Abstract
The evaluation of dyspnea and its responsiveness to therapy in COPD should consider the multidimensional nature of this symptom in each of its sensory-perceptual (intensity, quality), affective and impact domains. To gain new insights into mechanisms of dyspnea relief following pulmonary rehabilitation (PR), we examined effects on the major domains of dyspnea and their interaction with physiological training effects. This randomized, controlled study was conducted in 48 subjects with COPD. Subjects received either 8-weeks of PR or usual care (CTRL). Pre- and post-intervention assessments included: sensory-perceptual (i.e., exertional dyspnea intensity, dyspnea descriptors at end-exercise), affective (i.e., intensity of breathing-related anxiety during exercise, COPD self-efficacy, walking self-efficacy) and impact (i.e., activity-related dyspnea measured by the Baseline/Transition Dyspnea Index, Chronic Respiratory Questionnaire dyspnea component, St. George's Respiratory Disease Questionnaire activity component) domains of dyspnea; functional performance (i.e., 6-minute walk, endurance shuttle walk); pulmonary function; and physiological measurements during constant work rate cycle exercise at 75% of the peak incremental work rate. Forty-one subjects completed the study: PR (n = 17) and CTRL (n = 24) groups were well matched for age, sex, body size and pulmonary function. There were no significant between-group differences in pre- to post-intervention changes in pulmonary function or physiological parameters during exercise. After PR versus CTRL, significant improvements were found in the affective and impact domains but not in the sensory-perceptual domain of dyspnea. In conclusion, clinically meaningful improvements in the affective and impact domains of dyspnea occurred in response to PR in the absence of consistent physiological training effects.
Introduction
Dyspnea, or perceived respiratory discomfort, is a common symptom in COPD and contributes to activity limitation and poor health status (Citation1,2). Pulmonary rehabilitation (PR) programs incorporating exercise training and structured self-management instruction have been shown to effectively alleviate dyspnea but the underlying mechanisms of improvement are multifactorial and are poorly understood (Citation3–6).
The complex and multidimensional nature of dyspnea was carefully reviewed in the 1999 American Thoracic Society consensus statement (Citation7). It has been re-emphasized in the more recent statement which distinguishes three major domains: 1) sensory-perceptual experience (what breathing feels like); 2) affective distress (how distressing breathing is); and 3) symptom impact or burden (how dyspnea affects functional ability and health status)(Citation8). This new model has the potential to provide new insights into the mechanisms of dyspnea relief following PR and to help refine future management strategies.
Dyspnea relief following exercise training has its basis in both psychological and physiological mechanisms (Citation5,6,Citation9,10). Desensitization to dyspnea-related fear and anxiety, increased self-efficacy, improved emotional functioning and coping skills are all thought to be fundamental contributors (Citation10,11). Physiological training effects are also potentially important but are not universally achieved in PR programs for COPD. Previous studies have reported highly variable and often inconsistent improvements in respiratory function after training, which include: modest reduction (∼3–5 L/min) in ventilation as a result of improved oxidative capacity of skeletal muscles; slower breathing pattern; reduced dynamic lung hyperinflation; and increased strength or endurance of the respiratory muscles (Citation3,Citation5,Citation12–18).
The aim of this study was to determine the relative importance (and interaction) of psychological and physiological adaptations to training and their contribution to improvement in dyspnea and its main domains. Our hypothesis was that the extent of improvement in the affective distress and impact domains of dyspnea following PR would be closely linked to improvements in respiratory physiology (e.g., ventilation, breathing pattern and operating lung volumes) and, thereby, the sensory-perceptual (intensity and quality) domain. Thus, patients with COPD who were on optimal pharmacotherapy were randomized to either 8-weeks of PR (with multimodality exercise training) or usual care. We evaluated the impact of PR and usual care on the sensory-perceptual, affective and impact domains of dyspnea, and examined their association with various physiological training effects.
Methods
Subjects
Subjects included patients with COPD who were referred to the outpatient pulmonary rehabilitation program at Providence Care, Kingston, Ontario, Canada (affiliated with Queen's University and the local academic hospitals). Subjects were 40-80 years of age, had significant activity-related breathlessness (Baseline Dyspnea Index ≤ 8) (19), were on optimal pharmacotherapy in accordance with best practice recommendations (Citation20), and were clinically stable in the preceding 4 weeks. Exclusion criteria included: presence of other significant comorbidities that might contribute to dyspnea or exercise limitation; use of supplemental oxygen; or body mass index < 18 or > 40 kg/m2.
