Abstract
Receptor protein tyrosine phosphatases (RPTPs) have cell adhesion molecule–like extracellular domains coupled to cytoplasmic tyrosine phosphatase domains. PTPμ is the prototypical member of the type IIb subfamily of RPTPs, which includes PTPρ, PTPκ, and PCP-2. The authors performed the first comprehensive analysis of the subfamily in one system, examining adhesion and antibody recognition. The authors evaluated if antibodies that they developed to detect PTPmu also recognized other subfamily members. Notably, each antibody recognizes distinct subsets of type IIb RPTPs. PTPμ, PTPρ, and PTPκ have all been shown to mediate cell-cell aggregation, and prior work with PCP-2 indicated that it can mediate bead aggregation in vitro. This study reveals that PCP-2 is unique among the type IIb RPTPs in that it does not mediate cell-cell aggregation via homophilic binding. The authors conclude from these experiments that PCP-2 is likely to have a distinct biological function other than cell-cell aggregation.
INTRODUCTION
Receptor protein tyrosine phosphatases (RPTPs) comprise a family of proteins with extracellular cell adhesion molecule (CAM) domains coupled to cytoplasmic tyrosine phosphatase domains, and thus can influence many biological processes, including cancer progression (CitationOstman et al. 2006). The type IIb PTPμ-like subfamily of RPTPs includes PTPμ, PTPρ, PTPκ, and PCP-2 (PTPλ) (CitationBrady-Kalnay 2001). The extracellular segment of the PTPμ-like RPTPs contains a Meprin-A5 (neuropilin)-mu (μ) (MAM) domain, an immunoglobulin (Ig) domain, and four fibronectin type III (FNIII) repeats (CitationBrady-Kalnay 2001; CitationOstman et al. 2006). Their intracellular segment contain a juxtamembrane domain with sequence similarity to the cytoplasmic domain of classical cadherins, as well as two tandem tyrosine phosphatase domains. Globally, type IIb RPTPs share approximately 55% sequence identity, whereas their cytoplasmic domains share approximately 68% sequence identity.
PTPμ subfamily members bind homophilically (i.e., the “ligand” for PTPμ is an identical PTPμ molecule on an adjacent cell) (CitationAricescu et al. 2008; CitationBrady-Kalnay et al. 1993; CitationCheng et al. 1997; CitationGebbink et al. 1993; CitationSap et al. 1994; CitationYu et al. 2008; CitationZondag et al. 1995). The Ig domain of PTPμ is responsible for promoting homophilic interactions (CitationBrady-Kalnay and Tonks 1994), and proper cell surface localization (CitationDel Vecchio and Tonks 2005). However, various laboratories have demonstrated that both the MAM and Ig domains as well as the first two FNIII repeats play a role in efficient cell-cell adhesion of PTPμ (CitationAricescu et al. 2006, Citation2007; CitationBrady-Kalnay and Tonks 1994; CitationZondag et al. 1995). PTPρ and PTPκ also mediate cell-cell adhesion via homophilic binding (CitationSap et al. 1994; CitationYu et al. 2008). To date, extracellular fragments of PCP-2 have only been demonstrated to mediate aggregation of beads in vitro (CitationCheng et al. 1997). Despite the amino acid sequence similarity between type IIb RPTPs, no heterophilic interactions between the subfamily members have been observed. For example, PTPμ does not interact heterophilically with either PTPκ (CitationZondag et al. 1995) or PTPρ (CitationYu et al. 2008).
PCP-2 was originally identified in a human pancreatic adenocarcinoma cDNA library, and was shown to localize to adherens junctions (CitationWang et al. 1996). PCP-2 is predicted to be a tumor suppressor gene based on its reduced expression in melanoma cell lines and tissue biopsies (CitationMcArdle et al. 2001). Like other type IIb RPTPs (CitationLilien 2005; CitationSallee et al. 2006), PCP-2 also regulates the cadherin-catenin complex. PCP-2 interacts with and dephosphorylates β-catenin in vivo, thereby promoting E-cadherin–mediated adhesion (CitationYan et al. 2002, Citation2006). Although structural studies suggest there is little difference between type IIb RPTPs, whether PCP-2 is capable of mediating cell-cell adhesion and thus capable of functioning like other type IIb RPTPs remains to be tested.
In this study, we tested the specificity of PTPμ antibodies that we generated and are now commercially available. Our findings demonstrate that each PTPμ antibody recognizes distinct members of the type IIb subfamily of RPTPs. Based on recombinant PTPμ fragment binding experiments and sequence comparison, we were able to predict the epitopes on the type IIb RPTPs that the antibodies bind to. In addition, we characterize the ability of PCP-2 to mediate cellular aggregation. Our results demonstrate that PCP-2 is unique among the type IIb RPTPs in its inability to mediate cell-cell aggregation. Domain-swapping experiments demonstrate that either the MAM or Ig domain of PCP-2 is capable of weakly substituting for the homologous domain when inserted into otherwise wild-type PTPρ. We compared the type IIb subfamily members using homology modeling based upon the PTPμ crystal structure. This modeling illustrates the unique surface charges of the adhesive interfaces of each type IIb subfamily member, which may account for the fact that no heterophilic binding is observed in this subfamily. In summary, the experiments described in this article emphasize that despite sharing enough sequence similarity to be recognized by some of the same antibodies, the type IIb RPTPs function in distinct manners biologically. Our data suggest that PCP-2 has a function other than mediating cell-cell aggregation.
METHODS
Antibodies
The following monoclonal antibodies, generated using the intracellular domain of PTPμ as the antigen, were used in this study: SK6, SK7, SK10, SK15, and SK18 (CitationBrady-Kalnay et al. 1993; CitationBrady-Kalnay and Tonks 1994). A monoclonal antibody to the C-terminal portion of PTPμ (termed C-terminal PTPμ antibody) was also used (CitationGebbink et al. 1991), The C-terminal antibody was generated using a synthetic peptide of amino acids 1436–1452 corresponding to the predicted C-terminus of PTPμ (CitationGebbink et al. 1991).
Baculovirus Generation
Baculoviruses were generated by transfecting cells with the BaculoGold Linearized Baculovirus DNA (BD Biosciences, San Diego, CA) according to the manufacturer's protocol. Baculoviruses for PTPμ (CitationBrady-Kalnay et al. 1993) and PTPρ (CitationYu et al. 2008) were previously described. PTPRK-pBluescipt plasmid (kind gift from Dr. Jan Sap) was digested with EcoRI and HpaI and ligated into the pVL1393 vector digested with EcoRI and SmaI. Full-length PCP-2 was removed from the pMT2-PCP-2 construct using NotI and XbaI and ligated into the pVL1392 vector digested with NotI and XbaI. The green florescent protein (GFP) baculovirus pVL1393-GFP was obtained from BD Biosciences. The RFP baculovirus was generated by cloning the pmRFP1 coding sequence (CitationCampbell et al. 2002) into pVL-1393 using the restriction sites BamH1 and EcoR1.
