti-inflammatory and analgesic activities. The in vitro anti-inflammatory activity studies showed that all compounds were more COX-2 selective (COX-2 S.I. = 0.90 to 25.67) than indomethacin (COX-2 S.I. = 0.055), especially compound 10e (467 times more COX-2 selective than the reference drug indomethacin). While, the in vivo anti-inflammatory activity studies indicated that compounds 10d, e and f (have SO2Me as COX-2 pharmacophore) displayed good anti-inflammatory activity (57.9–80.1%) comparable to that of indomethacin (71.2–88.4%), especially 10d (73.1–80.1%) and 10e (71.8–73.5%). In addition, the docking experiments showed that all the docked compounds were fitted to COX-2 rather than COX-1 and compounds 10d–f (having SO2Me as COX-2 pharmacophore) showed excellent binding interactions (−20.7351 to −21.7493 kcal/mol) with two or three hydrogen bonding interactions in comparison with the COX-2 selective drug (valdecoxib, −17.0929 kcal/mol and three hydrogen bonding interactions), which was in parallel correlation with the in vitro COX inhibition evaluation. Also, the analgesic activities of these compounds 10a–f are clearly parallel to their anti-inflammatory activities. Because of their relatively potent anti-inflammatory and analgesic activities, the compounds 10d and 10e warrant continued preclinical development as potential anti-inflammatory and analgesic agents.
Experimental
Chemistry
Melting points were determined on a Thomas-Hoover capillary apparatus and are uncorrected. Infrared (IR) spectra were recorded as films on NaCl plates using a Nicolet 550 Series II Magna FT-IR spectrometer (Middleton, WI). 1H NMR and 13C NMR spectra were measured on a Bruker Avance III 400 MHz (Bruker BioSpin AG, Fällanden, Switzerland) for 1H and 100 MHz for 13C with BBFO Smart Probe and Bruker 400 AEON Nitrogen-Free Magnet, Faculty of Pharmacy, Beni-Suef University, Egypt, in DMSO-d6 with TMS as the internal standard, where J (coupling constant) values are estimated in Hertz (Hz) and chemical shifts were recorded in ppm on δ scale. Mass spectra (MS) were recorded on a Hewlett Packard 5988 spectrometer (Palo Alto, CA). Microanalyses for C, H and N were carried out on Perkin-Elmer 2400 analyzer (Perkin-Elmer, Norwalk, CT) at the Micro analytical unit of Cairo University, Egypt, and all compounds were within ± 0.4% of the theoretical values. All other reagents, purchased from the Aldrich Chemical Company (Milwaukee, WI), were used without further purification. 4-Methylsulfonylphenylhydrazine hydrochloride (8b) was prepared according to a previous procedureCitation18.
General procedure for synthesis of 3-methyl-2-phenylindole derivatives 9a–b
A mixture of propiophenone (7, 1.3 g, 0.01 mol) and the appropriate phenyl hydrazine hydrochloride (8a or 8b, 0.01 mol) in glacial acetic acid (30 mL) was heated under reflux for 10 h. After cooling, the reaction mixture was poured into ice-cold water. The separated solid was filtered, dried and crystallized from acetone to give pure compounds 9a and 9b. Physical and spectral data are listed below.
3-Methyl-2-phenyl-1H-indole (9a)
Buff solidCitation19; Yield 79%; m.p. 136–138 °C; IR (KBr) 3331 (NH), 3023 (CH aromatic), 2924 (CH aliphatic) cm−1; 1H NMR (DMSO-d6) δ 2.44 (s, 3H, CH3), 7.04 (t, 1H, J = 7.2 Hz, phenyl H-4), 7.12–7.16 (m, 1H, indole H-5), 7.35–7.42 (m, 2H, indole H-6, H-7), 7.50–7.56 (m, 3H, phenyl H-3, H-5 and indole H-4), 7.70 (d, 2H, J = 7.6 Hz, phenyl H-2, H-6), 11.19 (s, 1H, NH, D2O exchangeable); 13C NMR (DMSO-d6) δ 10.30, 107.22, 111.48, 118.88, 119.03, 122.01, 127.43, 127.95, 129.16, 129.85, 133.55, 134.19, 136.38; EIMS (m/z) 208 (M + 1, 17.88%), 207 (M+, 100%), 206 (M-1, 89.24%). Anal. Calcd for C15H13N: C, 86.92; H, 6.32; N, 6.76. Found: C, 86.79; H, 6.22; N, 7.01.