Study design
This was a randomized, controlled study (ClinicalTrials.gov Identifier: NCT01513616) in which subjects were assigned to either an 8-week pulmonary rehabilitation program (PR) or a usual care control group (CTRL). Ethical approval was received from Queen's University Health Sciences and Affiliated Teaching Hospitals Research Ethics Board (DMED-986-06) and all subjects gave informed consent. After completion of the study, CTRL subjects were offered participation in the PR program.
In an initial screening visit, subjects completed pulmonary function tests, were familiarized with all testing procedures and symptom scales, and performed an incremental cycle exercise test. Experimental visits conducted immediately before and after the 8-wk intervention period included: symptom-related questionnaires, pulmonary function tests, tests of functional ability and a constant-work rate (CWR) cycle exercise test with detailed physiological measurements. Prior to testing, subjects used their respiratory medications as usual but withdrew short-acting bronchodilators for 6 h. All visits were conducted at the same time of day for each subject.
Interventions
As part of the standard practice at our institution, all patients referred to the pulmonary rehabilitation program first met with a COPD Nurse Practitioner who provided individualized COPD counselling, education and an action plan for management of exacerbations. On average, the time spent with each patient was 60–90 minutes.
Pulmonary rehabilitation program (PR)
PR consisted of three supervised 2.5-hour sessions per week over an 8-week period. Sessions included graduated exercise training for upper and lower limbs, i.e., walking on treadmill and in corridor, cycle ergometer, arm ergometer, strength/resistance exercises for upper and lower limbs, and mobility exercises. Subjects worked at their highest attainable work rate for the longest tolerable duration by targeting at least a “moderate” intensity of breathing discomfort on the modified 10-point Borg scale (Citation21). The standardized educational portion of the program consisted of weekly information sessions using the “Living Well with COPD” self-management education modules (Boehringer Ingelheim Canada, Burlington, Ontario; www.livingwellwithcopd.com, password: copd) (Citation22). Topics included: COPD information; breathing techniques; preventing and controlling symptoms; managing emotions and stress; proper use of medications and inhalation devices; and maintaining a healthy lifestyle.
Usual medical care (CTRL)
CTRL subjects received instructions about how best to manage their disease and were provided with standard access to treatment in the event of an exacerbation. They were also offered the “Living Well with COPD” program.
Procedures
Pulmonary function tests and symptom-limited cycle exercise tests were conducted with a cardiopulmonary testing system (Vs62j body plethysmograph and Vmax229d; SensorMedics, Yorba Linda, CA, USA) as previously described (Citation23). The incremental exercise test consisted of stepwise increases in work rate of 10W/min. CWR exercise tests were conducted pre-intervention (in duplicate) and post-intervention and consisted of a 1-minute warm-up followed by an increase in work rate to 75% of the maximum incremental work rate; endurance time was defined as the duration of loaded pedaling. Breath-by-breath metabolic, cardiopulmonary and breathing pattern parameters were collected via mouthpiece. Operating lung volumes were derived from dynamic inspiratory capacity (IC) (Citation24). Subjects rated the intensity of dyspnea (“how strong is your breathing discomfort?”), breathing-related anxiety (“how anxious are you about your breathing discomfort?”) and leg discomfort at rest, every minute during exercise and at end-exercise using the 10-point Borg scale (Citation21). At exercise cessation, subjects were asked about their main reason(s) for stopping exercise and completed a dyspnea descriptor questionnaire (Citation25).
Functional tests performed pre-intervention (in duplicate) and post-intervention included: the 6-minute walk test (6MWT) (Citation26); and the endurance shuttle walk test (ESWT) which was conducted after duplicate incremental shuttle walk tests (Citation27,28). Peripheral muscle strength was evaluated during isometric knee extension with a fixed dynamometer.
Disease-specific health status was evaluated with the St. George's Respiratory Questionnaire (SGRQ) and the Chronic Respiratory Questionnaire (CRQ) (Citation29,30). Anxiety and depression were measured with Hospital Anxiety and Depression Scale (HADS) (Citation31). Subjects’ confidence in walking various distances was assessed with a 6-minute walk self-efficacy questionnaire (Citation32).
