Abstract
G6PD, 6PGD and GR have been purified separately in the single step from rat lung using 2′, 5′-ADP Sepharose 4B affinity chromatography. The purified enzymes showed a single band on sodium dodecyl sulfate polyacrylamide gel electrophoresis (SDS-PAGE). The molecular weights of the enzymes were estimated to be 134 kDa for G6PD, 107 kDa for 6PGD and 121 kDa for GR by Sephadex G-150 gel filtration chromatography, and the subunit molecular weights was respectively found to be 66, 52 and 63 kDa by SDS-PAGE. Optimum pH, stable pH, optimum ionic strength, optimum temperature, KM and Vmax values for substrates were determined. Product inhibition studies were also performed. The enzymes were inhibited by levofloxacin, furosemide, ceftazidime, cefuroxime and gentamicin as in vitro with IC50 values in the range of 0.07–30.13 mM. In vivo studies demonstrated that lung GR was inhibited by furosemide and lung 6PGD was inhibited by levofloxacin.
Introduction
Both glucose-6-phosphate dehydrogenase (EC 1.1.1.49; G6PD) and 6-phospho gluconate dehydrogenase (EC 1.1.1.44; 6PGD) are two main enzymes in the hexose monophosphate shunt, where ribose 5-phosphate (R5P) and NADPH are generated. R5P is required for the synthesis of nucleotides. NADPH has very important functions in the prevention of proteins, lipids, DNA, RNA and other molecules from oxidative damage in all cellsCitation1,Citation2. NADPH also serves several vital functions including lipid synthesis, fatty acid chain elongation, some amino acid syntheses and cholesterol synthesisCitation3.
Glutathione reductase is known as a substrate specific antioxidant enzyme (E.C.1.8.1.7; GR) that catalyzes the conversion of glutathione from oxide form to reduced form, an important cellular antioxidant, the presence of NADPHCitation4. GSH is necessary in the decomposition of the hydrogen peroxide and other organic peroxides, the resistance of oxidative cases, bioremediation of xenobiotics, and defending of the disulfide bonds of cellular proteinsCitation5. Moreover, it is reported that GSH has a major role in the arrangement of apoptosis and is essential for the development of cellsCitation6.
G6PD, 6PGD and GR are housekeeping enzymes, present in the microorganisms, blood cells, plant and animal tissuesCitation7. They have been purified from several mammalian tissues with various techniques such as rat liver and kidneyCitation8–10, human erythrocyteCitation11,Citation12, rat small intestineCitation13, grass carp hepatopancreasesCitation14, rainbow trout liverCitation15 and lamb kidneyCitation16, and some of their characteristics and kinetic properties have been determined.
It is well known that many pharmaceutical agents have many adverse effects on the enzyme activities in vitro and in vivoCitation17,Citation18. These drugs may lead to important problems beyond its anticipated therapeutic effects during clinical useCitation19,Citation20. Inhibition of these enzymes will cause to decrease the amount of NADPH and GSH which are required in several biological functions including cleaning-up of oxidative stress products and xenobiotic detoxification. Accordingly, these shortages cause some problems, such as extended period disease, affect the quality of life of patients, increase costs of patient care, the triggering of other diseases and the likeCitation19,Citation21,Citation22.
There is no article available on the biochemical characterization and purification of three enzymes from rat lung, and on the effects of five drugs on the enzyme activities. This study was aimed to purify and characterize rat lung G6PD, 6PGD and GR, and to investigate effects of some antibiotics on the enzyme activities in laboratory conditions. In addition, effects of levofloxacin and furosemide on the activities of the enzymes were investigated in rat lung as in vivo.
Materials and methods
Materials
The protein standards and chemicals were obtained from Sigma Chem. (Munich, Germany) and Merck (Darmstadt, Germany). Affinity material was purchased from Pharmacia (Pittsburgh, PA). Blue Dextran 2000 were supplied from Sigma Chem. (St. Louis, MO).
Preparation of crude extract
Animals were sacrificed after anesthesia. Lungs removed were washed with a cold isotonic saline solution and then, preserved at −80 °C. Tissue was cut into small pieces and powdered by liquid nitrogen. The sample was transferred to the 1 mM EDTA + 2 mM DDT + 20 mM Tris–HCl (pH = 7.5) solution and centrifuged at 4 °C, 12 000 rpm for 20 min. Next experiments were executed with the clear supernatant.
