Abstract
Background: Sleep quality is poor in severe emphysema. We hypothesized that in addition to nocturnal oxygen desaturation, the severity of airflow obstruction and degree of thoracic hyperinflation are responsible. Methods: Twenty-five patients (14 males, 64 ± 6 [ ± SD] yrs, BMI 24.7 ± 4.2 kg/m2) with severe emphysema (FEV1 = 28 ± 8% predicted, TLC = 125 ± 14% predicted) were studied. Measurements included spirometry, lung volumes, arterial blood gas, length of the diaphragm's zone of apposition (LZAP) and a polysomnogram. Results: Total sleep time (TST) was 227 ± 93 minutes with a sleep efficiency (SE) of 56 ± 21%. The mean SaO2, lowest SaO2, and% TST with a SaO2 < 90% were 90 ± 5%, 83 ± 8% and 29 ± 40%, respectively. TST correlated with FEV1% (r = 0.5, p = 0.02), FVC% (r = 0.4, p = 0.03) and LZAP (r = 0.5, p = 0.01). SE correlated with FEV1% (r = 0.5, p = 0.02) and LZAP (r = 0.5, p = 0.01), but not with FVC% (r = 0.4, p = 0.07). Additionally, TST and SE correlated negatively with residual volume% (r = -0.4, p = 0.046, and r = -0.4, p = 0.03, respectively). There was no correlation between TST and SE and measures of nocturnal oxygenation. Multiple linear regression was used to predict TST, with 50% (r2 = 0.49) explained by a combination of LZAP (27%), mean SaO2 (23%), and the lowest SaO2 (< 1%). To predict SE, 44% (r2 = 0.43) was explained by a combination of LZAP (29%), mean SaO2 (14%), and the lowest SaO2 (1%). Conclusion: Although parameters of respiratory function and mechanics correlate with sleep quality, both nocturnal oxygenation and measurements of respiratory function/mechanics predict sleep quality in severe emphysema.
INTRODUCTION
Patients with severe emphysema are known to have poor sleep quality (Citation1–6). Patients often report difficulty initiating and maintaining sleep (Citation1–6), as well as excessive daytime sleepiness (Citation2, Citation4, Citation6). Objectively, patients have increases in sleep latency and the number of arousals, and an increased disproportional amount of time in light sleep coupled with a decrease in total sleep time (TST) (Citation1–3, Citation7). Yet, despite these findings, the physiological determinants that are responsible for poor sleep quality in severe emphysema have not been identified.
Prior studies have noted that patients with severe emphysema often develop significant nocturnal oxygen desaturation (NOD), particularly during rapid eye movement (REM) sleep (Citation1,2, Citation7–10). Episodes of NOD can occur, despite the presence of an awake PaO2 > 60 mmHg (Citation1,2, Citation7–10). As a result, a number of studies have attempted to identify awake physiological parameters that correlate with NOD (Citation9–16). However, it is not known whether NOD is responsible for the poor sleep quality seen in these patients.
We previously noted an improvement in sleep quality in patients with emphysema with the use of non-invasive positive pressure ventilation, despite a lack of change in nocturnal gas exchange (Citation17). As a result, we hypothesized that other physiological factors in addition to NOD, such as the severity of airflow obstruction and hyperinflation, as well as abnormalities in respiratory mechanics, would be responsible for the poor sleep quality observed in severe emphysema.
METHODS
Patient selection
Twenty-seven consecutive patients undergoing evaluation for lung volume reduction surgery (LVRS) as part of the National Emphysema Treatment Trial (NETT) were enrolled into this single center ancillary study. The data includes patients that were previously reported on as part of another study (Citation18). Criteria for patient enrollment in the NETT have been previously published (Citation19). The protocol for this NETT ancillary sleep study was explained in detail to the patients (). Patients were excluded from the study if 1) they withdrew from the NETT trial, 2) refused to have an overnight polysomnogram study on room air, or 3) had a known history of obstructive sleep apnea. The NETT and this ancillary study were approved by the Institutional Review Board for Human Research (Temple University School of Medicine, Philadelphia, Pa.) and the NETT Ancillary Studies Committee.