Dyspnea evaluation
Measurements collected within each dyspnea domain are summarized in . Dyspnea intensity was evaluated during CWR cycle testing and at the end of the 6MWT and ESWT with the 10-point Borg scale (Citation21). Subjects were also asked to describe their sensation of dyspnea at end-exercise using a descriptor questionnaire (Citation25). Intensity of breathing-related anxiety was rated during CWR cycle testing, as noted above (Citation10). The COPD Self-Efficacy Scale (CSES) was used to assess subjects’ confidence in managing breathing difficulty in a given situation (Citation33). Activity-related dyspnea was assessed using the Baseline Dyspnea Index (BDI) (Citation19), the Medical Research Council (MRC) dyspnea scale (Citation34), the SGRQ activity component (Citation29), and the CRQ dyspnea component (Citation30). Pre- to post-intervention changes in activity-related dyspnea were also assessed with the Transition Dyspnea Index (TDI) (Citation19).
Table 1. Domains of dyspnea measurement
Statistical analysis
A sample size estimation of 20 per group was based on dyspnea intensity ratings measured previously in our laboratory (Citation5), a relevant difference in Borg ratings of ±1, α = 0.05, 80% power and a two-tailed test of significance. Results are presented as means ±SD. Between-group comparisons of baseline subject characteristics were performed using unpaired t-tests. A repeated measures analysis of variance (ANOVA) was performed to evaluate changes over time (pre- and post-intervention); the group by time interaction term in this analysis was used to test if pre-post changes were different between the two groups. To simplify further analysis, in particular for those variables with a significant group by time interaction in the repeated measures ANOVA, paired t-tests were applied to evaluate the pre-post changes within groups. All statistical tests used a p < 0.05 level of significance. Inter-relationships between pre- to post-intervention changes were analyzed using Pearson's correlations. Analyses were performed using PASW statistics version 18 (SPSS Inc.; Chicago, IL) or Systat 8.0 for Windows (Systat Software, Inc.; Chicago, IL).
Results
A significant proportion of patients referred to the pulmonary rehabilitation program were not eligible for the study due to the presence of multiple co-morbidities, use of supplemental oxygen, or a BMI outside of the acceptable range. The main reason that eligible patients declined to participate was due to the large number of measurements being obtained and the inability to attend the study visits. Forty-eight subjects were enrolled in the study between November 2006 and September 2010 (): 4 CTRL subjects (3 COPD exacerbations, 1 symptomatic cardiac arrhythmia) and 3 PR subjects (2 COPD exacerbations, 1 non-compliant with the exercise program due to knee problems) did not complete the study. Groups were well matched for age, sex, body size and baseline pulmonary function and exercise capacity ().
Table 2. Subject characteristics at study entry
The attendance rate of the subjects who completed PR was 22.4 ± 2.2 sessions, i.e., 93% compliance. The duration of each exercise training session increased over the course of the program as demonstrated by the cycling sessions, which went from 4.7 ± 1.7 to 9.8 ± 2.0 min at week 1 to 8, respectively.
Physiological and functional measurements
There were no significant between-group differences in pre-intervention pulmonary function measurements or their response to intervention (). Physiological measurements collected during CWR cycle exercise were also not significantly different between groups in response to intervention (); although heart rate at a standardized time during exercise decreased by 3–6 beats/min (p < 0.05) within the PR group, there was no significant between-group difference in this response. Although not statistically significant across groups, there were tendencies for some measurements to improve at a standardized time near end-exercise (‘isotime’) in response to PR but not CTRL: minute ventilation (VE) decreased by 1.8 ± 4.5 (p = 0.122) vs. 0.1 ± 5.5 L/min (p = 0.903), mean expiratory flow rate (VT/TE) decreased by 0.07 ± 0.12 (p = 0.044) vs. 0.00 ± 0.14 L/s (p = 0.880), and IC increased by 0.06 ± 0.14 (p = 0.079) vs. 0.03 ± 0.38 L (p = 0.749), respectively.
Figure 1. Ventilatory responses to CWR cycle exercise in response to pulmonary rehabilitation (PR) or usual care control (CTRL). Data plotted are means ± SEM for measurements collected at steady-state rest, isotime and peak exercise. Fb, breathing frequency.