5-Methanesulfonyl-3-methyl-2-phenyl-1H-indole (9b)
Brown solid; Yield 82%; m.p. 197–199 °C; IR (KBr) 3413 (NH), 3048 (CH aromatic), 2912, 2862 (CH aliphatic), 1307, 1158 (SO2) cm−1; 1H NMR (DMSO-d6) δ 2.47 (s, 3H, CH3), 3.19 (s, 3H, SO2CH3), 7.42 (t, 1H, J = 7.6 Hz, phenyl H-4), 7.53–7.59 (m, 3H, phenyl H-3, H-5 and indole H-7), 7.65–7.72 (m, 3H, phenyl H-2, H-6 and indole H-6), 8.16 (s, 1H, indole H-4), 11.81 (s, 1H, NH, D2O exchangeable); 13C NMR (DMSO-d6) δ 10.08 (CH3), 45.02 (SO2CH3), 108.84, 112.02, 119.23, 120.19, 128.21, 128.23, 129.18, 129.32, 131.51, 132.57, 136.95, 138.44; EIMS (m/z) 286 (M + 1, 24.21%), 285 (M+, 100%). Anal. Calcd for C16H15NO2S: C, 67.34; H, 5.30; N, 4.91. Found: C, 67.55; H, 5.58; N, 4.69.
General procedure for synthesis of 3-methyl-2-phenyl-1-substituted-indole derivatives (10a–f)
To a solution of the respective 2-phenyl indole derivative 9a or 9b (2.5 mmol) in dry DMF (5 mL), NaH (0.11 g, 4.5 mmol) was slowly added with stirring at room temperature for 30 min. Then, the reaction flask was cooled down on a bath of ice followed by a slow addition for a solution of the appropriate alkyl or acyl halide (2.5 mmol) in DMF (5 mL) and the mixture was stirred at room temperature overnight. The reaction mixture was poured into ice-cold water and extracted with hexane (4 × 10 mL). The organic layer was dried and crystallized from methanol/chloroform mixture (4:1) to give pure compounds 10a–f. Physical and spectral data are listed below.
(3-Methyl-2-phenyl-indol-1-yl)-phenyl-methanone (10a)
Buff solid; Yield 54%; m.p. 300–302 °C; IR (KBr) 3273 (CH aromatic), 2924 (CH aliphatic), 1675 (C=O) cm−1; 1H NMR (DMSO-d6) δ 2.50 (s, 3H, CH3), 7.04 (t, 1H, J = 7.2 Hz, phenyl H-4), 7.11–7.15 (m, 1H, indolyl H-5), 7.34–7.42 (m, 2H, indolyl H-6, H-7), 7.45 (m, 7H, phenyl H-2, H-3, H-5, H-6, indolyl H-4 and benzoyl H-3, H-5), 7.68–7.95 (m, 3H, benzoyl H-2, H-4, H-6); 13C NMR (DMSO-d6) δ 9.63 (CH3), 113.82, 116.35, 119.78, 123.29, 124.89, 127.93, 128.27, 128.46, 129.61, 129.77, 130.10, 131.19, 132.43, 133.22, 135.37, 136.95, 169.47 (C=O); EIMS (m/z) 312 (M + 1, 3.43%), 311 (M+, 13.64%), 105 (C7H5O+, 100%). Anal. Calcd for C22H17NO: C, 84.86; H, 5.50; N, 4.50. Found: C, 85.13; H, 5.56; N, 4.38.
