Abstract
Adult stem cell niches are characterized by a dichotomy of cycling and quiescent stem cells: while the former are responsible for tissue turnover, their quiescent counterparts are thought to become active upon tissue injury thus underlying the regenerative response. Moreover, quiescence prevents adult stem cells from accumulating mutations thus ensuring a reservoir of unaltered stem cells. In the intestine, while cycling stem cells were shown to give rise to the main differentiated lineages, the identity of their quiescent equivalents remains to date elusive. This is of relevance for conditions such as Crohn's disease and ulcerative colitis where quiescent stem cells may underlie metaplasia and the increased cancer risk associated with chronic inflammation. Tumours are thought to share a comparable hierarchical structure of adult tissues with pluripotent and self-renewing cancer stem cells (CSCs) giving rise to more differentiated cellular types. As such, neoplastic lesions may encompass both cycling and quiescent CSCs. Because of their infrequent cycling, quiescent CSCs are refractory to chemo- and radiotherapy and are likely to play a role in tumour dissemination, dormancy and recurrence.
Introduction
Quiescence in adult stem cell niches
Adult stem cells constitute a population of long-lived cells that have the capacity to self-renew and differentiate not only while fuelling daily turnover in homeostasis but also to underlie the regenerative response upon tissue injury. Given the importance of these tasks, it was originally proposed that adult stem cells must be of slow-cycling nature to preserve their DNA integrity by preventing the accumulation of errors during DNA replication. It has only been in the last few years that the existence of a dichotomy within the adult stem cell population has been acknowledged, i.e. the coexistence of frequently cycling and quiescent adult stem cells each with distinct functions; while frequently cycling adult stem cells are responsible for the daily tissue turnover, their quiescent counterparts are mainly responsible for tissue regeneration in response to damage (CitationFuchs, 2009). This dichotomy of rapidly cycling and quiescent adult stem cells appears to be conserved throughout evolution and was assessed in a broad spectrum of tissues and organisms.
In mice, the presence of quiescent stem cells next to more actively cycling ones has been observed in a variety of tissues (), including cornea (CitationCotsarelis, Cheng, Dong, Sun, & Lavker, 1989), hair follicle (CitationLyle et al., 1999; CitationCotsarelis, Sun, & Lavker,1990; CitationTumbar et al., 2004, CitationLyle et al., 1998; CitationJaks et al., 2008), the hematopoietic system (CitationWilson et al., 2008; CitationFoudi et al., 2009), mammary gland (CitationWelm et al., 2002; CitationShackleton et al., 2006; CitationPece et al., 2010), forebrain (CitationMorshead et al., 1994), pancreas (CitationTeng et al., 2007), prostate (CitationTsujimura et al., 2002), small intestine (CitationPotten, 1998; CitationPotten et al., 2003; CitationNishimura, Wakabayashi, Toyoda, Kashima, & Misufuji, 2003; CitationBarker et al., 2007; CitationRoth, 2011) and the stomach (CitationQiao et al., 2007; CitationBarker et al., 2010). The presence of quiescent stem cells in the intestinal tract will be discussed below.
Table 1. Overview of quiescent stem cells characterized to date.
The methods of choice to identify and isolate quiescent stem cells are mainly based on their intrinsic label-retaining capacity, a consequence of their infrequent cell cycle rates. In general, different cell types within a given tissue can be marked by different dyes, which are either incorporated into the cell membrane, DNA or chromatin. Frequently dividing cells will quickly and progressively lose the label while slow cycling ones will retain it, thus allowing their selective isolation. These cells are referred to as label-retaining cells (LRCs). Early studies on LRCs isolation employed tritiated thymidine (3HTdR) or the thymidine analog Bromodeoxyuridine (5-bromo-2-deoxyuridine, BrdU) (CitationBickenbach, 1981; CitationPotten, 1998). Both are mutagenic agents which become incorporated into the DNA of replicating cells. Thus, 3HTdR- and BrdU-labelling occur in a cell division-dependent fashion. To increase labelling efficiency, a division stimulus such as tissue injury by means of radiation was often applied. From this perspective, the 3HTdR/BrdU labelling itself represents a mutagenic insult to the cells and may thus activate quiescent stem cells to cycle as part of their regenerative response (CitationFoudi et al., 2009). At present, the impact of this labelling procedure on the dynamics of quiescent stem cells is not entirely clear (CitationWilson et al., 2008). Nevertheless, non-mutagenic labelling procedures, which are possibly also independent of cell cycle status, should be preferred.
Labelling of quiescent cells using histone 2B (H2B)-green fluorescent protein (GFP) fusion protein represents an attractive alternative for label retention studies aimed at the identification of quiescent stem cells (CitationTumbar et al., 2004) (). In short, the H2B-GFP fusion gene is regulated by tetracycline-regulatable promoter (tet operator or TetO) in combination with a tissue-specific promoter driving the expression of the transcriptional transactivator (tTA; Tet-Off) version. In the absence of tetracycline or of its more frequently employed analogue doxycycline, production of H2B-GFP is constitutive as the tTA binds to the tet operator and activates H2B-GFP transcription. In the presence of doxycycline, however, the tTA is released from the operator, thus turning off H2B-GFP expression. Vice versa in the Tet-On system, H2B-GFP is normally silenced by constitutive expression of the reverse transcriptional transactivator (rtTA). In this case, doxycycline treatment will inactivate rtTA thus inducing H2B-GFP expression. Notably, in both cases H2B-GFP histone protein will incorporate into chromatin in a replication-independent manner (CitationFoudi et al., 2009). Furthermore H2B-GFP chromatin labelling is not mutagenic (CitationFoudi et al., 2009). H2B-GFP labelling has been employed for the isolation and characterization of quiescent or infrequently dividing cells in a broad spectrum of adult stem cell niches, among others in the skin (CitationTumbar et al., 2004; CitationHsu,Pasolli, & Fuchs, 2011) and the hematopoietic system (CitationFoudi et al., 2009; CitationWilson et al., 2008). However, promiscuous expression of the H2B-GFP transgene has been reported in hematopoietic stem cells (CitationChallen & Goodell, 2008). Therefore, negative controls are extremely important while using the H2B-GFP transgene.
