Abstract
Background: In workplace respiratory disease prevention, a thorough understanding is needed of the relative contributions of lung function loss and respiratory symptoms in predicting adverse health outcomes. Methods: Copenhagen City Heart Study respiratory data collected at 4 examinations (1976–2003) and morbidity and mortality data were used to investigate these relationships. With 15 or more years of follow-up for a hospital diagnosis of chronic obstructive pulmonary disease (COPD) morbidity, COPD or coronary heart disease (CHD) mortality, and all-cause mortality, risks for these outcomes were estimated in relation to asthma, chronic bronchitis, shortness of breath, and lung function level at examination 2 (1981–1983) or lung function decline established from examinations 1 (1976–1978) to 2 using 4 measures (FEV1 slope, FEV1 relative slope, American College of Occupational and Environmental Medicine's Longitudinal Normal Limit [LNL], or a limit of 90 milliliters per year [ml/yr]). These risks were estimated by hazard ratios (HR) and 95% confidence intervals (CI) adjusted for age, height-adjusted baseline forced expiratory volume in 1 second (FEV1/height2), and height. Results: For COPD morbidity, the increasing trend in the HR (95% CI) by quartiles of the FEV1 slope reached a maximum of 3.77 (2.76–5.15) for males, 6.12 (4.63–8.10) for females, and 4.14 (1.57–10.90) for never-smokers. Significant increasing trends were also observed for mortality, with females at higher risk. Conclusion: Lung function decline was associated with increased risk of COPD morbidity and mortality emphasizing the need to monitor lung function change over time in at-risk occupational populations.
INTRODUCTION
Studies of various populations demonstrate that lung function can predict adverse health outcomes, such as chronic obstructive pulmonary disease (COPD) morbidity and mortality, coronary heart disease (CHD) mortality, and all-cause mortality (Citation1–16). COPD is a costly disease, with high prevalence rates in the United States and internationally, high associated healthcare costs, early retirement from work, and excess premature mortality. COPD is also associated with CHD, another costly disease (Citation17–24). While the principal risk factor for COPD is tobacco smoking, occupational exposures also contribute to the development of COPD and its cost (Citation22,Citation25–33). Industry-based studies identify increased healthcare utilization and disability associated with COPD, and demonstrate the need for prevention of excessive loss of lung function (Citation34,35). In workers with potential exposure to occupational respiratory hazards, effective monitoring of longitudinal lung function changes is one approach to early recognition of lung function impairment and provides opportunities for developing prevention strategies to reduce potentially modifiable risk factors associated with long-term adverse health outcomes.
Periodic lung function evaluation can support effective prevention strategies in the workplace. Important issues to consider are: the critical rate at which lung function decline becomes a significant predictor of morbidity and mortality; the measures of lung function decline that best predict morbidity and mortality; and the role of respiratory symptoms and asthma in the prediction. Specific cut-off points in the forced expiratory volume in 1 second (FEV1) rate of decline were considered in a previous study of mortality in coal miners (Citation36). This study showed increased mortality risk with rates of decline above about 60 ml/yr in never-smokers and with declines of 90 ml/yr in all miners; furthermore, the decline of 60 ml/yr or more was associated with increased disability and early retirement (Citation22).
The aim of this study was to evaluate the risks of COPD morbidity based on hospitalizations, COPD or CHD mortality, and all-cause mortality associated with excessive lung function decline, current respiratory symptoms of chronic bronchitis and shortness of breath, and current asthma in an aging community cohort. Specific objectives were to evaluate the usefulness of various measures of lung function decline in predicting morbidity and mortality, to investigate the critical rate of lung function decline that becomes a significant predictor of morbidity and mortality, and to assess the usefulness of current respiratory symptoms and asthma in predicting morbidity and mortality relative to lung function level and decline.
MATERIALS AND METHODS
Study Population and Definitions
Our study used data from the Copenhagen City Heart Study, a prospective study of cardiovascular disease in 23,000 men and women aged 20 years and older (Citation37, 38). The study involved 4 clinical examinations (including spirometry) and a self-administered questionnaire conducted over a 28-year period (1976–78, 1981–83, 1991–94, and 2001–03). A minimum of 3 spirometry tests were performed at each session, in a sitting position, using an electronic spirometer (N 403 Monaghan, United States). At least 2 measurements had to be within 5% of one another (Citation37,38). For analysis of lung function decline, we selected subjects who had spirometry tests at examinations 1 and 2, approximately 5 years apart. Change in FEV1 was evaluated because it is the most useful measurement for assessing the rate of lung function decline (Citation39). Height measurements were collected as part of the physical examination. Age and gender data were obtained from the Copenhagen Population Register at the time of study enrollment. Smoking status was self-reported at each examination.