The PTPρ-PCP-2 MAM and Ig chimeras were generated by fusion polymerase chain reaction (PCR) as previously described (CitationHiguchi et al. 1988). Briefly, taking the PTPρ-PCP-2 MAM chimera as an example, the MAM domain of PCP-2 was amplified from a PCP-2 plasmid. In order to perform the cloning, two fragments were amplified from pVL1392- PTPρ at the same time. One fragment encodes the PTPρ signal sequence starting from the EcoRV site, which is in the vector, just 5′ to the MAM domain of PTPρ. An overhang of 18 nucleotides that corresponds to the first 18 nucleotides of the MAM domain of PCP-2 was introduced to the 3′ end of the fragment by the reverse primer. The other primer starts at the sequence at the 3′ end of the MAM domain of PTPρ to the BglII site, which is the closest restriction site available in the DNA sequence of PTPρ. An overhang of 18 nucleotides that matches the last 18 nucleotides of the MAM domain of PCP-2 was introduced to the 5′ end of the fragment by the forward primer. The PCR products were mixed and used as templates for another round of PCR to generate PTPρ-PCP-2 MAM chimeric DNA. The fused PCR products were cloned into pVL1392-PTPρ using EcoRV and BglII. No additional amino acids were introduced, as confirmed by DNA sequencing.
The PCP-2 MAM domain: forward primer 5′-cccggcgcccgggctcagactccggcagctggctgc-3′; reverse primer 5′-tcgcagaaaatgaggtgcctttgcgcaggggtagct-3′. Two flanking sequence of PTPρ: forward primer-1 5′-ttgctgatatcatggagataattaaaa-3′, reverse primer-1 5′-gcagccagctgccggagtctgagcccgggcgccggg-3′ or forward primer-2 5′-agctacccctgcgcaaaggcacctcattttctgcga-3′ and reverse primer-2 5′-cctttgaggagaagatctacatcc-3′. Primers used to fuse these three fragments together: forward primer 5′-ttgctgatatcatggagataattaaaa-3′ and reverse primer 5′-cctttgaggagaagatctacatcc-3′.
PTPρ-PCP-2 Ig chimera was generated using the same strategy as used for MAM-swapping mutant except that the primers are as follows: The PCP-2 Ig domain: forward primer 5′-gcacctcattttctgcgactgggcgacgtggaggtc-3′; reverse primer 5′-aatgggcgtgggaggctccttgacgatgagctccgc-3′. Two flanking sequences of PTPρ: forward primer-1 5′-ttgctgatatcatggagataattaaaa-3′; reverse primer-1 5′-gacctccacgtcgcccagtcgcagaaaatgaggtgc-3′ and forward primer-2 5′-gcggagctcatcgtcaaggagcctcccacgcccatt-3′ and reverse primer-2 5′-cctttgaggagaagatctacatcc-3′. Primers used to fuse three fragments together: forward primer: 5′-ttgctgatatcatggagataattaaaa-3′; reverse primer 5′-cctttgaggagaagatctacatcc-3′.
PTPρ-PCP-2 extracellular chimera: Extracellular domain of PCP-2 was amplified from a PCP-2 plasmid using forward primer 5′-tgaccagaattcatggcccgtgcccaggcg-3′ and reverse primer 5′-tgaccagaattctccttgcaggcagctttc-3′ starting from the start codon ATG to the sequence 5′ of the EcoRI site in the PTPρ gene, which is very close to the transmembrane domain. The fragment was cloned into pVL1392-PTPρ using EcoRI, which was introduced into the primers.
Sf9 Cell Culture
Sf9 insect cells (BD Biosciences, San Jose, CA) were maintained at 27°C in Graces Insect Medium (BD Biosciences). Medium was supplemented with 10% fetal bovine serum (FBS) (Hyclone, Logan, UT) and gentamicin (MP Biomedicals, Solon, OH). Cells were plated in T25 flasks and 1 ml of the viruses described above were added to Sf9 cell medium to induce the expression of proteins of interest. Cells were harvested 30 h post infection for further analysis. For heterophilic cell-cell aggregation assays, coinfection with 1 ml of RFP or GFP virus and 1 ml of PTP virus was performed by simultaneous addition to the Sf9 cell culture. The aggregation assays were performed 48 h post infection for maximal fluorescent protein expression.
Immunoblotting
Sf9 cells were lysed in a standard lysis buffer (20 mM Tris·HCl, pH 7.5, 1% Triton X-100, 150 mM sodium chloride, 2 mM benzamidine, 2.5 μg/ml aprotinin, 2.5 μg/ml leupeptin, and 0.5 μg/ml pepstatin). Samples were solubilized in an equal volume of 2× sodium dodecyl sulfate (SDS) loading buffer and separated by SDS–polyacrylamide gel electrophoresis (PAGE). Samples were transferred to nitrocellulose membrane (Whatman, Piscataway, NJ) and immunoblotted with anti-PTPμ antibodies as described (CitationBrady-Kalnay et al. 1993).
Immunocytochemistry
Sf9 cells were fixed with 2% paraformaldehyde diluted in 1× phosphate-buffered saline (PBS) for 30 min at room temperature. Cells were permeabilized and blocked with 0.5% saponin containing 10% goat serum diluted in 1× PBS for 45 min. Cells were incubated with mouse monoclonal anti-PTPμ (SK15) antibody for 1 h, then washed, followed by addition of Texas Red–conjugated goat anti-mouse IgG secondary antibody (Jackson ImmunoResearch Laboratories, West Grove, PA) for 1 h, washed, and imaged. Images were captured with a Nikon TE-200 inverted fluorescence microscope.
Cell Surface Expression of RPTPs
Cell surface expression of PTPμ, PTPρ, PTPκ, and PCP-2 proteins in baculovirus-infected Sf9 cells was determined using a modified biotin-avidin reaction with the Cell Surface Protein Isolation Kit (Pierce, Rockford, IL), as previously described (CitationYu et al. 2008). Briefly, Sf9 cell medium was removed and the flasks were incubated with rocking for 30 min at 4°C in 5 ml of 0.25 mg/ml Sulfo-NHS-SS-Biotin in 1× PBS. The cells were mechanically removed from the flask, centrifuged, washed, and lysed according to the manufacturer's protocols. To isolate cell surface proteins, the cell lysate was added to a NeutrAvidin Gel column and incubated for 60 min at room temperature (RT) with rocking prior to washing and eluting proteins with 200 μl of sample buffer containing a final concentration of 55 mM dithiothreitol (DTT) for 60 min at RT. The flow through was run on 6% SDS-PAGE gels and transferred to nitrocellulose. Total cell surface protein levels were normalized by immunoblotting cell lysates with an anti-actin antibody (JLA20). The normalized cell surface protein was run on 6% SDS-PAGE gels, transferred to nitrocellulose, and immunoblotted with antibodies to PTPμ (SK7, SK15 or and SK18) or actin (JLA20) followed by secondary antibody conjugated to horseradish peroxidase (HRP). The HRP signal was detected using a Fluor-S MAX MultiImager (Bio-Rad Laboratories, Hercules, CA).