(4-Chlorophenyl)-(3-methyl-2-phenyl-indol-1-yl)-methanone (10b)
Buff solid; Yield 57%; m.p. 99–101 °C; IR (KBr) 3061 (CH aromatic), 2992 (CH aliphatic), 1685 (C=O) cm−1; 1H NMR (DMSO-d6) δ 2.40 (s, 3H, CH3), 7.10–7.34 (m, 8H, 5 phenyl protons and indolyl H-5, H-6, H-7), 7.52–7.85 (m, 5H, indolyl H-4 and 4 benzoyl protons); 13C NMR (DMSO-d6) δ 9.64 (CH3), 114.05, 116.66, 119.81, 123.55, 125.15, 127.84, 128.51, 128.86, 130.21, 130.52, 131.95, 132.27, 134.19, 136.17, 136.89, 137.76, 168.63 (C=O); EIMS (m/z) 347 (M + 2, 9.70%), 345 (M+, 25.82%), 139 (C7H4ClO+, 100%). Anal. Calcd for C22H17NO: C, 76.41; H, 4.66; 10.25; N, 4.05. Found: C, 76.35; H, 4.49; N, 4.23.
1-Benzyl-3-methyl-2-phenyl-1H-indole (10c)
Yellow solidCitation20; Yield 49%; m.p. 110–112 °C; IR (KBr) 3059, 3027 (CH aromatic), 2923, 2859 (CH aliphatic) cm−1; 1H NMR (DMSO-d6) δ 2.23 (s, 3H, CH3), 5.31 (s, 2H, CH2), 6.83 (d, 2H, J = 6.8 Hz, benzyl H-2, H-6), 7.08–7.21 (m, 5H, benzyl H-3, H-4, H-5 and indole H-5, H-6), 7.33 (d, 1H, J = 7.6 Hz, indole H-7), 7.39–7.51 (m, 5H, phenyl protons), 7.57 (d, 1H, J = 7.6 Hz, indole H-4); 13C NMR (DMSO-d6) δ 9.76 (CH3), 47.06 (CH2), 108.76, 110.89, 119.13, 119.65, 122.23, 126.43, 127.40, 128.52, 128.76, 128.87, 129.05, 130.71, 131.84, 136.81, 137.70, 138.87; EIMS (m/z) 298 (M + 1, 12.57%), 297 (M+, 53.35%), 91 (, 100%). Anal. Calcd for C22H19N: C, 88.85; H, 6.44; N, 4.71. Found: C, 88.93; H, 6.56; N, 4.63.
(5-Methanesulfonyl-3-methyl-2-phenyl-indol-1-yl)-phenyl-methanone (10d)
Buff solid; Yield 55%; m.p. 151–153 °C; IR (KBr) 3061 (CH aromatic), 2925, 2859 (CH aliphatic), 1677 (C=O), 1321, 1178 (SO2) cm−1; 1H NMR (DMSO-d6) δ 2.41 (s, 3H, CH3), 3.19 (s, 3H, SO2CH3), 7.21–7.72 (m, 11H, phenyl protons, benzoyl protons and indolyl H-7), 7.76 (d, 1H, J = 7.2 Hz, indolyl H-6), 8.29 (s, 1H, indolyl H-4); 13C NMR (DMSO-d6) δ 10.08 (CH3), 45.02 (SO2CH3), 108.84, 112.03, 116.31, 119.60, 120.20, 127.33, 128.23, 128.64, 129.18, 129.31, 129.49, 130.35, 130.82, 131.60, 132.57, 138.79, 169.56 (C=O); EIMS (m/z) 390 (M + 1, 0.27%), 389 (M+, 0.93%), 285 (, 100%). Anal. Calcd for C23H19NO3S: C, 70.93; H, 4.92; N, 3.60. Found: C, 80.13; H, 5.04; N, 3.81.