Current respiratory symptoms of chronic bronchitis and current asthma were self-reported at examinations 1 and 2, while shortness of breath was initially ascertained at examination 2. In preliminary univariate analyses, we identified asthma (“Do you suffer from asthma?”) (Citation40), chronic bronchitis (“Do you bring up phlegm, in the morning or during the day, for as long as 3 months each year?”), and shortness of breath (“Do you often feel out of breath?”) as the best predictors of future morbidity and mortality.
Morbidity data were from the National Patient Register, mortality data from the Civil Registration System, and causes of death from the National Register of Causes of Death. Three health outcomes were studied: 1) primary and secondary hospital diagnoses of COPD morbidity (International Classification of Diseases [ICD]-8 491–492, and ICD-10 J41–J44); 2) COPD or CHD mortality (ICD-8 410–414, and ICD-10 I20–I25) as an underlying or contributing cause; and 3) all-cause mortality. COPD morbidity follow-up was until 12/31/2003. Mortality follow-up was until 12/31/2006 for cause-specific mortality, and until 8/11/2007 for all causes.
Statistical methods
The Cox proportional hazards model was used to estimate the hazard (instantaneous event rate) of morbidity or mortality in relation to lung function level and decline, respiratory symptoms, and asthma, while adjusting for essential covariates that would typically be available and taken into consideration in a clinical context (Citation41). Analyses were conducted by gender and for never-smokers. Additional Cox models using penalized splines were conducted for the overall cohort to identify the rate of FEV1 slope at which morbidity and mortality risk began to increase. For COPD morbidity, time to event (or censor) was calculated from examination 2 until COPD diagnosis, death, or end of follow-up. For mortality, time to event (or censor) was calculated from examination 2 until death or end of follow-up.
First, we evaluated the relationships of the level of lung function measurements by examination (1 or 2) with morbidity and mortality. Models included quartiles of lung function level (FEV1/height2) (Citation42), respiratory symptoms, asthma, and age. The highest lung function level (75th percentile and above) served as the reference category. As the results were generally similar, only examination 2 results are presented, because shortness of breath was often statistically significant (p < 0.05) and was unavailable at examination 1.
Second, we evaluated the relationship of the rate of lung function decline, and respiratory symptoms and asthma ascertained at examination 2 with morbidity and mortality, adjusted for baseline age, height-adjusted baseline lung function (FEV1/height2), and height. In separate analyses, we evaluated 4 measures of lung function decline: 1) the FEV1 slope (the difference in FEV1 between examinations 1 and 2, divided by the time between the examinations) in quartiles; 2) the FEV1 relative slope (slope divided by baseline FEV1) in quartiles; 3) the American College of Occupational and Environmental Medicine's (ACOEM) Longitudinal Normal Limit (LNL) decline based on the American Thoracic Society's (ATS) 15% annual limit and an expected decline of 30 ml/yr (Citation43); and 4) a FEV1 decline of 90 ml/yr or more (Citation44).
Table 1 Characteristics of the sub-cohorts for Cox proportional hazards model analysis using data for lung function level and decline
Table 2 Cox proportional hazards models for COPD morbidity (hospital diagnosis of COPD [ICD-8 491–492, ICD-10 J41–J44])
The ACOEM LNL is designed to identify excessive declines during early years of spirometry follow-up (Citation1–8 years) when data are sparse and a reliable slope cannot be estimated (Citation43). To identify trends in risk, we categorized the slopes into quartiles and used the quartiles with the lowest rates of decline (75th percentile and above) as the reference categories. The risks associated with excessive lung function decline, defined by the 2 longitudinal limits (LNL and a decline of 90 ml/yr or more), were compared to subjects with “normal” decline. The 2 limits were compared for predicting risk, and for model fit using the Akaike's information criterion (AIC) (Citation45).
