Abstract
Objective. Examination of the amniotic fluid (AF) proteome has been previously attempted to identify useful biomarkers in predicting the outcome of preterm labor (PTL). Isobaric Tag for Relative and Absolute Quantitation (iTRAQ™) labeling allows direct ratiometric comparison of relative abundance of identified protein species among multiplexed samples. The purpose of this study was to apply, for the first time, the combination of iTRAQ and tandem mass spectrometry to identify proteins differentially regulated in AF samples of women with spontaneous PTL and intact membranes with and without intra-amniotic infection/inflammation (IAI).
Methods. A cross-sectional study was designed and included AF samples from patients with spontaneous PTL and intact membranes in the following groups: (1) patients without IAI who delivered at term (n = 26); (2) patients who delivered preterm without IAI (n = 25); and (3) patients with IAI (n = 24). Proteomic profiling of AF samples was performed using a workflow involving tryptic digestion, iTRAQ labeling and multiplexing, strong cation exchange fractionation, and liquid chromatography tandem mass spectrometry. Twenty-five separate 4-plex samples were prepared and analyzed.
Results. Collectively, 123,011 MS2 spectra were analyzed, and over 25,000 peptides were analyzed by database search (X!Tandem and Mascot), resulting in the identification of 309 unique high-confidence proteins. Analysis of differentially present iTRAQ reporter peaks revealed many proteins that have been previously reported to be associated with preterm delivery with IAI. Importantly, many novel proteins were found to be up-regulated in the AF of patients with PTL and IAI including leukocyte elastase precursor, Thymosin-like 3, and 14-3-3 protein isoforms. Moreover, we observed differential expression of proteins in AF of patients who delivered preterm in the absence of IAI in comparison with those with PTL who delivered at term including Mimecan precursor, latent-transforming growth factor β-binding protein isoform 1L precursor, and Resistin. These findings have been confirmed for Resistin in an independent cohort of samples using ELISA. Gene ontology enrichment analysis was employed to reveal families of proteins participating in distinct biological processes. We identified enrichment for host defense, anti-apoptosis, metabolism/catabolism and cell and protein mobility, localization and targeting.
Conclusions. (1) Proteomics with iTRAQ labeling is a profiling tool capable of revealing differential expression of proteins in AF; (2) We discovered 82 proteins differentially expressed in three clinical subgroups of premature labor, 67 which were heretofore unknown. Of particular importance is the identification of proteins differentially expressed in AF from women who delivered preterm in the absence of IAI. This is the first report of the positive identification of biomarkers in this subgroup of patients.
Introduction
‘High-dimensional biology’ refers to the use of high throughput techniques that allow simultaneous examination of changes in the genome (DNA), transcriptome (mRNA), proteome (proteins), metabolome (metabolites), lipidome (lipids), glycome (carbohydrates), and other biochemical components in a biological sample. The goal of such approaches is to improve understanding of the physiology and mechanisms of disease, [Citation1–3] to identify biomarkers for diagnostic or prognostic purposes, as well as to develop therapeutic tools [Citation4–45]. A critical aspect of high-dimensional biology is intelligent mining of complex data sets generated with the use of these techniques, collectively referred to as ‘omics’ sciences. Thus, computational biology is an integral part of high-dimensional biology.
Examination of the amniotic fluid (AF) proteome has been employed as a means to identify biomarkers for spontaneous preterm labor (PTL) and other obstetrical disorders [Citation13,Citation16–18,Citation31,Citation44,Citation45]. Mass spectrometry-based proteomics falls into two broad categories: ‘top-down’ and ‘bottom-up’ [Citation46]. In the former, intact proteins are characterized directly via mass spectrometry, whereas in the latter, proteins are first digested, and the resulting peptides are then analyzed to identify and sometimes to quantify proteins (the ‘bottom-up’ approach is frequently used with liquid chromatography). Although Michaels et al. [Citation47] have pioneered the use of ‘bottom-up’ proteomics of AF, most approaches to date have used a ‘top-down’ methodology for analysis of AF. In fact, although the digestion step produces many more fragment products than the original number of proteins, peptides can be more easily and accurately identified in the mass spectrometer in comparison with the intact proteins in the top-down approach. The main disadvantages of the bottom-up approach are that (a) the re-assembly of peptides to deduce their parent proteins is a complex computational task, and (b) quantitation of the proteins is less straightforward. In practice, a ‘bottom-up’ workflow tends to be lower-throughput than top-down counterparts.
Isobaric Tag for Relative and Absolute Quantitation (iTRAQ™) is a new technique applicable to ‘bottom-up’ mass spectrometry [Citation48–50]. Isobaric labeling refers to the derivatization of primary amino groups of peptides in iTRAQ. These isobaric tags do not differ in mass, and upon fragmentation of the isotope encoded reporter ions from the peptides during mass spectrometry, relative quantitative information of each tag can be measured. In iTRAQ, there are four distinct labels. Each is comprised of a ‘reporter’ element and a ‘balance’ element. The reporters have approximate masses of 114–117 Da, whereas their corresponding balances have masses of 28–31 Da such that they sum to about 145 Da (hence the term ‘isobaric’). In the first stage of tandem mass spectrometry, a peptide species labeled with any of the four reagents will appear at the same mass-to-charge ratio (m/z), whereas at the second stage of mass spectrometry, the labile bond between the reporter and balance elements is broken, producing distinct reporter ion peaks at 114–117 Da. The relative abundance of peptides labeled with the four reagents is directly indicated by the heights of these four peaks. Moreover, as several samples are combined and analyzed together, throughput is substantially increased compared with the analysis of unlabeled samples, which must be accomplished one by one. Thus, although there are ‘bottom-up’ approaches that do not involve the use of labeling reagents, the two-fold advantages of simpler quantitation and higher throughput due to multiplexing are apparent.
The aim of the present work was to apply, for the first time, iTRAQ-based proteomic analysis of AF of patients with spontaneous PTL with intact membranes to identify differentially expressed proteins in the presence or absence of intra-amniotic infection/inflammation (IAI).
Material and methods
Study design and population
A cross-sectional study was designed by searching our clinical database and bank of biological samples, selecting AF samples from 75 patients with an episode of spontaneous PTL and intact membranes who were classified into: (a) PTL without IAI who delivered at term (n = 26); (b) PTL without IAI who delivered preterm (<37 weeks gestation) within 7 days of amnniocentesis (n = 25); and (c) PTL with IAI (n = 24).
All women provided written informed consent prior to the collection of AF. The collection of AF and its utilization for research purposes were approved by the Institutional Review Boards of Sotero del Rio Hospital (Santiago, Chile) and the Eunice Kennedy Shriver National Institute of Child Health and Human Development, NIH, DHHS. Many of these samples have been previously used to study the biology of inflammation, hemostasis, metabolomics, proteomics, and growth factor concentrations in normal pregnant women and those with pregnancy complications.
Definitions
Spontaneous PTL was defined by the presence of regular uterine contractions occurring at a frequency of at least two every 10 min associated with cervical change before 37 completed weeks of gestation that required hospitalization. Intra-amniotic infection was defined as a positive AF culture for micro-organisms. Intra-amniotic inflammation was diagnosed by an AF interleukin (IL)-6 concentration > 2.6 ng/ml [Citation51].
Sample collection
AF samples were obtained from transabdominal amniocenteses performed for evaluation of microbial status of the amniotic cavity. Samples of AF were transported to the laboratory in a sterile capped syringe and cultured for aerobic/anaerobic bacteria and genital mycoplasmas. White blood cell count, glucose concentration, and Gram-stain were also performed shortly after collection as previously described [Citation52–54]. The results of these tests were used for clinical management. AF IL-6 concentrations were used only for research purposes. AF not required for clinical assessment was centrifuged at 1300 g for 10 min at 4°C and the supernatant was aliquoted and stored at −70°C until analysis.
Overview of the experimental workflow
The basic experimental strategy is to digest AF proteins, identify each peptide in the resulting mixture and measure their concentrations relative to corresponding peptides in a reference sample, and then to compute the relative concentration of the digested proteins from their daughter peptides. From this information, differentially regulated proteins among the three clinical groups can be identified.
illustrates schematically the workflow used to implement this strategy. First, a pooled reference sample was created by mixing a small amount of protein from each of the AF samples. This mixture was then separated into replicate aliquots. Samples were depleted of albumin and Immunoglobulin G (IgG) so that proteins at lower concentrations would be unmasked and therefore more easily detected. Individual samples were digested with trypsin. The resulting tryptic peptides were then labeled with one of three iTRAQ isobaric labeling reagents [each reagent produces a tandam mass spectrometry (MS/MS) reporter ion at 115, 116, or 117 Da], whereas an aliquot of the reference pool was always labeled with the iTRAQ reagent producing a 114 Da reporter ion. Groups of four samples, comprising the pooled reference sample (114) and three individual samples (115, 116, and 117), were combined together into a “4-plex.” Each of these multiplexed mixtures was then subjected to strong cation exchange (SCX) fractionation in order to simplify the peptide mixture for subsequent analysis. Each of the SCX fractions was subjected to liquid chromatography MS/MS (LC-MS/MS) analysis. The eluent from the LC column was spotted onto matrix-assisted laser desorption ionization (MALDI) target plates, and subsequently subjected to tandem mass spectrometry analysis (MS/MS). In the first stage of mass spectrometry, a precursor ion has the same mass from all four samples in the mix due to the fact that the iTRAQ reagents are constructed with a reporter unit and a balance unit, the sum of whose masses is the same for all four of the reagents. However, upon collisional dissociation for MS/MS analysis, the reporter unit produces a low-mass peak whose intensity indicates the relative amount of peptide in the mixture that was labeled with the corresponding reagent. Thus, the MS/MS spectrum contains two kinds of information: (a) a ladder of peaks characteristic of the peptide sequence that is used to identify the peptide, and (b) the four reporter ion peaks at 114–117 Da that are used to quantify the relative amount of peptide from the four samples in the mix. The following sections describe in detail the experimental workflow (summarized in ).