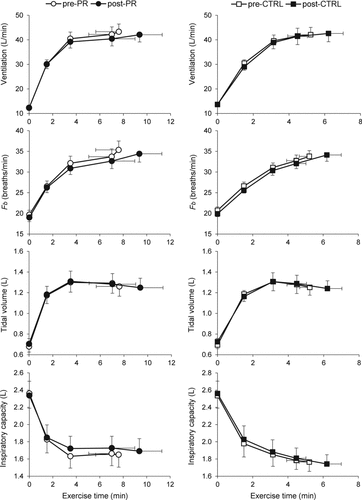
Table 3. Anthropometric and pulmonary function measurements of subjects who completed the study
Over 70% of each group stopped CWR exercise testing due to breathing discomfort, alone or in combination with leg discomfort; the distribution of reasons for stopping exercise did not change significantly in response to intervention. Although there was a 106 ± 194 s (p = 0.039) increase in CWR cycle endurance time within the PR group, the between-group difference was not statistically significant (mean difference 47 s; p = 0.411). In the group as a whole, changes in CWR cycle endurance correlated with changes in isotime exercise IC (r = 0.456, p = 0.003) and dyspnea intensity (r = –0.308, p = 0.050); changes in dyspnea and IC were inter-related (r = –0.421, p = 0.006).
The only functional task that showed a significant between-group difference in favour of PR was the 6MWT distance (p = 0.044)(). Improvements in 6MWT distance correlated with increased 6-min walk distance self-efficacy (r = 0.371, p = 0.017). There was no significant association between baseline pulmonary function or other physiological parameters and the magnitude of change in functional task performance or physiological parameters.
Table 4. Questionnaires and functional tasks
Dyspnea domains
Sensory-perceptual experience
There was no significant change in dyspnea intensity () or choice of qualitative descriptors of dyspnea at end-exercise during the CWR cycle, ESWT or 6MWT. Submaximal ratings of dyspnea intensity during the CWR cycle test fell significantly within the PR group but there was no significant between-group difference ().
Affective distress
The intensity of dyspnea-related anxiety during CWR cycling decreased significantly after PR compared with CTRL (). The CSES also improved significantly after PR compared with CTRL ().
Symptom impact
After PR compared with CTRL, activity-related dyspnea improved significantly based on all questionnaires (). Of the three dyspnea domains, the symptom impact measurements were most responsive to PR, with the TDI improvement showing the greatest statistical between-group significance (p < 0.0005). TDI correlated well with changes in the CRQ dyspnea score (r = 0.424, p = 0.006) but not with changes in the other symptom impact questionnaires.
Figure 3. Measurements of activity-related dyspnea (symptom impact) in response to pulmonary rehabilitation (PR) or usual care control (CTRL). Scores are shown for the Transition Dyspnea Index (TDI) and its components (Functional Impairment, Magnitude of Task, Magnitude of Effort), the Medical Research Council MRC) dyspnea scale, the Chronic Respiratory Questionnaire (CRQ) dyspnea component, and the St. George's Respiratory Questionnaire (SGRQ) activity component. Values are means ± SEM. *p < 0.05 within- and between-group difference; †p < 0.05 within-group difference for change with intervention.
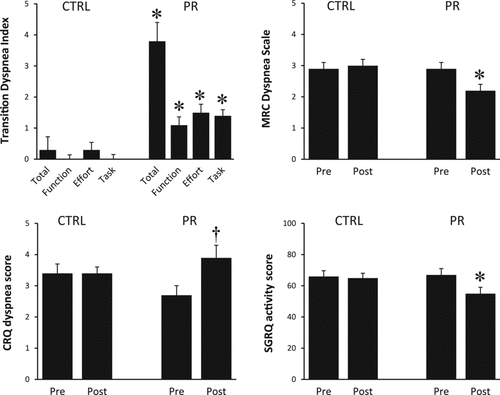
There was a significant association between changes in ratings of dyspnea intensity and breathing-related anxiety measured at isotime during CWR cycle tests (r = 0.534, p = 0.002). Changes in each symptom impact questionnaire also correlated significantly (p < 0.05) with improvements in the CSES. Other changes in measurements across dyspnea domains did not correlate significantly. There was no significant association between baseline pulmonary function or other physiological parameters and the magnitude of change in dyspnea-related scores.