Preliminary analysis of mean FEV1 values by year of examination revealed a slightly excessive increase in mean FEV1 in 1981 (n = 4,319), but not in 1982 (n = 5,353) or 1983 (n = 2,593), as compared to mean FEV1 for baseline examinations in 1976–78. It is unknown to the authors whether this increase in 1981 was due to early difficulties with the Monaghan spirometer that was replaced with a dry wedge spirometer by examination 3 or to other issues (Citation38). To adjust for this increase, we reduced the individual FEV1 measurements for 1981 by a fixed value of 289 ml for males and 201 ml for females to align the 1981 values with the 1976–78 and 1982–83 values. The fixed values represent the average difference in FEV1 values from examination 1 to 2 (for 1982 only), minus 30 ml to correct for annual loss from 1981 to 1982. Models excluding the 1981 values were conducted for a sensitivity analysis regarding this adjustment.
Copenhagen City Heart Study participants gave informed consent to participate and the study was performed in accordance with the 2nd Helsinki Declaration and approved by the Danish regional ethics committee. The present study was approved by the National Institute for Occupational Safety and Health Human Subjects Review Board and the West Virginia University Institutional Review Board. Analyses were conducted with PROC PHREG (p < 0.05) in SAS, version 9.2 (SAS Institute Inc, Cary, NC, USA). Penalized splines were prepared in R software (version 2.7.2).
RESULTS
Three sub-cohorts (males, females, and never-smokers) used in the Cox models are described in . The table shows the duration of follow-up for morbidity and mortality (Citation15–20 years), the mean and quartile values for FEV1/height2 (level of lung function) and the 2 types of slopes (lung function decline), and the frequency of symptoms and asthma. For never-smokers, we presented gender-specific quartiles because females represented 75% of the never-smoker sub-cohort. (Sample sizes for the analysis of lung function decline were smaller because members had to participate at both examinations 1 and 2.) Baseline characteristics for subjects who were lost to follow-up by examination 2 were a higher average age, lower average FEV1/height2, and a higher percentage of self-reported current asthma, symptoms of chronic bronchitis, and smoking.
COPD morbidity
Estimated HRs for COPD morbidity (hospital diagnosis of COPD) are presented in . The level of lung function (FEV1/height2) was a significant predictor of COPD morbidity for males and females starting from the second quartile. A similar, but less significant trend was observed in never-smokers, where significance was observed only at the fourth quartile.
Figure 1 Cox proportional hazards model results by gender (⧫ = males and • = females) for (a) COPD morbidity, (b) COPD or CHD mortality, and (c) all-cause mortality. Models adjusted for baseline age, height-adjusted baseline lung function (FEV1/height2), height, and respiratory symptoms and asthma as dichotomous variables. Relative slope is slope FEV1/baseline FEV1. LNL is the Longitudinal Normal Limit. See Table 1 for slope and relative slope quartile values.
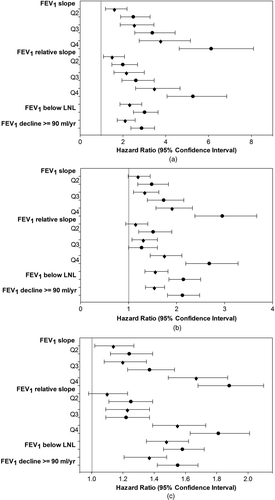
Figure 2 Cox proportional hazards model using a penalized spline for the FEV1 slope, males and females combined. The spline crossed above zero for the log HR at –54 ml/yr for (a) COPD morbidity, at –72 ml/yr for (b) COPD or CHD mortality, and at –77 ml/yr for (c) all-cause mortality. Models adjusted for baseline age, height-adjusted baseline lung function (FEV1/height2), and height. Tick marks on the x axis represent the frequency of the various FEV1 slope values.
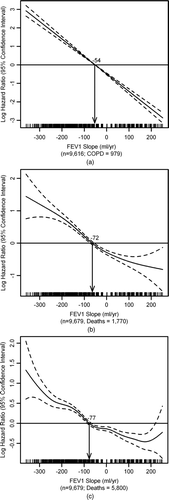
A similar increasing trend in COPD morbidity risk was seen for the FEV1 slope, except significance began at the third quartile for never-smokers. Females had the highest HRs for COPD morbidity for the slope, the relative slope, the LNL, and the 90 ml/yr limit (). Results from the penalized spline analysis showed that the log HR for COPD morbidity (males and females combined) started to increase at an FEV1 slope of –54 ml/yr (). The parameter for the linear rate was highly significant (p < 0.0001).