Purification of the enzymes
2′, 5′-ADP Sepharose 4B was prepared according to the method previously described for affinity columnCitation23. The supernatant was applied onto the column with 10 ml column material and the column was washed with 50 mM phosphate buffer (1 mM DTT, 1 mM EDTA, pH 7.35) until the final absorbance difference became zero at 280 nm. Firstly, G6PD was eluted with 80 mM phosphate + 80 mM KCl + 0.5 mM NADP + 1 mM EDTA, pH 7.85. Secondly, 6PGD was eluted with a buffer of 80 mM KCl + 5 mM NADP + 1 mM EDTA + 80 mM phosphate, pH 7.85. At last, the solution (0.5 mM NADPH + 1 mM GSH + 1 mM EDTA + 50 mM phosphate, pH 7.35) was used to remove the GR from the column. For each enzyme, fractions with activity have been pooled. Then, the eluates were dialyzed against 20 mM cold phosphate buffer, pH 7.3 at +4 °C for 24 h.
Enzyme assay
The measurements of enzyme activity were done according to Carlberg and Mannervik’s methodsCitation24 for GR and Beutler’s method for G6PD and 6PGDCitation25 with a Shimadzu Spectrophotometer UV-(1208) (Tokyo, Japan). All activity measurements were carried out at the saturated concentration of substrates and coenzymes in optimal pH and ionic strength determined experimentally.
Protein determination
The amount of protein in each step was detected with the method stated by Bradford at 595 nmCitation26 and bovine serum albumin was used to draw a standard graph.
Electrophoresis
In order to determine the subunit molecular weights and control the enzyme purity, SDS-polyacrylamide gel electrophoresis was made based on the method described by LaemmliCitation27, and bovine carbonic anhydrase (30 kDa), chicken ovalbumin (45 kDa) and bovine albumin (66 kDa), rabbit phosphorylase B (97 400) and E. coli P-galactosidase (116 000) and rabbit myosin (205 000) were used as markers.
Gel filtration
Sephadex G-150 was used to determine the native molecular mass of enzymes. The column was prepared in the 40 ml bed volume and equilibrated with 50 mM Tris–HCl (pH 7.5) + 100 mM KCl buffer. Standard graph was drawn using the molecular mass standards (12.4–200 kDa) whose elution volumes were detected by measuring the protein absorbance at 280 nm and the void volume of column was calculated with Blue Dextran 2000. The elution volume of each enzyme was identified by surveying the enzyme activity.
Biochemical characteristics of enzymes
To determine the optimum conditions for the enzymes, the activities of enzymes were tested in 0.05 M H3PO4–H3BO3 buffer within the pH range of 5.5–9.5 for optimum pH, in the range from 20 mM to 1 M Tris–HCl buffer for optimum ionic strength, between 0 °C and 90 °C for optimum temperatures and in 0.2 M Tris–HCl buffer in pH of 8.0, 8.5 and 9.0, and in 0.2 M phosphate buffer in pH of 5.5, 6.0, 6.5, 7.0 and 7.5 for stable pH at the equal units of enzyme and buffer solutions.
Lineweaver–Burk graphs were used to determine both KM and Vmax values of substrates/coenzymes and the Ki constants and types of inhibition for product inhibitionCitation28. The activities of the enzymes were tested at five different substrate/coenzyme concentrations with a changeless concentration of another substrate. Effect of NADPH on G6PD was determined by two different concentrations of NADPH (0.1–0.2 mM) with 0.2 mM G6P and NADP+ concentrations varied from 4 μM to 20 μM. To determine inhibition type and Ki value, NADPH on 6PGD were tested by two different inhibitor concentrations (NADPH: 0.16 and 0.26 mM) with 0.2 mM G6P and NADP+ concentrations varying from 4 μM to 20 μM. For NADP+ and GSH, product inhibitions on the GR were investigated. For each product, varying two concentrations were examined (GSH: 15 and 25 mM; NADP+: 0.4 and 0.8 mM). All of the kinetic studies on the enzymes were made at 25 °C in optimal pH and ionic strength.
In vitro drug effects
To determine the effects of five antibiotics on rat lung, enzymes purified were put in the reaction solution by varying the five different drug concentrations. The enzyme activities without drugs were taken as 100%. For each drug (levofloxacin between 0.05 and 0.12 mM, furosemide between 0.1 and 0.3 mM, ceftazidime between 0.7 and 3 mM, between cefuroxime 3 and 15 mM, and gentamicin between 5 and 25 mM for G6PD; levofloxacin between 0.05 and 0.12 mM, furosemide between 0.1 and 0.3 mM, ceftazidime between 0.7 and 3 mM, cefuroxime between 3 and 15 mM, and gentamicin between 5 and 25 mM for 6PGD; levofloxacin between 0.01 and 0.04 mM, furosemide between 0.05 and 0.17 mM, ceftazidime between 0.3 and 1 mM, cefuroxime between 0.5 and 3 mM, and gentamicin between 15 and 42 mM for GR), Activity%–[Drug] graphs were drawn and used to calculate the drug concentrations (IC50 values) causing a 50% decrease in enzyme activity. The types of inhibition and Ki constants were determined via Lineweaver–Burk graphs.