Health status and affective questionnaires
The CRQ total score improved after PR compared with CTRL, as did its fatigue component (). The SGRQ total score improved within the PR group but this change was not different between groups; there were no changes in its symptom or impact components. HADS anxiety and depression improved significantly after PR compared with CTRL; within each subscale, those who had the greatest pre-intervention scores were those who experienced the greatest improvements (p < 0.0005). Changes in CRQ total scores correlated with changes in SGRQ total scores (r = –0.511, p = 0.001). Improvements in SGRQ total scores correlated with improvements in HADS anxiety (r = 0.404, p = 0.009) and depression (r = 0.415, p = 0.007) scores; while improvements in CRQ total scores correlated with improvements in HADS anxiety scores (r = –0.487, p = 0.001).
Discussion
The main findings of this study are as follows: 1) PR was primarily associated with improvements in the affective and impact domains of the new multidimensional model of dyspnea; and, 2) lack of improvement in the sensory-perceptual domain of dyspnea likely reflects the inconsistent improvements in ventilation, breathing pattern and operating lung volumes in response to PR in this sample.
Physiological training effects
In contrast to our previous non-randomized study in a similar COPD population (Citation5), there were no significant improvements in physiological responses to CWR cycle exercise in the PR group compared with the CTRL group. However, significant reductions in exercise heart rate, and small increases in quadriceps and inspiratory muscle strength were measured within the PR group. There was also a tendency for small improvements in VE, breathing pattern and operating lung volumes within the PR group that was not demonstrated in the CTRL group but did not reach statistical significance. The lack of a significant between-group difference in respiratory responses during exercise was unexpected and different from previous studies which emphasized cycle exercise training as the main intervention (Citation12,13,Citation16,Citation35).
In our study, multi-modality exercise training was individualized based on targeted dyspnea intensity ratings, training duration increases were carefully monitored by experienced physiotherapists, and adherence to the program was excellent over 8 weeks. Unlike most previous studies, we examined the additive effects of PR on pulmonary physiology in those already receiving their regular foundational pharmacotherapy. Thus, it is possible that previously reported effects of training on ventilation, breathing pattern and operating lung volumes, may have been attenuated by concurrent maximal bronchodilator treatment. At the end of 8 weeks, 6MWT distance improved significantly by a mean difference of 19 m after PR compared with CTRL. Between-group comparisons of change in CWR cycle endurance time and ESWT showed no statistical difference. However, pre- to post-intervention improvements in CWR cycle endurance time (by 106 s) and ESWT (by 256 s) were statistically significant within the PR group.
Effect on the sensory-perceptual domain of dyspnea
There was no significant between-group difference in isotime dyspnea intensity during CWR exercise, the reported exercise-limiting symptom or the qualitative descriptor choices of dyspnea measured at end-exercise. However, within the PR group, the increase in exercise endurance during cycle and walking tests was not accompanied by any further increase in peak dyspnea ratings. The lack of improvement in measurements within the sensory-perceptual domain of dyspnea (intensity and quality) likely reflects the lack of between-group differences in breathing efficiency, dynamic lung hyperinflation and ventilatory requirements. Changes in the aforementioned physiological parameters have previously been closely linked to improvement in the sensory-perceptual domain of dyspnea following various bronchodilators or oxygen treatment (Citation36–38). Similarly, in this study it is noteworthy that significant inter-relationships were found between the improvements in CWR cycle endurance time, isotime exercise IC and isotime dyspnea intensity. However, the overall respiratory physiological effects were smaller and more inconsistent compared with the effects of pharmacotherapy where the impact on affective distress and functional capacity is, in turn, likely to be less.
Effect on the affective domain of dyspnea
There is increasing recognition that dyspnea in COPD is modulated by affective (unpleasantness, fear, anxiety, depression) factors (Citation39). Our study confirms the results of previous studies that patients can discriminate and separately rank intensity of respiratory discomfort and dyspnea-related anxiety or distress during a standardized exercise test (Citation10,Citation40). Compared with CTRL, the combination of exercise training and education was associated with between-group improvements in dyspnea-related anxiety. This indicates that patients felt less anxious at a given level of exercise. There were also improvements in self-efficacy and global ratings of both anxiety and depression. Components of PR which were directed at improving emotional functioning, self-efficacy and coping skills, together with sustained psychosocial support over 8 weeks, were likely instrumental in positively influencing dyspnea-related affective distress. The significant PR-associated improvements in global ratings of both anxiety and depression are thought to favorably influence dyspnea perception (Citation11).