Comparing results for the dichotomous outcomes, the LNL was significantly associated with COPD morbidity for all 3 sub-cohorts, as was the 90 ml/yr limit. When compared using the AIC (Citation45), the fit of these 2 models were similar. The LNL limit provided a slightly better fit for females, but the 90 ml/yr limit was slightly better for males and never-smokers.
Respiratory symptoms and asthma were often significantly associated with COPD morbidity in the models with the level of lung function and lung function decline. The risks associated with chronic bronchitis and shortness of breath were higher than for asthma for males and females. In never-smokers, the risk associated with asthma was the highest with a 4-fold increase.
Mortality due to COPD or CHD, and all-cause mortality
For mortality outcomes, there was significant association with decreasing level of lung function for all 3 sub-cohorts (Tables and ). The risk of COPD or CHD mortality in relation to the level of lung function was slightly higher than for all-cause mortality, with females and never-smokers often having higher risks than males. For COPD or CHD mortality, females in the fourth quartile had more than a 5-fold increase in risk, never-smokers a nearly 4-fold increase in risk, and males a 3-fold increase in risk as compared to the reference. Hazard ratios for all-cause mortality were more similar for the 3 sub-cohorts.
Table 3 Cox proportional hazards models for COPD (ICD-8 491–492, ICD-10 J41–J44) or CHD mortality (ICD-8 410–414, and ICD-10 I20–I25)
Table 4 Cox proportional hazards models for all-cause mortality
Increased risk of mortality began at the second quartile of the FEV1 slope and relative slope for females, and most often at the third quartile for males and the fourth quartile for never-smokers. Figures 2b and 2c show the penalized splines for the slope for the mortality outcomes in males and females combined. The nonlinear terms were significant (p = 0.01 for COPD or CHD mortality and p = 0.0001 for all-causes) and the spline crossed above zero for the log HR at –72 ml/yr for COPD or CHD mortality and at –77 ml/yr for all-cause mortality.
As for the dichotomous criteria (the LNL and the 90 ml/yr limit), there were significant associations with both mortality outcomes, with the exception of the LNL and COPD or CHD mortality in never-smokers. The AIC values for these 2 criteria were similar by sub-cohort and outcome, but the models with the LNL fit best overall, with the exceptions of COPD or CHD mortality in males and in never-smokers. A comparison of all of the results by sub-cohort demonstrates that females had the highest HRs for both mortality outcomes for all 4 measures of lung function decline.
Asthma was not significantly associated with increased risk of mortality. Chronic bronchitis was significantly associated with mortality, but not for never-smokers; COPD or CHD mortality was approximately 30% more likely for males and 60% for females who reported chronic bronchitis. In the models with the level of lung function, shortness of breath was significantly associated with mortality for all 3 sub-cohorts; doubling the risk of COPD or CHD mortality for males and females, and increasing the risk of all-cause mortality by 50%. Similar results were observed in the models with lung function decline.
DISCUSSION
Unlike studies concerned with identifying morbidity and mortality risk factors, our interest was examining the relationship of respiratory status (lung function, respiratory symptoms, and asthma) with morbidity and mortality. This study relates to clinical practice and prevention as it focuses on the predictive, rather than causative, nature of these respiratory factors. The level of lung function was associated with a high risk for morbidity and mortality, supporting the need for prevention. For example, in at-risk occupational populations, monitoring lung function over time can be used to identify individuals at risk of lung function impairment and initiate appropriate intervention. Our results add to current knowledge on lung function decline as a predictor of morbidity and mortality in aging populations.
Evaluating the usefulness of the measures of lung function decline
The results demonstrate the predictive capacities of the 4 criteria for lung function decline (the FEV1 slope and relative slope, the ACOEM LNL based on the ATS 15% year-to-year limit, and a FEV1 decline of 90 ml/yr or more) for morbidity and mortality (Citation43,44). Associations were stronger with COPD morbidity, as might be expected, given its identification using a hospital diagnosis which would focus on greater disease severity (Figure 1a). Also as expected, associations with mortality due to COPD or CHD were slightly higher than with all-cause mortality (Figures 1b and 1c); possibly due to a more direct relationship of COPD and CHD mortality with lung function.
Females were at greater risk of morbidity and mortality than males across all measures of lung function decline. This may be partially explained by smaller lung capacity in females; loss of lung function could pose more risk (Citation46). Also, given that females in this study had lower tobacco consumption than males, this may suggest higher susceptibility in females. Other studies also find females more susceptible to the effects of smoking and with more rapid decline than males, and possibly with greater propensity for the development of COPD (Citation47,48). HRs for never-smokers showed an increasing trend for all outcomes, but less statistical significance (Tables –).