Figure 1. Diagram of the iTRAQ workflow. The sequence of the workflow is indicated by the arrows in the right side. Pooled reference: Small aliquots from each of the 75 samples of amniotic fluid were pooled to create a reference sample. Deplete-concentrate-reduce-block: The amniotic fluid samples were depleted of albumin and IgG, subsequently concentrated and reduced. Digestion: The samples were subjected to trypsin digestion. Labeling: The reference sample and three individual samples (denoted A, B, and C in the figure) were labeled with iTRAQ reagents (the numbers 114–117 refer to the reporter mass of the individual iTRAQ reagent). Mixing and fractionation: The four labeled samples were mixed and subjected to strong cation exchange (SCX) fractionation to simplify the mixture prior to conducting liquid chromatography and mass spectrometry (LC-MS/MS). Each SCX fraction was subjected to LC-MS/MS. Liquid chromatography: Proteins were separated by liquid chromatography. The retention time is displayed in the horizontal axis. MS1: The first stage of mass spectrometry for a specific retention time is indicated schematically. Each peak in the mass spectrum (horizontal axis is m/z) corresponds to an individual peptide. MS2: A single peak is selected for collisional dissociation and the fragments subjected to a second stage mass spectrometry (tandem MS/MS). The reporter ions from the iTRAQ label appear in the low mass range, distinct from peptide fragment peaks. The pattern of peaks in the final mass spectrum is matched to a database in order to identify the parent peptide. The intensities of the reporter ion peaks indicate the relative abundance of the four samples.
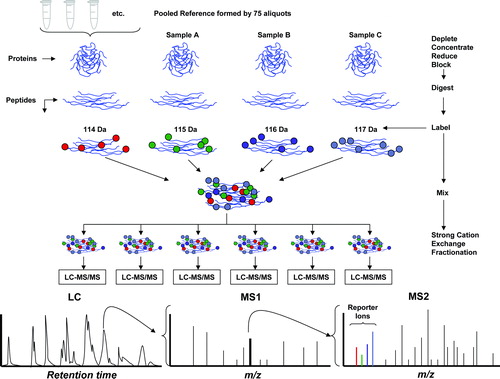
Amniotic fluid protein extraction and digestion [Citation55]
AF samples were depleted of albumin using Vivapure immunoaffinity anti-human serum albumin capture resins (Satorius Stedium, Bohemia NY, USA) and IgG with ultralink immobilized protein G (Pierce, Rockford, IL, USA). Protein concentrations of individual depleted AF samples were determined with bicinchoninic acid protein assay kit (Pierce, Rockford, IL, USA). Forty micrograms of total protein from each sample was precipitated by incubation with 4.5 ml of chilled acetone at 4°C for 3 h, followed by centrifugation (3000 rcf) for 30 min. Triethylammonium bicarbonate (0.5 M) and 2% sodium dodecylsulfate were added to dissolve the protein pellet before mixing it with a reducing reagent [50 mM Tris-(2-carboxyethyl) phosphine]. The reduction was completed by incubating the solution at 60°C for 1 h. The reduced cysteine residues were then blocked with 200 mM of methyl methanethiosulfonate, at room temperature for 10 min. Trypsin digestion of proteins in each sample was performed with 10 μg of trypsin (1 μg/μl) (Worthington Biochemical Corp., Lakewood, NJ, USA) in the presence of CaCl2 overnight at 37°C.
Reference pool and quality control
To generate a pooled sample as a reference standard for each iTRAQ 4-plex, 17 μg of each depleted sample was combined into a pool which was then separated into 25 aliquots of 40 μg each. Each of these pooled reference standards was subsequently processed as described above.
Internal protein concentration standards were provided for quality control by spiking known amounts of non-human protein into each individual sample, including the pooled reference standard samples. Two micrograms of Phage T4 (New England BioLabs, Ipswich, MA, USA) and 0.2 μg of Escherichia coli RecA (New England BioLabs, Ipswich, MA, USA) was added into each sample of 40 μg of AF protein [Citation56,Citation57].
Labeling with multiplex reagents
Seventy microliters of iTRAQ reagent (isobaric reagents 114, 115, 116, and 117 from Applied Biosystems, Framington, MA, USA) in ethanol was added to each sample and incubated at room temperature for 1 h. Following this derivatization step, the four samples were mixed, dried, and re-suspended in 3 ml of SCX loading buffer [25% v/v acetonitrile (ACN), 10 mM KH2PO4, pH 3.0, with phosphoric acid]. In each mix, the reference pool was labeled with the 114 reagent. For the other three reagents, individual samples were randomly assigned such that each mix contained one sample from each of the three clinical groups (except that one mix contained two samples from the term delivery group and none from the group with IAI, due to the unequal numbers of samples in the three groups).
Strong cation exchange chromatography
Each peptide mixture was separated on an SCX chromatography column (Polysulfoethyl column, The Nest Group, Southborough, MA). The column was first equilibrated with SCX loading buffer. The pH of the iTRAQ labeled sample was verified to be less than 3.3. Additional loading buffer was added if necessary to adjust the pH. The sample was then loaded at a rate of ≈1 drop/s onto the column and washed with 1 ml of SCX loading buffer to remove salts, trys-(2-carboxyethyl) phosphene and unincorporated iTRAQ reagent. Peptides were eluted with a linear gradient of 0–1000 mM KCl (in 25% v/v ACN, 10 mM KH2PO4, pH 3.0). Six pooled fractions of the elutant were collected for analysis with LC-MS/MS.
Liquid chromatography
The SCX fractions were re-suspended in 40 μl of high performance liquid chromatography (HPLC) loading buffer (94.9%:5%:0.1% water:acetonitrile: trifluoroacetic acid containing 2 mM ammonium acetate). Twenty-five microliters of each fraction were injected on an UltiMate 3000 Chromatography System equipped with a Probot fraction collector (Dionex LC-Packings, Hercules, CA). HPLC fractions were spotted directly onto the MALDI target. An integrated syringe pump on the fraction collector enabled the mixing of the HPLC eluent and MALDI matrix prior to deposition onto the MALDI plate. To ensure good mass calibration of the MALDI MS spectra, an internal molecular mass standard [ACTH (18–39) peptide; MH+ = 2465.199 Da] was added to the matrix solution at a concentration of 400 femtomole/μl. Peptides were separated over a 35-min elution gradient and spotted onto the MALDI target at intervals of 30 s. Protein elution from the column was monitored with ultraviolet light at a wavelength of 214 nm using a 45-nl flow cell. Prior to each set of six HPLC injections representing an iTRAQ mix, a mixture of peptide standards (Michrom Bioresources, Auburn, CA, USA) was injected to monitor the consistency of HPLC retention times. Each HLPC run was spotted in a 6 × 35 spot format (210 fractions) such that three injections were accommodated on a single MALDI plate. Thus, two such plates were required to collect the chromatographic separation of each complete iTRAQ mix represented in six SCX fractions.
Mass spectrometry
Mass spectrometry analysis was performed with a Sciex/AB 4800 MALDI MS/MS instrument (MDS/Sciex, Concord, Ontario, Canada). First, LC-MS data were collected from all the six HPLC injections. To ensure the accuracy of mass measurements, two internal standards were used for mass calibration, a 2465.199 Da peptide standard that was co-infused with the matrix during deposition onto the MALDI target, and a 568.136 Da matrix trimer ion. The settings for acquisition and processing methods in the Sciex/AB 4800 software were optimized for the reliable detection of monoisotopic peaks to slightly below the intensity threshold to be used later for MS/MS precursor selection. This threshold was important for the correct distinction of useful components from chemical noise.
In order to maximize MS/MS performance and efficiency, a custom MS/MS precursor selection program was used to generate a non-redundant precursor list from the two dimensional chromatography space ensuring that each precursor was analyzed from the SCX-HPLC fraction from which the best quantification was possible. In order to maintain the consistency of precursor selection across all iTRAQ mixes, exclusion and inclusion lists were generated and dynamically updated for the next mix in the queue. Precursor ions on these lists were identified through their accurate mass, HPLC retention time and SCX fraction number and categorized as: (a) precursors suitable for quantification (fully alkylated, fully iTRAQ-labeled peptides) – constituting the inclusion list; (b) precursors not suitable for quantification (incomplete alkylation/iTRAQ labeling, chemical by-products, peptides from trypsin, or unidentified precursors) – constituting the exclusion list; and (c) opportunistic precursors that match precursor selection criteria but were not included in either list. Opportunistic precursors that could be identified in Mascot searches against the human Uniref protein database were used to build and update the inclusion and exclusion lists depending on how they matched to criteria (a) and (b).
Mass spectrometry data were acquired in two phases. The first phase started with the acquisition of data from the first iTRAQ mixture with empty inclusion and exclusion lists. Precursors identified from this mixture were sorted into the inclusion and exclusion lists using the criteria previously described. The remaining mixtures were then run sequentially with the dynamically updated exclusion and inclusion lists. Items on the inclusion list were preferentially selected for MS/MS, whereas items on the exclusion list were not selected. The exclusion and inclusion lists continuously grew until the last iTRAQ mixture in the queue was analyzed.
A second phase of mass spectrometry analysis was performed in order to ensure a uniform likelihood for the detection of precursors across all 25 4-plexes. In each 4-plex, precursors were targeted for acquisition that had been added to the inclusion list later during the first phase. Also, precursors that yielded poor signal-to-noise for quantification in the first phase were re-analyzed with a longer signal integration time in the second phase (increasing the number of laser shots to about 5000, as compared to about 2000 during the first phase). By completing the two phases, a highly overlapping set of proteins were measured in each iTRAQ 4-plex.