Expression of Fusion Proteins in Esherichia coli
Plasmids containing the intracellular GST-PTPμ fusion proteins, GST-iPTPμ: 765–1452, GST-iPTPμ: 765–1178, GST-PTPμ: 765–958, and GST-iPTPμ: 816–1452 (iPTPμ-Δ765–815) were previously described (CitationPhillips-Mason et al. 2006). These fusion proteins were expressed in bacteria under the control of the lac operon and isolated on glutathione Sepharose 4B (GE Healthcare, Piscataway, NJ).
The pTrcHis plasmid was used to generate T7-6(His)-tagged PTPμ fusion proteins. Generation of His-iPTPμ: 774–1161, His-iPTPμ: 1213–1452, and His-iPTPμ: 915–1161 have been described (CitationPhillips-Mason et al. 2008). Using PCR, we generated a plasmid encoding most of the juxtamembrane domain (His-iPTPμ: 774–924) that expresses a T7/His-tagged fusion protein containing amino acids 774–924 of PTPμ. The T7/His-tagged fusion proteins were expressed in E. coli under the control of the lac operon. These proteins were purified using the PrepEase His-Tagged Protein Purification Kit (USB, Cleveland, OH).
Aggregation Assays
Sf9 cells were harvested by trituration. The cell suspensions were added to glass scintillation vials and incubated at 27°C at 90 rpm in a gyratory shaker for 30 min. For a given condition, the cell suspension was transferred to a 100-mm Petri dish either prior to (0 time point) or 30 min after aggregation. Images were captured with a Nikon TE-200 inverted fluorescent microscope (Tokyo, Japan). To quantify cell aggregates, pictures of four randomly selected fields were taken per dish. The area of each object (single cells and aggregates) was determined by the Metamorph software (Molecular Devices, Downington, PA) using an auto-threshold setting for light objects and appropriate size filters that allowed the counting of cells and aggregates but not debris. The four replicates were combined to yield average readings per condition. The percent aggregation was then calculated as the average aggregate area at 30 min minus the average aggregate area at 0 min divided by the average aggregate area at 30 min [(N30 − N0)/N30]. A minimum of three independent experiments was performed per condition. Statistical significance was determined in Microsoft Excel using Student's t test. Error bars indicate standard error.
Surface Electrostatic Potential Calculations of Type IIb RPTPs
Homology models were generated by submitting the human protein sequence for the PTP of interest to the phyre protein homology server (http://www.sbg.bio.ic.ac.uk/phyre). Resultant models were inspected and corrected in Coot (http://www.biop.ox.ac.uk/coot/) with structural idealization in REFMAC (http://www.ysbl.york.ac.uk/∼garib/refmac/). APBS (http://www.poissonboltzmann.org/apbs/) was utilized in concert with PyMOL (Schrödinger, New York, NY) to calculate and visualize electrostatic surface potentials.
Results
Cross-reactivity of PTPμ Intracellular Antibodies
Previously, a series of antibodies termed the SK antibodies were generated using the entire intracellular domain of PTPμ as an antigen (CitationBrady-Kalnay et al. 1993). The cytoplasmic domains of the type IIb RPTPs share approximately 55% sequence identity at the amino acid level (CitationBrady-Kalnay 1998). Given this high degree of similarity, it is likely that these antibodies cross-react with other type IIb subfamily members and may serve as useful reagents for studies evaluating these RPTPs as well. To test this, type IIb RPTPs were expressed in Sf9 insect cells by infecting them with baculovirus encoding the different RPTPs. Sf9 cell lysates were then probed with an antibody generated by using a peptide from the C-terminus of PTPμ, termed the C-terminal antibody (CitationGebbink et al. 1991) and the SK series monoclonal antibodies: SK6, SK7, SK10, SK15, SK18 (CitationBrady-Kalnay et al. 1993) ( and ). PTPμ intracellular antibodies recognize proteins at the expected molecular weight of type IIb RPTPs, approximately 200 kDa. All of these antibodies recognize PTPμ. Of the six PTPμ antibodies, three, SK6, SK10, and the PTPμ C-terminal antibody, exclusively bound to PTPμ (). In addition to recognizing PTPμ, SK18 recognizes PTPρ, SK7 recognizes PTPκ and PCP-2, and SK15 recognizes PTPρ and PCP-2 ( and ). All of these proteins, as expected, were expressed on the surface of Sf9 cells ().
Figure 1. Cross-reactivity of PTPμ intracellular antibodies with other type IIb RPTPs. Sf9 cells infected to express the type IIb RPTPs subfamily members were lysed and analyzed by immunoblotting with the following anti-PTPμ cytoplasmic antibodies: SK6, SK7, SK10, SK15, SK18, and a C-terminal PTPμ antibody (A). Proteins expressed at the cell surface were isolated and immunoblotted with SK7 and SK18 (B). A summary of the cross-reactivity of the SK series of antibodies and the C-terminal PTPμ antibody with type IIb RPTP members is shown in (C).
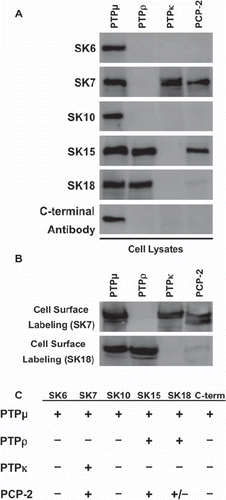
Identification of the Binding Regions of the SK Series of PTPμ Intracellular Antibodies
To identify the binding regions of the PTPμ intracellular antibodies used above, the antibodies were tested for their ability to recognize recombinant PTPμ cytoplasmic domain deletion constructs in immunoblot experiments. All antibodies examined recognized the complete cytoplasmic domain fusion protein GST-iPTPμ: 765–1452, encoding the juxtamembrane domain and two tyrosine phosphatase domains, containing amino acids (AA) 765–1452 ( and ). SK6, SK7, and SK10 all recognized the same PTPμ fusion proteins and failed to recognize the fusion proteins lacking residues within the juxtamembrane domain, His-PTPμ: 915–1161 and His-PTPμ: 1213–1452 (). SK15 did not recognize fusion proteins lacking the first phosphatase domain, including GST-iPTPμ: 765–958, His-iPTPμ: 1213–1452, or His-iPTPμ: 774–924 (). SK18 also did not recognize fusion proteins lacking the first phosphatase domain, including His-iPTPμ: 1213–1452 or the His-iPTPμ: 774–924 (). Unlike SK15, however, SK18 did recognize the fusion proteins GST-iPTPμ: 765–958 (), indicating that the binding site resides between residues 925 and 958. As a control, the PTPμ C-terminal antibody was also evaluated in our studies. The C-terminal PTPμ antibody was generated using a peptide of residues 1436–1452. As is expected, this antibody did not recognize proteins lacking the membrane distal phosphatase domain, which contains residues 1436-1452 (). These results indicate that the epitopes recognized by the different PTPμ antibodies are localized to the following sequences: SK6, SK7, and SK10: 816–914; SK15: 958–1161; SK18: 925–958; the C-terminal PTPμ antibody: 1436–1452.