In vivo drug effect
Twelve young Sprague–Dawley rats with a weight of 140–180 g were supplied from Ataturk University Experimental Medical Application and Research Centre. Animals were maintained under standard laboratory conditions before the experiment. Drug dosages were determined according to the literatureCitation29. Levofloxacin and furosemide were administered as 10 mg/kg via intraperitoneal. After 25 min of the injection, rats were anesthetized with ethanol and sacrificed. The lungs were hastily taken, washed with cold isotonic saline (0.9 NaCl) and stored at −80 °C until use. This study received ethical approval from the Ethics Committee on Animal Research of Ataturk University.
Analysis of kinetic data
In vitro data were analyzed by Microsoft Office Excel 2010 and was given as ± SD. One-way analysis of variance (ANOVA) with post hoc least significant difference (LSD) test was used to compare the group means for the results of the in vivo studies and p < 0.05 was noted statistically considerable. Statistical analyses were made using SPSS 15.0 (Armonk, NY).
Results
As seen in , by delivering NADP+ and NADPH gradient elution with 2′, 5′-ADP Sepharose 4B affinity chromatographic techniques, G6PD enzyme was eluted in tubes 5–9, 6PGD enzyme in tubes 15–19 and GR enzyme in tubes 24–28. G6PD was eluted with 0.5 mM NADP+, while 6PGD was eluted with 5 mM NADP+ and GR with 0.5 mm NADPH. The enzymes were pure and showed a single band (). shows that G6PD enzyme with 181.38 EU/mg protein specific activity was purified 580 times with 96% efficiency, 6PGD enzyme with 133.976 EU/mg protein specific activity 784 times with 90% efficiency, GR enzyme with 34.88 EU/mg protein specific activity 940 times with 95% efficiency. SDS-PAGE and gel filtration chromatography was implemented for molecular mass calculation of natural and subunits of the enzymes. Rf–log MW graph was drawn with the help of and subunit molecular mass of G6PD, 6PGD and GR enzymes were respectively calculated as 66, 52 and 63 kDa. Natural molecular mass of same enzymes were respectively calculated as 134, 110 and 121 kDa via Kav–log MW graph plotted according to the results of gel filtration chromatography. Studies made for optimal conditions (pH, ionic strength, temperature and stable pH) have been represented in .
Table 1. Purification scheme of G6PD, 6PGD and GR from rat lung.
Table 2. Optimum conditions for G6PD, 6PGD and GR the rat lung.
Vmax and KM values of the substrate and coenzymes were obtained from the Lineweaver–Burk graphs. KM values of G6P, 6PGA and GSSG were 0.028, 0.030 and 0.230 mM, and Vmax value of the same substrate were 0.431, 1.398 and 0.263 EU/ml, respectively. KM values calculated for NADP+ at rat lung G6PD and 6PGD were 0.0053 and 0.030 mM, and Vmax values at same enzymes were 0.446 and 1.398 EU/ml. Vmax and KM values were found as 0.020 mM and 0.125 EU/ml for NADPH at rat lung GR.
Figure 1. Purification of rat lung G6PD, 6PGD and GR enzymes by 2′, 5′-ADP sepharose 4B affinity column.
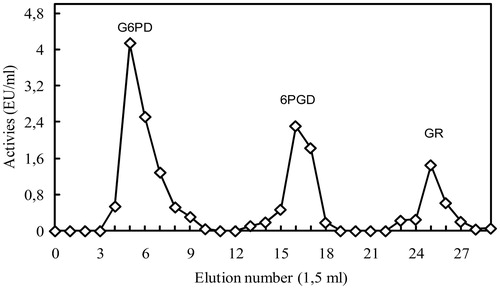
Figure 2. SDS-PAGE bands of enzymes (Lane 1: rat lung 6PGD, Lane 2: GR rat lung G6PD; Lane 3: rat lung, Lane 4: Standard proteins: bovine carbonic anhydrase (30 kDa), chicken ovalbumin (45 kDa) and bovine albumin (66 kDa), rabbit phosphorylase B (97 400) and E. coli P-galactosidase (116 000) and rabbit myosin (205 000)).