Pulmonary function tests and arterial blood gases
Pulmonary function tests were performed after completion of the outpatient pulmonary rehabilitation sessions. A System 6200 Autobox DL-plethysmograph (Sensormedics Corp., Yorba Linda, CA) was utilized to perform the pulmonary function tests. Measurements included FVC, FEV1, and FEV1/FVC. Body plethysmography was used to measure thoracic gas volumes. The data are reported as post-bronchodilator in absolute numbers and as a percentage of the normal predicted values (Citation20,21). After completion of the pulmonary function tests, a resting room air arterial blood gas was drawn from the radial artery.
Length of the Zone of Apposition of the Diaphragm (LZAP)
Length of the zone of apposition of the diaphragm (LZAP) was measured in real-time using a B mode ultrasound (HDI 5000, Phillips Medical Systems, Bothell, WA). Measurements were made while seated by placing the probe at the mid-axillary line between the 8th and 9th intercostal space, as previously described (Citation22,23). As total lung capacity (TLC) is reached during inspiration, LZAP approaches zero and allows for the identification of the costal origin of the diaphragm. LZAP was determined at functional residual capacity (FRC) as the length of the diaphragm from its costal insertion to the point where the diaphragm peels away from the chest wall at the lung's leading edge (Citation22,23).
Pulmonary rehabilitation
Prior to enrollment, all patients had completed a 6–10-week outpatient pulmonary rehabilitation program consisting of a minimum of 16 educational and 16 exercise sessions. An exercise physiologist supervised each patient throughout the program. Exercises included lifting arm and leg weights, arm cycling, and the use of a motor-driven treadmill. An individualized program was derived based on symptom-limited maximums obtained at baseline. An increase in the intensity of the program was based on individual patient performance.
Sleep studies
All polysomnograms were performed while breathing room air. Recordings included measurements of rib cage and abdominal motion (Respitrace; Ambulatory Monitoring Inc., White Plains, NY), and air flow measured with a pressure transducer. Other variables recorded included electrocardiogram, electro-oculogram, digastric electromyogram, electroencephalogram and finger pulse oximetry (model N-100; Nellcor Puritan Bennett; Pleasanton, Ca). All variables were continuously recorded and stored on a computerized system (Alice Citation3; Healthdyne Information Enterprises; Marietta, Ga.). Sleep was staged and arousals defined using established criteria (Citation24). An arousal was defined as an abrupt shift of EEG frequency including alpha, theta and/or frequencies > 16 Hz (but not spindles) that lasts at least 3 seconds, with at least 10 seconds of stable sleep preceding the change (Citation24). Obstructive apneas were defined by the lack of airflow for greater than 10 seconds that were associated with the presence of rib cage and abdominal movement (Citation24).
Obstructive hypopneas were defined by the presence of any of the following: a 30% decrease in airflow for greater than 10 seconds that was associated with the presence of rib cage and abdominal movement and oxygen desaturation of at least 4%; or a 50% decrease in airflow associated with a ≥ 3% decrease in oxygen saturation or an arousal (Citation24). Apneas were defined as central if there was a lack of respiratory effort during the period of absent airflow (Citation24). The apnea-hypopnea index was calculated as the number of apneic and hypopneic events per hour of sleep. Other calculated variables included: TST, sleep efficiency (SE) (TST divided by time in bed), arousal index, and the percent of TST with an arterial oxygen saturation (SaO2) <90%. The acquaintance night studies were performed in a similar manner as the study night polysomnograms but the data was not scored. The data for the study night polysomnograms were initially scored by a single senior technologist. To ensure consistency of polysomnogram interpretations, the same author (SK) reviewed each study.