Data management
From each LC-MS/MS run in the Sciex/AB 4800 database, MS/MS peak lists were extracted (limited to the m/z region above 100 Da) using the instrument software. Ninety-four peaklist files were generated. Typically, each 4-plex was represented by four files (two sets of SCX fractions: 1–3 and 4–6, and for each of these two spectrum acquisition passes). Peaklist files and associated experimental data were indexed into a MySQL database to facilitate data management. Custom-written Perl programs were used to perform database queries to: (a) extract and format peaklist files for input to protein identification engines; (b) extract and normalize reporter ion intensities for each peptide; and (c) correlate quantitation and identification information.
Protein identification
A single large peaklist file was extracted from the database as input for protein identification. All MS/MS spectra were analyzed using Mascot software version 2.2.03 (Matrix Science, London, UK) and X!Tandem version 2007.01.01.2 (www.thegpm.org). X!Tandem was set up to search the ipi. HUMAN database (version 3.28, 136040 entries) with reverse search included. All searches were conducted assuming trypsin cleavage. The same was the case for Mascot searches. Mascot and X!Tandem searched the database with a fragment ion mass tolerance of 0.40 Da and a parent ion tolerance of 100 PPM. Fixed modifications specified for both Mascot and X!Tandem included MMTS on cysteine residues and iTRAQ modification of lysine and the N-terminus. Oxidation of methionine and iTRAQ modification of tyrosine were specified for both search engines as variable modifications.
Scaffold (version 01.07.00, Proteome Software, Portland, OR) was used to validate MS/MS based peptide and protein identifications. Peptide identifications were accepted if they could be established at greater than 95.0% probability as specified by the Peptide Prophet algorithm [Citation58]. Protein identifications were accepted if they could be established at greater than 95.0% probability and contained at least two identified peptides. Protein probabilities were assigned by the Protein Prophet algorithm (which takes into account the Mascot and X!Tandem probabilities) [Citation59]. Proteins that contained similar peptides and could not be differentiated based on MS/MS analysis alone were grouped to satisfy the principles of parsimony. De novo sequencing using PEAKS Software (Bioinformatics Solutions, Inc., Waterloo, Ontario, Canada) was used to confirm peptide identity for precursors not identified by database search, yet determined to be of interest in differential expression analyses [Citation60].
Quality control
Tryptic peptides derived from the spiked-in quality-control proteins Phage T4 and E. coli recA were identified by database search with X!Tandem. Mean reporter ion ratios for these peptides relative to the pooled reference 114 reporter ion were computed for each sample individually. Because the concentrations of the spiked standards were the same for pooled reference and experimental samples, these ratios should theoretically be near 1.0. AF samples whose mean spike ratios deviated by more than 50% from unity were excluded from further consideration (seven from patients with PTL who delivered at term, four from patients who delivered preterm without IAI, and three from patients who delivered preterm with IAI).
Determination of differential expression
Statistical analysis
To compensate for experimentally induced variation in total peptide concentrations, a global median normalization (or standardization) was performed such that the median of the sample/reference ratios was unity [Citation61,Citation62]. Relative peptide expression values were determined by computing the ratio of the peak intensity for each reporter ion corresponding to an individual sample to that of the corresponding reference pool reporter ion. MS/MS spectra in which no reference reporter ion at 114 Da was observed were excluded from the determination of relative abundance.
The amount of an identified protein in a sample relative to the reference pool was determined by computing the median of all of the relative peptide expression values observed in a sample corresponding to that protein. For pair-wise comparisons among the three clinical groups, the median of the individual sample protein expression values was formed for each group and the ratio of medians represented the relative protein expression ratio. p-values were computed with the Mann–Whitney rank sum test. A p-value <0.05 was considered significant.
Analysis for over-representation of biological processes
An analysis was undertaken to determine which biologic processes were overrepresented in patients with PTL as a function of the proteins identified to be differentially regulated. Gene ontology (GO) terms that were enriched among the set of differentially regulated proteins in IAI relative to all proteins identified in all samples in the present study. We used BiNGO (version 2.0) [Citation63] to calculate GO term enrichment, and CytoScape (version 2.5.1) [Citation64] to visualize the resulting network of GO biological processes. We then used the IPIhuman database version 3.35 to convert protein references to corresponding gene names. Redundant gene references were removed in order to avoid bias due to multiple protein database references to the same gene. Distributions were tested for statistical significance using the hypergeometric test (equivalent to an exact Fisher test [Citation65]), and the Benjamini and Hochberg [Citation66] correction method for false discovery rate (FDR) was applied. GO terms that were enriched at the 95% confidence level after correction for multiple comparisons were accepted as significant.
Results
The demographic and clinical characteristics of the patients included in this study are described in . The gestational age at amniocentesis did not differ significantly among the groups. Among patients with PTL who delivered preterm, gestational age at delivery was not significantly different between patients with and without IAI.
Table I. Demographic and clinical characteristics of patients with spontaneous preterm labor with intact membranes.
Protein identification
A total of 123,011 MS/MS spectra were acquired across the two mass spectrometry phases from 25 iTRAQ 4-plex mixtures, representing AF samples from 75 patients. Of these spectrum observations, 26,801 spectra produced confident peptide identifications. Three hundred nine distinct proteins were identified with greater than 95% confidence and at least two distinct identified peptides, with an estimated FDR of 1.06%. Additionally, 16 proteins (S100A12, RETN, FK506, HIST1H1D, YWHAB, BASP1, SERPINB1, ARHGDIA, PGD, MRLC2, CTSL1, FKBP1A, NME1, YWHAQ, CALRETICULIN, and CPM) which were provisionally identified by database searching based on one peptide each were validated by de novo sequencing and subsequently included in the differential expression analysis.
Differential regulation: preterm delivery with intra-amniotic infection/inflammation
catalogs proteins that have been reported in the literature to be differentially regulated in the AF of women with preterm delivery in the presence of IAI. lists proteins which were determined in the present study to be differentially regulated between patients who delivered preterm with infection and/or inflammation in comparison with those in the remaining two groups. Criteria for inclusion in were: (a) confirmed protein identity; (b) p-value for differential expression of the protein of less than 0.05; and (c) the fold-change in relative abundance for the protein between the two groups of at least 1.5. Seventy-seven proteins were found to be up-regulated in the AF of patients with IAI, but only six were down-regulated. All except five of the proteins listed in that had previously been determined to be associated with infection/inflammation related PTL and delivery are found in . Moreover, one of those (azurocidin) that does not appear in nonetheless was detected, identified and was differentially regulated, but was excluded from the Table because it was detected in a number of samples insufficient to generate statistical significance.
Table II. Proteins previously reported in the literature to be differentially up-regulated in the amniotic fluid of patients with IAI.
Table III. Proteins differentially expressed in amniotic fluid samples of patients with preterm delivery with IAI, compared with patients without IAI who delivered at term.
Differential expression: preterm delivery without intra-amniotic infection/inflammation
lists proteins which were determined to be differentially regulated between patients who delivered preterm without IAI in comparison to those of patients with PTL without IAI that delivered at term. Four proteins were found to be up-regulated in patients in PTL/delivery without IAI: Resistin (RETN), Thymosin-like 3 (TMSL3), Antileukoproteinase (SLPI), and Growth-inhibiting protein 12 (also known as Lactoferrin, LTF). Two proteins were down-regulated: latent-transforming growth factor β-binding protein isoform 1L (LTBP1) and Mimecan precursor (OGN).
Gene ontology analysis
The subset of genes listed in Table III (n = 83) was compared for enrichment against the basis set of genes (n = 447) corresponding to the proteins identified in the entire experiment. shows the resulting network. Colored nodes indicate GO terms that are significantly enriched.
Table IV. Proteins differentially expressed in amniotic fluid samples of patients with preterm delivery without IAI, compared with patients who delivered at term.
Discussion
Principal findings of this study
(1) Unbiased analysis using liquid chromatography-tandem mass spectrometry with isobaric labeling (iTRAQ) of the AF proteome of women with PTL identified proteins differentially regulated in women with IAI and in those without IAI who delivered preterm; (2) iTRAQ identified many proteins not previously known to be differentially expressed in cases of IAI and confirmed the differential expression of many proteins previously reported in the context of hypothesis-driven research; (3) importantly, this proteomic approach (‘bottom-up’) allowed the identification for the first time of candidate proteins which are differentially regulated in women with PTL leading to preterm delivery in the absence of IAI; and (4) a systems biology approach indicated that two biological processes – actin cytoskeleton organization biogenesis and protein targeting – were found to be prevalent in the AF of patients with PTL and IAI, a novel observation with biological implications. Collectively, these observations suggest that a ‘bottom-up’ proteomic experimental strategy is a powerful approach for the discovery of potential biomarkers in PTL as well as to gain an understanding in the mechanisms of disease.
‘Top-down’ or ‘bottom-up’ proteomic approaches for the analysis of amniotic fluid proteome in preterm labor?
The term ‘top-down’ proteomics refers to the analysis of intact proteins in biological matrices [Citation46]. The general approach consists in detecting differentially regulated proteins between two clinical conditions (health vs. disease) followed by identification of such proteins or peptides. This method has been successfully used in identifying biological markers in PTL with IAI [Citation18]. The main challenge in ‘top-down’ proteomics in the absence of front-end separation of complex protein mixtures is protein identification which is often unsuccessful [Citation46]. To address this challenge, ‘bottom-up’ proteomics was developed [Citation67]. It reverses the treatment of the problem by identifying proteins first, and then asking the question: ‘which of the identified proteins is differentially regulated between clinically-relevant subgroups’. This is accomplished by digestion of proteins in the biological matrix, peptide identification using tandem mass spectrometry, and determination of differentially regulated proteins among two or more clinical conditions. This approach overcomes the main bottleneck of ‘top-down’ approaches, namely protein identification. Moreover, the recent addition of isobaric labeling technologies [Citation48–50] (e.g. iTRAQ) to ‘bottom-up’ approaches facilitates both the throughput and quantitation capabilities of LC-MS/MS based studies, and has recently been used successfully to identify biomarkers [Citation62,Citation68–73]. This report represents the first utilization of iTRAQ-based LC-MS/MS approach to characterize the differential expression of proteins in the AF of patients with PTL.