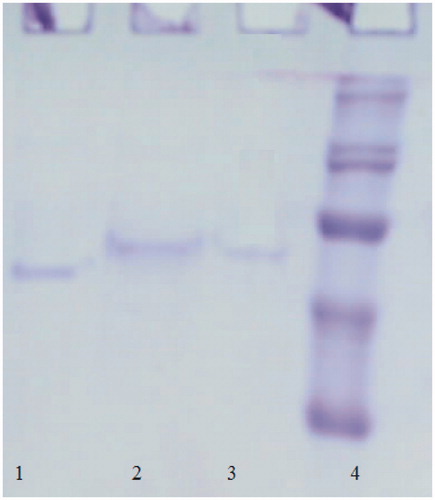
Figure 3. Graphs drawn to calculate the Ki and IC50 values of levofloxacin on the rat lung G6PD, 6PGD and GR.
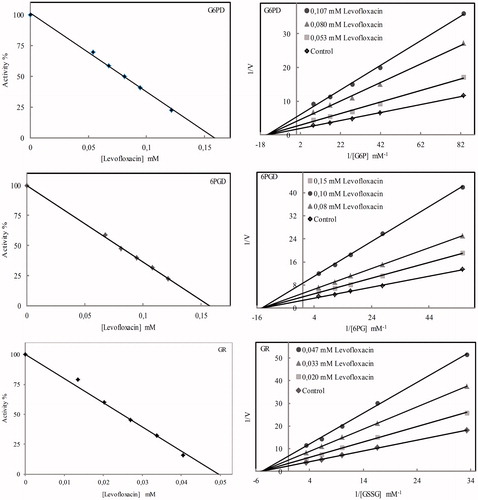
The product inhibition studies showed that NADPH markedly inhibited the activity of G6PD enzyme as uncompetitive with a Ki of 27 ± 2 μM and the activity of 6PGD as noncompetitive with a Ki of 147 ± 5 μM. Also, rat lung GR were noncompetitively inhibited by GSH with Ki constant with 16.66 ± 1.11 mM and NADP+ with Ki constant with 0.531 ± 0.04 mM.
Inhibition effect of drugs on enzymes is expressed in IC50 values and Ki constants. IC50 and Ki graphs plotted to determine effects on enzymes activities of levofloxacin are shown in . IC50 values and Ki constants in show that furosemide and levofloxacin are the drugs that inhibit G6PD, 6PGD and GR enzymes the most. Other drugs are ranked in terms of inhibition effects from largest to smallest respectively as ceftazidime, cefuroxime and gentamicin. In addition to in vivo studies, for levofloxacin and furosemide drugs with the highest inhibition effects, in vitro studies were conducted on rats. 6PDG enzyme with p values smaller than 0.05 is highly inhibited by levofloxacin and GR enzyme by furosemide. G6PD enzyme is activated by furosemide ().
Table 3. IC50 values, Ki constants and inhibition type obtained from regression analysis graphs for rat lung G6PD, 6PGD and GR in the presence of different drugs (NC: noncompetitive; C: competitive).
Table 4. In vivo effects of levofloxacin and furosemide on rat lung G6PD, 6PGD and GR activities.
Discussion
Glucose 6-phosphate dehydrogenase (G6PD) is a housekeeping enzyme catalyzing the first step of the phosphogluconate pathway. Similarly, 6-phosphogluconate dehydrogenase (EC.1.1.1.44; 6PGD) catalyzes the third reaction in the same pathway, and in the existence of NADP+, converts from 6-phosphogluconate molecule to D-ribulose 5-phosphate. The hexose monophosphate shunt is the essential source for NADPH generation in all cells, and deficiency of both enzymes significantly reduces the amount of NADPH playing a vital role for the cellCitation30,Citation31. As a member of oxidoreductase enzymes, glutathione reductase (GR) enzyme (NADPH; GSSG oxidoreductase EC 1.6.4.2) converts oxidized glutathione (GSSP) into reduced glutathione (GSH). For this reason, this enzyme is significant as an antioxidantCitation32,Citation33.
2′, 5′-ADP Sepharose 4B material is used effectively to purify the NADPH/NADP+ dependent enzymes for years. Erat purified G6PD and GR enzymes in a single step with human erythrocyte-gradient elutionCitation34. In another study by us, G6PD, 6PDG and GR enzymes were purified from rat kidney with gradient elution for the first time. We applied the same method here with success and these three enzymes were separately eluted in the same step. These specific activities, % efficiency and purification coefficients are optimal values for kinetic studies. Moreover, compared to the literature, the purification process was fairly successful and suitable for scientific studiesCitation13,35–37.