Figure 2. Binding of the SK series of PTPμ antibodies is eliminated when specific amino acids are deleted in the cytoplasmic domain of PTPμ fusion proteins. Recombinant PTPμ fusion proteins containing discrete amino acid composition were resolved by SDS-PAGE and immunoblotted with the SK series of antibodies and the C-terminal PTPμ antibody (A). An illustration of the PTPμ recombinant proteins is shown in (B) along with a summary of the epitope binding experiments.
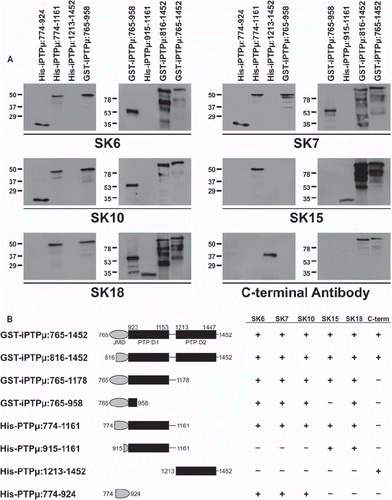
Identification of SK Antibody Series Binding Sites by Amino Acid Sequence Comparison of Type IIb RPTP Subfamily Members
The specificity of PTPμ intracellular antibodies for type IIb RPTPs () and the ability of these antibodies to bind to various PTPμ deletion constructs () were cross-referenced with the RPTP type IIb cytoplasmic domain sequences (). Using this information, we made predictions as to where the PTPμ intracellular antibodies likely bind. For example, to determine the epitopes for the PTPμ specific antibodies SK6 and SK10, we identified regions of amino acid differences between PTPμ and other type IIb subfamily members within the predicted epitope domains determined using the fusion protein experiments. On the other hand, for antibodies that cross-reacted with other subfamily members, we looked for regions of greatest similarity between the different members to identify the most likely region of epitope binding. SK6 and SK10 binding was eliminated when residues 816–914 were truncated from intracellular PTPμ fusion proteins (). Based on the lack of cross-reactivity with other type IIb RPTPs (), SK6 and SK10 are predicted to bind to residues 816–867, a region with little homology to other type IIb RPTPs (, black box). SK7 also did not recognize the recombinant PTPμ proteins lacking residues 816-914 (). In cross-reactivity studies, SK7 recognized PTPμ, PTPκ, and PCP-2 (), suggesting that the epitope is a region of sequence similarity between these three RPTPs, which could either be between residues 850 and 855 or residues 868 and 877 (, black box). SK15 did not bind to intracellular PTPμ truncations lacking residues 958–1161 within the first phosphatase domain (). SK15 also recognizes PTPμ, PTPρ, and PCP-2 (). The region between residues 958 and 1161 has a high degree of similarity between all subfamily members. Therefore, we could not further narrow down the binding site of SK15 within this region (). SK18 did not recognize the fusion proteins His-iPTPμ: 1213–1452 or the His-iPTPμ: 774–924, which lack amino acids within the first phosphatase domain but did recognize GST-iPTPμ: 765–958 (). PTPμ and PTPρ but not PCP-2 were recognized by SK18 (). The SK18 binding region is predicted to be an area of least sequence similarity with PCP-2 somewhere between residues 924 and 958, most likely 941–947 (). The PTPμ C-terminal antibody did not bind any PTPμ intracellular truncations that lacked the membrane distal phosphatase domain (), in agreement with the fact that this antibody was generated using a synthetic peptide of residues 1436–1452 corresponding to the predicted C-terminus of PTPμ () (CitationGebbink et al. 1991). Our analysis did not allow us to narrow down the epitope further than these 16 residues. Our studies indicate that SK6, SK10, and the C-terminal antibody are highly specific to PTPμ. In addition, our antibody analysis indicates that small variations between the subfamily members in the juxtamembrane domain and/or membrane distal phosphatase domain are sufficient to prevent antibodies that bind to epitopes within these domains from recognizing all subfamily members.
Figure 3. Identification of the epitopes of the SK series of PTPμ antibodies based on amino acid sequence comparison between type IIb RPTPs. SK6 and SK10 (A) are predicted to bind to amino acids 816–914 in the juxtamembrane domain (JMD-W) and are outlined by a black box. SK7 is predicted to bind to the residues outlined by the black box in (B), 850–855 or 868–877, also in JMD-W. SK15 likely recognizes 958–1161 in the first phosphatase domain (PTP1) (C), whereas SK18 recognizes the residues outlined by the black box in (D), 941–947, also in PTP1. The PTPμ C-terminal antibody is known to bind to residues 1436–1452 (E). Regions of identity between the type IIb RPTP amino acid sequences are shaded gray.
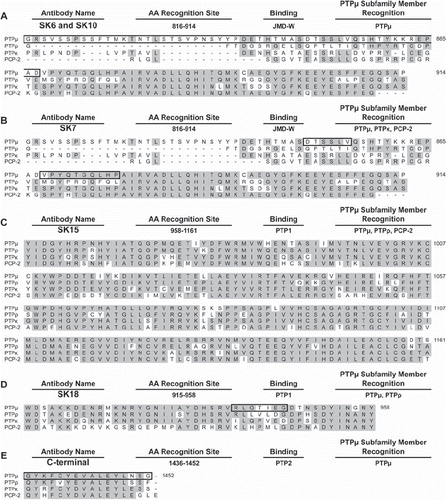
PCP-2 Does Not Mediate Cell-Cell Aggregation In Vivo
Our antibody studies demonstrate a high degree of amino acid sequence similarity and cross-reactivity of the PTPμ-subfamily members. Given this high degree of similarity we wanted to examine whether all of the subfamily members are able to mediate cell-cell adhesion. Previous studies demonstrated that PTPμ, PTPρ, and PTPκ all induce cell-cell aggregation of nonadhesive Sf9 insect cells (CitationBrady-Kalnay et al. 1993; CitationCheng et al. 1997; CitationGebbink et al. 1993; CitationSap et al. 1994a; CitationYu et al. 2008; CitationZondag et al. 1995). To date, PCP-2 had not been tested for its ability to mediate aggregation of cells. To this end, Sf9 cells were infected with baculovirus to induce expression of PTPμ, PTPρ, PTPκ, or PCP-2. RPTP-expressing Sf9 cells were then used in aggregation assays. We confirmed that PTPμ, PTPρ, and PTPκ mediate Sf9 cell-cell aggregation (). Unlike other type IIb RPTPs, however, PCP-2 did not induce cell-cell aggregation when expressed in Sf9 cells ().