Proteins differentially regulated in intra-amniotic infection/inflammation
We have found a large number of proteins that are differentially regulated (down as well as up) in the AF of women with IAI. This includes most of the proteins previously identified via hypothesis-driven research [Citation13,Citation18,Citation45,Citation74–82], listed in . We interpret this recapitulation of the results of many hypothesis-driven experiments as a validation of the previous findings with an unbiased, discovery-based proteomic approach. Importantly, we also identified differential regulation of many proteins which have not been previously implicated in the process of IAI. These findings can serve as the basis to determine the clinical value of each protein, or a proteomic “fingerprint” formed by multiple proteins, as possible biomarkers. Moreover, it opens new lines of investigation to explore the role of each newly identified protein in this study in preterm parturition.
Gene ontology enrichment analysis
The breadth and scope of the present findings allow for the first time a systems-biology approach to the proteomic characterization of AF of patients with PTL with IAI. Four main branches are apparent in this network, which are labeled ‘host defense’, ‘mobility, localization and targeting’, ‘anti-apoptosis’, and ‘metabolism/catabolism’. Within each of these branches there are clusters of GO terms that are significantly enriched and functionally inter-related ().
Figure 2. A description of the gene ontology biological processes over-represented in the amniotic fluid of women with spontaneous preterm labor in the presence of intra-amniotic infection/inflammation. (A) Pie chart that displays the main four gene ontology terms that were significantly enriched in the amniotic fluid of women with preterm labor with IAI: (1) metabolism/catabolism; (2) mobility, localization and targeting; (3) anti-apoptosis; and (4) host defense. (B) The four main branches in the network diagram represent four gene ontology terms described in the pie chart. Color nodes represent the biological processes that are significantly over-represented (the darker the color, the greater the significance is). The open nodes represent biological processes that did not reach statistical significance. As described in detail in the text, BiNGO (version 2.0) was used to calculate GO term enrichment, and CytoScape (version 2.5.1) was used to visualize the resulting network of GO biological processes.
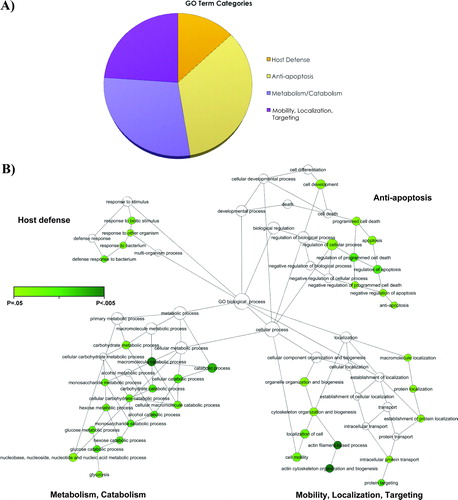
Figure 3. Clusters of biological processes identified in the main four gene ontology terms. The four pie charts represent the main gene ontology terms over-represented in the amniotic fluid of women with spontaneous preterm labor in the presence of intra-amniotic infection/inflammation. Each pie chart displays clusters of biological processes that are significantly enriched and functionally inter-related.
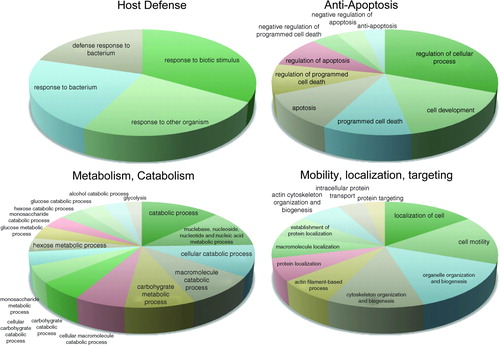
Metabolism and catabolism
Parturition is an extremely energy-intensive process, so it is not surprising that biological processes relating to energy production are overrepresented. There are 26 gene products (Supplementary Table S1) which were identified to participate in the metabolism/catabolism cluster in . Among these, there are several that appear in at least 15 of the 16 significantly enriched nodes of this branch. They include α-enolase, triosphosphate isomerase, 6-phosphogluconate dehydrogenase (decarboxylating), pyruvate kinase isozymes M1/M2, fructose-bisphosphate aldolase A, 6-phosphogluconolactonase, malate dehydrogenase (cytoplasmic), and phosphoglycerate kinase 1. It is interesting that this cluster converges on glycolysis, whose importance in energy production is self-evident (See ). The precise reason for the over-expression of these proteins in AF requires further study. It may be expected that the over-expression would occur in the myometrium, cervix, membranes, and the human fetus. Thus, the change in expression in AF may reflect the activity in membranes and the human fetus, among other sites.
Mobility, localization, and targeting
There are two sub-clusters within this branch of . One sub-cluster is related to protein localization and targeting and the second sub-cluster is related to cell mobility and localization. The term ‘targeting’ in GO refers to the process of locating specific proteins to particular membrane-bounded subcellular organelles, such as the peroxisome, and it usually requires an organelle specific protein sequence motif. It is not surprising that host response to infection or inflammation, as well as parturition, requires the re-orientation/re-organization of proteins within the cell.
The protein localization and targeting sub-cluster (Table S2) includes α-filamin-A, myosin-9 polymeric-immunoglobulin receptor, and a group of 14-3-3 family proteins. The finding that this group also includes PDIA3 (protein disulfide isomerase family A, member 3) suggests that endoplasmic reticulum (ER) stress [Citation83] may be an additional biological process implicated in PTL associated with IAI leading to preterm parturition. ER stress results from the accumulation of unfolded/misfolded proteins in the ER as a consequence of an imbalance between the protein-folding load and the capacity of the ER to fold them [Citation84]. PDIA3 protein interacts with lectin chaperones, calreticulin and calnexin, to modulate folding of newly synthesized glycoproteins [Citation85]. PDIA3 participates in electron transport within the mitochondria and can lead to the H2O2 generation during the process of proteins folding. In cases of inflammation, increased activity of this pathway leads to enhanced H2O2 generation and oxidative stress [Citation86]. A relationship between ER stress and inflammation is now well recognized [Citation84]. Yet, this study represents the first evidence of a link between ER stress and preterm parturition.
The second sub-cluster (Table S2) relates to cell mobility and localization, and includes actin as well as actin-interacting species such as cytoplasmic actin 1, α-actinin-4 and -1, myosin-9, macrophage migration inhibitory factor, macrophage capping protein, integrin α-M and plastin-2. Also prominent in this sub-group is a set of gene products including Calgranulin B, Vinculin, Profilin-1, Cofilin-1, Leukocyte elastase and Moesin.
Anti-apoptosis
A cluster of anti-apoptotic proteins (Table S3) was overrepresented in the AF of women with PTL and IAI. This observation represents the first report that this pathway is active in PTL associated with IAI. Among the proteins participating in this pathway are: myeloperoxidase, heat shock protein HSPA1B, two members of the 14-3-3 family, Cofilin-1, Glutathione S-transferase (GST), Rho GDP association inhibitor, and macrophage migration inhibitory factor.
Why should gene products involved in anti-apoptosis be over-expressed in the AF in women who have IAI? A possible explanation is that IAI is characterized by an influx of neutrophils into the amniotic cavity in the context of infection [Citation54,Citation74,Citation87–96], and that such neutrophils play a key role in host defense. It is now well-established that neutrophils engaged in host protection undergo delayed apoptosis [Citation97–100]. This allows prolonged survival of neutrophils favoring an increased number and life span of these key cells. It is interesting that several of the proteins that are identified through GO as being involved in promoting anti-apoptosis also play a role in the regulation of oxidative stress. For example, myeloperoxidase has anti-apoptotic properties [Citation101], and also participates in the generation of reactive oxygen species by neutrophils [Citation102]. The latter is reflected in GO by its annotation as involved in defense response and oxidation reduction. Thus, computational biology methods allow elucidation of pleiotropy (one protein exerting multiple functions).
GST belongs to a family of enzymes comprising cytosolic, mitochondrial, and microsomal proteins [Citation103]. These enzymes catalyze the conjugation of reduced glutathione via a sulfhydryl group, on a wide variety of substrates, including peroxidised lipids [Citation104] and xenobiotics [Citation105]. GSTs may also bind toxins [Citation106] and function as transport proteins. Just as in the case of MPO, this group of enzymes has multiple properties, including anti-apoptosis and control of redox.
Heat shock protein (HSP)A1B (also known as HSP70) was differentially expressed in the AF of women with IAI. This observation is in keeping with our previous report [Citation107]. The precise role of HSP70 in AF is unknown. It is possible that HSP70 participates in stimulating prostaglandin (PG) production during PTL associated with IAI [Citation38,Citation108–117]. Indeed, HSP70 induces cyclooxygenase enzyme COX-2 protein expression and PGE2 production in human umbilical vein endothelial cells [Citation118]. Further studies are required to examine the role of HSP70 in COX-2 expression and PG production by gestational tissues.
The 14-3-3 proteins, first identified in 1967, are a family of acidic regulatory peptides found in plants and animals [Citation119]. These proteins are highly conserved and seven isoforms have been identified in mammals (β, γ, ε, σ, ζ, τ and η) [Citation120,Citation121]. 14-3-3 proteins can interact with many cellular proteins including transcription factors, enzymes, cytoskeletal proteins, signaling molecules, apoptosis factors, and tumor suppressors, as a result of their specific phospho-serine/phospho-threonine binding activity [Citation120,Citation121]. Because 14-3-3 interactions are primarily phosphorylation-dependent, the 14-3-3 proteins have been implicated as important components of crucial biological processes that are regulated by phosphorylation, including signal transduction, cell-cycle regulation metabolism control and apoptosis [Citation119,Citation122,Citation123]. This is the first report documenting an association between the up-regulation of members of the 14-3-3 protein families and PTL.