These enzymes have been purified from several mammalian tissues. It has been reported that they were homodimer protein with native molecular mass in the range of 100–125 kDA. The active forms of the purified enzymes may be homodimer since natural molecular masses of enzymes are almost twice as much as subunit molecular masses. These molecular masses and subunit numbers show parallelism with the literature findingsCitation9,23,36,38–41.
Tests carried out to calculate the effect of ionic strength on enzymes, the most suitable ionic strength environment was determined as 200 mM Tris–HCl for G6PD, 200 mM phosphate for 6PGD and 80 mM Tris–HCl buffer for GR enzyme. Calculations show that G6PD, 6PGD and GR enzymes show the highest activity with pH values respectively as 8.0 (50 mM H3PO4–H3BO3), 8.0 (50 mM H3PO4–H3BO3) and 8.5 (80 mM Tris–HCI). Hence, these pH values are accepted as optimal pH values. Research showed that these results are highly parallel to other results in the literatureCitation8,37,42–44.
For optimal temperature calculation of the enzymes, activities of optimal ionic strength and optimal pH enzymes were measured at 10 °C intervals within 10–70 °C range. Calculations show that G6PD, 6PDG and GR enzymes show the highest activity respectively at 55 °C, 40 °C and 65 °C values. Therefore, this temperature is accepted as the optimal temperature. According to these results, GR is the most resistant enzyme against temperature. This result is also in parallel with similar results in the literatureCitation16,35,45–48.
For calculation of stable pH of enzymes, 200 mM phosphate buffers with 5.5, 6.0, 6.5, 7.0 and 7.5 pH, and 200 mM Tris–HCl buffers with 8.0, 8.5 and 9.0 pH were preserved at +4 °C together with the equal amount of enzyme solution, and activity measurements were performed for 72 h with 12 h intervals. The most stable pH of G6PD, GR and 6PDG enzymes are determined as 6.0 (0.2 mM phosphate buffer), 7.0 (0.2 M phosphate buffer) and 8.5 (200 mM Tris–HCl), respectively. Different stable pH profiles observed with buffalo erythrocyte (G6PD)Citation35, chicken liver (6PGD)Citation37 and rainbow trout (Oncorhynchus mykiss) liver (GR)Citation15.
The KM value for NADP+ was smaller than that for G6P and indicated that the enzyme had a strong affinity towards the coenzyme. Similar conclusions were reported for G6PD from human placentalCitation49 and rainbow trout (Oncorhynchus mykiss) erythrocytesCitation50.
G6PD enzyme is inhibited by NADPH and metabolically controlled with NADPH inhibition. We observed that NADPH efficiently inhibited the G6PD enzyme and inhibition type was determined as uncompetitive. The inhibition type of NADPH on G6PD enzyme from different origin was reported as noncompetitive and competitive, but, the inhibition type on rat lung G6PD is unlike to that in the previous studiesCitation13,Citation16,Citation50,Citation51.
6PG has the lower affinity towards 6PGD when compared with NADP+ since the KM for 6PGA is higher than that for NADP+. Similar results were obtained for 6PGD of rat small intestine and rabbit mammary glandCitation36,Citation52. The rat lung 6PGD was noncompetitively inhibited by NADPH with a Ki of 0.147 ± 0.05 mM. Ki values obtained for NADPH are lower than that in otherCitation53 and higher than that reported in some studiesCitation8,Citation36,Citation52. The inhibition type was same as that obtained from rat erythrocytesCitation36, but different from maize and human brainCitation54,Citation55.
According to KM values found for GSSG and NADPH, the KM value for NADPH is smaller than the one for GSSG. Thus, the affinity of NADPH to the enzyme is greater than GSSG. Similar values were reported for GR from human erythrocyte and bovine liverCitation56,Citation57.
NADP+ has a higher rate of inhibition on the enzyme activities than GSH. Similar results have been reported by othersCitation58,Citation59. Rat lung GR was noncompetitively inhibited by GSH and NADP+. Inhibition type determined for GSH was similar to previous studies, but, inhibition type determined for NADP+ was differentCitation59,Citation60.
