Abstract
Objective: Pregnancy is characterized by activation of the innate immune response demonstrated by phenotypic and metabolic changes in granulocytes and monocytes. This state of activation has been implicated in the pathophysiology of multiorgan dysfunction of pregnant women with acute viral or bacterial infection. Tumor necrosis factor-related apoptosis-inducing ligand (TRAIL) is one of the mediators responsible for neutrophil apoptosis. Gene deletion of TRAIL results in delayed neutrophil apoptosis and resolution of inflammation after the administration of bacterial endotoxin. The aim of this study was to determine whether maternal plasma concentrations of the soluble form of TRAIL (sTRAIL) differ in women with uncomplicated pregnancy and those with acute pyelonephritis.
Method: A cross-sectional study was conducted to include women in the following groups: (1) non-pregnant (n = 23); (2) uncomplicated pregnancies (n = 93) and (3) pregnancies with acute pyelonephritis (n = 23). Plasma concentrations of sTRAIL were determined by enzyme-linked immunoassay.
Results: (1) Women with uncomplicated pregnancies had a lower mean plasma sTRAIL concentration (pg/mL) than non-pregnant women (31.5 ± 10.1 versus 53.3 ± 12.5; p < 0.001); (2) plasma sTRAIL concentrations did not change as a function of gestational age (Pearson correlation = −0.1; p = 0.4); (3) the mean plasma sTRAIL concentration (pg/mL) was significantly lower in pregnant women with acute pyelonephritis than in those with uncomplicated pregnancies (20.5 ± 6.6 versus 31.5 ± 10.1; p < 0.001) and (4) among patients with acute pyelonephritis, patients with bacteremia had a significantly lower mean plasma concentration of sTRAIL (pg/mL) than those without bacteremia (15.1 ± 4.8 versus 24.7 ± 4.6; p < 0.001).
Conclusion: Women with uncomplicated pregnancies are associated with a significantly lower mean maternal plasma concentration of sTRAIL than that observed in non-pregnant women. Moreover, a further decrease in plasma sTRAIL concentration was observed in pregnant women with acute pyelonephritis, and this could account, at least in part, for the exaggerated intravascular inflammatory response previously reported in pyelonephritis during pregnancy.
Introduction
Human pregnancy presents a unique immunological challenge [Citation1–3]. Upregulation of the innate immune response and downregulation of the adaptive immune response serves to balance the risk of maternal infection and tolerance of the fetal semi-allograft [Citation1,Citation2]. Despite activation of the innate immune system, pregnant women are more susceptible to infection-related complications [Citation1,Citation4–7]. The Shwartzman reaction requires two separate exposures to endotoxin in non-pregnant animals [Citation4]. In contrast, pregnant animals develop the Shwartzman reaction after a single injection of endotoxin [Citation4]. This has been interpreted as evidence that normal pregnancy is characterized by a state of physiologic intravascular inflammation. Therefore, pregnancy can be considered a Shwartzman “preparative” condition [Citation4,Citation8]. Evidence suggests that leukocyte plays an important role and its number and activation state correlate with the severity of the generalized Shwartzman reaction [Citation4,Citation9].
During pregnancy, neutrophils undergo phenotypic and metabolic changes in response to infection and acute inflammation [Citation2,Citation3,Citation10–16]. The number of circulating neutrophils increases with advancing gestational age in normal pregnancy [Citation17,Citation18]. Impaired neutrophil apoptosis is associated with a systemic inflammatory response syndrome (SIRS) [Citation19]. Jimenez et al. [Citation19] proposed that while prolonged neutrophil survival may constitute an appropriate adaptive response, impairment of this process may contribute to systemic inflammatory injury and possibly a multiple organ dysfunction. Tumor necrosis factor-related apoptosis-inducing ligand (TRAIL), a type II membrane-bound tumor necrosis factor (TNF) family ligand [Citation20,Citation21], has been implicated in the pathogenesis of multiorgan failure during the course of infection and may govern the resolution of infection-induced inflammation through its actions in delaying neutrophil apoptosis [Citation22–24]. While TRAIL and its receptors are found in the placenta and fetal membranes throughout gestation [Citation25,Citation26], few investigators have examined its relevance in normal and complicated pregnancy in the third trimester [Citation25–28].
Proposed roles of TRAIL in pregnancy include that it establishes immune tolerance at the feto-maternal interface [Citation25,Citation26] and induces smooth muscle cell apoptosis in the spiral arteries during implantation [Citation28,Citation29]. Recently, was observed a higher serum concentration of sTRAIL in patients with recurrent miscarriage than in those with uncomplicated pregnancy at 12 weeks of gestation, supporting that sTRAIL may play a role in the pathogenesis of early pregnancy complications [Citation30]. Yet, TRAIL has not been examined in the context of complications of pregnancy and in particular, in the presence of systemic inflammation which occur in the late second or third trimester, for instance acute pyelonephritis [Citation31–35] (a common reason for hospitalization during pregnancy) [Citation36]. Moreover, pregnant women with acute pyelonephritis are more susceptible to infection-related complications, such as acute respiratory distress syndrome (ARDS) [Citation36–46], sepsis [Citation36,Citation47], septic shock [Citation36,Citation48,Citation49], anemia [Citation36] and transient renal dysfunction [Citation50] than in non-pregnant women affected by this condition.
This study aims to determine whether maternal plasma sTRAIL concentrations during pregnancy differ from those in non-pregnant women and those of pregnant woman with acute pyelonephritis.