Host defense
This cluster (Table S4) includes several proteins known to be up-regulated in the AF of women delivering preterm with IAI, including neutrophil defensins [Citation77], lactoferrin [Citation76], FALL-39 antimicrobial protein [Citation18], and Calgranulin C [Citation13,Citation18]. Also included in this cluster are peptidoglycan recognition protein, bone-marrow proteoglycan, and two heat shock proteins (HSPB1 and HSPA1B). The role of antimicrobial peptides [Citation76,Citation77,Citation124–127] and pattern recognition receptors [Citation128–136] as well as heat shock proteins in host defense against IAI [Citation107], has been subject of previous communications by our group, and the interested reader is referred to those publications for details.
Proteins differentially regulated in patients with preterm labor leading to preterm delivery without intra-amniotic infection/inflammation
Of major importance is that we were able to identify and provide quantitative assessment of a cluster of proteins that are differentially regulated in women destined to deliver preterm in the absence of IAI. Such proteins are listed in . Notably, it includes SLPI, Leukocyte elastase inhibitor, a γ-chain of hemoglobin, and Resistin. The identification of Resistin as a potentially differentially regulated protein in this study has allowed us to conduct a targeted approach in which AF Resistin concentrations were measured (using ELISA) in the three clinical groups included in this study [Citation137]. That study found that AF Resistin concentration was higher in women with IAI, as well as in those with PTL without IAI leading to preterm delivery within 48 h and 7 days of amniocentesis, than in those who delivered at term [Citation137]. Therefore, the differential regulation of Resistin in AF found in this study has already been confirmed in an independent set of patients. We have previously reported the behavior of SLPI in PTL [Citation138] as well as hemoglobin [Citation139,Citation140]. Further studies are required to determine if the concentration of these proteins, alone or in combination, have prognostic value.
‘Systems biology’ analysis of preterm delivery in the presence of intra-amniotic infection/inflammation
Proteomic studies such as the present one offer a ‘wide-angle’ picture of protein expression within a system; in this case, AF in women presenting with PTL. Among women diagnosed with IAI who delivered preterm there is a large number of proteins that appear to be differentially regulated in comparison with patients without IAI, within a constellation of an even larger number of proteins that were sampled experimentally. The scope of such studies permits a statistical analysis to suggest the significance of biological processes that are important in the presence of IAI. We use the term ‘systems biology’ here as it relates to such a holistic as opposed to a reductionistic approach to understanding biological process underlying preterm delivery with IAI.
Strengths and weaknesses of this study
The major strength of this study is that it is the first ‘bottom-up’ proteomic approach used in the characterization of AF in the setting of PTL, and that differentially regulated proteins have been found in women who delivered preterm with and without IAI. Moreover, since some of the proteins found to be differentially regulated with this approach have been previously reported to participate in preterm parturition, this may be considered validation of our findings and approach. A potential weakness of ‘bottom-up’ proteomics is that because protein identification is predicated on the observations of tryptic peptides, the method may be unable to distinguish isoforms, fragments, or other post-translational modification from intact proteins which may be found in health or disease. Gravett et al. [Citation18] and our group [Citation45] have previously reported an example of such case when fragments of insulin-like growth factor binding protein (IGFBP)-1 which is proteolytically degraded in cases of infection, were found in AF [Citation141]. Such findings would be more difficult to detect with ‘bottom-up’ proteomics. Consequently, ‘bottom-up’ and ‘top-down’ proteomics are complementary methods that may be used in concert to discern the full complement of proteins differentially regulated in a biologic matrix.
Conclusions
This work provides evidence that a ‘bottom-up’ proteomics approach can be used to discern differentially regulated proteins in the AF of women with PTL. We have identified a large number of proteins which have been implicated in PTL through decades of hypothesis-driven research, and have also discovered proteins which thus far have not been implicated in preterm parturition. These observations confirm the power of the combined use of proteomics and computational biology as a discovery platform to gain insight into the complex biological processes of preterm parturition. The complexity of such processes has eluded definition using conventional reductionist, hypothesis-driven research. It is important to note that discovery-based approaches are fundamentally hypothesis-generating methodologies, and thus, provide new hypotheses which can be tested using conventional approaches.
Supplementary Material
Download PDF (18 KB)Supplementary Material
Download PDF (15.5 KB)Supplementary Material
Download PDF (18.5 KB)Supplementary Material
Download PDF (18.5 KB)Acknowledgement
This research was supported (in part) by the Perinatology Research Branch, Division of Intramural Research, Eunice Kennedy Shriver National Institute of Child Health and Human Development, NIH, DHHS.
References
- Evans GA. Designer science and the ‘omic’ revolution. Nat Biotechnol 2000;18:127.
- Gracey AY, Cossins AR. Application of microarray technology in environmental and comparative physiology. Annu Rev Physiol 2003;65:231–259.
- Mehta T, Tanik M, Allison DB. Towards sound epistemological foundations of statistical methods for high-dimensional biology. Nat Genet 2004;36:943–947.
- Scatena CD, Adler S. Characterization of a human-specific regulator of placental corticotropin-releasing hormone. Mol Endocrinol 1998;12:1228–1240.
- Tashima LS, Millar LK, Bryant-Greenwood GD. Genes upregulated in human fetal membranes by infection or labor. Obstet Gynecol 1999;94:441–449.
- Aguan K, Carvajal JA, Thompson LP, Weiner CP. Application of a functional genomics approach to identify differentially expressed genes in human myometrium during pregnancy and labour. Mol Hum Reprod 2000;6:1141–1145.
- Alizadeh AA, Eisen MB, Davis RE, Ma C, Lossos IS, Rosenwald A, Boldrick JC, Sabet H, Tran T, Yu X, et al Distinct types of diffuse large B-cell lymphoma identified by gene expression profiling. Nature 2000;403:503–511.
- Muhle RA, Pavlidis P, Grundy WN, Hirsch E. A high-throughput study of gene expression in preterm labor with a subtractive microarray approach. Am J Obstet Gynecol 2001;185:716–724.
- Chan EC, Fraser S, Yin S, Yeo G, Kwek K, Fairclough RJ, Smith R. Human myometrial genes are differentially expressed in labor: a suppression subtractive hybridization study. J Clin Endocrinol Metab 2002;87:2435–2441.
- Romero R, Kuivaniemi H, Tromp G, Olson J. The design, execution, and interpretation of genetic association studies to decipher complex diseases. Am J Obstet Gynecol 2002;187:1299–1312.
- Romero R, Kuivaniemi H, Tromp G. Functional genomics and proteomics in term and preterm parturition. J Clin Endocrinol Metab 2002;87:2431–2434.
- van de Vijver MJ, He YD, van't Veer LJ, Dai H, Hart AA, Voskuil DW, Schreiber GJ, Peterse JL, Roberts C, Marton MJ, et al A gene-expression signature as a predictor of survival in breast cancer. N Engl J Med 2002;347:1999–2009.
- Buhimschi I, Christner R, Buhimschi C, Chaiworapongsa T, Romero R. Proteomic analysis of preterm parturition: a novel method of identifying the patient at risk for preterm delivery. Am J Obstet Gynecol 2002;187:S55.
- Weston GC, Ponnampalam A, Vollenhoven BJ, Healy DL, Rogers PA. Genomics in obstetrics and gynaecology. Aust N Z J Obstet Gynaecol 2003;43:264–272.
- van `t Veer LJ, Dai H, van de Vijver MJ, He YD, Hart AA, Bernards R, Friend SH. Expression profiling predicts outcome in breast cancer. Breast Cancer Res 2003;5:57–58.
- Romero R, Chaiworapongsa T, Gomez R, Kim YM, Edwin S, Bujold E, Yoon BH. Proteomic profiling of premature labor: a method to identify clinical biomarkers and mechanisms of disease. Am J Obstet Gynecol 2003;189:S63.
- Vuadens F, Benay C, Crettaz D, Gallot D, Sapin V, Schneider P, Bienvenut WV, Lemery D, Quadroni M, Dastugue B, et al Identification of biologic markers of the premature rupture of fetal membranes: proteomic approach. Proteomics 2003;3:1521–1525.
- Gravett MG, Novy MJ, Rosenfeld RG, Reddy AP, Jacob T, Turner M, McCormack A, Lapidus JA, Hitti J, Eschenbach DA, et al Diagnosis of intra-amniotic infection by proteomic profiling and identification of novel biomarkers. JAMA 2004;292:462–469.
- Romero R, Gomez R, Nien JK, Yoon BH, Luo R, Beecher C, Mazor M. Metabolomics in premature labor: a novel approach to identify patients at risk for preterm delivery. Am J Obstet Gynecol 2004;191:S2.
- Tromp G, Kuivaniemi H, Romero R, Chaiworapongsa T, Kim YM, Kim MR, Maymon E, Edwin S. Genome-wide expression profiling of fetal membranes reveals a deficient expression of proteinase inhibitor 3 in premature rupture of membranes. Am J Obstet Gynecol 2004;191:1331–1338.
- Merrick BA, Bruno ME. Genomic and proteomic profiling for biomarkers and signature profiles of toxicity. Curr Opin Mol Ther 2004;6:600–607.
- Monteoliva L, Albar JP. Differential proteomics: an overview of gel and non-gel based approaches. Brief Funct Genomic Proteomic 2004;3:220–239.
- Mitra AP, Lin H, Cote RJ, Datar RH. Biomarker profiling for cancer diagnosis, prognosis and therapeutic management. Natl Med J India 2005;18:304–312.
- Watanabe A, Cornelison R, Hostetter G. Tissue microarrays: applications in genomic research. Expert Rev Mol Diagn 2005;5:171–181.
- Bisits AM, Smith R, Mesiano S, Yeo G, Kwek K, MacIntyre D, Chan EC. Inflammatory aetiology of human myometrial activation tested using directed graphs. PLoS Comput Biol 2005;1:132–136.