Among the 4 criteria, quartiles for the slope had higher HRs, but goodness of fit (AIC) was generally better for the relative slope. The slightly lower risk and better fit for the relative slope are reasonable given the adjustment for baseline lung function level. As for the 2 limits (the LNL and the 90 ml/yr limit), the HRs were similar, but often slightly higher for the LNL, and the goodness-of-fit was often better for the LNL.
Investigating critical rates of lung function decline
HRs for COPD morbidity suggest a critical rate at the third quartile of the slope with risks greater than 2.5 times those for the reference (Figure 1a), corresponding to declines starting at 60 ml/yr for males and 57 ml/yr for females (). For COPD or CHD mortality, the critical rate is more likely at the fourth quartile of the slope, with risks nearly doubled for males and tripled for females (Figure 1b). For all-cause mortality, fourth quartile risks were increased by 67% and 88% for males and females, respectively (Figure 1c), corresponding to declines starting at 118 ml/yr for males and 95 ml/yr for females ().
Our results generally agree with several community-based and occupational studies of lung function decline and respiratory morbidity and mortality (Citation11,Citation14,Citation16,Citation22,Citation36). Similarities include slightly higher increased risks in females of COPD hospitalization and all-cause mortality associated with rapid decline as compared to males (Citation11, Citation16) and increased risk of cardiac mortality with increasing decline in males (Citation14). Also similar to our results, underground coal miners had a 2-fold risk of cardiovascular and nonmalignant respiratory disease mortality with rapid decline (Citation22), and declines above 90 ml/yr were statistically significant for mortality as were declines of 60 ml/yr or more in never-smokers (Citation36).
Assessing the usefulness of respiratory symptoms and asthma relative to lung function
Risk associated with lung function decline was often greater than with respiratory symptoms and asthma, though their risks remained statistically significant after adjustment for lung function. Asthma predicted COPD morbidity in males and never-smokers. Chronic bronchitis and shortness of breath predicted morbidity and mortality in males and females, with higher HRs in females for chronic bronchitis, which could relate to increased susceptibility to the effects of respiratory hazards (Citation48).
Other studies have also reported increased risk of morbidity and mortality in relation to reported respiratory symptoms (chronic bronchitis, cough, phlegm, and shortness of breath) and asthma (Citation49–53), even after controlling for FEV1 (Citation54). In contrast, we did not find increased risk between asthma and respiratory mortality; however, our study targeted COPD specifically (Citation49). Chronic bronchitis often remained a significant predictor of mortality in our study, even with adjustment for shortness of breath and height-adjusted baseline lung function (Citation54).
There are several limitations including the necessary adjustment in the FEV1 values collected in 1981. Therefore, influences of the adjusted data were investigated by excluding it from the analysis, and significant overestimation of the HRs was not identified. Rather, the inclusion of the adjusted data resulted in an underestimation of the HRs. In particular, COPD morbidity HRs were underestimated for the level of lung function, the slope, and the relative slope, but mainly for never-smokers and more often for females than for males. There was also underestimation of the HRs for COPD or CHD mortality for the level of lung function for never-smokers.
There are other possible limitations related to lung function decline. One is the use of only 2 spirometry measurements for the rate of change in FEV1, leaving the slope vulnerable to instability in either measurement, but the quartile groupings could have reduced this effect. The limit of 90 ml/yr or more was from literature pertaining to males (Citation44). Although females generally have smaller lungs (Citation46), results using this criterion were similar to those for the LNL and the fourth quartile of the slope for females, a corresponding decline of 95 ml/yr or more. In studying lung function decline, we did not account for possible mixing between cause and effect with regard to steeply declining lung function and COPD outcomes, but our interest was in prediction rather than causation.
Underdiagnosis of COPD and underreporting of COPD as a cause of death could have resulted in misclassification, biasing the results toward the null, and reducing the associations (Citation55). This was addressed by combining the often interrelated COPD and CHD mortality into 1 outcome (Citation23, 24). As COPD morbidity was limited to a hospital diagnosis, our results generally represent risk for individuals with more severe disease, but also likely the highest costs. Other possible selection bias includes subjects lost to follow-up by examination 2, who were older at baseline, had a lower lung function level, and a higher proportion of self-reported current asthma, chronic bronchitis, and smoking as compared those who participated at examinations 1 and 2. This could have resulted in an underestimation of risk.
