Abstract
Natural Killer (NK)-cells are peripheral blood lymphocytes that represent an important arm of the innate immune system. NK-cells play a critical role in the immune surveillance against tumors and virally infected cells in a major histocompatibiliy complex (MHC)-unrestricted fashion. We have explored such capacities of NK-cells after differentiation from hematopoietic stem and progenitor cells derived from human umbilical cord blood. Several culture conditions have been established supporting proliferation and subsequent differentiation of these cells in terms of receptor expression and specific lysis depending on the growth conditions in the presence and absence of supportive stromal feeders. We show that acquisition of Killer Immunoglobulin Receptor (KIR) as well as NK Cytotoxicity Receptor expressions is independent of culture condition whereas absence of stromal feeders did not support acquisition of CD94/NKG2A expression. Such KIR-positive/NKG2A-negative cells generated under different culture conditions showed strong and specific cytolytic activity which could have impact on further immunotherapeutic strategies.
Introduction
Natural Killer (NK)-cells, originally described as non-T, non-B cells, are large granular lymphocytes that were identified in mice by the innate capacity to rapidly lyse some tumor cells. Unlike T-cells, they can kill targets without prior sensitization and exhibit spontaneous cytotoxicity activity towards cells that do not express class I molecules of the major histocompatibility complex (MHC) (CitationBorrego et al., 2002; CitationOrange & Ballas, 2006). NK cells express a large variety of activating receptors on their surface capable of recognizing target cells (CitationMoretta et al., 2002). They also express inhibitory receptors whose signals are able to override basal activation signals (CitationHallett & Murphy, 2006; CitationLanier, 2008). The ligands for the predominant inhibitory receptors are major histocompatibility complex (MHC) class I molecules that are expressed by most normal cells. As originally proposed by the “missing self” hypothesis, down-regulation by tumor transformation or infection can make cells susceptible to NK lysis due to partial or complete loss of class-I MHC expression (CitationGarcia-Lora, Algarra & Garrido, 2003; CitationOrange, Fassett, Koopma, Boyson, & Strominger, 2002). These cell modifications are perceived by NK-cells and, in that event, the normal balance between activating and inhibitory signals provided by diverse receptors is compromised and can make cells susceptible to a NK-cells-response. In humans, the predominant NK inhibitory receptors interacting with MHC class I molecules are the heterodimeric CD94/NKG2A, the killer immunoglobulin-like receptors (KIRs) and the leukocyte immunoglobulin-like receptors (LIRs or ILTs). While the ligands for the type I transmembrane glycoproteins KIR and LIRs are mainly the classical human leukocyte antigen (HLA) class I molecules (HLA-A, B and C), the ligand for NKG2A is the nonclassical class I molecule HLA-E (CitationBorrego, Ulbrecht, Weiss, Colugan, and brooks, 1998; CitationBrooks, Posch, Scorzelli, Borrego, & Coligan, 1997).
NK cells are characterized by the absence of conventional antigen receptors, and are phenotypically identified by the presence of CD56 and absence of CD3 surface antigens (CitationRobertson and Ritz, 1990). The majority of human NK cells (≈90%) have low density expression of CD56 (CD56dim) whereas ≈10% of NK cells are CD56bright (CitationCooper, Fehniger, & Caligiuri, 2001). While CD56bright NK cells appear to have an important immunoregulatory role, they are less effective mediators of antibody-dependent cellular cytotoxicity (ADCC) and natural cytotoxicity. CD56dim NK cells are more naturally cytotoxic against NK-sensitive targets (CitationFarag and Caligiuri, 2006). In spite of that, CD56bright and CD56dim NK cells show similar levels of cytotoxicity when treated with IL-2 both in vitro and in vivo (CitationCaligiuri et al., 1990; CitationNagler, Lanier, & Phillips, 1990; CitationRobertson et al., 1992). Although there is no direct functional significance of high- or low-level expression of CD56 (CitationLanier, Testi, Bindl, & Phillips , 1989), Cooper and colleagues reviewed a number of other cell-surface markers that confer unique phenotypic and functional properties to CD56bright and CD56dim subsets (CitationCooper et al., 2001).
Several studies have been engineered to enhance NK-cell activity, however the molecular mechanisms and regulation of the NK cell alloreactivity in humans is yet largely unknown and the repertoire of the receptors that are involved in alloreactivity (KIRs) is quite variable. In fact, in vitro differentiation of NK cells from CD34+ hUCB progenitors has been already studied in vitro under different conditions (CitationCarayol et al., 1998; CitationGrzywacz et al., 2006; CitationKao et al., 2007; CitationLewis & Verfaillie, 2000; CitationMiller & McCullar, 2001). However, these experiments have generated conflicting results. Feeder layers were either shown to have no beneficial effects simultaneously on the NK cell differentiation, expansion and maturation (CitationLewis & Verfaillie, 2000), to increase CD56+ expansion but not maturation (CitationCarayol et al., 1998), or to promote CD56+ expansion and maturation (CitationGrzywacz et al., 2006, CitationMiller and McCullar, 2001). Overall, the possibility to generate/differentiate NK-cells from CD34+, with and without stroma was already demonstrated (CitationPapamichail, Perez, Gritzapis, & Baxevanis, 2004). However, the possibility to generate NK-cells from UCB without stroma/adhesive microenvironmental factors for a possible clinical application in terms of adoptive cellular therapy, was never been demonstrated so far.