- Wilson RD. Genomics: new technology for obstetrics. J Obstet Gynaecol Can 2005;27:63–75.
- Havelock JC, Keller P, Muleba N, Mayhew BA, Casey BM, Rainey WE, Word RA. Human myometrial gene expression before and during parturition. Biol Reprod 2005;72:707–719.
- Huber A, Hudelist G, Czerwenka K, Husslein P, Kubista E, Singer CF. Gene expression profiling of cervical tissue during physiological cervical effacement. Obstet Gynecol 2005;105:91–98.
- Friel L, Kuivaniemi H, Gomez R, Goddard K, Nien JK, Tromp G, Lu Q, Xu Z, Behnke E, Solari M, et al Genetic predisposition for preterm PROM: results of a large candidate–gene association study of mothers and their offspring. Am J Obstet Gynecol 2005;193:S17.
- Word RA, Landrum CP, Timmons BC, Young SG, Mahendroo MS. Transgene insertion on mouse chromosome 6 impairs function of the uterine cervix and causes failure of parturition. Biol Reprod 2005;73:1046–1056.
- Ruetschi U, Rosen A, Karlsson G, Zetterberg H, Rymo L, Hagberg H, Jacobsson B. Proteomic analysis using protein chips to detect biomarkers in cervical and amniotic fluid in women with intra-amniotic inflammation. J Proteome Res 2005;4:2236–2242.
- Tarca AL, Romero R, Draghici S. Analysis of microarray experiments of gene expression profiling. Am J Obstet Gynecol 2006;195:373–388.
- Haddad R, Tromp G, Kuivaniemi H, Chaiworapongsa T, Kim YM, Mazor M, Romero R. Human spontaneous labor without histologic chorioamnionitis is characterized by an acute inflammation gene expression signature. Am J Obstet Gynecol 2006;195:394–424.
- Portilla D, Li S, Nagothu KK, Megyesi J, Kaissling B, Schnackenberg L, Safirstein RL, Beger RD. Metabolomic study of cisplatin-induced nephrotoxicity. Kidney Int 2006;69:2194–2204.
- Hassan SS, Romero R, Haddad R, Hendler I, Khalek N, Tromp G, Diamond MP, Sorokin Y, Malone J Jr. The transcriptome of the uterine cervix before and after spontaneous term parturition. Am J Obstet Gynecol 2006;195:778–786.
- Bukowski R, Hankins GD, Saade GR, Anderson GD, Thornton S. Labor-associated gene expression in the human uterine fundus, lower segment, and cervix. PLoS Med 2006;3:e169.
- Romero R, Tarca AL, Tromp G. Insights into the physiology of childbirth using transcriptomics. PLoS Med 2006;3:e276.
- Haddad R, Gould BR, Romero R, Tromp G, Farookhi R, Edwin SS, Kim MR, Zingg HH. Uterine transcriptomes of bacteria-induced and ovariectomy-induced preterm labor in mice are characterized by differential expression of arachidonate metabolism genes. Am J Obstet Gynecol 2006;195:822–828.
- Pennell CE, Oldenhof AD, Perkins JE, Dunk CE, Keunen J, Tan P, Bocking AD, Lye SJ. Identification of a gene expression signature in leukocytes that predicts preterm delivery in women with threatened preterm labour. J Soc Gynecol Investig 2006;13:175A.
- Romero R, Tromp G. High-dimensional biology in obstetrics and gynecology: functional genomics in microarray studies. Am J Obstet Gynecol 2006;195:360–363.
- Ward K. Microarray technology in obstetrics and gynecology: a guide for clinicians. Am J Obstet Gynecol 2006;195:364–372.
- Mason CW, Swaan PW, Weiner CP. Identification of interactive gene networks: a novel approach in gene array profiling of myometrial events during guinea pig pregnancy. Am J Obstet Gynecol 2006;194:1513–1523.
- Klein LL, Reisdorph N, Jonscher KR, Kushner EJ, Gibbs RS, McManaman JL. A novel method for vaginal proteomics in preterm labor. J Soc Gynecol Investig 2006;13:144A.
- Romero R, Espinoza J, Rogers WT, Moser A, Nien JK, Kusanovic JP, Gotsch F, Erez O, Gomez R, Edwin S, et al Proteomic analysis of amniotic fluid to identify women with preterm labor and intra-amniotic inflammation/infection: the use of a novel computational method to analyze mass spectrometric profiling. J Matern Fetal Neonatal Med 2008;21:367–388.
- Bujold E, Romero R, Kusanovic JP, Erez O, Gotsch F, Chaiworapongsa T, Gomez R, Espinoza J, Vaisbuch E, Mee KY, et al Proteomic profiling of amniotic fluid in preterm labor using two-dimensional liquid separation and mass spectrometry. J Matern Fetal Neonatal Med 2008;21:697–713.
- Han X, Aslanian A, Yates JR III. Mass spectrometry for proteomics. Curr Opin Chem Biol 2008;12:483–490.
- Michaels JE, Dasari S, Pereira L, Reddy AP, Lapidus JA, Lu X, Jacob T, Thomas A, Rodland M, Roberts CT Jr, et al Comprehensive proteomic analysis of the human amniotic fluid proteome: gestational age-dependent changes. J Proteome Res 2007;6:1277–1285.
- Aggarwal K, Choe LH, Lee KH. Shotgun proteomics using the iTRAQ isobaric tags. Brief Funct Genomic Proteomic 2006;5:112–120.
- Chong PK, Gan CS, Pham TK, Wright PC. Isobaric tags for relative and absolute quantitation (iTRAQ) reproducibility: implication of multiple injections. J Proteome Res 2006;5:1232–1240.
- Zieske LR. A perspective on the use of iTRAQ reagent technology for protein complex and profiling studies. J Exp Bot 2006;57:1501–1508.
- Yoon BH, Romero R, Moon JB, Shim SS, Kim M, Kim G, Jun JK. Clinical significance of intra-amniotic inflammation in patients with preterm labor and intact membranes. Am J Obstet Gynecol 2001;185:1130–1136.
- Romero R, Emamian M, Quintero R, Wan M, Hobbins JC, Mazor M, Edberg S. The value and limitations of the Gram stain examination in the diagnosis of intraamniotic infection. Am J Obstet Gynecol 1988;159:114–119.
- Romero R, Jimenez C, Lohda AK, Nores J, Hanaoka S, Avila C, Callahan R, Mazor M, Hobbins JC, Diamond MP. Amniotic fluid glucose concentration: a rapid and simple method for the detection of intraamniotic infection in preterm labor. Am J Obstet Gynecol 1990;163:968–974.
- Romero R, Quintero R, Nores J, Avila C, Mazor M, Hanaoka S, Hagay Z, Merchant L, Hobbins JC. Amniotic fluid white blood cell count: a rapid and simple test to diagnose microbial invasion of the amniotic cavity and predict preterm delivery. Am J Obstet Gynecol 1991;165:821–830.
- Tam SW, Pirro J, Hinerfeld D. Depletion and fractionation technologies in plasma proteomic analysis. Expert Rev Proteomics 2004;1:411–420.
- Hsieh HC, Chen YT, Li JM, Chou TY, Chang MF, Huang SC, Tseng TL, Liu CC, Chen SF. Protein profilings in mouse liver regeneration after partial hepatectomy using iTRAQ technology. J Proteome Res 2009;8:1004–1013.
- Boersema PJ, Raijmakers R, Lemeer S, Mohammed S, Heck AJ. Multiplex peptide stable isotope dimethyl labeling for quantitative proteomics. Nat Protoc 2009;4:484–494.
- Keller A, Nesvizhskii AI, Kolker E, Aebersold R. Empirical statistical model to estimate the accuracy of peptide identifications made by MS/MS and database search. Anal Chem 2002;74:5383–5392.
- Nesvizhskii AI, Keller A, Kolker E, Aebersold R. A statistical model for identifying proteins by tandem mass spectrometry. Anal Chem 2003;75:4646–4658.
- Ma B, Zhang K, Hendrie C, Liang C, Li M, Doherty-Kirby A, Lajoie G. PEAKS: powerful software for peptide de novo sequencing by tandem mass spectrometry. Rapid Commun Mass Spectrom 2003;17:2337–2342.
- Lin WT, Hung WN, Yian YH, Wu KP, Han CL, Chen YR, Chen YJ, Sung TY, Hsu WL. Multi-Q: a fully automated tool for multiplexed protein quantitation. J Proteome Res 2006;5:2328–2338.
- Armenta JM, Hoeschele I, Lazar IM. Differential protein expression analysis using stable isotope labeling and PQD linear ion trap MS technology. J Am Soc Mass Spectrom 2009. March 4 [Epub ahead of print].
- Maere S, Heymans K, Kuiper M. BiNGO: a Cytoscape plugin to assess overrepresentation of gene ontology categories in biological networks. Bioinformatics 2005;21:3448–3449.
- Shannon P, Markiel A, Ozier O, Baliga NS, Wang JT, Ramage D, Amin N, Schwikowski B, Ideker T. Cytoscape: a software environment for integrated models of biomolecular interaction networks. Genome Res 2003;13:2498–2504.
- Weiling F. What about R.A. Fisher's statement of the ‘too good’ data of J.G. Mendel's Pisum paper? J Hered 1986;77:281–283.
- Benjamini Y, Hochberg Y. Controlling the false discovery rate – A practical and powerful approach to multiple testing. J R Stat Soc Series B Stat Methodol 1995;57:289–300.
- McDonald WH, Yates JR III. Shotgun proteomics and biomarker discovery. Dis Markers 2002;18:99–105.
- DeSouza LV, Grigull J, Ghanny S, Dube V, Romaschin AD, Colgan TJ, Siu KW. Endometrial carcinoma biomarker discovery and verification using differentially tagged clinical samples with multidimensional liquid chromatography and tandem mass spectrometry. Mol Cell Proteomics 2007;6:1170–1182.