Our findings are relevant to clinical and workplace disease prevention and cost-reduction (Citation34,35). Investment in health promotion and protection for COPD could create savings for employers (Citation56, 57). The strong associations of COPD morbidity with lung function level and decline correspond with high medical costs for COPD and indicate that effective monitoring and interpretation of lung function decline for early disease detection could lead to cost-savings.
In conclusion, the risk of COPD morbidity, COPD or CHD mortality, and all-cause mortality showed an increasing trend with lung function decline after adjustment for baseline lung function, respiratory symptoms, and asthma. The level and rate of lung function decline generally demonstrated a higher risk of morbidity and mortality than the respiratory symptoms and asthma. These results provide further evidence that evaluation of lung function decline, in addition to the lung function level, is important in spirometry monitoring programs.
DECLARATION OF INTEREST
The authors report no conflicts of interest. The authors alone are responsible for the content and writing of the paper.
ACKNOWLEDGMENTS
Funding was received from the National Institute for Occupational Safety and Health. The authors would like recognize Drs. Christopher Martin, Edward Petsonk, Anoop Shankar, and Ms. Patricia Schleiff for their helpful comments.
REFERENCES
- Krzyzanowski M, Jedrychowski W, Wysocki M. Factors associated with the change in ventilatory function and the development of chronic obstructive pulmonary disease in a 13-year follow-up of the Cracow Study. Risk of chronic obstructive pulmonary disease. Am Rev Respir Dis 1986; 134:1011–1019.
- Bang KM, Gergen PJ, Kramer R, Cohen B. The effect of pulmonary impairment on all-cause mortality in a national cohort. Chest 1993; 103:536–540.
- Beaty TH, Cohen BH, Newill CA, Menkes HA, Diamond EL, Chen CJ. Impaired pulmonary function as a risk factor for mortality. Am J Epidemiol 1982; 116:102–113.
- Beaty TH, Newill CA, Cohen BH, Tockman MS, Bryant SH, Spurgeon HA. Effects of pulmonary function on mortality. J Chronic Dis 1985; 38:703–710.
- Hole DJ, Watt GC, Davey-Smith G, Hart CL, Gillis CR, Hawthorne VM. Impaired lung function and mortality risk in men and women: Findings from the Renfrew and Paisley prospective population study. BMJ 1996; 313:711–716.
- Krzyzanowski M, Wysocki M. The relation of thirteen-year mortality to ventilatory impairment and other respiratory symptoms: The Cracow Study. Int J Epidemiol 1986; 15:56–64.
- Mannino DM, Buist AS, Petty TL, Enright PL, Redd SC. Lung function and mortality in the United States: Data from the First National Health and Nutrition Examination Survey follow up study. Thorax 2003; 58:388–393.
- Neas LM, Schwartz J. Pulmonary function levels as predictors of mortality in a national sample of US adults. Am J Epidemiol 1998; 147:1011–1018.
- Pelkonen M, Notkola IL, Tukiainen H, Tervahauta M, Tuomilehto J, Nissinen A. Smoking cessation, decline in pulmonary function and total mortality: A 30 year follow up study among the Finnish cohorts of the Seven Countries Study. Thorax 2001; 56:703–707.
- Rodriguez BL, Masaki K, Burchfiel C, Curb JD, Fong KO, Chyou PH, Marcus EB. Pulmonary function decline and 17-year total mortality: The Honolulu Heart Program. Am J Epidemiol 1994; 140:398–408.
- Ryan G, Knuiman MW, Divitini ML, James A, Musk AW, Bartholomew HC. Decline in lung function and mortality: The Busselton Health Study. J Epidemiol Community Health 1999; 53:230–234.
- Schunemann HJ, Dorn J, Grant BJ, Winkelstein W, Jr., Trevisan M. Pulmonary function is a long-term predictor of mortality in the general population: 29-year follow-up of the Buffalo Health Study. Chest 2000; 118:656–664.
- Persson C, Bengtsson C, Lapidus L, Rybo E, Thiringer G, Wedel H. Peak expiratory flow and risk of cardiovascular disease and death. A 12-year follow-up of participants in the population study of women in Gothenburg, Sweden. Am J Epidemiol 1986; 124:942–948.