Materials and methods
Study design
A cross-sectional study was conducted by searching our clinical database and bank of biologic samples to include women in the following groups: (1) non-pregnant women; (2) uncomplicated pregnancies and (3) pregnancies with acute pyelonephritis. The non-pregnant group consisted of women who had no history of acute or chronic inflammatory conditions. The uncomplicated pregnancy group included pregnant women between 20 and 42 weeks of gestation who: (1) had no medical, obstetrical or surgical complications; (2) were not in labor and (3) delivered a normal term (≥37 weeks) infant whose birth weight was between the 10th and 90th percentile for gestational age. These women were enrolled from either Labor and Delivery unit (in cases of scheduled cesarean section) or the antenatal clinic, and followed until delivery. Acute pyelonephritis (n = 23) during pregnancy was diagnosed in the presence of fever (temperature ≥ 38 °C), clinical signs (e.g. back pain), pyuria and a positive urine culture for microorganisms. Women were grouped as follows: (1) acute pyelonephritis with positive urine culture only (without bacteremia) and (2) acute pyelonephritis with positive blood and urine cultures (with bacteremia).
All women provided written informed consent prior to the collection of plasma samples. The collection and utilization of samples for research purposes was approved by the Human Investigation Committee of Wayne State University and the IRB of Eunice Kennedy Shriver National Institute of Child Health and Human Development, NIH, DHHS. Many samples were previously studied in the investigation of intravascular inflammation, angiogenesis, and cytokine biology in normal and complicated pregnancies.
Sample collection and human sTRAIL
Venipuncture was performed in non-pregnant women, upon diagnosis of acute pyelonephritis and at 20–42 weeks of gestation upon enrollment for women with uncomplicated pregnancies. The blood was collected in EDTA tubes, centrifuged at 1300 g then stored at −70 °C. The concentrations of sTRAIL were measured using enzyme-linked immunoassay (ELISA) (R&D Systems, Minneapolis, MN). This assay employs the quantitative sandwich immunoassay technique. Briefly, recombinant human TRAIL standards and maternal plasma specimens were incubated in duplicate wells of the microtiter plates pre-coated with monoclonal antibodies specific for TRAIL. During this incubation, the immobilized antibodies in the microtiter plate bound TRAIL were present in both the standards and samples. After washing unbound substances, polyclonal antibodies to human TRAIL conjugated to an enzyme (horseradish peroxidase) were added to the assay wells. Once the incubation period was over, the assay plates were washed to remove unbound antibody-enzyme reagents. Upon addition of a substrate solution (tetramethylbenzidine), color developed in the assay plates proportionally to the amount of TRAIL bound in the initial step. Microtiter plates were read using a programmable spectrophotometer (SpectraMax M5 Multi-Mode Microplate Reader, Molecular Devices, Sunnyvale, CA). The inter- and intra-assay coefficients of variation were 6.52% and 3.66%, respectively. The sensitivity of sTRAIL was 8.22 pg/mL; patients having concentrations below this threshold were considered to have had a value of 8.1 pg/mL (99% of the limit of detection).
Statistical analysis
Normalilty of arithmetic data was assessed using the Kolmogorov-Smirnov test and visual plot inspection. Differences in frequency distributions of categorical variables were tested using the Chi-square or Fisher’s exact test, where appropriate. Bivariate comparisons of arithmetic data were examined using an unpaired Student’s t-test or Mann–Whitney U test, where appropriate. One-way analysis of variance with post-hoc Dunnett T3 tests were utilized to examine the differences in mean sTRAIL concentrations across groups. Multivariable general linear models were constructed to examine the effect of potentially confounding factors selected based on clinical knowledge. These were entered into a “full model,” and variable reduction was performed to derive a “final model” based on the plausibility of regression coefficients, association between independent variables and the magnitude of change in the association between study group and predicted geometric mean sTRAIL concentration. Statistical analysis was performed with SPSS 19 (IBM Corp, Armonk, NY) and SAS version 9.3 (Cary, NC). A p value < 0.05 was considered statistically significant.
Results
Demographic, clinical and obstetric characteristics are displayed in by study group: (1) non-pregnant women (n = 23); (2) pregnant women who had an uncomplicated pregnancies (n = 93) and, (3) pregnant women with acute pyelonephritis (n = 23). The frequency of nulliparous women was greater in non-pregnant group than in the uncomplicated pregnancy and pregnancy with acute pyelonephritis groups. Otherwise, there were no significant differences in characteristics between study groups.
Table 1. Clinical characteristics by study group: (1) non-pregnant, (2) uncomplicated pregnancy and (3) pregnancy with acute pyelonephritis.
sTRAIL was detected in 97.8% (136/139) of samples. Pregnant women with acute pyelonephritis had a lower mean ± SD plasma sTRAIL concentration (pg/mL) than women with uncomplicated pregnancies (20.5 ± 6.6 versus 31.5 ± 10.1; p < 0.001; ). The mean ± SD plasma sTRAIL concentration (pg/mL) was significantly lower in the uncomplicated pregnancy than in the non-pregnant group (31.5 ± 10.1 versus 53.3 ± 12.5; p < 0.001; ). These differences remained significant after adjusting for nulliparity; other potentially confounding factors (maternal age and race) were not significantly associated with sTRAIL concentrations, and their inclusion in a multivariable model did not alter the magnitude or significance of its association with study group (least squares mean sTRAIL for each group changed by <1% following multivariable adjustment).
Figure 1. The mean maternal plasma sTRAIL concentration in non-pregnant women, uncomplicated pregnancy and pregnancy with acute pyelonephritis. Women with uncomplicated pregnancy had a significantly lower mean maternal plasma sTRAIL concentration than non-pregnant women (mean ± SD: 31.5 ± 10.1 pg/mL versus mean ± SD: 53.3 ± 12.5 pg/mL; p < 0.001). Pregnant women with acute pyelonephritis had a significantly lower mean maternal plasma sTRAIL concentration than those with uncomplicated pregnancy (mean ± SD: 20.5 ± 6.6 pg/mL versus mean ± SD: 31.5 ± 10.1 pg/mL; p < 0.001).