Figure 4. PCP-2 does not mediate homophilic cell-cell aggregation. Nonadhesive Sf9 cells were infected with baculoviruses expressing different type IIb RPTP subfamily members and allowed to aggregate for 30 min prior to imaging. PTPμ, PTPρ, PTPκ all mediate homophilic cell aggregation, whereas PCP-2 does not.
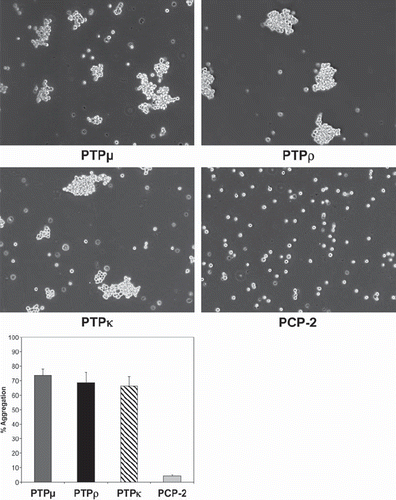
PCP-2 Does Not Interact Heterophilically to Mediate Cell-Cell Aggregation
CAMs can bind heterophilically to mediate adhesion (CitationDuguay et al. 2003; CitationNiessen and Gumbiner 2002). To test whether PCP-2 may bind heterophilically to other type IIb RPTPs to mediate cell-cell aggregation, one set of Sf9 cells was coinfected with viruses expressing full-length PCP-2 and green fluorescent protein (GFP), whereas another set of Sf9 cells was coinfected with viruses expressing either full-length PTPμ, PTPρ, or PTPκ and red fluorescent protein (RFP). The PCP-2–expressing cells were mixed with an equal number of cells that express PTPμ, PTPρ, or PTPκ and tested in aggregation assays. As shown in the overlay image of , the PCP-2–labeled cells did not bind heterophillically or aggregate with the PTPμ-, PTPρ-, or PTPκ-expressing cells.
Figure 5. PCP-2 does not interact in trans with other type IIb RPTPs to mediate heterophilic cell-cell aggregation. Sf9 cells expressing both GFP and PCP-2 were mixed with Sf9 cells expressing RFP and either PTPμ, PTPρ, or PTPκ. After 30 min of aggregation, the cells were imaged. Phase-contrast images show that aggregates form. The overlay fluorescent images demonstrate that these aggregates are composed exclusively of RFP-expressing cells, and exclude GFP/PCP-2–expressing cells.
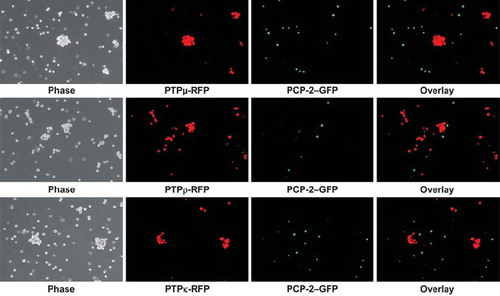
PTPκ-Expressing Cells Sort Away From Cells Expressing Other Type IIb RPTPs
Cells expressing PCP-2 do not aggregate with cells that express other type IIb RPTPs. We wanted to evaluate whether the same was true for another type IIb RPTP, PTPκ. To evaluate whether cells that express PTPκ sort away from cells that express other type IIb RPTPs, aggregation assays were conducted by mixing equal numbers of cells expressing either PTPκ and PTPμ, or PTPκ and PTPρ. Using the overlay image of the coinfected fluorescent protein expression to distinguish the two populations of cells as described above, we found that Sf9 cells expressing PTPκ sort away from Sf9 cells that express either PTPμ (), in agreement with previously published findings using lipophilic dyes (CitationZondag et al. 1995), or PTPρ to exclusively form aggregates of cells that express only one type IIb RPTP (). These results are similar to our recent study demonstrating that PTPρ and PTPμ sort into independent aggregates (CitationYu et al. 2008). To date, no heterophilic binding of type IIb RPTPs capable of mediating cell-cell aggregation has been observed. A control experiment with Sf9 cells expressing only RFP or GFP demonstrated that these two fluorescent proteins do not induce Sf9 cell aggregation. To confirm the coexpression of both the fluorescent protein and the phosphatase, we performed immunocytochemistry on Sf9 cells coinfected with the two proteins (). The overlay images of the PCP-2/GFP or PTPμ/GFP coinfected cells demonstrate that the Sf9 cells express both the phosphatase and GFP.
Figure 6. PTPκ-expressing cells sort away from PTPμ- and PTPρ-expressing cells. Sf9 cells coinfected with RFP and PTPκ were mixed with cells coinfected with GFP and either PTPμ or PTPρ and subjected to aggregation assay for 30 min. A control experiment with Sf9 cells expressing only RFP or GFP demonstrate that these two fluorescent proteins do not induce Sf9 cell aggregation. The overlay images demonstrate that only exclusively red or green aggregates formed in all aggregation experiments, demonstrating that the type IIb RPTPs sort away from each other. Phosphatase expression was detected in Sf9 cells grown in culture by immunocytochemistry with SK15 antibody and a Texas Red secondary antibody. The GFP expression following coinfection is shown in green. The overlay image demonstrates that GFP is coexpressed in the same cells as the phosphatases PCP-2 or PTPμ.