We attempted to recapitulate the development of functional NK-cells from multipotent hematopoietic UCB-stem cells in vitro reconstituting the complete process of NK-cell development and maturation from HSPC to functionally competent effector cells. We also investigate the possibility to generate a specific NK cell population (CD56+ KIR+ NKG2A-) from UCB without stroma/ adhesive microenvironmental factors.
In summary, multipotent hematopoietic stem and progenitor cells were retrieved from human umbilical cord blood (hUCB) samples and induced to proliferate and mature in vitro into NK cells, with determination of the temporal expression of the specific surface receptors and analysis of the functional effector characteristics of NK cells. To analyze the role of the niche created by support cells in the transition of primitive stem cells to less primitive cells, the established AFT024 cell line was used in the in vitro culture system (CitationMoore, Emma, & Lemischka, 1997) and compared to conditioned medium and standard medium enriched with specific growth factors and interleukins.
Methods
Cell samples
In accordance to guidelines of the local Ethic Committee, human umbilical cord blood (hUCB) was harvested by umbilical vein puncture after normal full-term deliveries under previous informed consent from pregnant woman before delivery. Samples were collected in 150 cc sterile bags, containing 21 ml of citrate-phosphate-dextrose (CPD) (MacoPharma, Tourcoing, France), and kept at room temperature for less than 12 h. In total, 18 hUCB were included, with at least 5 hUCBs being used in each experiment.
Isolation of mononuclear cells
Mononuclear cells (MNCs) from a total of 18 hUCBs were isolated using 1.077 g/ml Ficoll-Histopaque (Sigma, St. Louis, MO, USA) by gradient centrifugation (400 g, 30 min, 20°C). Low density MNCs were collected, washed twice (1600 rpm, 6 min, 4°C) and resuspended in Dulbecco's phosphate buffered saline (DPBS; Sigma), supplemented with 0.5% of fetal bovine serum (FBS; HyClone Laboratories, Logan, Utah, USA) and 2 mM of ethylene diamine tetraacetic acid (EDTA; Sigma). When necessary, lysis of residual red cells was performed with ice-cold lysis buffer consisting of 8.29 g NH4Cl, 1 g KHCO3 (Merck, Darmstadt, Germany; Sigma) and 0.2 ml of 0.5 M EDTA in 1 L H2O. After 15 min, cells were washed twice and resuspended in DPBS supplemented with 0.5% of FBS and 2 mM of EDTA.
Selection of CD34+ cells
The CD34+ cell fraction from MNCs was immunomagnetically selected using a Magnetic Activated Cell Selection (MACS) system (Miltenyi Biotec, Auburn, CA, USA). The Progenitor Cell Isolation Kit uses antibodies recognizing the CD34 epitope QBEND/10 and was performed according to manufacturer's instructions with minor alterations. Briefly, MNCs were incubated with 75 μl MACS microbeads CD34+ /108 total cells. Cell clumps were removed by passing the cells through a pre-separation Filter and labeled cells were separated using a high gradient magnetic separation column in a strong magnetic field. Magnetically retained cells were released by flushing down the column with a plunger and thereafter eluted into a 15 ml Falcon tube. Cell aliquots were taken for cell viability using Trypan blue exclusion method (Sigma) and an Improved Neubauer Haemocytometer for count. The purity of the CD34+ fraction was determined by flow cytometry, being consistently above 90%.
AFT024 feeder layers
AFT024 cells, a stromal cell line derived from murine fetal liver (Heinrich Heine University, Duesseldorf, Germany), were used as feeder layers. Cells were cultured in 75 cc flasks with Dulbecco's modified Eagles medium (DMEM) containing 1000 mg glucose/L (Gibco, Paisley, Scotland, UK), and supplemented with 20 % FBS, 100 U/ml Penicilin and 100 μl/ml Streptomicin (Pen/Strep; Gibco), 2 mM L-Glutamin (Gibco) and 24 μM β-mercaptoethanol (Gibco). Cells were cultured for one week at 33°C with 5 % CO2 in humidified air. When a confluent layer formed, cells were recovered by digestion (5 min, 37°C) with 10 ml of a trypsin/EDTA solution (trypsin/EDTA; Gibco). To increase cell number, cells were cultured again in 2, 3 or 4 new 75 cc-culture flasks until confluency. AFT024 cells were then plated in 24-well plates (105 cells/1 ml/well). When confluent, cells were uniformly irradiated (2000 rad) using a cobalt irradiator (Theratron 780C; Atomic Energy of Canada Ltd., Chalk River, Ontario, Canada). After irradiation, plates were incubated at 37°C and the culture medium changed the day after.
Cell culture
CD34+ cells were used in a two-step protocol, consisting of 1 week of proliferation and 3 weeks of differentiation. Cells were plated at different concentrations (4 × 103 cells/well, 2 × 103 cells/well, 1 × 103 cells/well and 0.1 × 103 cells/well) and incubated in three different conditions: 12 samples with supplemented medium+ AFT024 feeder layers (AFT), 5 samples with supplemented medium (C), and 5 samples with supplemented medium conditioned from AFT024 (CM). Cells were cultured (5% CO2 in air at 37°C) with the medium being changed once a week. In the first week, the medium consisted of a 2:1 proportion of DMEM containing 4500 mg glucose/L (Sigma) and HAMs F12 (Biochrom AG, Berlin, Germany), supplemented with 20 % of heat inactivated human AB serum (BioWittaker, Walkersville, USA). This was then supplemented with Pen/Strep (100 IU/100 μl/ml), β-mercaptoethanol (24 μM), ascorbic acid (20 mg/L), selenium selenate (50 μ/L), ethanolamine (50 μM) (Sigma), L-Glutamine (2 mM) (Gibco) and a cocktail of recombinant human cytokines, 1000 IU/ml IL-2, 5 mg/ml IL-3, 20 mg/ml IL-7, 20 mg/ml stem cell factor (SCF) and 10 mg/ml Flt3-L (PeproTech, London, UK). At the end of the first week, the medium was changed and the cells were cultured with a 2:1 proportion of DMEM containing 4500 mg glucose/L and HAMs F12, supplemented with 10 % of heat inactivated human AB serum, and the cocktail of cytokines described above, with the exception of IL-3 that was replaced by IL-15 (10 mg/ml) and the addition of IL-21 (10 mg/ml) (PeproTech).