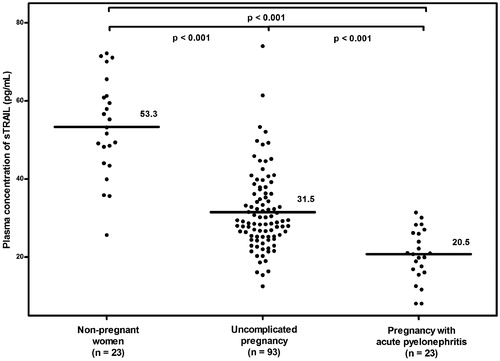
Among pregnant patients, plasma sTRAIL concentrations were not associated with gestational age at venipuncture (β = −0.0008, p = 0.75), after holding study group constant. Among women with acute pyelonephritis (), the mean plasma sTRAIL concentration was significantly lower in those with bacteremia than in those without bacteremia (15.1 ± 4.8 versus 24.7 ± 4.6; p < 0.001; ). Adjustment for maternal age, race, nulliparity and gestational age at venipuncture did not alter the magnitude or significance of this relation.
Figure 2. The mean maternal plasma sTRAIL concentration in pregnancy with acute pyelonephritis without bacteremia and those with bacteremia. Pregnant women with acute pyelonephritis who had bacteremia had a significantly lower mean maternal plasma concentration of sTRAIL than those without bacteremia (mean ± SD: 15.1 ± 4.8 pg/mL versus mean ± SD: 24.7 ± 4.6 pg/mL; p < 0.001).
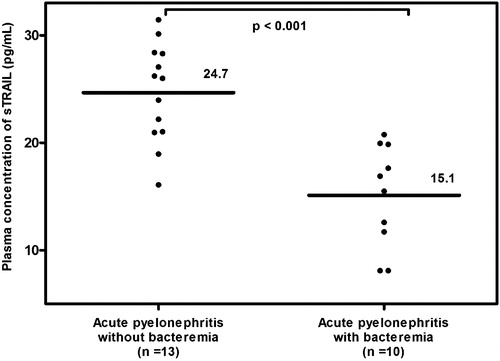
Table 2. Clinical characteristics by culture status among women in the acute pyelonephritis group.
Discussion
Principal findings of the study
(1) The mean plasma sTRAIL concentration was lower in pregnant than in non-pregnant women; (2) pregnant women with acute pyelonephritis had a lower mean plasma sTRAIL concentration than in those with uncomplicated pregnancies and (3) among pregnant women with acute pyelonephritis, those with bacteremia had a lower mean plasma sTRAIL concentration than those without bacteremia.
Biology of TRAIL
TRAIL, or Apo2L, is a membrane protein which is the third pro-apoptotic member other than TNF and FAS of the TNF family [Citation20,Citation21,Citation51,Citation52]. TRAIL can induce apoptosis signals through death domains. Binding of TRAIL to its death receptors, TRAIL-R1 or TRAIL-R2, results in the formation of death-inducing signaling complex (DISC). Fas-associated death domain (FADD) translocates and recruits pro-caspase-8 and -10 to DISC result in apoptosis [Citation53–58]. In contrast, binding of TRAIL to TRAIL-R3/Decoy receptor 1 (DcR1) [Citation59–63] or TRAIL-R4/DcR2 [Citation62,Citation63] cannot induce an apoptotic signal; therefore, both are considered decoy receptors. TRAIL can also bind to osteoprotegerin, a protein involved in bone remodeling that acts through ligands other than TRAIL, with a low affinity [Citation64,Citation65]. TRAIL is expressed predominantly in the spleen, lung, prostate, immune cells (e.g. neutrophils, monocytes, lymphocytes, dendritic cells, natural killer cells, macrophage), endothelial cells, syncytiotrophoblasts, cytotrophoblasts and decidual cells [Citation20,Citation25,Citation26,Citation59,Citation61,Citation66]. The soluble form of TRAIL also elicits apoptosis signals similar to that of the cell surface TRAIL [Citation67,Citation68].
Unlike other death receptor ligands of the TNF family, TRAIL is unique because it induces apoptosis of transformed cells (i.e. cancer) but not in the majority of normal cells [Citation69,Citation70]. The presence of decoy receptors for TRAIL in normal (but not in tumor cells) could partly explain why most normal cells are resistant to TRAIL-induced apoptosis [Citation61,Citation71]. This unique property has led to investigation using recombinant TRAIL for therapy, especially in the field of oncology [Citation72,Citation73]. In addition to apoptotic signaling, TRAIL can also elicit non-apoptotic signals such as nuclear factor kappa B (NF-κb) [Citation51,Citation74,Citation75], extracellular signal-regulated kinase [Citation51,Citation52,Citation69,Citation76] and c-Jun NH2-terminal kinase pathways depending on cell types [Citation51,Citation52,Citation57,Citation62,Citation66,Citation77]. The biological significance of NF-κB activation by TRAIL, however, has not been fully elucidated [Citation78].
TRAIL knock-out mice develop normally and do not develop tumors spontaneously [Citation52]. However, they are more susceptible to metastasis of implanted tumors (Renca tumor cell, mammary carcinoma, and fibrosarcoma) [Citation79,Citation80]. Recently, TRAIL has been implicated in the pathophysiology of autoimmune disease, since TRAIL-deficient mice have increased numbers of immature thymocytes, impaired thymic deletion, and develop collagen-induced arthritis and streptozocin-induced diabetes [Citation81].
sTRAIL is detectable in the serum/plasma of healthy individuals [Citation82–84] and is increased in patients with autoimmune diseases (such as systemic sclerosis, systemic lupus erythematosus, rheumatoid arthritis) [Citation82,Citation85,Citation86] and viral infection such as human immunodeficiency virus (HIV) [Citation87]. In contrast, serum/plasma concentrations of sTRAIL are decreased in patients with ischemic heart disease [Citation88,Citation89].