The antibiotics are commonly utilized clinically for the treatment of many diseases throughout the world. However, it is well known that drugs have a remarkable toxic effect on the human and experimental animalCitation61. It has been reported that various drugs inhibited as in vivo and in vitro the enzyme activities of G6PD, 6PGD and GRCitation30,Citation43,Citation62. Particularly, IC50 values and Ki constants of levofloxacin and furosemide drugs are significantly low. In some studies, it was reported that two drugs inhibited on the sheep and chicken liver glutathione reductaseCitation37,Citation63 and on human erythrocyte 6-phosphogluconate dehydrogenaseCitation64. Our results detected for furosemide are similar to previous results, but levofloxacin exhibited further higher inhibitory effect than previous reports.
We selected levofloxacin and furosemide with the most effective drugs in vitro experiments for in vivo studies. The results of in vivo experiments showed that levofloxacin exhibited an inhibitory effect on the activities of rat lung 6PGD. The GR activity was inhibited by furosemide, but this drug demonstrated an activation effect on the G6PD activity. The reason that levofloxacin did not show a strong inhibition in vivo might be due to its protein binding property which may result in a decrease in the amount of the drug that reaches to the lungCitation65. It is an important finding that the two drugs did not exhibit a strong inhibitory effect in vivo as they did under in vitro conditions on the enzyme activities essential for both antioxidant defense system and the other metabolic processes.
Declaration of interest
The authors report no conflicts of interest. The authors alone are responsible for the content and writing of this article.
References
- Luzzatto L, Testa U. Human erythrocyte glucose 6-phosphate dehydrogenase: structure and function in normal and mutant subjects. Curr Top Hematol 1978;1:1–70
- Ying W. NAD+/NADH and NADP+/NADPH in cellular functions and cell death: regulation and biological consequences. Antioxid Redox Signal 2008;10:179–206
- Stanton RC. Glucose-6-phosphate dehydrogenase, NADPH, and cell survival. IUBMB Life 2012;64:362–9
- Wu G, Fang YZ, Yang S, et al. Glutathione metabolism and its implications for health. J Nutr 2004;134:489–92
- Poot M, Teubert H, Rabinovitch PS, Kavanagh TJ. De novo synthesis of glutathione is required for both entry into and progression through the cell cycle. J Cell Physiol 2005;163:555–60
- van den Dobbelsteen DJ, Nobel CSI, Schlegel J, et al. Rapid and specific efflux of reduced glutathione during apoptosis induced by anti-Fas/APO-1 antibody. J Biol Chem 1996;271:15420–7
- Ibrahim MA, Ghazy AHM, Salem AMH, et al. Biochemical characterization of buffalo liver glucose-6-phosphate dehydrogenase isoforms. Protein J 2015;34:193–204
- Corpas FJ, García-Salguero L, Barroso JB, et al. Kinetic properties of hexose-monophosphate dehydrogenases. II. Isolation and partial purification of 6-phosphogluconate dehydrogenase from rat liver and kidney cortex. Mol Cell Biochem 1995;144:97–104
- Carlberg I, Mannervik B. Purification and characterization of the flavoenzyme glutathione reductase from rat liver. J Biol Chem 1975;250:5475–80
- Javier Corpas F, García-Salguero L, Peragón J, Lupiáñez J. Kinetic properties of hexose-monophosphate dehydrogenases. I. Isolation and partial purification of glucose-6-phosphate dehydrogenase from rat liver and kidney cortex. Life Sci 1994;56:179–89
- Senturk M, Kufrevioglu OI, Ciftci M. Effects of some antibiotics on human erythrocyte glutathione reductase: an in vitro study. J Enzyme Inhib Med Chem 2008;23:144–8
- Adem S, Comakli V, Kuzu M, Demirdag R. Investigation of the effects of some phenolic compounds on the activities of glucose-6-phosphate dehydrogenase and 6-phosphogluconate dehydrogenase from human erythrocytes. J Biochem Mol Toxicol 2014;28:510–4
- Ceyhan D, Danişan A, Öğüş IH, Özer N. Purification and kinetic properties of 6-phosphogluconate dehydrogenase from rat small intestine. Protein J 2005;24:293–301