(4-Chlorophenyl)-(5-methanesulfonyl-3-methyl-2-phenyl-indol-1-yl)-methanone (10e)
Buff solid; Yield 58%; m.p. 204–206 °C; IR (KBr) 3047 (CH aromatic), 2943, 2914 (CH aliphatic), 1672 (C=O), 1317, 1175 (SO2) cm−1; 1H NMR (DMSO-d6) δ 2.33 (s, 3H, CH3), 3.27 (s, 3H, SO2CH3), 7.23–7.29 (m, 5H, phenyl protons), 7.37 (d, 2H, J = 8.0 Hz, benzoyl H-3, H-5), 7.56 (d, 2H, J = 8.0 Hz, benzoyl H-2, H-6), 7.81 (d, 2H, J = 8.8 Hz, indolyl H-7), 7.86 (d, 2H, J = 8.8 Hz, indolyl H-6), 8.29 (s, 1H, indolyl H-4); 13C NMR (DMSO-d6) δ 9.51 (CH3), 44.58 (SO2CH3), 114.61, 116.61, 119.58, 123.30, 128.40, 128.69, 129.02, 130.24, 130.31, 131.48, 132.19, 133.35, 135.82, 138.42, 138.51, 139.00, 168.56 (C=O); EIMS (m/z) 425 (M + 2, 4.52%), 423 (M+, 11.15%), 139 (C7H4ClO+, 100%). Anal. Calcd for C23H18ClNO3S: C, 65.17; H, 4.28; N, 3.30. Found: C, 65.32; H, 4.41; N, 3.21.
1-Benzyl-5-methanesulfonyl-3-methyl-2-phenyl-1H-indole (10f)
Buff solid; Yield 42%; m.p. 235–237 °C; IR (KBr) 3031 (CH aromatic) 2921, 2861 (CH aliphatic), 1343, 1182 (SO2) cm−1; 1H NMR (DMSO-d6) δ 2.47 (s, 3H, CH3), 3.19 (s, 3H, SO2CH3), 5.40 (s, 2H, CH2), 6.83 (d, 2H, J = 7.6 Hz, benzyl H-2, H-6), 7.19–7.21 (m, 3H, benzyl H-3, H-4, H-5), 7.41–7.65 (m, 7H, phenyl protons and indole H-6, H-7), 8.18 (s, 1H, indole H-4); 13C NMR (DMSO-d6) δ 10.06 (CH3),45.03 (SO2CH3), 47.35 (CH2), 110.55, 111.59, 112.04, 119.38, 120.19, 127.66, 128.08, 128.23, 129.00, 129.12, 129.32, 130.73, 130.93, 132.16, 138.12, 138.72; EIMS (m/z) 376 (M + 1, 13.95%), 375 (M+, 47.96%), 91 (, 100%). Anal. Calcd for C23H21NO2S: C, 73.57; H, 5.64; N, 3.73. Found: C, 73.42; H, 5.53; N, 3.81.
Biological evaluation
Animals
Swiss albino mice (20–25 g) and Wistar rats (150–175 g) were obtained from the National Research Center, Cairo, Egypt. They were used throughout the study and were kept at controlled conditions (temperature 23 ± 2 °C, humidity 60 ± 10%) at a 12/12 h light/dark cycle. All procedures relating to animal care and treatments were conducted in accordance with protocols approved by the Research Ethical Committee of Faculty of Pharmacy Beni-Suef University (2014-Beni-Suef, Egypt).
COX-1/COX-2 inhibition colorimetric assay
The in vitro inhibition of COX-1/COX-2 was measured using colorimetric COX (ovine) Inhibitor Screening Assay Kit (Cayman Chemical, Ann Arbor, MI) according to manufacturer's instructions. This assay directly measures PGF2α that was produced by stannous chloride reduction of COX-derived PGH2 by enzyme immunoassay. All assays were conducted in triplicates and IC50 values are the average of three determinations for each compound.