Effect on the impact domain of dyspnea
As in many previous studies examining the impact of PR, significant and clinically meaningful between-group differences in TDI, CRQ-dyspnea and SGRQ-activity were evident, confirming that alleviation of dyspnea following PR is linked to increased functional ability. It is noteworthy that improvements in the impact domain occurred independently of changes in the other two domains and in the absence of significant physiological training effects measured during cycle exercise.
Limitations
A larger sample size may have provided greater statistical power to uncover differences in sensory-perceptual, functional and physiological responses to PR; however, the study was sufficiently powered to demonstrate important improvements in the impact and affective dyspnea domains. Potential differences that we could not uncover within the current study may be better addressed in a future large multicentre randomized trial; such a study would also allow for stratification of patients relative to baseline dyspnea, physiology or clinical phenotypes. Exercise training was multimodality (i.e., cycling, walking, arm ergometry, resistance training, etc.) and the cycle training intensity per se may not have been high enough to achieve a task-specific physiological training effect, especially in patients with more advanced COPD. However, our program and its participants were broadly representative of most supervised hospital-based programs and adherence to the training protocol was excellent.
Conclusion
An 8-week PR program, which incorporated both education and physical training, effectively alleviated chronic dyspnea as measured by a new multidimensional model in patients with COPD. Improvement was more consistently seen in the affective and impact domains of dyspnea than in the sensory-perceptual domain. The lack of between-group differences in the intensity and quality of dyspnea during cycle exercise may be explained by the lack of between-group differences in ventilation, breathing pattern and operating lung volumes. The study raises the important question of whether closer attention should be paid to the achievement of improved respiratory physiology as a primary goal of exercise training in COPD. However, for many patients with advanced COPD entering multi-modality PR programs, physiological training effects are difficult to achieve and even to measure. Clearly, further studies are required to determine if more targeted, higher intensity training protocols that potentially improve pulmonary physiology are more effective in achieving sustained improvements in activity-related dyspnea across all three major domains.
Declaration of Interest Statement
DEO has received research funding from AstraZeneca, Boehringer Ingelheim, GlaxoSmithKline, Merck, Novartis, Nycomed and Pfizer; and has served on speakers bureaus, consultation panels and advisory boards for AstraZeneca, Boehringer Ingelheim, GlaxoSmithKline, Nycomed and Pfizer. KW received research fellowships from the Swedish Heart-Lung Foundation, Swedish Heart and Lung Association and Sweden-America Foundation. JAG was supported by the John Alexander Stewart Fellowship, Department of Medicine, Queen's University; the Natural Sciences and Engineering Research Council of Canada; and the Canadian Lung Association and Canadian Thoracic Society. KAW, MEP, NA, LS and JP have no conflicts of interest to report. Funding support for this study came from the Spear/Start Fund, Queen's University, Kingston, Ontario, Canada.
Acknowledgments
The authors would like to thank Mark Parshall, PhD, RN (College of Nursing, University of New Mexico, Albuquerque, NM, USA) for his suggestions regarding the multidimensional construct of dyspnea measurement (). The authors would also like to thank Yuk-Miu Lam, PhD (Department of Community Healthy & Epidemiology, Queen's University, Kingston, ON, Canada) for his assistance with the statistical analysis.
References
- Jones PW. Health status measurement in chronic obstructive pulmonary disease. Thorax 2001; 56:880–887.
- Troosters T, Sciurba F, Battaglia S, Langer D, Valluri SR, Martino L, Benzo R, Andre D, Weisman I, Decramer M. Physical inactivity in patients with COPD, a controlled multi-center pilot-study. Respir Med 2010; 104:1005–1011.
- Reardon J, Awad E, Normandin E, Vale F, Clark B, ZuWallack RL. The effect of comprehensive outpatient pulmonary rehabilitation on dyspnea. Chest 1994; 105:1046–1052.
- Lacasse Y, Goldstein R, Lasserson TJ, Martin S. Pulmonary rehabilitation for chronic obstructive pulmonary disease. Cochrane Database System Rev 2006(4): CD003793.
- O'Donnell DE, McGuire M, Samis L, Webb KA. General exercise training improves ventilatory and peripheral muscle strength and endurance in chronic airflow limitation. Am J Respir Crit Care Med 1998; 157:1489–1497.