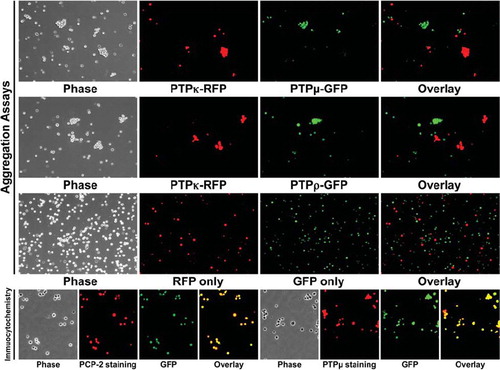
Swapping the MAM or Ig Domain of PCP-2 Into PTPρ Creates a Unique Protein Capable of Mediating Weak Cell-Cell Aggregation
In order to identify whether the MAM or Ig domains of PCP-2 are functionally capable of mediating cell-cell adhesion, we conducted domain-swapping experiments between PCP-2 and PTPρ. We made domain-swapping mutants between PTPρ and PCP-2 based upon our recent studies on the ability of PTPρ to mediate cell adhesion (CitationYu et al. 2008; CitationZhang et al. 2010). We swapped the MAM, Ig, or entire extracellular domain of PCP-2 into an otherwise wild-type PTPρ expression vector and expressed the chimeric proteins in Sf9 cells. All constructs were efficiently expressed and localized to the cell surface (). As a control for the cell surface labeling experiments, we verified that actin was expressed in the cell lysates but not in the cell surface labeling samples (). We then analyzed whether the chimeras were capable of mediating cell-cell aggregation. Both the chimeric PTPρ/PCP-2 MAM and the PTPρ/PCP-2 Ig proteins were able to mediate cell-cell aggregation, although at a much reduced level than wild-type PTPρ (). The PTPρ/PCP-2 MAM chimera mediated 35% cell-cell aggregation and the PTPρ/PCP-2 Ig chimera mediated 21% cell-cell aggregation, compared to 69% cell-cell aggregation mediated by wild-type PTPρ (). The PTPρ/PCP-2-Extra chimera did not mediate aggregation (), indicating the defect lies in the PCP-2 extracellular domain.
Figure 7. Expression of PTPρ-PCP-2 chimeric proteins on the surface of Sf9 cells. PTPρ-PCP-2 chimera proteins were engineered (A) and expressed in Sf9 cells. Within the extracellular segment, the oval represents the MAM domain, the circle with the S-S symbol represents the Ig domain, and the solid rectangles represent the FNIII repeats. In the intracellular segment, the oval represents the juxtamembrane domain, and the aqua shaded rectangles represent the PTP domains. Total cellular protein as well as cell surface expressed proteins were isolated and immunoblotted with SK15 (B). Control expression of actin is shown for the cell lysates (B). There is no actin contamination of the cell surface, labeling samples thus serving as a control for the procedure.
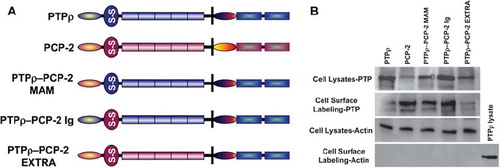
Figure 8. Swapping of either the MAM or Ig domain of PCP-2 into PTPρ creates a functional protein capable of mediating weak cell-cell aggregation. PTPρ-PCP-2 MAM and PTPρ-PCP-2 Ig chimeric proteins are both capable of mediating weak cell-cell aggregation (A), more than PCP-2 but less than wild-type PTPρ (B). PTPρ-PCP-2 Extra is not capable of mediating cell-cell aggregation.
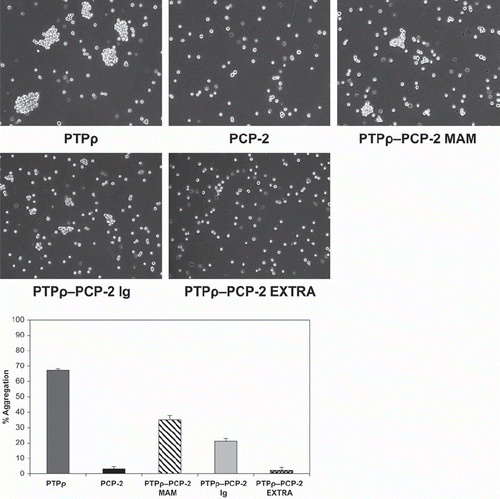
We also conducted cell-sorting experiments with the chimeric PTPρ/PCP-2 proteins and with wild-type PTPρ. PTPρ sorted away from all chimeric proteins, demonstrating that the specificity for homophilic interaction is encoded within the MAM/Ig unit. The chimeras are unique homophilic binding proteins that are not able to interact with wild-type PTPρ (). Similar results were observed in chimeric studies of PTPμ and PTPκ using lipophilic dyes (CitationZondag et al. 1995). The data suggest that together the MAM/Ig unit generates a unique binding site within the chimeric protein. The table shown in summarizes all heterophilic binding aggregation assays performed to date and demonstrates that no heterophilic binding has ever been observed in aggregation assays for the type IIb RPTPs.
Figure 9. Chimeric PTPρ-PCP-2 proteins sort away from each other. Sf9 cells coinfected with RFP and PTPρ were mixed with cells coinfected with GFP and the chimeric PCP-2 proteins and evaluated in aggregation assays for 30 min. The overlay images demonstrate that only exclusively red or green aggregates formed in these experiments, demonstrating that chimeric proteins sort away from wild-type PTPρ and create a unique recognition site. A summary table of all heterophilic binding experiments is shown at the bottom of this figure.
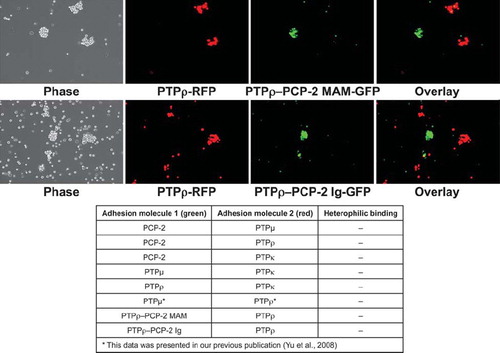
Electrostatic Calculations of Type IIb Subfamily Homology Models Depict Distinctions in Surface Charges in the MAM and Ig Domains
Based upon crystal contacts observed in the crystal structure of PTPμ, Aricescu and colleagues concluded that the adhesive interface of the PTPμ dimer was between the MAM and Ig domain of one PTPμ molecule and the first and second FNIII domains of another (CitationAricescu et al. 2007). We therefore wanted to evaluate the surface charge of exposed residues in PCP-2 and compare it to that of other type IIb RPTPs. Using the Phyre protein homology server to generate homology models, we were able to compare the surface charges of the different type IIb RPTPs (). These results demonstrate distinct differences in electrostatic surface potential, especially on the backside of the MAM-Ig domain surface, which was predicted to be important for the adhesive interface of type IIb subfamily RPTPs (CitationAricescu et al. 2007) ().
Figure 10. Representation of type IIb RPTPs using solvent accessible surface charge data. The diagram is contoured from −10 kT to 10 kT with a red (negative electrostatic potential) to white (neutral charge) to blue (positive electrostatic potential) ramping scheme. The location of the MAM and FNIII-3 domains are indicated to orient the reader. The ribbon diagram illustrates both the trans-dimer interface and the various domains described in this article. The MAM domain is shown as magenta. The Ig domain is shown in green. FNIII repeat 1 is yellow, whereas FNIII repeat 2 is blue and FNIII repeat 3 is red.