- Ogata Y, Charlesworth MC, Higgins L, Keegan BM, Vernino S, Muddiman DC. Differential protein expression in male and female human lumbar cerebrospinal fluid using iTRAQ reagents after abundant protein depletion. Proteomics 2007;7:3726–3734.
- Song X, Bandow J, Sherman J, Baker JD, Brown PW, McDowell MT, Molloy MP. iTRAQ experimental design for plasma biomarker discovery. J Proteome Res 2008;7:2952–2958.
- Latterich M, Abramovitz M, Leyland-Jones B. Proteomics: new technologies and clinical applications. Eur J Cancer 2008;44:2737–2741.
- Bouchal P, Roumeliotis T, Hrstka R, Nenutil R, Vojtesek B, Garbis SD. Biomarker discovery in low-grade breast cancer using isobaric stable isotope tags and two-dimensional liquid chromatography-tandem mass spectrometry (iTRAQ-2DLC-MS/MS) based quantitative proteomic analysis. J Proteome Res 2009;8:362–373.
- Ralhan R, DeSouza LV, Matta A, Chandra TS, Ghanny S, Dattagupta S, Thakar A, Chauhan SS, Siu KW. iTRAQ-multidimensional liquid chromatography and tandem mass spectrometry-based identification of potential biomarkers of oral epithelial dysplasia and novel networks between inflammation and premalignancy. J Proteome Res 2009;8:300–309.
- Yoon BH, Jun JK, Park KH, Syn HC, Gomez R, Romero R. Serum C-reactive protein, white blood cell count, and amniotic fluid white blood cell count in women with preterm premature rupture of membranes. Obstet Gynecol 1996;88:1034–1040.
- Athayde N, Romero R, Gomez R, Maymon E, Pacora P, Mazor M, Yoon BH, Fortunato S, Menon R, Ghezzi F, et al Matrix metalloproteinases-9 in preterm and term human parturition. J Matern Fetal Med 1999;8:213–219.
- Pacora P, Maymon E, Gervasi MT, Gomez R, Edwin SS, Yoon BH, Romero R. Lactoferrin in intrauterine infection, human parturition, and rupture of fetal membranes. Am J Obstet Gynecol 2000;183:904–910.
- Espinoza J, Chaiworapongsa T, Romero R, Edwin S, Rathnasabapathy C, Gomez R, Bujold E, Camacho N, Kim YM, Hassan S, et al Antimicrobial peptides in amniotic fluid: defensins, calprotectin and bacterial/permeability-increasing protein in patients with microbial invasion of the amniotic cavity, intra-amniotic inflammation, preterm labor and premature rupture of membranes. J Matern Fetal Neonatal Med 2003;13:2–21.
- Chaiworapongsa T, Romero R, Espinoza J, Kim YM, Edwin S, Bujold E, Gomez R, Kuivaniemi H. Macrophage migration inhibitory factor in patients with preterm parturition and microbial invasion of the amniotic cavity. J Matern Fetal Neonatal Med 2005;18:405–416.
- Shim SS, Romero R, Jun JK, Moon KC, Kim G, Yoon BH. C-reactive protein concentration in vaginal fluid as a marker for intra-amniotic inflammation/infection in preterm premature rupture of membranes. J Matern Fetal Neonatal Med 2005;18:417–422.
- Park SJ, Yoon WG, Song JS, Jung HS, Kim CJ, Oh SY, Yoon BH, Jung G, Kim HJ, Nirasawa T. Proteome analysis of human amnion and amniotic fluid by two-dimensional electrophoresis and matrix-assisted laser desorption/ionization time-of-flight mass spectrometry. Proteomics 2006;6:349–363.
- Nien JK, Yoon BH, Espinoza J, Kusanovic JP, Erez O, Soto E, Richani K, Gomez R, Hassan S, Mazor M, et al A rapid MMP-8 bedside test for the detection of intra-amniotic inflammation identifies patients at risk for imminent preterm delivery. Am J Obstet Gynecol 2006;195:1025–1030.
- Peltier MR, Barney BM, Brown MB. Effect of experimental genital mycoplasmosis on production of matrix metalloproteinases in membranes and amniotic fluid of Sprague-Dawley rats. Am J Reprod Immunol 2007;57:116–121.
- Bhatt K, Feng L, Pabla N, Liu K, Smith S, Dong Z. Effects of targeted Bcl-2 expression in mitochondria or endoplasmic reticulum on renal tubular cell apoptosis. Am J Physiol Renal Physiol 2008;294:F499–F507.
- Zhang K, Kaufman RJ. From endoplasmic-reticulum stress to the inflammatory response. Nature 2008;454:455–462.
- Veijola J, Pettersson RF. Transient association of calnexin and calreticulin with newly synthesized G1 and G2 glycoproteins of uukuniemi virus (family Bunyaviridae). J Virol 1999;73:6123–6127.
- Kim JR, Kwon KS, Yoon HW, Lee SR, Rhee SG. Oxidation of proteinaceous cysteine residues by dopamine-derived H2O2 in PC12 cells. Arch Biochem Biophys 2002;397:414–423.
- Romero R, Ceska M, Avila C, Mazor M, Behnke E, Lindley I. Neutrophil attractant/activating peptide-1/interleukin-8 in term and preterm parturition. Am J Obstet Gynecol 1991;165:813–820.
- Romero R, Yoon BH, Mazor M, Gomez R, Diamond MP, Kenney JS, Ramirez M, Fidel PL, Sorokin Y, Cotton D, et al The diagnostic and prognostic value of amniotic fluid white blood cell count, glucose, interleukin-6, and gram stain in patients with preterm labor and intact membranes. Am J Obstet Gynecol 1993;169:805–816.
- Romero R, Yoon BH, Mazor M, Gomez R, Gonzalez R, Diamond MP, Baumann P, Araneda H, Kenney JS, Cotton DB, et al A comparative study of the diagnostic performance of amniotic fluid glucose, white blood cell count, interleukin-6, and gram stain in the detection of microbial invasion in patients with preterm premature rupture of membranes. Am J Obstet Gynecol 1993;169:839–851.
- Cherouny PH, Pankuch GA, Romero R, Botti JJ, Kuhn DC, Demers LM, Appelbaum PC. Neutrophil attractant/activating peptide-1/interleukin-8: association with histologic chorioamnionitis, preterm delivery, and bioactive amniotic fluid leukoattractants. Am J Obstet Gynecol 1993;169:1299–1303.
- Gomez R, Romero R, Galasso M, Behnke E, Insunza A, Cotton DB. The value of amniotic fluid interleukin-6, white blood cell count, and gram stain in the diagnosis of microbial invasion of the amniotic cavity in patients at term. Am J Reprod Immunol 1994;32:200–210.
- Yoon BH, Yang SH, Jun JK, Park KH, Kim CJ, Romero R. Maternal blood C-reactive protein, white blood cell count, and temperature in preterm labor: a comparison with amniotic fluid white blood cell count. Obstet Gynecol 1996;87:231–237.
- Yoon BH, Romero R, Park JS, Chang JW, Kim YA, Kim JC, Kim KS. Microbial invasion of the amniotic cavity with Ureaplasma urealyticum is associated with a robust host response in fetal, amniotic, and maternal compartments. Am J Obstet Gynecol 1998;179:1254–1260.
- Lee SE, Romero R, Kim CJ, Shim SS, Yoon BH. Funisitis in term pregnancy is associated with microbial invasion of the amniotic cavity and intra-amniotic inflammation. J Matern Fetal Neonatal Med 2006;19:693–697.
- Lee SE, Romero R, Jung H, Park CW, Park JS, Yoon BH. The intensity of the fetal inflammatory response in intraamniotic inflammation with and without microbial invasion of the amniotic cavity. Am J Obstet Gynecol 2007;197:294–296.
- Seong HS, Lee SE, Kang JH, Romero R, Yoon BH. The frequency of microbial invasion of the amniotic cavity and histologic chorioamnionitis in women at term with intact membranes in the presence or absence of labor. Am J Obstet Gynecol 2008;199:375.e1–375.e5.
- Bouillet P, Metcalf D, Huang DC, Tarlinton DM, Kay TW, Kontgen F, Adams JM, Strasser A. Proapoptotic Bcl-2 relative Bim required for certain apoptotic responses, leukocyte homeostasis, and to preclude autoimmunity. Science 1999;286:1735–1738.
- Lagasse E, Weissman IL. bcl-2 inhibits apoptosis of neutrophils but not their engulfment by macrophages. J Exp Med 1994;179:1047–1052.
- Rowe SJ, Allen L, Ridger VC, Hellewell PG, Whyte MK. Caspase-1-deficient mice have delayed neutrophil apoptosis and a prolonged inflammatory response to lipopolysaccharide-induced acute lung injury. J Immunol 2002;169:6401–6407.
- Simon HU. Neutrophil apoptosis pathways and their modifications in inflammation. Immunol Rev 2003;193: 101–110.
- Song R, Kubo M, Morse D, Zhou Z, Zhang X, Dauber JH, Fabisiak J, Alber SM, Watkins SC, Zuckerbraun BS, et al Carbon monoxide induces cytoprotection in rat orthotopic lung transplantation via anti-inflammatory and anti-apoptotic effects. Am J Pathol 2003;163:231–242.
- Hampton MB, Kettle AJ, Winterbourn CC. Inside the neutrophil phagosome: oxidants, myeloperoxidase, and bacterial killing. Blood 1998;92:3007–3017.
- Hayes JD, Pulford DJ. The glutathione S-transferase supergene family: regulation of GST and the contribution of the isoenzymes to cancer chemoprotection and drug resistance. Crit Rev Biochem Mol Biol 1995;30:445–600.
- Gibson DD, Hornbrook KR, McCay PB. Glutathione-dependent inhibition of lipid peroxidation by a soluble, heat-labile factor in animal tissues. Biochim Biophys Acta 1980;620:572–582.