Cell phenotyping
Fluorescein isothiocyanate (FITC)-conjugated, phycoerythrin (PE)-conjugated and phycoerythrin-Cy5 (PE-Cy5)-conjugated monoclonal antibodies (MoAbs) were used for the analysis of controls and specific cell surface markers. MoAbs anti-mouse IgG1 (clone 679.1Mc7) -FITC, anti-mouse IgG2a (clone 7T4-1F5)-PE, anti-CD34 (clone 581)-FITC, anti-CD56 (clone N901)-PECy5, anti-CD158a (clone EB6B)-PE, anti-p50.3 (clone FES172)-PE, anti-NKp30 (clone Z25)-PE, anti-NKp44 (clone Z231)-PE, anti-NKp46 (clone BAB281)-PE and anti-NKG2a (clone Z199)-PE were purchased from Beckman Coulter (Immunotech, Marseille, France). MoAbs anti-mouse IgG1 (clone MOPC-21)-FITC/PE/PECy5, anti-CD45 (clone HI30)-FITC/PE/PECy5, anti-CD94 (clone HP-3D9)-FITC, anti-CD3 (clone UCHT1)-FITC, anti-NKB1 (clone DX9)-PE and anti-KIR-NKAT2 (clone DX27)-PE were acquired from Beckton & Dickinson (BD PharMingen, San Diego, CA). Once a week, a sample of the cultured cells was harvested for immunophenotyping. Briefly, cells were resuspended and collected in a 15 cc Falcon tube, washed in DPBS supplemented with 0.5 % FBS and 2 mM EDTA, and divided into aliquots to evaluate defined surface markers and stain cells with isotype-matched antibodies ensuring the sample control. All aliquots were incubated with 5 l of MoAbs per 5x105 cells for 30 min in dark conditions at 4°C, and washed twice in DPBS supplemented with 0.5 % FBS and 2 mM EDTA, according to the manufacturer's instructions. Flow cytometry was performed in a FACScalibur apparatus equipped with Cell Quest Pro software, and data were analyzed with the Paint-a-Gate software (Becton Dickinson, Oxford, UK). Each sample was analyzed at least for 20000 events. Results are presented as the percentage of cells in a selected lymphocyte region.
Cytotoxicity tests
After 4 weeks, CD56+ cells were purified from cultures (C, AFT) (n = 5) by MACS and used as effector cells. K562 cells, a human erythroleukemia cell line (ICLC HTL94001; Interlab Cell Line Collection, Genova, Italy), were used as target cells. Cytotoxic activity was assessed using a non-radioactive cytotoxicity assay (CytoTox 96; Promega, Madison, WI, USA) according to the manufacturer's instructions. Briefly, effector cells were incubated with 1 × 104 K562 cells and 4 replicates were performed for each experiment in 96-well round bottom plates at different effector:target rates, 10:1, 5:1, 2.5:1, 1.25:1, 0.63:1, 0.31:1 and 0.16:1. Plates were maintained for 4 h in a humidified chamber at 37°C, 5 % CO2, and cytotoxicity was determined by lactacte dehydrogenase (LDH) release from target cells. Culture medium or 0.8 % Triton X-100 (CytoTox 96) was added to target cells for calculation of spontaneous (S) and maximum release, respectively. Supernatants were collected and LDH was assayed using an ELISA plate reader (Stat Fax 2100 Microplate Reader; Awareness Technology, Inc., Palm City, FL, USA). Cytotoxicity was calculated using the following formula:
% Cytotoxicity = [Experimental – (Effector Spontaneous + Target Spontaneous)/Target maximum - Target Spontaneous] × 100.
Statistics
Results of experimental points from different experiments were reported as the mean ± standard deviation (SD). Significance levels for cytometry results were determinate by two-sided Pearson Chi-Square analysis and statistical significance for cytotoxicity values was tested by two-sided Student's t-test analysis.
Results
Proliferation
Positively selected CD34+ cells () were cultured in a defined medium containing IL-2, IL-3, IL-7, SCF and Flt3-L (C) (n = 5), C+ AFT024 feeder layers (AFT024) (n = 12) () and C+ AFT024 conditioned medium (CM) (n = 5). Cobblestone-like areas became visible at the end of the first week of culture in all three culture conditions, suggesting an effective proliferation of the original CD34+ cells (). Quantitatively, the percentage of CD34+ cells was significantly higher (p < 0.001) in AFT (26.89 ± 12.46%) than in CM (11.76 ± 5.96%) or C (13.16% ± 6.79%), and in C in relation to CM, which suggests a positive effect of direct cell contacts but not of a factor released by the feeder layers. As expected, in the subsequent three weeks of culture using a modified mixture directed to NK cell differentiation (IL-2, IL-7, IL-15, IL-21, SCF and Flt3-L), the pool of CD34+ cells progressively decreased (0.01 ± 0.02% in AFT, 0.15 ± 0.30 % in CM and 0.00 ± 0.00 in C) (; ). In this period, the same type of significance was observed between the three culture conditions (p < 0.001), with exception of day 28, where no differences were found between AFT and C (p = 0.374).