Roles of TRAIL at feto-maternal interface
TRAIL and its receptors (TRAIL-R) can be detected in the placenta and fetal membranes throughout gestation [Citation25,Citation26]. TRAIL receptor expression in villous trophoblasts changes with advancing gestational age (TRAIL-R1 and TRAIL-R2 increase, while TRAIL-R3 and TRAIL-R4 decrease) [Citation25,Citation26]. Immunohistochemistry studies have reported that TRAIL expression is more prominent in syncytiotrophoblasts than cytotrophoblasts [Citation25,Citation26], whereas the distribution of the TRAIL receptor differs depending on the type of receptor. TRAIL-R1 and TRAIL-R2 were mainly localized in cytotrophoblasts, while TRAIL-R3 and TRAIL-R4 (decoy receptors) were confined to syncytiotrophoblasts [Citation25,Citation26]. Since syncytiotrophoblasts are in direct contact with maternal blood containing immune cells, the distribution of TRAIL and its receptors on syncytiotrophoblast may confer protection of trophoblasts against immune cells [Citation27]. Moreover, the TRAIL receptor can be translocated inside cells. For example, in a model of villous cytotrophoblast culture, the administration of recombinant TRAIL did not increase trophoblast apoptosis, but relocated TRAIL-R2 from the cytoplasm into the nucleus. This phenomenon may explain the resistance of trophoblasts to TRAIL-induced apoptosis [Citation90]. In contrast, TNF-α treatment enhanced TRAIL-induced apoptosis by relocalizing TRAIL-R2 from the nucleus to the plasma membrane [Citation90].
Plasma concentration of sTRAIL in women with uncomplicated pregnancies
The study reported herein is the first to demonstrate that the mean plasma concentration of sTRAIL is significantly lower in pregnant than in non-pregnant women. Moreover, its concentration in women with uncomplicated pregnancies remains largely unchanged throughout the second half of pregnancy. The lack of correlation between plasma concentrations of sTRAIL and gestational age in this study is consistent with the findings of Zauli et al. [Citation91], who reported that serum concentrations of sTRAIL were stable from 12 to 16 weeks of gestation. In contrast, others have shown that the amniotic fluid concentration of sTRAIL is significantly higher at term than those in midtrimester [Citation27].
Recently, Agostinis et al. [Citation30] demonstrated that maternal serum concentrations of sTRAIL in patients with recurrent miscarriage were higher than in normal pregnant women at 12 weeks of gestation [Citation30]. Moreover, they found that soluble recombinant TRAIL inhibits human first-trimester-derived trophoblastic cell migration and adhesion to decidual endothelial cells and fibronectin in vitro [Citation30]. However, this study was unable to determine whether elevated sTRAIL concentrations caused or was a consequence of miscarriage. Furthermore, it is noteworthy that the sTRAIL concentration which inhibited migration and adhesion of trophoblast to decidual endothelial cells is similar to that found in the serum of pregnant women with uncomplicated pregnancies [Citation91].
Our results suggest that lower maternal plasma concentrations of sTRAIL in women with uncomplicated pregnancies than non-pregnant women may explain, at least in part, the neutrophilia observed in normal pregnancy [Citation17,Citation18]. The pathophysiologic significance and mechanisms of lower plasma sTRAIL concentrations in women with uncomplicated pregnancies requires further investigation.
Plasma concentration of sTRAIL in pregnancies with acute pyelonephritis
TRAIL may be involved in regulation of the immune response against infection, since it is expressed on almost every type of immune cell (e.g., neutrophils, monocytes, macrophages, CD4+ T cells, NK cells and dendritic cells) [Citation92–94]. Although most studies have examined the role of TRAIL in viral infections such as HIV [Citation87,Citation95,Citation96], cytomegalovirus [Citation97], influenza [Citation98,Citation99], reovirus [Citation100] and respiratory syncytial virus [Citation101], this protein is also involved in regulation of inflammatory response to bacterial infections [Citation102]. Indeed, TRAIL and its receptors have been implicated in the removal of senescent circulating neutrophils and activated tissue neutrophils, leading to resolution of inflammation [Citation23,Citation24]. In an animal model of endotoxin-induced acute lung injury and zymoman-induced peritonitis, TRAIL-knock-out mice had an enhanced inflammatory response with increased neutrophil numbers and reduced neutrophil apoptosis in bronchoalveolar and peritoneal lavage fluid, respectively [Citation24]. Similarly, in an animal model of pneumococcal-induced pneumonia, TRAIL knock-out mice had significantly decreased lung bacterial clearance and survival when compared to wild-type mice [Citation103]. The alveolar macrophage cell death in these mice shifted from apoptosis to necrosis [Citation103]. Moreover, the inflammatory response (TNF, interleukin-6, chemokine (C-X-C motif) ligand 1, and interferon gamma) in the lungs was increased, indicating that TRAIL regulates the inflammatory response elicited by bacterial infection [Citation103]. In an animal model of pneumococcal-induced meningitis, TRAIL knock-out mice had prolonged inflammation, impaired coordination, decreased motor strength, and increased neuronal cell death than in wild-type mice [Citation104]. Moreover, intrathecal administration of recombinant TRAIL corrected these deficits [Citation104]. Taken together, these observations suggest that TRAIL is involved in inflammatory signaling cascades and is a protective cytokine against an exaggerated proinflammatory response during the course of acute infection [Citation23].