- Tockman MS, Pearson JD, Fleg JL, Metter EJ, Kao SY, Rampal KG, Cruise LJ, Fozard JL. Rapid decline in FEV1. A new risk factor for coronary heart disease mortality. Am J Respir Crit Care Med 1995; 151:390–398.
- Mannino DM, Davis KJ. Lung function decline and outcomes in an elderly population. Thorax 2006; 61:472–477.
- Mannino DM, Reichert MM, Davis KJ. Lung function decline and outcomes in an adult population. Am J Respir Crit Care Med 2006; 173:985–990.
- Mannino DM, Homa DM, Akinbami LJ, Ford ES, Redd SC. Chronic obstructive pulmonary disease surveillance–United States, 1971–2000. MMWR Surveill Summ 2002; 51:1–16.
- Leigh JP, Romano PS, Schenker MB, Kreiss K. Costs of occupational COPD and asthma. Chest 2002; 121:264–272.
- Sin DD, Stafinski T, Ng YC, Bell NR, Jacobs P. The impact of chronic obstructive pulmonary disease on work loss in the United States. Am J Respir Crit Care Med 2002; 165:704–707.
- Akazawa M, Halpern R, Riedel AA, Stanford RH, Dalal A, Blanchette CM. Economic burden prior to COPD diagnosis: A matched case-control study in the United States. Respir Med 2008; 102:1744–1752.
- World Health Organization. COPD Burden. http://www.who.int/ respiratory/copd/burden/en/index.html (accessed August 2010).
- Beeckman LA, Wang ML, Petsonk EL, Wagner GR. Rapid declines in FEV1 and subsequent respiratory symptoms, illnesses, and mortality in coal miners in the United States. Am J Respir Crit Care Med 2001; 163:633–639.
- Schneider C, Bothner U, Jick SS, Meier CR. Chronic obstructive pulmonary disease and the risk of cardiovascular diseases. Eur J Epidemiol 2010; 27:27.
- Sin DD. Is COPD Really a Cardiovascular Disease? Chest 2009; 136:2.
- Balmes J, Becklake M, Blanc P, Henneberger P, Kreiss K, Mapp C, Milton D, Schwartz D, Toren K, Viegi G. American Thoracic Society Statement: Occupational contribution to the burden of airway disease. Am J Respir Crit Care Med 2003; 167:787–797.
- Hnizdo E, Sullivan PA, Bang KM, Wagner G. Association between chronic obstructive pulmonary disease and employment by industry and occupation in the US population: A study of data from the Third National Health and Nutrition Examination Survey. Am J Epidemiol 2002; 156:738–746.
- Trupin L, Earnest G, San Pedro M, Balmes JR, Eisner MD, Yelin E, Katz PP, Blanc PD. The occupational burden of chronic obstructive pulmonary disease. Eur Respir J 2003; 22:462–469.
- Harber P, Tashkin DP, Simmons M, Crawford L, Hnizdo E, Connett J. Effect of occupational exposures on decline of lung function in early chronic obstructive pulmonary disease. Am J Respir Crit Care Med 2007; 176:994–1000.
- Blanc PD, Eisner MD, Earnest G, Trupin L, Balmes JR, Yelin EH, Gregorich SE, Katz PP. Further exploration of the links between occupational exposure and chronic obstructive pulmonary disease. J Occup Environ Med 2009; 51:804–810.
- Blanc PD, Iribarren C, Trupin L, Earnest G, Katz PP, Balmes J, Sidney S, Eisner MD. Occupational exposures and the risk of COPD: Dusty trades revisited. Thorax 2009; 64:6–12.
- Boggia B, Farinaro E, Grieco L, Lucariello A, Carbone U. Burden of smoking and occupational exposure on etiology of chronic obstructive pulmonary disease in workers of Southern Italy. J Occup Environ Med 2008; 50:366–370.
- Rodriguez E, Ferrer J, Marti S, Zock JP, Plana E, Morell F. Impact of occupational exposure on severity of COPD. Chest 2008; 134:1237–1243.
- Weinmann S, Vollmer WM, Breen V, Heumann M, Hnizdo E, Villnave J, Doney B, Graziani M, McBurnie MA, Buist AS. COPD and Occupational Exposures: A Case-Control Study. J Occup Environ Med 2008; 50:561–569.
- Darkow T, Chastek BJ, Shah H, Phillips AL. Health care costs among individuals with chronic obstructive pulmonary disease within several large, multi-state employers. J Occup Environ Med 2008; 50:1130–1138.