Figure 1. Establishment of hUCB CD34+ cell co-cultures with AFT024 (a-c), proliferation of progenitor cells (d) and phenotypic behavior along the 4 week period (e). a) CD34+ cells from hUCB after MACS enrichment; b) monolayer of stroma cells after irradiation (day 0); c) CD34+ enriched cells over stroma AFT024 (day 0); d) Cobblestone-like areas over feeder layer (day 7); e) percentage of CD34+ cells obtained by flow cytometry after each week of culture; p < 0.001.
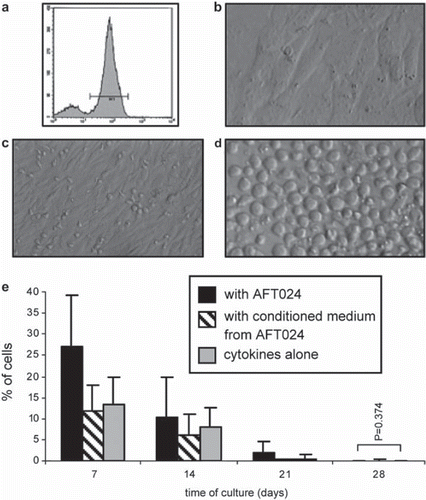
Table 1. Percentage of CD56+ CD3− and proportion of CD56+ CD3−KIR+ , CD56+ CD3−NCR+ and CD56+ CD3−NKG2A/CD94− cells generated by CD34+ cells grown in the described three conditions. Kinetics of expression along the time can be better visualized in .
Differentiation
During the same differentiation period, the relative numbers of NK cells (CD56+ CD3-) progressively increased as CD34+ cells decreased (, ). Generally, this increase was significantly higher (p < 0.001) in controls up to day 21, whereas on day 28, it was significantly higher (p < 0.001) in AFT (81.38 ± 13.43%) and CM (83.89 ± 10.58%) in relation to C (68.69 ± 19.52%). Specifically, the significant differences were found between all culture conditions in all weeks of the experiment period (p < 0.001). Two exceptions with no significant differences were found between C and CM at day 7 (p = 0.466) and between AFT024 and CM at day 28 (; ). This data thus suggest that NK cell differentiation is first delayed by contact with feeder layers, but then stimulated after 14 days of culture by some factor(s) released from the stroma.
Figure 2. Phenotype of in vitro cultured hUCB CD34+ cells. Flow cytometry analysis after 7 (a) and 28 (b) days of culture. Note that the CD34+ cells almost become extinct between day 7 (d7) and day 28 (d28) with the simultaneous appearance of CD56+ phenotype. Differentiation areas can be seen in c) at day 14 (d14) of culture. Detailed data is shown in .
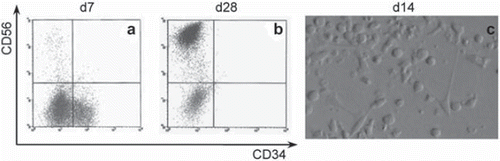
Figure 3. Flow cytometry analysis of differentiated hUCB CD34+ cells at the end of the experimental period. Cells were analysed by flow cytometry and positivity for the different mAbs was defined in the morphological gate represented in a). NK cells population is shown in b) and the expression several receptors are shown in c) for the KIRmix, in d) for the NCRmix and in e) for NKG2A.
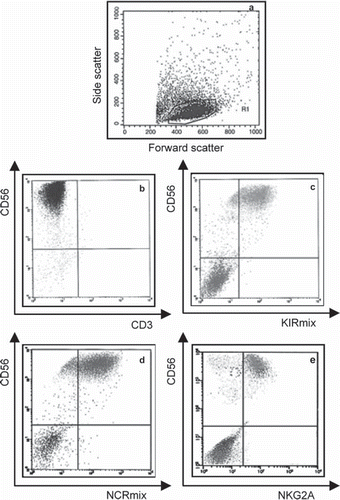
Figure 4. hUCB CD34+ cells differentiation into NK cells along the 4-week period. Cells were analysed by flow cytometry and positivity for the different mAbs was defined in the morphological lymphoid gate defined in . Results from a) and b) were additionally defined in the CD56+ CD3+ gated region (see ) and results from d) were also defined in the CD56+ CD94+ gated region (data not showed). The graphics show the CD56+ CD3+ cells generated in vitro from CD34+ progenitors (a) and the kinetics of expression of KIRs (b), NKps (c) and CD94/NKG2A- (d) (p < 0.001). See for detailed data.