- Hu W, Zhi L, Zhuo MQ, et al. Purification and characterization of glucose 6-phosphate dehydrogenase (G6PD) from grass carp (Ctenopharyngodon idella) and inhibition effects of several metal ions on G6PD activity in vitro. Fish Physiol Biochem 2013;39:637–47
- Tekman B, Ozdemir H, Senturk M, Ciftci M. Purification and characterization of glutathione reductase from rainbow trout (Oncorhynchus mykiss) liver and inhibition effects of metal ions on enzyme activity. Comp Biochem Physiol C Toxicol Pharmacol 2008;148:117–21
- Ulusu N, Tandogan B. Characterization of glucose-6-phosphate dehydrogenase purified from lamb kidney cortex. Turk J Biochem 2005;30:178–82
- Morse EE. Toxic effects of drugs on erythrocytes. Ann Clin Lab Sci 1988;18:13–18
- Jacobasch G, Rapoport SM. Hemolytic anemias due to erythrocyte enzyme deficiencies. Mol Aspects Med 1996;17:143–70
- Riedl MA, Casillas AM. Adverse drug reactions: types and treatment options. Am Fam Physician 2003;68:1781–90
- Pirmohamed M, Breckenridge AM, Kitteringham NR, Park BK. Fortnightly review: adverse drug reactions. BMJ 1998;316:1295
- Sultana J, Cutroneo P, Trifirò G. Clinical and economic burden of adverse drug reactions. J Pharmacol Pharmacother 2013;4:S73–7
- Alomar MJ. Factors affecting the development of adverse drug reactions (Review article). Saudi Pharm J 2014;22:83–94
- Adem S, Ciftci M. Purification of rat kidney glucose 6-phosphate dehydrogenase, 6-phosphogluconate dehydrogenase, and glutathione reductase enzymes using 2′,5′-ADP sepharose 4B affinity in a single chromatography step. Protein Expr Purif 2012;81:1–4
- Carlberg I, Mannervik B. Glutathione reductase. Meth Enzymol 1985;113:484–90
- Beutler E. Red cell metabolism manual of biochemical methods. London: Academic Press; 1971
- Bradford MM. A rapid and sensitive method for the quantitation of microgram quantities of protein utilizing the principle of protein dye binding. Anal Biochem 1976;72:248–54
- Laemmli UK. Cleavage of structural proteins during the assembly of the head of bacteriophage T4. Nature 1970;227:680–5
- Lineweaver H, Burk D. The determination of enzyme dissociation constants. J Am Chem Soc 1934;56:658–66
- Reagan-Shaw S, Nihal M, Ahmad N. Dose translation from animal to human studies revisited. FASEB J 2008;22:659–61
- Çiftçi M, Beydemir S, Yilmaz H, Bakan E. Effects of some drugs on rat erythrocyte 6-phosphogluconate dehydrogenase: an in vitro and in vivo study. Pol J Pharmacol 2002;54:275
- Çoban TAK, Çifti M, Küfrevioglu OI. Purification and investigation of some kinetic properties of glucose-6-phosphate dehydrogenase from parsley (Petroselinum hortense) leaves. Prep Biochem Biotechnol 2002;32:173–87
- Carlberg I, Mannervik B. Purification and characterization of glutathione reductase from calf liver. An improved procedure for affinity chromatography on 2′,5′-ADP-sepharose 4B. Anal Biochem 1981;116:531–6
- Dringen R. Metabolism and functions of glutathione in brain. Prog Neurobiol 2000;62:649–71
- Erat M. Purification of human erythrocyte glucose 6-phosphate dehydrogenase and glutathione reductase enzymes using 2′,5′-ADP sepharose 4B affinity column material in single chromatographic step. Protein Expr Purif 2004;34:257–60
- Çiftçi M, Beydemir Ş, Yılmaz H, Altıkat S. Purification of glucose 6-phosphate dehydrogenase from Buffalo (Bubalus bubalis) erythrocytes and investigation of some kinetic properties. Protein Expr Purif 2003;29:304–10
- Beydemir Ş, Çiftçi M, Yilmaz H, Küfrevioğlu ÖI. 6-Phosphogluconate dehydrogenase: purification, characterization and kinetic properties from rat erythrocytes. Turk J Vet Anim Sci 2004;28:707–14
- Erat M. Purification of 6-phosphogluconate dehydrogenase from chicken liver and investigation of some kinetic properties. Prep Biochem Biotechnol 2005;35:53–69
- Holten D. Relationships among the multiple molecular forms of rat liver glucose 6-phosphate dehydrogenase. Biochim Biophys Acta 1972;268:4–12
- Le Trang N, Bhargava KK, Cerami A. Purification of glutathione reductase from gerbil liver in two steps. Anal Biochem 1983;133:94–9