Data analysis
Data are displayed as the mean ± SD. The relationships between the measurements of sleep quality (TST and SE) and physiological variables were assessed by Pearson correlation coefficients. P < 0.05 was considered significant. Multiple linear regression was used to evaluate the contribution of the various parameters in the prediction of each component of sleep quality. The significance level for retention in a model was 0.05. All statistical analyses were carried out using SAS V9.1.3 software (SAS Institute, Cary, NC).
RESULTS
Patient characteristics
Twenty-seven consecutive patients were evaluated, with 25 patients (14 males, 64 ± 6 yrs, BMI 24.7 ± 4.2 kg/m2) completing the study. Two patients refused to sleep without their oxygen and were excluded from the study. All 25 patients had evidence of severe airflow limitation (FEV1 = 28 ± 8% predicted) and hyperinflation (TLC = 125 ± 14 % predicted). The mean room air PaO2 was 63 ± 8 mm Hg and PaCO2 was 43 ± 5 mm Hg. Thirteen of the 25 patients were on home oxygen therapy (oxygen flow rate by nasal cannula – 2 ± 0.6 L/min). The group that completed the study and those that were excluded had similar severity of airflow obstruction and oxygenation (FEV1 L [%predicted] = 0.8 ± 0.3 L [28 ± 8%] and 0.7 ± 0.3 L [32 ± 9%] [p = 0.6], and PaO2 63 ± 8 mmHg and 57 ± 23 mmHg [p = 0.4], respectively). describes the baseline characteristics of the 25 patients that completed the study.
Table 1. Baseline Characteristics of Patients (N = 25)Footnote*
Sleep quality and nocturnal oxygenation
Sleep quality was poor during the night; with a decreased total sleep time of 227 ± 93 minutes, and a SE of 56 ± 21%. The spontaneous arousal index was 11 ± 5 arousals/hour (). Sleep architecture demonstrated an increase in N1 and N2 sleep and decrease in the percentage of N3 and REM sleep (). Twenty-three of the 25 patients had an episode of REM sleep during the night. There was no evidence of obstructive or central sleep apnea with an apnea-hypopnea index of 1 ± 2 events/hour. In regards to nocturnal oxygenation, the mean SaO2 while on room air during the night was 90 ± 5%, and the lowest SaO2 was 83 ± 8%. The % TST with SaO2 < 90% was 29 ± 40%, and the % REM with SaO2 < 90% was 31 ± 40%.
Correlates of sleep quality
TST correlated with FEV1% predicted (r = 0.5, p = 0.02) and FVC% predicted (r = 0.4, p = 0.03), and demonstrated a negative correlation with RV% predicted (r = -0.4, p = 0.046) (). LZAP was measured in 24 of the patients and correlated with TST (r = 0.5, p = 0.01) (). No measurements of daytime or nocturnal oxygenation correlated with TST ().
Figure 2. There was a positive correlation between both total sleep time and sleep efficiency and the length of the zone of apposition of the diaphragm (LZAP) (r = 0.5, p = 0.01 and r = 0.5, p = 0.01, respectively).
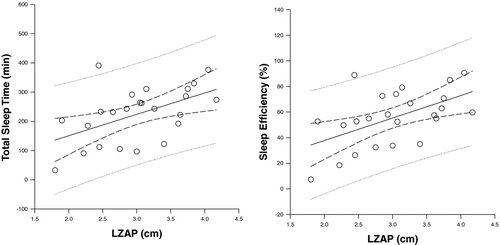
Table 2. Pearson correlation coefficients between physiological variables and measurements of sleep quality
SE correlated with FEV1% predicted (r = 0.5, p = 0.02) but not with FVC% predicted (r = 0.4, p = 0.07). Similar to TST, there was negative correlation between SE and RV% predicted, (r = -0.4, p = 0.03) () and a positive correlation with LZAP (r = 0.5, p = 0.01) (). Again, no measurements of daytime or nocturnal oxygenation correlated with SE (). Multiple linear regression was utilized to determine which parameters predicted TST and SE ().