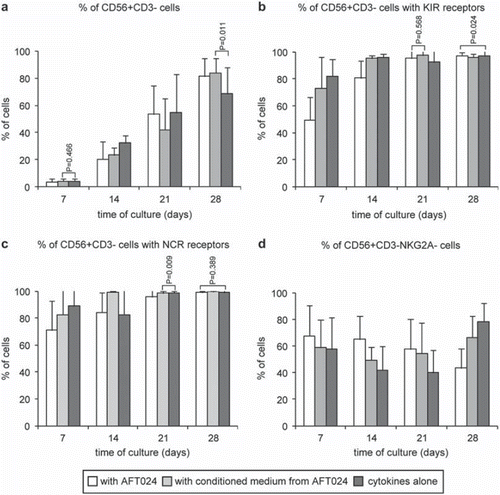
KIR acquisition
Analysis of surface receptors during NK cell maturation revealed the expression of the most important KIRs (NKAT2, NKB1, CD158a, p50.3) and the known Natural Killer Cytotoxicity Receptors, NCRs (NKp30, NKp40, NKp46). Acquisition of at least one KIR was found in almost all CD56+ CD3- cells (>95%) at the end of the culture period, with data suggesting that KIRs expression is initially delayed due to direct cell contacts with feeder layers, followed by a stronger stimulation under these conditions. Generally, the presence of KIRs was detected very early during cultures, being significantly higher (p < 0.001) in C than in CM or AFT, both at day 7 (81.97 ± 12.36% in C, 72.97 ± 23.42% in CM and 49.51 ± 17.02% in AFT) and day 14 (96.12 ± 2.27% in C, 95.19 ± 2.09% in CM and 80.65 ± 12.91% in AFT), thereafter becoming significantly higher (p < 0.001) in AFT (95.15 ± 6.46% at day 21 and 97.15 ± 2.32% at day 28) and CM (97.59 ± 2.90% at day 21 and 95.98 ± 2.54% at day 28) in relation to C 92.43 ± 10.32% at day 21 and 96.90 ± 4.15% at day 28). Specifically, shows detailed data of expression of this receptors with significant differences between all culture conditions in all weeks of the experimental period (p < 0.001) with exception for day 21 and day 28 where no significant differences were found between AFT024 and CM (p = 0.568) and between AFT024 and C, respectively ( & ).
NCR acquisition
The majority of the cells (>50%) also expressed at least one NCR very early during cultures (day 7) (89.03 ± 13.10% in C, 82.37 ± 24.91% in CM and 71.01 ± 21.58% in AFT), and most of the CD56+ CD3− cells had these receptors at the end of the 4 week maturation period (>98%) (99.01 ± 1.65% in C, 99.45 ± 0.47% in CM and 98.89 ± 1.11% in AFT), with data suggesting that NK cell differentiation is first delayed by contact with feeder layers and then stimulated by some factor(s) released from the stroma. The mean percentage of NCR is specified in where all cultures present significant differences (p < 0.001) with exception for day 21 and day 28 where no differences were seen between C and CM and between AFT024 and C (p = 0.389), respectively ( & ).
CD94/NKG2A acquisition
CD94/NKG2A was the less acquired surface receptor: its expression decreased along all period of culture in presence of AFT024 (67.60 ± 22.89% at day 7 to 43.35 ± 14.49% at day 28). In the absence of stroma, its expression decreased until 2nd week with conditioned medium (58.80 ± 20.64% at day 7 to 49.39 ± 10.03% at day 14) and until 3rd week with control medium (57.58 ± 23.92% at day 7 to 40.12 ± 17.03% at day 21), starting to increase from there. Minimal expression was achieved at the end of 3rd week in absence of any factors released from the stroma. Specifically, the mean percentage of CD94/NKG2A expression is shown in where all cultures have significant differences between them (p < 0.001).
Cytotoxicity
To evaluate the functionality of the in vitro derived mature NK cells, cytotoxicity assays were performed against K562 target cells with CD56+ CD3− effector cells derived from AFT and C day 28 cultures (). Significant higher cytotoxicity levels were found for cells from AFT at the different E:T ratios tested, 70.7% (C) and 90.6% (AFT) at 10:1, 59.6% vs. 70.2% at 5:1, 32.7% vs. 50.4% at 2.5:1, 18.5% vs. 27.4% at 1.25:1, 9.8% vs. 16.8% at 0.62:1 (p < 0.001), 8.2% vs. 11.4% at 0.31: 1 (p = 0.004), 3.4% vs. 5.7% at 0.16:1 (p = 0.013), 3.9% vs. 5.5% at 0.08:1 (p = 0.025), with no significant differences at 0.04:1 (1.7% vs. 2.5%) and at 0.02:1 (2.0% vs. 2.9%).
Figure 5. Cytotoxicities mediated by in vitro differentiated NK cells derived from hUCB-HSCs (effector cells) against K-562 target cells. These activities were measured using a Non-Radioactive Cytotoxicity test at the effector:target (E:T) cell ratios indicated. Each data bar represents a mean SD percentage value of five separate experiments, each performed in quadruplicate (p < 0.05).