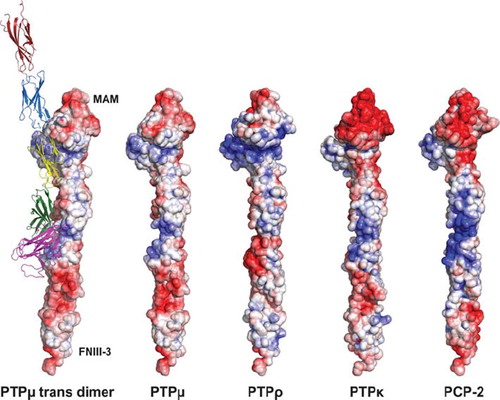
Discussion
The exact domain necessary for mediating homophilic binding in vitro and cell-cell aggregation via homophilic binding of the prototypical type IIb RPTP, PTPμ, has been a topic of study for 15 years. Homophilic binding of PTPμ-coated covaspheres requires only the Ig domain of PTPμ (CitationBrady-Kalnay and Tonks 1994). Homophilic cell-cell aggregation, on the other hand, requires the MAM domain, Ig domain, and the first two FNIII repeats of PTPμ based upon a series of studies by multiple labs (CitationAricescu et al. 2006, Citation2007; CitationBrady-Kalnay and Tonks 1994; CitationZondag et al. 1995). Furthermore, the specificity of type IIb RPTP homophilic cell-cell aggregation is determined in part by the MAM domain (CitationZondag et al. 1995). There is evidence that suggests that MAM binding is required for cis interactions between PTPμ molecules and not for trans interactions necessary to mediate cell-cell adhesion. For example, Cismasiu and colleagues demonstrated that when a mutant form of the MAM domain is expressed in cells, binding of PTPμ to the MAM domain is abolished, but cell-cell aggregation is not (CitationCismasiu et al. 2004).
The sequence identity between the PTPμ-subfamily members is approximately 55% in the extracellular domain. However, the data presented here show a large structural similarity between type IIb RPTP subfamily members, and indicates that PCP-2 likely has distinct surface charges in the vicinity previously identified as being important to mediating trans-dimer formation (CitationAricescu et al. 2007). Nevertheless, we were surprised to find that PCP-2 does not mediate cell-cell aggregation. To identify the domains of PCP-2 responsible for deficient cell-cell adhesion, we conducted domain swap experiments between PCP-2 and PTPρ. When the MAM or Ig domains of PCP-2 are swapped into otherwise wild-type PTPρ, they are able to mediate weak cell-cell aggregation, albeit to a lesser extent than wild-type PTPρ. This suggests that the MAM and Ig domains of PCP-2 can function within the context of the other functional extracellular domains of PTPρ. This study also suggests that the MAM and Ig domains each confer specificity to homophilic binding due to the fact that the PTPρ/PCP-2 MAM or PTPρ/PCP-2 Ig chimeras sorted away from wild-type PTPρ ().
Analysis of the crystal packing of PTPμ dimers suggests that 17 residues are likely important in mediating the adhesive interface between two PTPμ molecules (as shown in and in CitationAricescu et al. 2007). Mutation of a selection of six of these residues abolished cell-cell adhesion (CitationAricescu et al., 2007, Citation2008). When all of the residues of the dimer interface are compared to the residues of PCP-2, two differences are observed: Gly179 in the MAM domain is a serine in PCP-2; and Trp299 in the first FNIII repeat is an isoleucine residue in PCP-2 (numbering of amino acids begins with the signal sequence). Notably, Trp299 was shown to be absolutely essential for mediating cell-cell adhesion in aggregation assays (CitationAricescu et al. 2007). This suggests that if the hypothesized trans-dimer interface is as predicted, this amino acid change may be sufficient to render PCP-2 unable to mediate cell-cell adhesion. Furthermore, structural comparison of the MAM domain of PTPμ with the ligand-binding domains of EphB2 receptor and laminarinase highlighted two regions, termed L1 and L2, that are also potentially part of the dimer interface (CitationAricescu et al. 2006). In the L2 loop, two residues required for mediating cell-cell aggregation differ between PTPμ and PCP-2: Pro57 is a tyrosine and Pro61 is a glutamic acid residue in PCP-2 (CitationAricescu et al. 2006). None of these changes are likely to be the entire answer, however, as these residues are not necessarily conserved in PTPκ or PTPρ, either. For example, PTPκ has a serine at residue 179, and a leucine at residue 299 yet it is still able to mediate cell-cell aggregation.
Previously, a soluble PCP-2 construct containing the MAM, Ig, and first FNIII repeat was shown to mediate bead aggregation in vitro (CitationCheng et al. 1997). Our study demonstrates that wild-type PCP-2 cannot mediate cell-cell aggregation. As mentioned earlier, the Ig domain of PTPμ alone is sufficient to mediate in vitro bead aggregation (CitationBrady-Kalnay and Tonks 1994). Three amino acid residues within the Ig domain of PTPμ were proposed as important in mediating this adhesive interface: Phe188, Arg239, and Arg240 (CitationAricescu et al. 2007). These three residues are conserved in PCP-2. Furthermore, our domain-swapping experiments demonstrate that the MAM and Ig domains of PCP-2 are both capable of some adhesive function when expressed with functional FNIII domains of PTPρ. Therefore, we hypothesize that PCP-2, like PTPμ, is able to mediate bead aggregation in large part due to its Ig domain. Future studies will aim to identify which residues in PCP-2 render it unable to mediate cell-cell aggregation while still able to mediate in vitro bead binding.
PCP-2 is predicted to be a tumor suppressor gene based upon its reduced expression in melanomas (CitationMcArdle et al. 2001). PCP-2 regulates the cadherin-catenin complex by interacting with and dephosphorylating β-catenin, thereby promoting E-cadherin–mediated adhesion (CitationYan et al. 2002, Citation2006). Because of its inability to mediate cellular adhesion itself, PCP-2 likely functions as a tumor suppressor gene via regulation of tyrosine phosphorylation, potentially of the cadherin-catenin complex.
The high degree of sequence identity in the cytoplasmic domain (68%) between the type IIb RPTPs was predictive in determining the cross-reactivity of the PTPμ cytoplasmic domain antibodies. This is a necessary study, as the antibodies we generated are now commercially available from companies such as Santa Cruz Biotechnologies (SK10 ad SK15) and Calbiochem (SK15), and are advertised as PTPμ- specific. Although SK6, SK10, and the C-terminal PTPμ antibody are specific to PTPμ, the other cytoplasmic antibodies cross-react with other subfamily members. Future studies utilizing these antibodies will need to take this cross-reactivity into consideration.
In summary, the data presented in this paper supports earlier observations that type IIb RPTPs function in distinct manners to regulate cell adhesion. Studies such as this are important to understand how RPTPs function in cells to gain insight into how alterations in RPTPs function in disease states, such as during tumor progression.
Declaration of interest: The authors report no conflicts of interest. The authors alone are responsible for the content and writing of the paper.