Carrageenan-induced rat paw edema assay
Rats were administered with vehicle, test compounds, indomethacin or celecoxib at a dose of 10 mg/kg by oral gavages. Immediately thereafter, the rats received 100 µL of vehicle or carrageenan (1% in saline) s.c. on the plantar surface of the left hind paw under mild anesthesia, essentially, as reported beforeCitation21. The paw edema was evaluated by measuring paw-volume changes at 1, 3 and 6 h after carrageenan injection using a plethysmometer. The right hind paw served as a reference of non-inflamed paw for comparison. Results are expressed as paw-volume change (mL).
Acetic acid-induced writhing test
The writhing tests were carried as described beforeCitation22. Briefly, mice were administered orally vehicle, test compounds, indomethacin or celecoxib at a dose of 10 mg/kg 30 min prior to intraperitoneal administration of 0.7% v/v acetic acid solution (10 mL/kg body weight). The number of writhes (i.e. abdominal constriction followed by dorsiflexion and extension) occurring during a 20-min period beginning 5 min after acetic acid injection was measured. The results are expressed as the number of writhes per 20-min period.
Statistical analysis
Comparisons among the groups were analyzed for statistical significance using the one-way ANOVA followed by Dunnett’s test. Data were represented as mean ± standard deviation (SD). Differences were considered significant at *p < 0.05, and **p < 0.01, respectively.
Molecular modeling and docking
The crystal structures of indomethacin bound at COX-1 (PDB: ID 4COX)Citation17 and valdecoxib bound at the COX-2 (PDB:ID 2AW1) active sitesCitation18 [obtained from the protein data bank at Research Collaboration for Structural Bioinformatics (RSCB) Protein Database (PDB)]. Docking of the co-crystallized ligand should be carried out to study the scoring energy(s), root mean standard deviation (RMSD) and amino acid interactions. The RMSD, which is a measure of superposing was 0.50 Å for the lead compounds. Docking was performed using London dG force and refinement of the results was done using force field energy. Preparation of the synthesized compounds for docking was achieved via their 3D structure built by Molecular Operating Environment (MOE, Version 2005.06, Chemical Computing Group Inc., Montreal, Quebec, Canada). Certain procedures were taken before docking, which include: 3D protonation of the structures, running conformational analysis using systemic search, selecting the least energetic conformer and applying the same docking protocol used with ligands. Docking for the synthesized compounds was applied. Amino acid interactions and the hydrogen bond lengths are summarized in .
Declaration of interest
The authors have declared no conflict of interest.
References
- Fletcher BS, Kujubu DA, Perrin DM, Herschman HR. Structure of the mitogen-inducible TIS10 gene and demonstration that the TIS10-encoded protein is a functional prostaglandin G/H synthase. J Biol Chem 1992;267:4338–44
- Zarghi A, Najafnia L, Daraee B, et al. Synthesis of 2,3-diaryl-1,3-thiazolidine-4-one derivatives as selective cyclooxygenase (COX-2) inhibitors. Bioorg Med Chem Lett 2007;17:5634–7
- Zebardast T, Zarghi A, Daraie B, et al. Design and synthesis of 3-alkyl-2-aryl-1,3-thiazinan-4-one derivatives as selective cyclooxygenase (COX-2) inhibitors. Bioorg Med Chem Lett 2009;19:3162–5
- Smith WL, Dewitt DL. Prostaglandin endoperoxide H synthases-1 and -2. Adv Immunol 1996;62:167–215