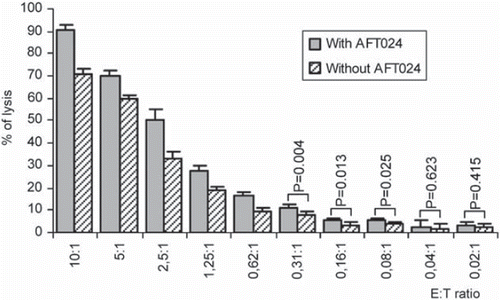
Discussion
Since it was previously thought that the addition of stromal cells was important for the expansion of NK cells, we investigated if the presence of supporting stroma or alternatively, stroma-conditioned medium, could improve the generation and subsequent functionally maturation of NK cells from undifferentiated HSPC. The murine stromal cell line AFT024 was selected for its described capacity to support and amplify the differentiation of CD34+ cells (CitationMoore et al., 1997). NK cell maturation is a dynamic and continuous process as NK cells respond differently to external signals to secrete cytokines and to destroy target cells. To define the phenotype profile and functional activity of NK cells differentiated in vitro, different cultures were performed combining cytokines with or without stroma cells or using conditioned medium released from the stroma. All culture conditions showed proliferative capacity but in spite of the cytokines included in the medium and the factors released from the stroma, it seems that the direct contact with stroma have beneficial effects on CD34+ expansion and on the capacity to avoid cell commitment. This indicates that stroma is a more potent inducer on proliferation and preservation of hUCB progenitor cells than the two other conditions. During the 4-week period, the cytometry results showed an exponential decrease of CD34+ cells with a simultaneous increase of CD56+ CD3− in all culture conditions. However, in the first weeks of culture in contact with stroma, cells yielded a lower proportion of CD56+ CD3− cells compared with the other conditions. In contact with stroma, cells maintained for longer period the immature status. This can explain the initial delay in the commitment of NK phenotype. On the other hand, our results did not reveal any major differences in the capacity of CD34+ cells collected from hUCB to generate high numbers of NK cells after 4 weeks in the presence of stroma cells or in conditioned medium from the stroma. However, in absence of stroma, there is a reduced production of the relative number of CD56+ cells meaning that in spite of IL-15 in combination with Flt3-L, SCF, IL-2, IL-7 and IL-21 be sufficient to induce human CD34+ cells to differentiate into NK cells, it seems that the addition of AFT024 cells did not qualitatively influence NK differentiation from CD34+ cells. In spite of that, Miller and colleagues verified that cytokines alone are inefficient to support NK cell differentiation and the conditioned medium only supports differentiation partially (CitationMiller & McCullar, 2001). However, we observed that the factors produced by stroma cells may improve the initial development of NK cell phenotype. Our results are supported by others that concluded that cytokines together with stromal cells clearly increase the number of generated NK cells but didn't qualitatively alter NK cell differentiation (CitationCarayol et al., 1998) and that stromal cells do not bring any beneficial effect compared to conditioned medium, once released factors and/or cytokines are sufficient to induce NK cell differentiation (CitationLewis & Verfaillie, 2000). Additionally, during the process of maturation, NK cells establish a characteristic cell-surface phenotype and the capacity to elicit effector functions. Several markers of mature NK cells were used including a number of KIRs such NKAT2, NKB1, CD158a and p50.3, NCRs such NKp30, NKp40 and NKp46 and the inhibitory receptor CD95/NKG2A. The maturity of NK cells, detected by the presence of receptors, was checked and, in independent conditions, we found that the vast majority of differentiated CD56+ CD3− cells co-expressed at least one of the KIRs and NCRs. Since CD56+ phenotype was delayed in presence of stroma, the same occurred for the appearance of such receptors when in contact with stroma. Nevertheless, having in mind the results of the end of culture period, it seems that the appearance of these receptors is independent of the culture condition. The high levels of KIRs and NCRs might be consistent with a mature NK phenotype.
We know that the activation of immune responses depends on a tight balance between activating and inhibitory signals. For cell therapeutic purposes, it would be desirable to downregulate NKG2A expression in order to decrease the activation threshold of effector cells and to allow for more efficient lysis of target cells. In all our tested conditions, the complex NKG2A/CD94 is present in NK cells in spite of its expression being markedly lower than the other receptors. There are some reports of in vitro differentiated CD56+ cells that express CD94+ on its majority independently of the presence or not of stroma (CitationCarayol et al., 1998). Simultaneously, less than 5% of CD56+ cells present KIRs expressed after the CD94/NKG2A. With our system, the presence of stroma induced a continuous increase of surface expression of this complex along the time. Curiously, NKG2A/CD94 positive cells in conditions CM and C got its maximums earlier than condition AFT024. However, we also saw a strong decline since then suggesting that the lack of cells to establish contact with NK cells may diminish the stimuli to express this receptor. We thought that the lower expression of the complex CD94/NKG2A compared with that of the other receptors could be explained by the fact that maturation of NKG2A is dependent on the association with CD94 by glycosylation requiring much more metabolic complexity. Thus, it appears that there are some factors released by stroma that could be important for the first steps of induction towards NK cells lineage but acquisition of receptors seems to be independent of stroma signals. Some published data refers that NK cells receptors are acquired in an orderly and nonrandom manner during in vitro human NK cell differentiation (CitationMiller & McCullar, 2001; CitationMrozek, Anderson, & Caligiuri, 1996; CitationGrzywacz et al., 2006) and, differing from our results, indicated that the first inhibitory receptor to be surface-expressed is CD94/NKG2A while KIRs appear only later and in low percentages (CitationBriard, Brouty-Boye, Azzarone, & Jasmin, 2002; CitationIizuka et al., 1999; CitationGrzywacz et al., 2006; CitationMiller & McCullar, 2001). Cytotoxicity results were consistent with the phenotypic behavior implying that these NK