Table 3. Multiple linear regression analyses of Total Sleep Time (TST) and Sleep Efficiency (SE)
The respiratory mechanics and function variables that were utilized are listed in . When variables were collinear, the one with the highest r value was used in the analysis. In the analysis to predict TST, 50% (r2 = 0.49) was explained by a combination of LZAP (27%), mean SaO2 during the night (23%), and the lowest SaO2 during the night (< 1%). Similar results were seen in the analysis to predict SE, with 44% (r2 = 0.43) explained by a combination of LZAP (29%), mean SaO2 during the night (14%), and the lowest SaO2 during the night (1%).
DISCUSSION
Although the physiological parameters associated with the development of NOD in emphysema have been identified (Citation2, Citation9–12), the physiological determinants of poor sleep quality are unknown. There are 3 major findings regarding sleep in severe emphysema patients from this study: 1) sleep quality is poor; 2) NOD is common and; 3) while parameters of respiratory function and mechanics correlate with sleep quality, both nocturnal oxygenation and measurements of respiratory mechanics and function are important predictors of sleep quality.
Previous studies have reported poor sleep quality in severe emphysema (Citation1–3, Citation7). Subjective complaints of difficulty initiating and maintaining sleep, and associated daytime sleepiness have been noted to be more common in those patients with respiratory symptoms, including cough, dyspnea, and wheezing (Citation2, Citation4–6). However, objective assessment of sleep quality by polysomnogram in patients with emphysema has been performed in only small cohorts (Citation1–3, Citation7). Cormick et al. (Citation2) noted a TST of only 208 minutes and an increased arousal index in a group of 16 emphysema patients. Wynne et al. (Citation1) studied 7 severe emphysema patients and noted a decreased TST (218 minutes), with 30% of the night spent7break; awake.
In 20 severe emphysema patients, Calverley et al. (Citation3) noted an increased sleep latency, a decreased amount of uninterrupted sleep, and decreases in slow wave sleep (SWS) and REM sleep, when compared to healthy controls. Similar findings were noted by Fleetham et al (Citation7) in 24 severe emphysema patients, with a decreased TST, increased number of oxygen desaturation related arousals, and decreased SWS and REM sleep. Our findings were similar to these previous studies, with a TST of only 227 ± 93 minutes, SE of 56 ± 21%, and small percentages of N3 and REM sleep (). In addition, our findings are in marked contrast to age matched notrmal controls and those with less severe forms of emphysema, suggesting an association between poor sleep quality and severe emphysema (Citation25, 26).
Despite the recognition of poor sleep quality in emphysema, physiological determinants associated with its development have not been well characterized. Cormick et al. (Citation2) noted that the arousal frequency appeared to correlate with the severity of nocturnal hypoxemia, suggesting that sleep quality was worse in those more hypoxemic. Fleetham et al. (Citation7) also noted arousals to be associated with periods of NOD. However, the arousal frequency did not decrease when the hypoxemia was corrected with supplemental oxygen. In contrast, Calverley et al. (Citation3) noted that with supplemental oxygen, sleep latency decreased and TST increased, as did the percentages of REM sleep and uninterrupted sleep. In the present study we found that both the mean SaO2 and lowest SaO2 predicted poor sleep quality as measured by TST and SE.
However, in our previous study examining the effects of LVRS on sleep quality and nocturnal gas exchange in emphysema, the improvement in TST and SE following LVRS did not correlate with the observed improvement in nocturnal oxygenation (Citation18). In addition, we previously noted improved sleep quality without an associated improvement in nocturnal gas exchange with the use of noninvasive positive pressure ventilation in a group of stable patients with hypercapnic COPD (Citation17). Finally, in normal subjects, hypoxemia by itself is a poor arousal stimulus (Citation27). Therefore, other factors in addition to hypoxemia appear to be responsible for the poor sleep quality observed in these patients.