- Maglysh SS, Gorbach ZV, Ostrovskiı̆ IuM. Purification and some properties of molecular forms of 6-phosphogluconate dehydrogenase from rat liver. Biochemistry (Mosc) 1982;47:2035–41
- Shreve DS, Levy HR. On the molecular weight of human glucose 6-phosphate dehydrogenase. Biochem Biophys Res Commun 1977;78:1369–75
- Acan N, Tezcan E. Sheep brain glutathione reductase: purification and general properties. FEBS Lett 1989;250:72–4
- Erat M, Şakiroğlu H, Çiftçi M. Effects of some antibiotics on glutathione reductase activities from human erythrocytes in vitro and from rat erythrocytes in vivo. J Enzyme Inhib Med Chem 2005;20:69–74
- Turkoglu V, Altun M, Ciftci M. Purification and characterization of glucose 6-phosphate dehydrogenase from Lake Van fish (Chalcalburnus tarichii pallas, 1811) liver. J Physiol Biochem 2006;62:155–61
- Beydemir Ş, Yilmaz H, Çiftçi M, et al. Purification of glucose 6-phosphate dehydrogenase from goose erythrocytes and kinetic properties. Turk J Vet Anim Sci 2003;27:1179–85
- Holleschau AM, Rathbun WB. Thermal inactivation study of glutathione peroxidase and glutathione reductase activities in lenses of primates and non-primates. Curr Eye Res 1991;10:221–9
- Lopez-Barea J, Lee CY. Mouse-liver glutathione reductase. Purification, kinetics, and regulation. Eur J Biochem 1979;98:487–99
- Sawa Y, Suzuki K, Ochiai H. Purification and characterization of 6-phosphogluconate dehydrogenase from Phormidium sp. Agric Biol Chem 1985;49:2543–9
- Özer N, Aksoy Y, Ögüs IH. Kinetic properties of human placental glucose-6-phosphate dehydrogenase. Int J Biochem Cell Biol 2001;33:221–6
- Ciftci M, Ciltas A, Erdogan O. Purification and characterization of glucose 6-phosphate dehydrogenase from rainbow trout (Oncorhynchus mykiss) erythrocytes. Vet Med Czech 2004;49:327–33
- Yilmaz H, Ciftci M, Beydemir S, Bakan E. Purification of glucose 6-phosphate dehydrogenase from chicken erythrocytes: investigation of some kinetic properties. Prep Biochem Biotechnol Title 2002;32:287–301
- Betts SA, Mayer RJ. Purification and properties of 6-phosphogluconate dehydrogenase from rabbit mammary gland. Biochem J 1975;151:263
- Scott MJ, Lucchesi JC. Structure and expression of the Drosophila melanogaster gene encoding 6-phosphogluconate dehydrogenase. Gene 1991;109:177–83
- Weisz KS, Schofield PJ, Edwards MR. Human brain 6-phosphogluconate dehydrogenase: purification and kinetic properties. J Neurochem 1985;44:510–17
- Bailey-Serres J, Nguyen MT. Purification and characterization of cytosolic 6-phosphogluconate dehydrogenase isozymes from maize. Plant Physiol 1992;100:1580–3
- Worthington DJ, Rosemeyer MA. Human glutathione reductase: purification of the crystalline enzyme from erythrocytes. Eur J Biochem 1974;48:167–77
- Ulusu NN, Tandoğan B. Purification and kinetic properties of glutathione reductase from bovine liver. Mol Cell Biochem 2007;303:45–51
- De Lamotte F, Vianey-Liaud N, Duviau MP, Kobrehel K. Glutathione reductase in wheat grain. 1. Isolation and characterization. J Agric Food Chem 2000;48:4978–83
- Erat M, Sakiroglu M, Ciftci M. Purification and characterization of glutathione reductase from bovine erythrocytes. Prep Biochem Biotechnol 2003;33:283–300
- Ulusu G, Erat M, Çiftçi M, et al. Purification and characterization of glutathione reductase from sheep liver. Turk J Vet Anim Sci 2005;29:1109–17
- Andrade RJ, Tulkens PM. Hepatic safety of antibiotics used in primary care. J Antimicrob Chemother 2011;66:1431–46
- Adem S, Ciftci M. Effects of some drugs on human erythrocyte 6-phosphogluconate dehydrogenase: an in vitro study. J Enzyme Inhib Med Chem 2007;22:751–4
- Erat M, Çiftçi M. In vitro effects of some antibiotics on glutathione reductase from sheep liver. J Enzyme Inhib Med Chem 2003;18:545–50
- Akyüz M, Erat M, Çiftçi M, et al. Effects of some antibiotics on human erythrocyte 6-phosphogluconate dehydrogenase: an in vitro and in vivo study. J Enzyme Inhib Med Chem 2004;19:361–5
- Fish DN, Chow AT. The clinical pharmacokinetics of levofloxacin. Clin Pharmacokinet 1997;32:101–19