- Goldstein RS, Gort EH, Stubbing D, Avendano MA, Guyatt GH. Randomised controlled trial of respiratory rehabilitation. Lancet 1994; 344:1394–1397.
- American Thoracic Society. Dyspnea. Mechanisms, assessment, and management: a consensus statement. Am J Respir Crit Care Med 1999; 159:321–340.
- Parshall MB, Schwartzstein RM, Adams L, Banzett RB, Manning HL, Bourbeau J, Calverley PM, Gift AG, Harver A, Lareau SC, Mahler DA, Meek PM, O'Donnell DE; the ATS Committee on Dyspnea. An official American Thoracic Society statement: update on the mechanisms, assessment, and management of dyspnea. Am J Respir Crit Care Med 2012; 185(4):435–452.
- Griffiths TL, Burr ML, Campbell IA, Lewis-Jenkins V, Mullins J, Shiels K, Turner-Lawlor PJ, Payne N, Newcombe RG, Ionescu AA, Thomas J, Tunbridge J. Results at 1 year of outpatient multidisciplinary pulmonary rehabilitation: a randomised controlled trial. Lancet 2000; 355:362–368.
- Carrieri-Kohlman V, Gormley JM, Douglas MK, Paul SM, Stulbarg MS. Exercise training decreases dyspnea and the distress and anxiety associated with it. Monitoring alone may be as effective as coaching. Chest 1996; 110:1526–1535.
- Janssens T, De Peuter S, Stans L, Verleden G, Troosters T, Decramer M, Van den Bergh O. Dyspnea perception in COPD. Association between anxiety, dyspnea-related fear, and dyspnea in a pulmonary rehabilitation program. Chest 2011; 140:618–625.
- Casaburi R, Porszasz J, Burns MR, Carithers ER, Change RSY, Cooper CB. Physiologic benefits of exercise training in rehabilitation of patients with severe chronic obstructive pulmonary disease. Am J Respir Crit Care Med 1997;155:1541–1551.
- Casaburi R, Patessio A, Ioli F, Zanaboni S, Donner CF, Wasserman K. Reduction in exercise lactic acidosis and ventilation as a result of exercise training in obstructive lung disease. Am Rev Respir Dis 1991; 143:9–18.
- Maltais F, LeBlanc P, Simard C, Jobin J, Berube C, Bruneau J, Carrier L, Belleau R. Skeletal muscle adaptation to endurance training in patients with chronic obstructive pulmonary disease. Am J Respir Crit Care Med 1996; 154:442–447.
- Vogiatzis I, Simoes DCM, Stratakos G, Kourepini E, Terzis G, Manta P, Athanasopoulos D, Roussos C, Wagner PD, Zakynthinos S. Effect of pulmonary rehabilitation on muscle remodelling in cachectic patients with COPD. Eur Respir J 2010; 36:301–310.
- Puente-Maestu L, Abad YM, Pedraza F, Sanchez G, Stringer WW. A controlled trial of the effects of leg training on breathing pattern and dynamic hyperinflation in severe COPD. Lung 2006; 184:159–167.
- Troosters T, Gosselink R, Decramer M. Short- and long-term effects of outpatient rehabilitation in patients with chronic obstructive pulmonary disease: a randomized trial. Am J Med 2000; 109:207–212.
- Borghi-Silva A, Arena R, Castello V, Simoes RP, Martins LE, Catai AM, Costa D. Aerobic exercise training improves autonomic nervous control in patients with COPD. Respir Med 2009; 103:1503–1510.
- Mahler DA, Weinberg DH, Wells CK, Feinstein AR. The measurement of dyspnea. Contents, interobserver agreement, and physiologic correlates of two new clinical indexes. Chest 1984; 85:751–758.
- O'Donnell DE, Aaron S, Bourbeau J, Hernandez P, Marciniuk DD, Balter M, Ford G, Gervais A, Goldstein R, Hodder R, Kaplan A, Keenan S, Lacasse Y, Maltais F, Road J, Rocker G, Sin D, Sinuff T, Voduc N. Canadian Thoracic Society recommendations for management of chronic obstructive pulmonary disease –2007 update. Can Respir J 2007; 14(Suppl B):5B–32B.