In the present study, we found that physiologic parameters associated with airflow obstruction (FEV1 and FVC) and air trapping (RV) correlated with sleep quality as expressed by TST and SE. In addition, LZAP, which is decreased in emphysema as a result of air trapping and hyperinflation (Citation22), was found to be correlated (). However, as noted in the multiple linear regression (), nocturnal oxygenation was also important in predicting sleep quality in severe emphysema.
The mechanisms by which abnormalities in respiratory mechanics affect sleep quality are unknown. Diaphragmatic function is progressively impaired at increasing lung volumes, as found in emphysema (Citation28–30). However, there is no further increase in lung volume nor further impairment in diaphragmatic function during sleep that has been described in emphysema patients (Citation31,32). The decrease in minute ventilation during sleep compared to wakefulness, primarily from a decrease in tidal volume, appears to be due to a decrease in ventilatory drive and possibly an increase in upper airway resistance (Citation31–33). Ballard et al. (Citation31) noted no change in lung volumes or lower airway resistance during sleep, but observed an increase in upper airway resistance and a decrease in P0.1 in normocapnic COPD patients. As a result, there was an observed decrease in tidal volume and thus minute ventilation during sleep in these patients.
O'Donoghue et al. (Citation32) noted a similar preservation of lung volume during sleep, with no increase in intrinsic PEEP or end-expiratory lung volume, and a preservation of diaphragmatic function in hypercapnic COPD patients. A similar decrease in tidal volume was noted that was associated with decreased ventilatory drive and increased upper airway resistance. However, when upper airway resistance was decreased with the administration of heliox, ventilatory drive decreased further in order to maintain what appeared to be a preset level of minute ventilation during sleep (Citation32). It has been suggested that this may be a protective mechanism to prevent the development of diaphragmatic muscle fatigue in these patients (Citation32). These findings are in contrast to an increase in central drive and arousal frequency seen in normal subjects subjected to an added inspiratory resistance (Citation34,35).
One proposed mechanism is that a smaller LZAP results in an inward movement of the lower rib cage, with the other muscles of inspiration having to compensate by increasing their activity, leading to a neural mediated arousal from sleep. Although previous studies have suggested that ventilatory drive is decreased during sleep in patients with emphysema, none of these studies have specifically examined the ventilatory drive and severity of hypoxemia prior to an arousal from sleep. Whether more severe abnormalities in respiratory mechanics and function results in more significant nocturnal hypoxemia and effort induced arousals, and whether the arousal threshold is different in patients with severe emphysema, are presently unknown.
Other factors can contribute to poor sleep quality in patients with emphysema. The presence of respiratory symptoms, including dyspnea, cough, and sputum production, has been shown to be associated with poor sleep quality (Citation2, Citation4–6), and may explain the prolonged awakenings rather than high arousal index seen in these patients (Citation2, Citation7). In addition, medications used in the treatment of emphysema can also affect sleep quality. However, the effects of these medications on sleep quality have varied, with some studies reporting adverse effects (Citation36), while others noted no change (Citation37–40) or an improvement in sleep quality (Citation41). In a study using tiotropium for 4 weeks, McNicholas et al. (Citation40) demonstrated an improvement in FEV1 and nocturnal SaO2 without an improvement in sleep quality.
However, it should be noted that the improvement in FEV1, while statistically significant, was quite small, at less than 200 cc. In addition, despite, an improvement in nocturnal oxygen saturation with tiotropium, there was no significant improvement in sleep quality, suggesting other factors must also contribute to the poor sleep quality seen in these patients. Our patients were all on similar medication regimens, with no significant difference based on the severity of their obstructive disease.