- Darkow T, Kadlubek PJ, Shah H, Phillips AL, Marton JP. A retrospective analysis of disability and its related costs among employees with chronic obstructive pulmonary disease. J Occup Environ Med 2007; 49:22–30.
- Sircar K, Hnizdo E, Petsonk E, Attfield M. Decline in lung function and mortality: Implications for medical monitoring. Occup Environ Med 2007; 64:461–466.
- Schnohr P, Jensen G, Lange P, Scharling H, Appleyard M. The Copenhagen City Heart Study. Eur Heart J 2001; 3:H1–83.
- Lokke A, Lange P, Scharling H, Fabricius P, Vestbo J. Developing COPD: A 25 year follow up study of the general population. Thorax 2006; 61:935–939.
- Burrows B, Lebowitz MD, Camilli AE, Knudson RJ. Longitudinal changes in forced expiratory volume in one second in adults. Methodologic considerations and findings in healthy nonsmokers. Am Rev Respir Dis 1986; 133:974–980.
- Rose GA, Blackburn H. Cardiovascular survey methods. Monogr Ser World Health Organ 1968; 56:1–188.
- Miller MR, Pedersen OF. New concepts for expressing forced expiratory volume in 1 s arising from survival analysis. Eur Respir J 2010; 35:873–882.
- Miller MR, Pedersen OF, Lange P, Vestbo J. Improved survival prediction from lung function data in a large population sample. Respir Med 2009; 103:442–448.
- Townsend MC. Evaluating pulmonary function change over time in the occupational setting. J Occup Environ Med 2005; 47:1307–1316.
- Buist AS, Vollmer WM. The use of lung function tests in identifying factors that affect lung growth and aging. Stat Med 1988; 7:11–18.
- Akaike H. A new look at the statistical model identification. IEEE Transactions on Automatic Control 1974; 19:716–723.
- Becklake MR, Kauffmann F. Gender differences in airway behaviour over the human life span. Thorax 1999; 54:1119–1138.
- Prescott E, Bjerg AM, Andersen PK, Lange P, Vestbo J. Gender difference in smoking effects on lung function and risk of hospitalization for COPD: results from a Danish longitudinal population study. Eur Respir J 1997; 10:822–827.
- Camp PG, O'Donnell DE, Postma DS. Chronic obstructive pulmonary disease in men and women: Myths and reality. Proc Am Thorac Soc 2009; 6:535–538.
- Knuiman MW, James AL, Divitini ML, Ryan G, Bartholomew HC, Musk AW. Lung function, respiratory symptoms, and mortality: Results from the Busselton Health Study. Ann Epidemiol 1999; 9:297–306.
- Vestbo J, Knudsen KM, Rasmussen FV. The value of mucus hypersecretion as a predictor of mortality and hospitalization. An 11-year register based follow-up study of a random population sample of 876 men. Respir Med 1989; 83:207–211.
- Vestbo J, Rasmussen FV. Respiratory symptoms and FEV1 as predictors of hospitalization and medication in the following 12 years due to respiratory disease. Eur Respir J 1989; 2:710–715.
- Lange P, Nyboe J, Appleyard M, Jensen G, Schnohr P. Relation of ventilatory impairment and of chronic mucus hypersecretion to mortality from obstructive lung disease and from all causes. Thorax 1990; 45:579–585.
- Carpenter L, Beral V, Strachan D, Ebi-Kryston KL, Inskip H. Respiratory symptoms as predictors of 27 year mortality in a representative sample of British adults. BMJ 1989; 299:357–361.
- Ebi-Kryston KL. Respiratory symptoms and pulmonary function as predictors of 10-year mortality from respiratory disease, cardiovascular disease, and all causes in the Whitehall Study. J Clin Epidemiol 1988; 41:251–260.
- Jensen HH, Godtfredsen NS, Lange P, Vestbo J. Potential misclassification of causes of death from COPD. Eur Respir J 2006; 28:781–785.
- Loeppke R, Taitel M, Haufle V, Parry T, Kessler RC, Jinnett K. Health and productivity as a business strategy: A multiemployer study. J Occup Environ Med 2009; 51:411–428.
- Loeppke R, Taitel M, Richling D, Parry T, Kessler RC, Hymel P, Konicki D. Health and productivity as a business strategy. J Occup Environ Med 2007; 49:712–721.