Our findings that pregnant women with acute pyelonephritis (especially those with bacteremia) had a lower mean plasma concentration of sTRAIL than those with uncomplicated pregnancies are consistent with previous experimental models demonstrated that TRAIL is a protective cytokine against bacterial infection [Citation23,Citation24,Citation103,Citation104]. The decrease in plasma concentrations of sTRAIL in the setting of acute infection may be associated with an increased inflammatory response to bacterial products, probably due to delayed neutrophil apoptosis. This hypothesis may partly explain why pregnant women with acute pyelonephritis are more susceptible to complications such as SIRS or ARDS than non-pregnant women. Moreover, maternal plasma sTRAIL concentration is related to the severity of bacterial infection.
Strengths and limitations
This is the first study to examine differences in maternal plasma concentrations of sTRAIL among non-pregnant women, women with uncomplicated pregnancy, and those with acute pyelonephritis. Due to the cross-sectional nature of the study, a temporal relationship of the changes in plasma sTRAIL concentrations and the development of acute infection during pregnancy could not be established.
Conclusions
Maternal plasma concentrations of sTRAIL are lower in women with uncomplicated pregnancies than in non-pregnant women and are further decreased among pregnant women with acute pyelonephritis, particularly in those with evidence of bacteremia. Low plasma sTRAIL concentration could account, at least in part, for the exaggerated intravascular inflammatory response previously reported in pyelonephritis during pregnancy.
Declaration of interest
The authors report no declarations of interest. This project has been funded in whole or in part with Federal funds from the Eunice Kennedy Shriver National Institute of Child Health and Human Development, National Institutes of Health, Department of Health and Human Services, under Contract No. HHSN275201300006C.
References
- Sacks G, Sargent I, Redman C. An innate view of human pregnancy. Immunol Today 1999;20:114–18
- Naccasha N, Gervasi MT, Chaiworapongsa T, et al. Phenotypic and metabolic characteristics of monocytes and granulocytes in normal pregnancy and maternal infection. Am J Obstet Gynecol 2001;185:1118–23
- Chen X, Christou NV. Relative contribution of endothelial cell and polymorphonuclear neutrophil activation in their interactions in systemic inflammatory response syndrome. Arch Surg 1996;131:1148–53; discussion 1153–44
- Mori W. The Shwartzman reaction: a review including clinical manifestations and proposal for a univisceral or single organ third type. Histopathology 1981;5:113–26
- Romero R, Gotsch F, Pineles B, Kusanovic JP. Inflammation in pregnancy: its roles in reproductive physiology, obstetrical complications, and fetal injury. Nutr Rev 2007;65:S194–202
- Muller-Berghaus G, Schmidt-Ehry B. The role of pregnancy in the induction of the generalized Shwartzman reaction. Am J Obstet Gynecol 1972;114:847–49
- Hankins GD, Whalley PJ. Acute urinary tract infections in pregnancy. Clin Obstet Gynecol 1985;28:266–78
- Moritz AR, Weir D. Unilateral inhibition of the renal Shwartzman phenomenon following injection of bacterial filtrate into the renal artery. J Exp Med 1937;66:755–60
- Thomas L, Good RA. Studies on the generalized Shwartzman reaction: I. General observations concerning the phenomenon. J Exp Med 1952;96:605–24
- Haslett C, Savill JS, Meagher L. The neutrophil. Curr Opin Immunol 1989;2:10–18
- Gervasi MT, Chaiworapongsa T, Naccasha N, et al. Maternal intravascular inflammation in preterm premature rupture of membranes. J Matern Fetal Neonatal Med 2002;11:171–5
- Gervasi MT, Chaiworapongsa T, Naccasha N, et al. Phenotypic and metabolic characteristics of maternal monocytes and granulocytes in preterm labor with intact membranes. Am J Obstet Gynecol 2001;185:1124–9
- Gervasi MT, Chaiworapongsa T, Pacora P, et al. Phenotypic and metabolic characteristics of monocytes and granulocytes in preeclampsia. Am J Obstet Gynecol 2001;185:792–7
- McGill SN, Ahmed NA, Hu F, et al. Shedding of L-selectin as a mechanism for reduced polymorphonuclear neutrophil exudation in patients with the systemic inflammatory response syndrome. Arch Surg 1996;131:1141–6; discussion 1147
- Sacks GP, Studena K, Sargent K, Redman CW. Normal pregnancy and preeclampsia both produce inflammatory changes in peripheral blood leukocytes akin to those of sepsis. Am J Obstet Gynecol 1998;179:80–6
- Sabatier F, Bretelle F, D’Ercole C, et al. Neutrophil activation in preeclampsia and isolated intrauterine growth restriction. Am J Obstet Gynecol 2000;183:1558–63
- Efrati P, Presentey B, Margalith M, Rozenszajn L. Leukocytes of normal pregnant women. Obstet Gynecol 1964;23:429–32
- von Dadelszen P, Watson RW, Noorwali F, et al. Maternal neutrophil apoptosis in normal pregnancy, preeclampsia, and normotensive intrauterine growth restriction. Am J Obstet Gynecol 1999;181:408–14