There are limitations with our study that need to be addressed. First, the number of patients in the study was small. However, the study size was similar to or larger than most of the previous studies that have quantitatively evaluated sleep quality in patients with emphysema (Citation1–3, Citation7). Second, the patients that we studied had severe emphysema (FEV1 = 28 ± 8% predicted, TLC = 125 ± 14% predicted) and were being evaluated for possible LVRS. However, the severity of disease was similar to previous studies, and our patients had received maximal medical therapy, including medications and 6–10 weeks of pulmonary rehabilitation, prior to the start of the study (Citation1–3, Citation7).
Whether similar findings would be seen in patients with less severe disease is presently unknown. Third, we did not measure periodic limb movements during the night. Restless legs syndrome has been shown to be more prevalent in patients with COPD and be associated with disturbed sleep (Citation42–44). However, none of these prior studies actually performed overnight polysomnograms to evaluate whether periodic limb movements contributed to these findings. Finally, the patients were studied in the sleep lab, which is a controlled environment and not as comfortable as the patient's own bedroom. As a result, sleep quality may be less than what would be observed at home. However, all patients underwent an acquaintance night study to account for a “first night” effect of sleeping in a new environment. In addition, the sleep quality in our patients was equivalent to previous studies in similar groups of patients (Citation1–3, Citation7).
In conclusion, sleep quality is poor and NOD is common in patients with severe emphysema. While parameters of respiratory function and mechanics correlate with sleep quality, both nocturnal oxygenation and measurements of respiratory mechanics and function predict sleep quality in these patients. Identifying the mechanism(s) responsible for this association awaits further study.
ACKNOWLEDGMENTS
Special thanks to Anne Marie Kuzma, RN and Gwendolyn Vance, RN for their help on this project. Sources of Support: National Emphysema Treatment Trial Research Group, funding in part by: NHLBI contract no. 1-76116, Centers for Medicare and Medicaid Services, and the Agency for Healthcare Research and Quality.
Declaration of interest
The authors report no conflicts of interest. The authors alone are responsible for the content and writing of the paper. No financial or other potential conflicts of interest exist for the National Emphysema Treatment Trial (NETT) Research Group
REFERENCES
- Wynne JW, Block AJ, Hemenway J, Hunt LA, Flick MR. Disordered breathing and oxygen desaturation during sleep in patients with chronic obstructive lung disease. Am J Med 1979; 66:573–579.
- Cormick W, Olson LG, Hensley MJ, Saunders NA. Nocturnal hypoxaemia and quality of sleep in patients with chronic obstructive lung disease. Thorax 1986; 41:846–854.
- Calverley PMA, Brezinova V, Douglas NJ, Catterall JR, Flenley DC. The effect of oxygenation on sleep quality in chronic bronchitis and emphysema. Am Rev Respir Dis 1982; 126:206–210.
- Klink ME, Dodge R, Quan SF. The relation of sleep complaints to respiratory symptoms in the general population. Chest 1994; 105:151–154.
- Dodge R, Cline MG, Quan SF. The natural history of insomnia and its relationship to respiratory symptoms. Arch Intern Med 1995; 155:1797–1800.
- Bellia V, Catalano F, Scichilone N, Incalzi RA, Spatafora M, Vergani C, Sleep disorders in the elderly with and without chronic airflow obstruction: the SARA study. Sleep 2003; 26:318–323.
- Fleetham J, West P, Mezon B, Conway W, Roth T, Kryger M. Sleep, arousals, and oxygen desaturation in chronic obstructive pulmonary disease: The effect of oxygen therapy. Am Rev Respir Dis 1982; 126;429–433.
- Koo KW, Sax DS, Snider GL. Arterial blood gases and pH during sleep in chronic obstructive pulmonary disease. Am J Med 1975; 58:663–670.
- Fletcher EC, Miller J, Divine GW, Fletcher JG, Miller T. Nocturnal oxyhemoglobin desaturation in COPD patients with arterial oxygen tensions above 60 mmHg. Chest 1987; 92:604–8.