cells can demonstrate specificity in the recognition process. In fact, our cytotoxicity results of in vitro differentiated NK cells against classical NK cell target, K562, was especially higher than published NK cell activities from fresh isolated NK cells of cord blood or peripheral blood. Condiotti et al. demonstrated that cytotoxic CD56+ cells could be produced from human UCB (CitationCondiotti, Zakai, Barak, and Nagler, 2001). Nevertheless the intent of their experiments was to prove the cytotoxic capabilities of NK cells in UCB, we went farther starting with CD34+ cells. Our studies showed that NK cell activity can be significantly enhanced during CD34+ differentiation in controlled conditions. Although K562 cells do not carry HLA-E, it was proposed by Figueiredo. et al. that this increased cytolytic activity is partially mediated by the natural cytotoxicity receptor NKp30 (CitationFigueiredo, Settsam, & Blasczyk, 2009) since it was recently shown that the ligand of NKp30 is expressed in K562 cells (CitationByrd, Hoffmann, Jarahian, Momburg, & Watzl, 2007). In general, the stroma was a more potent inducer for cord blood differentiation into functional NK cells than the other experimental conditions. However, in the presence of suitable cytokines and factors released from the stroma, CD34+ cells rapidly acquired large quantities of NCRs and KIR receptors, becoming cytotoxic at this level. In spite of some reports referring that stromal cells play an important role in NK cell maturation (CitationColucci, Caligiur, & Di Santo, 2003; CitationMiller & McCullar, 2001) and others reporting that CD34+ HSC cannot give rise to mature NK cells in absence of stroma (CitationLewis & Verfaillie, 2000), with our well-defined and highly reproducible culture conditions we were able to generate fully competent NK cells in vitro with or without stroma cells, demonstrating that NK cells functionality can be increased under appropriate conditions. The use of cytokines reveals itself as a remarkable tool for the production of functional mature cells. From the present findings we can argue that IL-15 in combination with Flt3-L, SCF, IL-2 and IL-21 were sufficient to induce human CD34+ cells to differentiate into mature cytotoxic NK cells in vitro. However, we cannot exclude the possibility that other factors produced by the stroma may have influenced the development of NK cells. Additionally, based on the work of others (CitationKato et al., 2007) and using specific drugs, downregulation of the NKG2A ligands expression can be attempted on targets. This could also be an attractive therapeutic strategy to induce susceptibility of leukemic cells to the cytotoxicity of NKG2A-lacking cells.
Conclusions
These results represent strong indications that we are in presence of mature NK cells generated in vitro and it seems that these receptors are indispensable for NK cell maturity. In fact, the capacity of hUCB CD34+ cells to acquire CD56 may therefore provide a cytotoxic cell population that may be of therapeutic potential in the treatment of hematopoietic malignancy. These data together with the possibility to achieve NK cells negative for CD94/NKG2A receptor will allow us to generate alloreactive NK-cells for clinical applications in adoptive cellular therapies. This study also extended our knowledge of the effects of different cytokines on the proliferation of NK cells from human cord blood progenitor cells. One of the reasons for the lower incidence of GVHD following UCB transplant may be the reduced cytotoxic potential of CB-derived NK cells. However, we can increase NK cells functionality under appropriate conditions. The reactivity of NK cells results from an integration of activating and inhibitory stimuli which are transmitted through their receptors. The capacity to manipulate this balance on NK cells may open new perspectives in immunotherapy. Thus, this balance can be altered in favor of activation by the induction of activating receptor expression on NK cells through the use of the correct cocktail of cytokines. This matter is not yet well understood and there are only a few preliminary studies in this field with no conclusive results. In fact, there are many reports with mixed conclusions difficult to reconcile. An improved understanding of these processes will continue to drive the clinical applications for NK cells as a tool against infection and tumors as well as the establishment of NK cell lines with “specific” characteristics will provide favorable tools for culture models.
Acknowledgements
We would like to thank the Gynecology and Obstetrics staff of Júlio Dinis Maternity, Porto, Portugal, for human umbilical cord blood collection, the North Regional Division of the Portuguese Institute of Blood, for supplying blood collection bags, the Department of Immunology, St John Hospital, for technical support in cytometry experiments, and the Department of Radiotherapy, St John Hospital, Porto, Portugal, for technical support in radiation experiments. We would like to thank Joana Marques (PhD) for her friendship, encouragement and kindness to check the use of English in the manuscript. This work was partially financially supported by FCT (POCTI/SAU-MMO/60709/04, 60555/4, 59997/04, UMIB)
Declaration of Interest: The authors report no conflicts of interest.
References
- Borrego F, Kabat J, Kim DK, Lieto L, Maasho K, Pena J, Solana R, Coligan JE (2002). Structure and function of major histocompatibility complex (MHC) class I specific receptors expressed on human natural killer (NK) cells. Mol Immunol. 38: 637–60.
- Borrego F, Ulbrecht M, Weiss EH, Coligan JE, Brooks AG (1998). Recognition of human histocompatibility leukocyte antigen (HLA)-E complexed with HLA class I signal sequence-derived peptides by CD94/NKG2 confers protection from natural killer cell-mediated lysis. J Exp Med. 187: 813–818.
- Briard D, Brouty-Boye D, Azzarone B, Jasmin C (2002). Fibroblasts from human spleen regulate NK cell differentiation from blood CD34(+) progenitors via cell surface IL-15. J Immunol. 168: 4326–4332.
- Brooks AG, Posch PE, Scorzelli CJ, Borrego F, Coligan JE (1997). NKG2A complexed with CD94 defines a novel inhibitory natural killer cell receptor. J Exp Med. 185: 795–800.
- Byrd A, Hoffmann SC, Jarahian M, Momburg F, Watzl C (2007). Expression analysis of the ligands for the Natural Killer cell receptors NKp30 and NKp44. PLoS One. 2: e1339.