- Borg GA. Psychophysical bases of perceived exertion. Med Sci Sports Exerc 1982; 14:377–381.
- Bourbeau J, Julien M, Maltais F, Rouleau M, Beaupre A, Begin R, Renzi P, Nault D, Borycki E, Schwartzman K, Singh R, Collet JP; for the Chronic Obstructive Pulmonary Disease axis of the Respiratory Network, Fonds de la Recherche en Sante du Quebec. Reduction of hospital utilization in patients with chronic obstructive pulmonary disease. A disease-specific self-management intervention. Arch Intern Med 2003; 163:585–591.
- O'Donnell DE, Hamilton A, Webb KA. Sensory-mechanical relationships during high-intensity, constant-work-rate exercise in COPD. J Appl Physiol 2006; 101:1025–1035.
- O'Donnell DE, Revill SM, Webb KA. Dynamic hyperinflation and exercise intolerance in chronic obstructive pulmonary disease. Am J Respir Crit Care Med 2001; 164:770–777.
- O'Donnell DE, Hong HH, Webb KA. Respiratory sensation during chest wall restriction and dead space loading in exercising men. J Appl Physiol 2000; 88:1859–1869.
- American Thoracic Society. ATS statement: guidelines for the six-minute walk test. Am J Respir Crit Care Med 2002; 166:111–117.
- Singh SJ, Morgan MD, Scott S, Walters D, Hardman AE. Development of a shuttle walking test of disability in patients with chronic airways obstruction. Thorax 1992; 47:1019–1024.
- Revill SM, Morgan MD, Singh SJ, Williams J, Hardman AE. The endurance shuttle walk: a new field test for the assessment of endurance capacity in chronic obstructive pulmonary disease. Thorax 1999; 54:213–222.
- Jones PW, Quirk FH, Baveystock CM, Littlejohns P. A self-complete measure of health status for chronic airflow limitation. The St. George's Respiratory Questionnaire. Am Rev Respir Dis 1992; 145:1321–1327.
- Guyatt GH, Berman LB, Townsend M, Pugsley SO, Chambers LW. A measure of quality of life for clinical trials in chronic lung disease. Thorax 1987; 42:773–778.
- Zigmond AS, Snaith RP. The hospital anxiety and depression scale. Acta Psychiatr Scand 1983; 67:361–370.
- Lox CL, Freehill AJ. Impact of pulmonary rehabilitation on self-efficacy, quality of life and exercise tolerance. Rehab Psych 1999; 44:208–221.
- Wigal JK, Creer TL, Kotses H. The COPD Self-Efficacy Scale. Chest 1991; 99:1193–1196.
- Brooks SM ( chair); ATS Task Group on Screening for Respiratory Disease in Occupational Settings. Surveillance for respiratory hazards in the occupational setting. Am Rev Respir Dis 1982; 126:952–956.
- Maltais F, LeBlanc P, Jobin J, Bérubé C, Bruneau J, Carrier L, Breton MJ, Falardeau G, Belleau R. Intensity of training and physiologic adaptation in patients with chronic obstructive pulmonary disease. Am J Respir Crit Care Med 1997; 155:555–561.
- O'Donnell DE, Fluge T, Gerken F, Hamilton A, Webb K, Aguilaniu B, Make B, Magnussen H. Effects of tiotropium on lung hyperinflation, dyspnoea and exercise tolerance in COPD. Eur Respir J 2004; 23:832–840.
- O'Donnell DE, Voduc N, Fitzpatrick M, Webb KA. Effect of salmeterol on the ventilatory response to exercise in chronic obstructive pulmonary disease. Eur Respir J 2004; 24:86–94.
- O'Donnell DE, D'Arsigny C, Webb KA. Effects of hyperoxia on ventilatory limitation during exercise in advanced chronic obstructive pulmonary disease. Am J Respir Crit Care Med 2001; 163:892–898.
- O'Donnell DE, Banzett RB, Carrieri-Kohlman V, Casaburi R, Davenport PW, Gandevia SC, Gelb AF, Mahler DA, Webb KA. Pathophysiology of dyspnea in chronic obstructive pulmonary disease: a roundtable. Proc Am Thorac Soc 2007; 4:145–168.
- Wilson RC, Jones PW. Differentiation between the intensity of breathlessness and the distress it evokes in normal subjects during exercise. Clin Sci 1991; 80:65–70.