REFERENCES
- Aricescu AR, Hon WC, Siebold C, Lu W, van der Merwe PA, Jones EY (2006). Molecular analysis of receptor protein tyrosine phosphatase mu-mediated cell adhesion. EMBO J. 25: 701–712.
- Aricescu AR, Siebold C, Choudhuri K, Chang VT, Lu W, Davis SJ, van der Merwe PA, Jones EY (2007). Structure of a tyrosine phosphatase adhesive interaction reveals a spacer-clamp mechanism. Science 317: 1217–1220.
- Aricescu AR, Siebold C, Jones EY (2008). Receptor protein tyrosine phosphatase mu: measuring where to stick. Biochem Soc Trans 36: 167–72.
- Brady-Kalnay, S. (1998). Ig Superfamily phosphatases. In Ig superfamily molecules in the nervous system. Vol. 6. P. Sonderegger. Harwood Academic Publishers, Amsterdam, Netherlands, 133–159.
- Brady-Kalnay SM (2001). Protein tyrosine phosphatases. In Cell Adhesion: Frontiers in Molecular Biology, Beckerle M. Oxford University Press, Oxford, UK, 217–258.
- Brady-Kalnay SM, Flint AJ, Tonks NK (1993). Homophilic binding of PTP mu, a receptor-type protein tyrosine phosphatase, can mediate cell-cell aggregation. J Cell Biol 122: 961–972.
- Brady-Kalnay SM, Tonks NK (1994). Identification of the homophilic binding site of the receptor protein tyrosine phosphatase PTP mu. J Biol Chem 269: 28472–28477.
- Campbell RE, Tour O, Palmer AE, Steinbach PA, Baird GS, Zacharias DA, Tsien RY (2002). A monomeric red fluorescent protein. Proc Natl Acad Sci U S A 99: 7877–7882.
- Cheng J, Wu K, Armanini M, O'Rourke N, Dowbenko D, Lasky LA (1997). A novel protein-tyrosine phosphatase related to the homotypically adhering κ and μ receptors. J Biol Chem. 272: 7264–7277.
- Cismasiu VB, Denes SA, Reilander H, Michel H, Szedlacsek SE (2004). The MAM (meprin/A5-protein/PTPmu) domain is a homophilic binding site promoting the lateral dimerization of receptor-like protein-tyrosine phosphatase mu. J Biol Chem 279: 26922–26931.
- Del Vecchio RL, Tonks NK (2005). The conserved immunoglobulin domain controls the subcellular localization of the homophilic adhesion receptor protein-tyrosine phosphatase mu. J Biol Chem 280: 1603–1612.
- Duguay D, Foty RA, Steinberg MS (2003). Cadherin-mediated cell adhesion and tissue segregation: qualitative and quantitative determinants. Dev Biol 253: 309–323.
- Gebbink MF, van Etten I, Hateboer G, Suijkerbuijk R, Beijersbergen RL, Geurts van Kessel A, Moolenaar WH (1991). Cloning, expression and chromosomal localization of a new putative receptor-like protein tyrosine phosphatase. FEBS Lett 290: 123–130.
- Gebbink MF, Zondag GC, Wubbolts RW, Beijersbergen RL, van Etten I, Moolenaar WH (1993). Cell-cell adhesion mediated by a receptor-like protein tyrosine phosphatase. J Biol Chem 268: 16101–16104.
- Higuchi R, Krummel B, Saiki RK (1988). A general method of in vitro preparation and specific mutagenesis of DNA fragments: study of protein and DNA interactions. Nucleic Acids Res 16: 7351–7367.
- Lilien J, Balsamlo, J (2005). The regulation of cadherin-mediated adhesion by tyrosine phosphorylation. Curr Opin Cell Biol 17: 459–465.
- McArdle L, Rafferty M, Maelandsmo GM, Bergin O, Farr CJ, Dervan PA, O'Loughlin S, Herlyn M, Easty DJ (2001). Protein tyrosine phosphatase genes downregulated in melanoma. J Invest Dermatol 117: 1255–1260.
- Niessen CM, Gumbiner BM (2002). Cadherin-mediated cell sorting not determined by binding or adhesion specificity. J Cell Biol 156: 389–399.
- Ostman A, Hellberg C, Bohmer FD (2006). Protein-tyrosine phosphatases and cancer. Nat Rev Cancer 6: 307–320.
- Phillips-Mason PJ, Gates TJ, Major DL, Sacks DB, Brady-Kalnay SM (2006). The receptor protein-tyrosine phosphatase PTPμ interacts with IQGAP1. J Biol Chem 281: 4903–4910.
- Phillips-Mason PJ, Mourton T, Major DL, Brady-Kalnay SM (2008). BCCIP associates with the receptor protein tyrosine phosphatase PTPmu. J Cell Biochem 105: 1059–1072.
- Sallee JL, Wittchen ES, Burridge K (2006). Regulation of cell adhesion by protein-tyrosine phosphatases: II. Cell-cell adhesion. J Biol Chem 281: 16189–16192.
- Sap J, Jiang YP, Friedlander D, Grumet M, Schlessinger J (1994a). Receptor tyrosine phosphatase R-PTP-κ mediates homophilic binding. Mol Cell Biol. 14: 1–9.
- Wang H, Lian Z, Lerch MM, Chen Z, Xie W, Ullrich A (1996). Characterization of PCP-2, a novel receptor protein tyrosine phosphatase of the MAM domain family. Oncogene 12: 2555–2562.
- Yan HX, He YQ, Dong H, Zhang P, Zeng JZ, Cao HF, Wu MC, Wang HY (2002). Physical and functional interaction between receptor-like protein tyrosine phosphatase PCP-2 and beta-catenin. Biochemistry 41: 15854–15860.
- Yan HX, Yang W, Zhang R, Chen L, Tang L, Zhai B, Liu SQ, Cao HF, Man XB, Wu HP . (2006). Protein-tyrosine phosphatase PCP-2 inhibits beta-catenin signaling and increases E-cadherin-dependent cell adhesion. J Biol Chem 281: 15423–15433.
- Yu J, Becka S, Zhang P, Zhang X, Brady-Kalnay SM, Wang Z (2008). Tumor-derived extracellular mutations of PTPRT/PTPrho are defective in cell adhesion. Mol Cancer Res 6: 1106–1113.
- Zhang P, Becka S, Craig S, Lodowski D, Brady-Kalnay S and Wang Z. (2009). Cancer-derived mutations in the fibronectin III repeats of PTPRT/PTP inhibit cell-cell aggregation. Cell Communication and Adhesion 16:146–53.
- Zondag GC, Koningstein GM, Jiang YP, Sap J, Moolenaar WH, Gebbink MF (1995). Homophilic interactions mediated by receptor tyrosine phosphatases mu and kappa. A critical role for the novel extracellular MAM domain. J Biol Chem 270: 14247–14250.