- Jimenez MF, Watson RW, Parodo J, et al. Dysregulated expression of neutrophil apoptosis in the systemic inflammatory response syndrome. Arch Surg 1997;132:1263–9; discussion 1269–70
- Wiley SR, Schooley K, Smolak PJ, et al. Identification and characterization of a new member of the TNF family that induces apoptosis. Immunity 1995;3:673–82
- Pitti RM, Marsters SA, Ruppert S, et al. Induction of apoptosis by Apo-2 ligand, a new member of the tumor necrosis factor cytokine family. J Biol Chem 1996;271:12687–90
- Renshaw SA, Parmar JS, Singleton V, et al. Acceleration of human neutrophil apoptosis by TRAIL. J Immunol 2003;170:1027–33
- Cziupka K, Busemann A, Partecke LI, et al. Tumor necrosis factor-related apoptosis-inducing ligand (TRAIL) improves the innate immune response and enhances survival in murine polymicrobial sepsis. Crit Care Med 2010;38:2169–74
- McGrath EE, Marriott HM, Lawrie A, et al. TNF-related apoptosis-inducing ligand (TRAIL) regulates inflammatory neutrophil apoptosis and enhances resolution of inflammation. J Leukoc Biol 2011;90:855–65
- Chen L, Liu X, Zhu Y, et al. Localization and variation of TRAIL and its receptors in human placenta during gestation. Life Sci 2004;74:1479–86
- Phillips TA, Ni J, Pan G, et al. TRAIL (Apo-2L) and TRAIL receptors in human placentas: implications for immune privilege. J Immunol 1999;162:6053–9
- Lonergan M, Aponso D, Marvin KW, et al. Tumor necrosis factor-related apoptosis-inducing ligand (TRAIL), TRAIL receptors, and the soluble receptor osteoprotegerin in human gestational membranes and amniotic fluid during pregnancy and labor at term and preterm. J Clin Endocrinol Metab 2003;88:3835–44
- Keogh RJ, Harris LK, Freeman A, et al. Fetal-derived trophoblast use the apoptotic cytokine tumor necrosis factor-alpha-related apoptosis-inducing ligand to induce smooth muscle cell death. Circ Res 2007;100:834–41
- Whitley GS, Cartwright JE. Trophoblast-mediated spiral artery remodelling: a role for apoptosis. J Anat 2009;215:21–6
- Agostinis C, Bulla R, Tisato V, et al. Soluble TRAIL is elevated in recurrent miscarriage and inhibits the in vitro adhesion and migration of HTR8 trophoblastic cells. Hum Reprod 2012;27:2941–7
- Soto E, Romero R, Vaisbuch E, et al. Fragment Bb: evidence for activation of the alternative pathway of the complement system in pregnant women with acute pyelonephritis. J Matern Fetal Neonatal Med 2010;23:1085–90
- Chaiworapongsa T, Romero R, Gotsch F, et al. Acute pyelonephritis during pregnancy changes the balance of angiogenic and anti-angiogenic factors in maternal plasma. J Matern Fetal Neonatal Med 2010;23:167–78
- Gotsch F, Romero R, Espinoza J, et al. Maternal serum concentrations of the chemokine CXCL10/IP-10 are elevated in acute pyelonephritis during pregnancy. J Matern Fetal Neonatal Med 2007;20:735–44
- Soto E, Richani K, Romero R, et al. Increased concentration of the complement split product C5a in acute pyelonephritis during pregnancy. J Matern Fetal Neonatal Med 2005;17:247–52
- Kusanovic JP, Romero R, Esoinoza J, et al. Maternal serum soluble CD30 is increased in pregnancies complicated with acute pyelonephritis. J Matern Fetal Neonatal Med 2007;20:803–11
- Jolley JA, Kim S, Wing DA. Acute pyelonephritis and associated complications during pregnancy in 2006 in US hospitals. J Matern Fetal Neonatal Med 2012;25:2494--8
- Cunningham FG, Leveno KJ, Hankins GD, Whalley PJ. Respiratory insufficiency associated with pyelonephritis during pregnancy. Obstet Gynecol 1984;63:121–5
- Amstey MS. Frequency of adult respiratory distress syndrome in pregnant women who have pyelonephritis. Clin Infect Dis 1992;14:1260–1
- Catanzarite VA, Willms D. Adult respiratory distress syndrome in pregnancy: report of three cases and review of the literature. Obstet Gynecol Surv 1997;52:381–92
- Cunningham FG, Lucas MJ, Hankins GD. Pulmonary injury complicating antepartum pyelonephritis. Am J Obstet Gynecol 1987;156:797–807
- Pruett K, Faro S. Pyelonephritis associated with respiratory distress. Obstet Gynecol 1987;69:444–6
- Cunningham FG, Lucas MJ. Urinary tract infections complicating pregnancy. Baillieres Clin Obstet Gynaecol 1994;8:353–73
- Elkington KW, Greb LC. Adult respiratory distress syndrome as a complication of acute pyelonephritis during pregnancy: case report and discussion. Obstet Gynecol 1986;67:18S–20S
- Soisson AP, Eldridge E, Kopelman JN, Duff P. Acute pyelonephritis complicated by respiratory insufficiency. A case report. J Reprod Med 1986;31:525–7
- Towers CV, Kaminskas CM, Garite TJ, et al. Pulmonary injury associated with antepartum pyelonephritis: can patients at risk be identified? Am J Obstet Gynecol 1991;164:974–8; discussion 978–80
- Yazigi R, Lerner S, Tejani N. Association of acute pyelonephritis with pulmonary complications in pregnancy. A report of two cases. J Reprod Med 1990;35:562–4