- Baars AJ, Breimer DD. The glutathione S-transferases: their role in detoxification and toxification of xenobiotics. Ann Biol Clin (Paris) 1980;38:49–56.
- Slone DH, Gallagher EP, Ramsdell HS, Rettie AE, Stapleton PL, Berlad LG, Eaton DL. Human variability in hepatic glutathione S-transferase-mediated conjugation of aflatoxin B1-epoxide and other substrates. Pharmacogenetics 1995;5:224–233.
- Chaiworapongsa T, Erez O, Kusanovic JP, Vaisbuch E, Mazaki-Tovi S, Gotsch F, Than NG, Mittal P, Kim YM, Camacho N, et al Amniotic fluid heat shock protein 70 concentration in histologic chorioamnionitis, term and preterm parturition. J Matern Fetal Neonatal Med 2008;21:449–461.
- Romero R, Emamian M, Wan M, Quintero R, Hobbins JC, Mitchell MD. Prostaglandin concentrations in amniotic fluid of women with intra-amniotic infection and preterm labor. Am J Obstet Gynecol 1987;157:1461–1467.
- Romero R, Wu YK, Mazor M, Hobbins JC, Mitchell MD. Amniotic fluid prostaglandin E2 in preterm labor. Prostaglandins Leukot Essent Fatty Acids 1988;34:141–145.
- Romero R, Wu YK, Sirtori M, Oyarzun E, Mazor M, Hobbins JC, Mitchell MD. Amniotic fluid concentrations of prostaglandin F2 alpha, 13,14-dihydro-15-keto-prostaglandin F2 alpha (PGFM) and 11-deoxy-13,14-dihydro-15-keto-11, 16-cyclo-prostaglandin E2 (PGEM-LL) in preterm labor. Prostaglandins 1989;37:149–161.
- Mitchell MD, Edwin S, Romero RJ. Prostaglandin biosynthesis by human decidual cells: effects of inflammatory mediators. Prostaglandins Leukot Essent Fatty Acids 1990;41:35–38.
- Mitchell MD, Romero RJ, Avila C, Foster JT, Edwin SS. Prostaglandin production by amnion and decidual cells in response to bacterial products. Prostaglandins Leukot Essent Fatty Acids 1991;42:167–169.
- Romero R, Baumann P, Gomez R, Salafia C, Rittenhouse L, Barberio D, Behnke E, Cotton DB, Mitchell MD. The relationship between spontaneous rupture of membranes, labor, and microbial invasion of the amniotic cavity and amniotic fluid concentrations of prostaglandins and thromboxane B2 in term pregnancy. Am J Obstet Gynecol 1993;168:1654–1664.
- Mitchell MD, Romero RJ, Edwin SS, Trautman MS. Prostaglandins and parturition. Reprod Fertil Dev 1995;7: 623–632.
- Helliwell RJ, Keelan JA, Marvin KW, Adams L, Chang MC, Anand A, Sato TA, O'Carroll S, Chaiworapongsa T, Romero RJ, et al Gestational age-dependent up-regulation of prostaglandin D synthase (PGDS) and production of PGDS-derived antiinflammatory prostaglandins in human placenta. J Clin Endocrinol Metab 2006;91:597–606.
- Romero R, Gotsch F, Pineles B, Kusanovic JP. Inflammation in pregnancy: its roles in reproductive physiology, obstetrical complications, and fetal injury. Nutr Rev 2007;65:S194–S202.
- Lee SE, Romero R, Park IS, Seong HS, Park CW, Yoon BH. Amniotic fluid prostaglandin concentrations increase before the onset of spontaneous labor at term. J Matern Fetal Neonatal Med 2008;21:89–94.
- Zhang F, Hackett NR, Lam G, Cheng J, Pergolizzi R, Luo L, Shmelkov SV, Edelberg J, Crystal RG, Rafii S. Green fluorescent protein selectively induces HSP70-mediated up-regulation of COX-2 expression in endothelial cells. Blood 2003;102:2115–2121.
- Fu H, Subramanian RR, Masters SC. 14-3-3 proteins: structure, function, and regulation. Annu Rev Pharmacol Toxicol 2000;40:617–647.
- Morrison DK. The 14-3-3 proteins: integrators of diverse signaling cues that impact cell fate and cancer development. Trends Cell Biol 2009;19:16–23.
- Obsilova V, Silhan J, Boura E, Teisinger J, Obsil T. 14-3-3 proteins: a family of versatile molecular regulators. Physiol Res 2008;57:S11–S21.
- Aitken A. 14-3-3 proteins: a historic overview. Semin Cancer Biol 2006;16:162–172.
- Hermeking H, Benzinger A. 14-3-3 proteins in cell cycle regulation. Semin Cancer Biol 2006;16:183–192.
- Nhan-Chang CL, Romero R, Kusanovic JP, Gotsch F, Edwin SS, Erez O, Mittal P, Kim CJ, Kim MJ, Espinoza J, et al A role for CXCL13 (BCA-1) in pregnancy and intra-amniotic infection/inflammation. J Matern Fetal Neonatal Med 2008;21:763–775.
- Soto E, Espinoza J, Nien JK, Kusanovic JP, Erez O, Richani K, Santolaya-Forgas J, Romero, R. Human beta-defensin-2: a natural antimicrobial peptide present in amniotic fluid participates in the host response to microbial invasion of the amniotic cavity. J Matern Fetal Neonatal Med 2007;20:15–22.
- Svinarich DM, Wolf NA, Gomez R, Gonik B, Romero R. Detection of human defensin 5 in reproductive tissues. Am J Obstet Gynecol 1997;176:470–475.
- Yevtushenko DP, Romero R, Forward BS, Hancock RE, Kay WW, Misra S. Pathogen-induced expression of a cecropin A-melittin antimicrobial peptide gene confers antifungal resistance in transgenic tobacco. J Exp Bot 2005;56:1685–1695.
- Abrahams VM, Aldo PB, Murphy SP, Visintin I, Koga K, Wilson G, Romero R, Sharma S, Mor G. TLR6 modulates first trimester trophoblast responses to peptidoglycan. J Immunol 2008;180:6035–6043.
- Ferrand PE, Fujimoto T, Chennathukuzhi V, Parry S, Macones GA, Sammel M, Kuivaniemi H, Romero R, Strauss JF III. The CARD15 2936insC mutation and TLR4 896 A > G polymorphism in African Americans and risk of preterm premature rupture of membranes (PPROM). Mol Hum Reprod 2002;8:1031–1034.
- Kim YM, Romero R, Chaiworapongsa T, Kim GJ, Kim MR, Kuivaniemi H, Tromp G, Espinoza J, Bujold E, Abrahams VM, et al Toll-like receptor-2 and -4 in the chorioamniotic membranes in spontaneous labor at term and in preterm parturition that are associated with chorioamnionitis. Am J Obstet Gynecol 2004;191:1346–1355.
- Kim YM, Romero R, Oh SY, Kim CJ, Kilburn BA, Armant DR, Nien JK, Gomez R, Mazor M, Saito S, et al Toll-like receptor 4: a potential link between ‘danger signals’, the innate immune system, and preeclampsia? Am J Obstet Gynecol 2005;193:921–927.
- Kim YM, Romero R, Chaiworapongsa T, Espinoza J, Mor G, Kim CJ. Dermatitis as a component of the fetal inflammatory response syndrome is associated with activation of toll-like receptors in epidermal keratinocytes. Histopathology 2006;49:506–514.
- Koga K, Cardenas I, Aldo P, Abrahams VM, Peng B, Fill S, Romero R, Mor G. Activation of TLR3 in the trophoblast is associated with preterm delivery. Am J Reprod Immunol 2009;61:196–212.
- Mor G, Romero R, Aldo PB, Abrahams VM. Is the trophoblast an immune regulator? The role of Toll-like receptors during pregnancy. Crit Rev Immunol 2005;25:375–388.
- Porras A, Kozar S, Russanova V, Salpea P, Hirai T, Sammons N, Mittal P, Kim JY, Ozato K, Romero R, et al Developmental and epigenetic regulation of the human TLR3 gene. Mol Immunol 2008;46:27–36.
- Romero R, Espinoza J, Kusanovic JP, Gotsch F, Hassan S, Erez O, Chaiworapongsa T, Mazor M. The preterm parturition syndrome. BJOG 2006;113:17–42.
- Kusanovic JP, Romero R, Mazaki-Tovi S, Chaiworapongsa T, Mittal P, Gotsch F, Erez O, Vaisbuch E, Edwin SS, Than NG, et al Resistin in amniotic fluid and its association with intra-amniotic infection and inflammation. J Matern Fetal Neonatal Med 2008;21:902–916.
- Helmig BR, Romero R, Espinoza J, Chaiworapongsa T, Bujold E, Gomez R, Ohlsson K, Uldbjerg N. Neutrophil elastase and secretory leukocyte protease inhibitor in prelabor rupture of membranes, parturition and intra-amniotic infection. J Matern Fetal Neonatal Med 2002;12:237–246.
- Vaisbuch E, Romero R, Erez O, Kusanovic JP, Gotsch F, Than NG, Mazaki-Tovi S, Mittal P, Edwin S, Hassan SS. Total hemoglobin concentration in amniotic fluid is increased in intraamniotic infection/inflammation. Am J Obstet Gynecol 2008;199:426–427.
- Vaisbuch E, Kusanovic JP, Erez O, Mazaki-Tovi S, Gotsch F, Kim CJ, Kim JS, Chaiworapongsa T, Edwin SS, Than NG, et al Amniotic fluid fetal hemoglobin in normal pregnancies and pregnancies complicated with preterm labor or prelabor rupture of membranes. J Matern Fetal Neonatal Med 2009;22:388–397.
- Lee SE, Han BD, Park IS, Romero R, Yoon BH. Evidence supporting proteolytic cleavage of insulin-like growth factor binding protein-1 (IGFBP-1) protein in amniotic fluid. J Perinat Med 2008;36:316–323.