- Caligiuri MA, Zmuidzinas A, Manley TJ, Levine H, Smith K A, Ritz J (1990). Functional consequences of interleukin 2 receptor expression on resting human lymphocytes. Identification of a novel natural killer cell subset with high affinity receptors. J Exp Med. 171: 1509–1526.
- Carayol G, Robin C, Bourhis JH, Bennaceur-Griscelli A, Chouaib S, Coulombel L, Caignard A (1998). NK cells differentiated from bone marrow, cord blood and peripheral blood stem cells exhibit similar phenotype and functions. Eur J Immunol. 28: 1991–2002.
- Colucci F, Caligiuri MA, Di Santo JP (2003). What does it take to make a natural killer? Nat Rev Immunol. 3: 413–425.
- Condiotti R, Zakai YB, Barak V, Nagler A (2001). Ex vivo expansion of CD56+ cytotoxic cells from human umbilical cord blood. Exp Hematol. 29: 104–113.
- Cooper MA, Fehniger TA, Caligiuri MA (2001). The biology of human natural killer-cell subsets. Trends Immunol. 22: 633–640.
- Farag SS Caligiuri MA (2006). Human natural killer cell development and biology. Blood Rev. 20: 123–137.
- Figueiredo C, Seltsam A, Blasczyk R (2009). Permanent silencing of NKG2A expression for cell-based therapeutics. J Mol Med. 87: 199–210.
- Garcia-Lora A, Algarra I, Garrido F (2003). MHC class I antigens, immune surveillance, and tumor immune escape. J Cell Physiol. 195: 346–355.
- Grzywacz B, Kataria N, Sikora M, Oostendorp RA, Dzierzak EA, Blazar BR, Miller JS, Verneris MR (2006). Coordinated acquisition of inhibitory and activating receptors and functional properties by developing human natural killer cells. Blood. 108: 3824–3833.
- Hallett WH Murphy WJ (2006). Positive and negative regulation of Natural Killer cells: therapeutic implications. Semin Cancer Biol. 16: 367–382.
- Iizuka K, Chaplin DD, Wang Y, Wu Q, Pegg LE, Yokoyama WM, Fu YX (1999). Requirement for membrane lymphotoxin in natural killer cell development. Proc Natl Acad Sci U S A. 96: 6336–6340.
- Kao IT, Yao CL, Kong ZL, Wu ML, Chuang TL, Hwang SM (2007). Generation of natural killer cells from serum-free, expanded human umbilical cord blood CD34+ cells. Stem Cells Dev. 16: 1043–1051.
- Kato N, Tanaka J, Sugita J, Toubai T, Miura Y, Ibata M, Syono Y, Ota S, Kondo T, Asaka M, Imamura M (2007). Regulation of the expression of MHC class I-related chain A, B (MICA, MICB) via chromatin remodeling and its impact on the susceptibility of leukemic cells to the cytotoxicity of NKG2D-expressing cells. Leukemia. 21: 2103–2108.
- Lanier LL (2008). Up on the tightrope: natural killer cell activation and inhibition. Nat Immunol. 9: 495–502.
- Lanier LL, Testi R, Bindl J, Phillips JH (1989). Identity of Leu-19 (CD56) leukocyte differentiation antigen and neural cell adhesion molecule. J Exp Med. 169: 2233–2238.
- Lewis ID Verfaillie CM (2000). Multi-lineage expansion potential of primitive hematopoietic progenitors: superiority of umbilical cord blood compared to mobilized peripheral blood. Exp Hematol. 28: 1087–1095.
- Miller JS Mccullar V (2001). Human natural killer cells with polyclonal lectin and immunoglobulinlike receptors develop from single hematopoietic stem cells with preferential expression of NKG2A and KIR2DL2/L3/S2. Blood. 98: 705–713.
- Moore KA, Ema H Lemischka IR (1997). In vitro maintenance of highly purified, transplantable hematopoietic stem cells. Blood. 89: 4337–4347.
- Moretta L, Biassoni R, Bottino C, Cantoni C, Pende D, Mingari MC, Moretta A (2002). Human NK cells and their receptors. Microbes Infect. 4: 1539–1544.
- Mrozek E, Anderson P,Caligiuri MA (1996). Role of interleukin-15 in the development of human CD56+ natural killer cells from CD34+ hematopoietic progenitor cells. Blood. 87: 2632–2640.
- Nagler A, Lanier LL, Phillips JH (1990). Constitutive expression of high affinity interleukin 2 receptors on human CD16-natural killer cells in vivo. J Exp Med. 171: 1527–1533.
- Orange JS Ballas ZK (2006). Natural killer cells in human health and disease. Clin Immunol. 118: 1–10.
- Orange JS, Fassett MS, Koopman LA, Boyson JE, Strominger JL (2002). Viral evasion of natural killer cells. Nat Immunol. 3: 1006–1012.
- Papamichail M, Perez SA, Gritzapis AD Baxevanis CN (2004). Natural killer lymphocytes: biology, development, and function. Cancer Immunol Immunother. 53: 176–186.
- Robertson MJ Ritz J (1990). Biology and clinical relevance of human natural killer cells. Blood. 76: 2421–2438.
- Robertson MJ, Soiffer RJ, Wolf SF, Manley TJ, Donahue C, Young D, Herrmann SH, Ritz J (1992). Response of human natural killer (NK) cells to NK cell stimulatory factor (NKSF): cytolytic activity and proliferation of NK cells are differentially regulated by NKSF. J Exp Med. 175: 779–788.