- Bubeck RW. Acute pyelonephritis during pregnancy with anuria, septicemia and thrombocytopenia. Del Med J 1968;40:143–7
- Cunningham FG, Morris GB, Mickal A. Acute pyelonephritis of pregnancy: a clinical review. Obstet Gynecol 1973;42:112–17
- Snyder CC, Barton JR, Habli M, Sibai BM. Severe sepsis and septic shock in pregnancy: indications for delivery and maternal and perinatal outcomes. J Matern Fetal Neonatal Med 2013;26:503--6
- Gilstrap LC 3rd, Cunningham FG, Whalley PJ. Acute pyelonephritis in pregnancy: an anterospective study. Obstet Gynecol 1981;57:409–13
- Falschlehner C, Emmerich CH, Gerlach B, Walczak H. TRAIL signalling: decisions between life and death. Int J Biochem Cell Biol 2007;39:1462–75
- Di Pietro R, Zauli G. Emerging non-apoptotic functions of tumor necrosis factor-related apoptosis-inducing ligand (TRAIL)/Apo2L. J Cell Physiol 2004;201:331–40
- Bodmer JL, Holler N, Reynard S, et al. TRAIL receptor-2 signals apoptosis through FADD and caspase-8. Nat Cell Biol 2000;2:241–3
- Kischkel FC, Lawrence DA, Chuntharapai A, et al. Apo2L/TRAIL-dependent recruitment of endogenous FADD and caspase-8 to death receptors 4 and 5. Immunity 2000;12:611–20
- Sprick MR, Weigand MA, Rieser E, et al. FADD/MORT1 and caspase-8 are recruited to TRAIL receptors 1 and 2 and are essential for apoptosis mediated by TRAIL receptor 2. Immunity 2000;12:599–609
- Kischkel FC, Hellbardt S, Behrmann I, et al. Cytotoxicity-dependent APO-1 (Fas/CD95)-associated proteins form a death-inducing signaling complex (DISC) with the receptor. EMBO J 1995;14:5579–88
- Schneider P, Thome M, Burns K, et al. TRAIL receptors 1 (DR4) and 2 (DR5) signal FADD-dependent apoptosis and activate NF-kappaB. Immunity 1997;7:831–6
- Rossi D, Gaidano G. Messengers of cell death: apoptotic signaling in health and disease. Haematologica 2003;88:212–8
- Pan G, Ni J, Wei YF, et al. An antagonist decoy receptor and a death domain-containing receptor for TRAIL. Science 1997;277:815–18
- Schneider P, Bodmer JL, Thome M, et al. Characterization of two receptors for TRAIL. FEBS Lett 1997;416:329–34
- Sheridan JP, Marsters SA, Pitti RM, et al. Control of TRAIL-induced apoptosis by a family of signaling and decoy receptors. Science 1997;277:818–21
- Degli-Esposti MA, Smolak PJ, Walczak H, et al. Cloning and characterization of TRAIL-R3, a novel member of the emerging TRAIL receptor family. J Exp Med 1997;186:1165–70
- Marsters SA, Sheridan JP, Pitti RM, et al. A novel receptor for Apo2L/TRAIL contains a truncated death domain. Curr Biol 1997;7:1003–6
- Simonet WS, Lacey DL, Dunstan CR, et al. Osteoprotegerin: a novel secreted protein involved in the regulation of bone density. Cell 1997;89:309–19
- Emery JG, McDonnell P, Burke MB, et al. Osteoprotegerin is a receptor for the cytotoxic ligand TRAIL. J Biol Chem 1998;273:14363–7
- Chaudhary PM, Eby M, Jasmin A, et al. Death receptor 5, a new member of the TNFR family, and DR4 induce FADD-dependent apoptosis and activate the NF-kappaB pathway. Immunity 1997;7:821–30
- Wajant H, Moosmayer D, Wuest T, et al. Differential activation of TRAIL-R1 and -2 by soluble and membrane TRAIL allows selective surface antigen-directed activation of TRAIL-R2 by a soluble TRAIL derivative. Oncogene 2001;20:4101–6
- Tecchio C, Huber V, Scapini P, et al. IFNalpha-stimulated neutrophils and monocytes release a soluble form of TNF-related apoptosis-inducing ligand (TRAIL/Apo-2 ligand) displaying apoptotic activity on leukemic cells. Blood 2004;103:3837–44
- Secchiero P, Gonelli A, Carnevale E, et al. TRAIL promotes the survival and proliferation of primary human vascular endothelial cells by activating the Akt and ERK pathways. Circulation 2003;107:2250–6
- Daniels RA, Turley H, Kimberley FC, et al. Expression of TRAIL and TRAIL receptors in normal and malignant tissues. Cell Res 2005;15:430–8
- Zhang XD, Nguyen T, Thomas WD, et al. Mechanisms of resistance of normal cells to TRAIL induced apoptosis vary between different cell types. FEBS Lett 2000;482:193–9
- Holoch PA, Griffith TS. TNF-related apoptosis-inducing ligand (TRAIL): a new path to anti-cancer therapies. Eur J Pharmacol 2009;625:63–72
- Zauli G, Bosco R, Secchiero P. Molecular targets for selective killing of TRAIL-resistant leukemic cells. Expert Opin Ther Targets 2011;15:931–42
- Lin Y, Devin A, Cook A, et al. The death domain kinase RIP is essential for TRAIL (Apo2L)-induced activation of IkappaB kinase and c-Jun N-terminal kinase. Mol Cell Biol 2000;20:6638–45
- Collison A, Foster PS, Mattes J. Emerging role of tumour necrosis factor-related apoptosis-inducing ligand (TRAIL) as a key regulator of inflammatory responses. Clin Exp Pharmacol Physiol 2009;36:1049–53
- Milani D, Zauli G, Rimondi E, et al. Tumour necrosis factor-related apoptosis-inducing ligand sequentially activates pro-survival and pro-apoptotic pathways in SK-N-MC neuronal cells. J Neurochem 2003;86:126–35