- Heijdra YF, Dekhuijzen PNR, van Herwaarden CLA, Folgering HTM. Nocturnal saturation and respiratory muscle function in patients with chronic obstructive pulmonary disease. Thorax 1995; 50:610–612.
- Bradley TD, Mateika J, Li D, Avendano M, Goldstein RS. Daytime hypercapnia in the development of nocturnal hypoxemia in COPD. Chest 1990; 97:308–312.
- Connaughton JJ, Catterrall JR, Elton RA, Stradling JR, Douglas NJ. Do sleep studies contribute to the management of patients with severe chronic obstructive pulmonary disease? Am Rev Respir Disease 1988; 138:341–344.
- Fletcher EC, Luckett RA, Miller T, Fletcher JG. Exercise hemodynamics and gas exchange in patients with chronic obstruction pulmonary disease, sleep desaturation and daytime Pa O2 above 60 mmHg. Am Rev Respir Dis 1989; 140:1237–1245.
- Fletcher EC, Scott D, Qian W, Luckett RA, Miller CC, Goodnight-White S. Evolution of nocturnal oxyhemoglobin desaturation in patients with chronic obstructive pulmonary disease and a daytime PaO2 above 60 mmHg. Am Rev Respir Dis 1991; 144:401–405.
- Mulloy E, McNicholas WT. Ventilation and gas exchange during sleep and exercise in severe COPD. Chest 1996; 109:387–394.
- Chaouat A, Weitzenblum E, Kessler R, Charpentier C, Ehrhart M, Levi-Valensi P, Sleep-related O2 desaturation and daytime pulmonary haemodynamics in COPD patients with mild hypoxaemia. Eur Respir J 1997; 10:1730–1735.
- Krachman SL. Quaranta AJ, Berger TJ, Criner GJ. Effects of noninvasive positive pressure ventilation on gas exchange and sleep in COPD patients. Chest 1997; 112:623–628.
- Krachman SL, Chatila W, Martin UJ, Nugent T, Crocetti J, Gaughan J, Effects of lung volume reduction surgery on sleep quality and nocturnal gas exchange in patients with severe emphysema. Chest 2005; 128:3221–3228.
- National Emphysema Treatment Trial Research Group. A randomized trial comparing lung volume reduction surgery with medical therapy for severe emphysema. N Engl J Med 2003; 348:2059–2073.
- Crapo RO, Morris AH, Gardner RM. Reference spirometric values using techniques and equipment that meet ATS recommendations. ARRD 1981; 123:659–664.
- Crapo RO, Morris AH, Clayton PD, Nixon CR. Lung volumes in healthy nonsmoking adults. Bull Eur Physiopathol Respir 1982; 18:419–425.
- Gorman RB, McKenzie DK, Pride NB, Tolman JF. Gandevia SC. Diaphragm length during tidal breathing in patients with chronic obstructive pulmonary disease. Am J Respir Crit Care Med 2002; 166:1461–1469.
- Gorman RB, McKenzie DK, Butler JE, Tolman JF. Gandevia SC. Diaphragm length and neural drive after lung volume reduction surgery. Am J Respir Crit Care Med 2005; 172:1259–1266.
- Iber C, Ancoli-Israel S, Chesson A, Quan SF, for the American Academy of Sleep Medicine. Westchester, Ill: American Academy of Sleep Medicine; 2007. The AASM Manual for the Scoring of Sleep and Associated Events: Rules, Terminology and Technical Specifications.
- Ohayon MM, Carskadon MA, Guilleminault C, Vitiello MV. Meta-analysis of quantitative sleep parameters from childhood to old age in healthy individuals: Developing normative sleep values across the human lifespan. Sleep 2004; 27(7):1255–1273.
- Sanders MH, Newman AB, Haggerty CL, Redline S, Lebowitz M, Samet J, O'Connor GT, Punjabi NM, Shahar E. Sleep and sleep-disordered breathing in adults with predominantly mild obstructive airway disease. Am J Respir Crit Care Med 2003; 167:7–14.