- Jouzeau JY, Terlain B, Abid A, et al. Cyclo-oxygenase isoenzymes. How recent findings affect thinking about nonsteroidal anti-inflammatory drugs. Drugs 1997;53:563–82
- Kalgutkar AS, Marnett AB, Crews BC, et al. Ester and amide derivatives of the nonsteroidal antiinflammatory drug, indomethacin, as selective cyclooxygenase-2 inhibitors. J Med Chem 2000;43:2860–70
- Herschman HR. Prostaglandin synthase 2. Biochim Biophys Acta 1996;1299:125–40
- Dubois RN, Abramson SB, Crofford L, et al. Cyclooxygenase in biology and disease. FASEB J 1998;12:1063–73
- Scheen AJ. Withdrawal of rofecoxib (Vioxx): what about cardiovascular safety of COX-2 selective non-steroidal anti-inflammatory drugs? Rev Med Liege 2004;59:565–9
- Dogne JM, Supuran CT, Pratico D. Adverse cardiovascular effects of the coxibs. J Med Chem 2005;48:2251–7
- Kaur J, Bhardwaj A, Huang Z, Knaus EE. N-1 and C-3 substituted indole Schiff bases as selective COX-2 inhibitors: synthesis and biological evaluation. Bioorg Med Chem Lett 2012;22:2154–9
- Bandgar BP, Sarangdhar RJ, Viswakarma S, Ahamed FA. Synthesis and biological evaluation of orally active prodrugs of indomethacin. J Med Chem 2011;54:1191–201
- Abdellatif KR, Chowdhury MA, Dong Y, et al. Dinitroglyceryl and diazen-1-ium-1,2-diolated nitric oxide donor ester prodrugs of aspirin, indomethacin and ibuprofen: synthesis, biological evaluation and nitric oxide release studies. Bioorg Med Chem Lett 2009;19:3014–18
- Huang Z, Velazquez CA, Abdellatif KR, et al. Ethanesulfohydroxamic acid ester prodrugs of nonsteroidal anti-inflammatory drugs (NSAIDs): synthesis, nitric oxide and nitroxyl release, cyclooxygenase inhibition, anti-inflammatory, and ulcerogenicity index studies. J Med Chem 2011;54:1356–64
- Chowdhury MA, Huang Z, Abdellatif KR, et al. Synthesis and biological evaluation of indomethacin analogs possessing a N-difluoromethyl-1,2-dihydropyrid-2-one ring system: a search for novel cyclooxygenase and lipoxygenase inhibitors. Bioorg Med Chem Lett 2010;20:5776–80
- Kurumbail RG, Stevens AM, Gierse JK, et al. Structural basis for selective inhibition of cyclooxygenase-2 by anti-inflammatory agents. Nature 1996;384:644–8
- Di Fiore A, Pedone C, D'Ambrosio K, et al. Carbonic anhydrase inhibitors: valdecoxib binds to a different active site region of the human isoform II as compared to the structurally related cyclooxygenase II “selective” inhibitor celecoxib. Bioorg Med Chem Lett 2006;16:437–42
- Abdellatif KR, Chowdhury MA, Dong Y, et al. Diazen-1-ium-1,2-diolated nitric oxide donor ester prodrugs of 5-(4-hydroxymethylphenyl)-1-(4-aminosulfonylphenyl)-3-trifluoromethyl-1H-pyrazole and its methanesulfonyl analog: synthesis, biological evaluation and nitric oxide release studies. Bioorg Med Chem 2008;16:9694–8
- Pena-Lopez M, Neumann H, Beller M. Ruthenium-catalyzed synthesis of indoles from anilines and epoxides. Chemistry 2014;20:1818–24
- Dou X, Yao W, Wen S, Lu Y. Facile regiospecific synthesis of 2,3-disubstituted indoles from isatins. Chem Commun (Camb) 2014;50:9469–72
- El-Nezhawy AO, Biuomy AR, Hassan FS, et al. Design, synthesis and pharmacological evaluation of omeprazole-like agents with anti-inflammatory activity. Bioorg Med Chem 2013;21:1661–70
- Abdelazeem AH, Abdelatef SA, El-Saadi MT, et al. Novel pyrazolopyrimidine derivatives targeting COXs and iNOS enzymes; design, synthesis and biological evaluation as potential anti-inflammatory agents. Eur J Pharm Sci 2014;62:197–211