- Muhlenbeck F, Haas E, Schwenzer R, et al. TRAIL/Apo2L activates c-Jun NH2-terminal kinase (JNK) via caspase-dependent and caspase-independent pathways. J Biol Chem 1998;273:33091–8
- Yerbes R, Palacios C, Lopez-Rivas A. The therapeutic potential of TRAIL receptor signalling in cancer cells. Clin Transl Oncol 2011;13:839–47
- Cretney E, Takeda K, Yagita H, et al. Increased susceptibility to tumor initiation and metastasis in TNF-related apoptosis-inducing ligand-deficient mice. J Immunol 2002;168:1356–61
- Sedger LM, Glaccum MB, Schuh JC, et al. Characterization of the in vivo function of TNF-alpha-related apoptosis-inducing ligand, TRAIL/Apo2L, using TRAIL/Apo2L gene-deficient mice. Eur J Immunol 2002;32:2246–54
- Lamhamedi-Cherradi SE, Zheng SJ, Maguschak KA, et al. Defective thymocyte apoptosis and accelerated autoimmune diseases in TRAIL-/- mice. Nat Immunol 2003;4:255–60
- Azab NA, Rady HM, Marzouk SA. Elevated serum TRAIL levels in scleroderma patients and its possible association with pulmonary involvement. Clin Rheumatol 2012;31:1359--64
- Mori K, Ikari Y, Jono S, et al. Association of serum TRAIL level with coronary artery disease. Thromb Res 2010;125:322–5
- Bisgin A, Kargi A, Yalcin AD, et al. Increased serum sTRAIL levels were correlated with survival in bevacizumab-treated metastatic colon cancer. BMC Cancer 2012;12:1--5
- Lub-de Hooge MN, de Vries EG, de Jong S, Bijl M. Soluble TRAIL concentrations are raised in patients with systemic lupus erythematosus. Ann Rheum Dis 2005;64:854–8
- Audo R, Calmon-Hamaty F, Baeten D, et al. Mechanisms and clinical relevance of TRAIL-triggered responses in the synovial fibroblasts of patients with rheumatoid arthritis. Arthritis Rheum 2011;63:904–13
- Herbeuval JP, Boasso A, Grivel JC, et al. TNF-related apoptosis-inducing ligand (TRAIL) in HIV-1-infected patients and its in vitro production by antigen-presenting cells. Blood 2005;105:2458–64
- Deftereos S, Giannopoulos G, Kossyvakis C, et al. Association of soluble tumour necrosis factor-related apoptosis-inducing ligand levels with coronary plaque burden and composition. Heart 2012;98:214–8
- Volpato S, Ferrucci L, Secchiero P, et al. Association of tumor necrosis factor-related apoptosis-inducing ligand with total and cardiovascular mortality in older adults. Atherosclerosis 2011;215:452–8
- Bai X, Williams JL, Greenwood SL, et al. A placental protective role for trophoblast-derived TNF-related apoptosis-inducing ligand (TRAIL). Placenta 2009;30:855–60
- Zauli G, Monasta L, Rimondi E, et al. Circulating TRAIL shows a significant post-partum decline associated to stressful conditions. PLoS One 2011;6:e27011--9
- Kayagaki N, Yamaguchi N, Nakayama M, et al. Involvement of TNF-related apoptosis-inducing ligand in human CD4+ T cell-mediated cytotoxicity. J Immunol 1999;162:2639–47
- Kaplan MJ, Ray D, Mo RR, et al. TRAIL (Apo2 ligand) and TWEAK (Apo3 ligand) mediate CD4+ T cell killing of antigen-presenting macrophages. J Immunol 2000;164:2897–904
- Wallin RP, Screpanti V, Michaelsson J, et al. Regulation of perforin-independent NK cell-mediated cytotoxicity. Eur J Immunol 2003;33:2727–35
- Zhu DM, Shi J, Liu S, et al. HIV infection enhances TRAIL-induced cell death in macrophage by down-regulating decoy receptor expression and generation of reactive oxygen species. PLoS One 2011;6:e18291--9
- Miura Y, Misawa N, Maeda N, et al. Critical contribution of tumor necrosis factor-related apoptosis-inducing ligand (TRAIL) to apoptosis of human CD4+ T cells in HIV-1-infected hu-PBL-NOD-SCID mice. J Exp Med 2001;193:651–60
- Andrews JI, Griffith TS, Meier JL. Cytomegalovirus and the role of interferon in the expression of tumor necrosis factor-related apoptosis-inducing ligand in the placenta. Am J Obstet Gynecol 2007;197:608 e601–6
- Brincks EL, Katewa A, Kucaba TA, et al. CD8 T cells utilize TRAIL to control influenza virus infection. J Immunol 2008;181:4918–25
- Herold S, Steinmueller M, von Wulffen W, et al. Lung epithelial apoptosis in influenza virus pneumonia: the role of macrophage-expressed TNF-related apoptosis-inducing ligand. J Exp Med 2008;205:3065–77
- Clarke P, Meintzer SM, Gibson S, et al. Reovirus-induced apoptosis is mediated by TRAIL. J Virol 2000;74:8135–9
- Bem RA, Bos AP, Wosten-van Asperen RM, et al. Potential role of soluble TRAIL in epithelial injury in children with severe RSV infection. Am J Respir Cell Mol Biol 2010;42:697–705
- Gurung P, Rai D, Condotta SA, et al. Immune unresponsiveness to secondary heterologous bacterial infection after sepsis induction is TRAIL dependent. J Immunol 2011;187:2148–54
- Steinwede K, Henken S, Bohling J, et al. TNF-related apoptosis-inducing ligand (TRAIL) exerts therapeutic efficacy for the treatment of pneumococcal pneumonia in mice. J Exp Med 2012;209:1937–52
- Hoffmann O, Priller J, Prozorovski T, et al. TRAIL limits excessive host immune responses in bacterial meningitis. J Clin Invest 2007;117:2004–13