- Berthon-Jones M, Sullivan CE. Ventilatory and arousal responses to hypoxia in normal sleeping humans. Am Rev Respir Dis 1982; 125:632–639.
- Laghi F, Harrison MJ, Tobin MJ. Comparison of magnetic and electrical phrenic nerve stimulation in assessment of diaphragmatic contractility. J Appl Physiol 1996; 80:1731–1742.
- Martinez FJ, Couser JI, Celli BR. Factors influencing ventilatory muscle recruitment in patients with chronic airflow obstruction. Am Rev Respir Dis 1990; 142:276–282.
- Smith J, Bellemare F. Effect of lung volume on in vivo contraction characteristics of human diaphragm. J Appl Physiol 1987; 62:1893–1900.
- Ballard RD, Clover CW, Suh BY. Influence of sleep on respiratory function in emphysema. Am J Respir Cirt Care Med 1995; 151:945–951.
- O'Donoghue FJ, Catcheside PG, Eckert DJ, McEvoy RD. Changes in respiration in NREM sleep in hypercapnic chronic obstructive pulmonary disease. J Physiol 2004; 559(2):663–673.
- Meurice JC, Marc I, Series F. Influence of sleep on ventilatory and upper airway response to CO2 in normal subjects and patients with COPD. Am J Respir Crit Care Med 1995; 152:1620–1626.
- Grugger M, Molloy J, Gould GA, Whyte KF, Raab GM, Shapiro CM, Ventilatory and arousal responses to added inspiratory resistance during sleep. Am Rev Respir Dis 1989; 140:1301–1307.
- Wiegand L, Zwillich CW, White DP. Sleep and the ventilatory response to resistive loading in normal men. J Appl Physiol 1988; 64:1186–1195.
- Mulloy E, McNicholas WT. Theophylline improves gas exchange during rest, exercise, and sleep in severe chronic obstructive pulmonary disease. Am Rev Respir Dis 1993; 148:1030–1036.
- Martin MJ, Pak J. Overnight theophylline concentrations and effects on sleep and lung function in chronic obstructive pulmonary disease. Am Rev Respir Dis 1992; 145:540–544.
- Berry RB, Desa MM, Branum JP, Light RW. Effect of theophylline on sleep and sleep-disordered breathing in patients with chronic obstructive pulmonary disease. Am Rev Respir Dis 1991; 143:245–250.
- Veale D, Cooper BG, Griffiths CJ, Corris PA, Gibson GJ. The effect of controlled-release salbutamol on sleep and nocturnal oxygenation in patients with asthma and chronic obstructive pulmonary disease. Respir Med 1994; 88:121–124.
- McNicholas WT, Calverley PMA, Lee A, Edwards JC. Long-acting inhaled anticholinergic therapy improves sleeping oxygen saturation in COPD. Eur Respir J 2004; 23:825–831.
- Martin RJ, Bucher B, Becki L, Hudgel DW, Lewis D, Pohl G, Effect of ipratropium bromide treatment on oxygen saturation and sleep quality in COPD. Chest 1999; 115:1338–1345.
- Benediktsdottir B, Janson C, Lindberg E, Arnardottir ES, Olafsson I, Cook E, Thorarinsdottir EH, Gislason T. Prevalence of retless legs syndrome among adults in Iceland and Sweden: Lung function, comorbidity, ferritin, biomarkers and quality of life. Sleep Med 2010; 11:1043–1048.
- Lo Coco D, Mattaliano A, Lo Coco A, Randisi B. Increased frequency of restless legs syndrome in chronic obstructive pulmonary disease patients. Sleep Med 2009; 10:572–576.
- Kaplan Y, Inonu H, Yilmaz A, Ocal S. Restless legs syndrome in patients with chronic obstructive pulmonary disease. Can J Neurol Sci 2008; 35(3):352–357.