Figure 1. Tet-inducible strategies in pulse-chase experiments to selectively label and prospectively isolate quiescent (stem) cells by H2B-GFP retention.
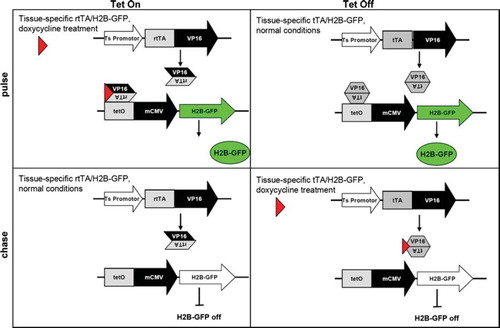
Nonetheless, the isolation and characterization of label retaining cells does not yet prove the existence of quiescent stem cells. Next to quiescent stem cells, any other long-lived, terminally differentiated cells will retain the (H2B-GFP) label for extended periods of time. Stemness of LRCs must be further demonstrated either by transplantation assays or by lineage tracing. The latter technique allows permanent (genetic) marking of the suspected stem cell population and its offspring. To this aim, a gene uniquely expressed in these cells must be identified to allow specific earmarking of the lineages arising from them. Transgenic and “knock-in” approaches where an inducible variant of the C-recombinase (Cre) is placed under the promoter of the marker gene are often employed for this purposes. Cre-expression in the alleged stem cells will recombine Lox target sequences (e.g. Rosa Lox-stop-Lox), thus allowing visualization and tracing of their offspring (CitationBarker et al., 2007).
A third method for the selective labelling of infrequently dividing or quiescent cells relies on the use of fluorescent lipophilic carbocyanines, including the DiI, DiO, PKH2 and PKH26 dyes which are integrated into the phospholipid bilayer of the cell membrane (CitationHoran, Melnicoff, Jensen, & Slezak et al., 1990; CitationSpotl, Sarti,Dierich, & Most, 1995). These membrane dyes can be used to label cells grown in vitro and study their proliferation both in vitro and in vivo. Cell division will result in the progressive dilution of the membrane label thus allowing LRC visualization and prospective isolation by means of immunofluorescence microscopy and fluorescence activated cell sorting (FACS). Membrane labelling dyes are easy to use though their main application is limited to the study of primary ex vivo cells or to established cell lines grown in vitro. As for 3HTdR, BrdU and H2B-GFP, label retention of membrane dyes is not per se indicative of stemness but only of the slow cycling characteristics of a specific subset of cells. Transplantation assays and/or lineage tracing studies need to be performed on the LRCs to assess their self-renewal and differentiation capacities.
Overall, use of H2B-GFP or of fluorescent membrane dyes should be preferred to 3HTdR- and BrdU-based labelling methods to identify potential quiescent stem cells because of their non-mutagenic and cell-cycle independent features, and of the possibility to visualize and prospectively isolate the labelled cells by immunofluorescence and FACS thus allowing subsequent in vitro and in vivo assessment of their stem-like characteristics.
The intestinal stem cell niche
The intestine has served as an ideal model of the adult stem cell niche from flies to mammals mainly because of its high turnover rates and its architectural organization. The mouse small intestine is lined by a continuously regenerating epithelium composed of invaginations, the crypts of Lieberkühn, and extrusions, the villi. This structure is partially conserved in the large intestine (proximal and distal colon) where, however, only crypts (and no villi) are present. Adult stem cells reside in the base of the crypts from where they give rise to a highly proliferative population of transit-amplifying (TA) cells. TA cells eventually differentiate into the four main intestinal cell lineages, namely absorptive enterocytes, secretory goblet cells, hormone-producing enteroendocrine cells and Paneth cells. While enterocytes, goblet and enteroendocrine cells form the epithelial lining of the villus, Paneth cells are the only terminally differentiated cell type which reside within the crypt stem cell zone of the upper intestinal tract, though they are absent in the colon.
Early lineage tracing studies carried out by Cheng and Leblond showed that mitotically active, multipotent stem cells reside in the crypt base and are able to give rise to all four differentiated cell types of the small intestine (CitationCheng & Leblond, 1974). Further studies defined the crypt stem cell zone between positions + 1 and + 4 from the base of the crypt (CitationBjerknes & Cheng, 1981). This groundbreaking work was not followed up until Barker and colleagues identified the Wnt target gene Lgr5 as a marker for the crypt base columnar cells (CitationBarker et al., 2007). Lgr5+ cells cycle on average once every 24 hours and can generate all epithelial lineages, as shown by lineage tracing. Moreover, single sorted Lgr5+ stem cells can form crypt-villus organoids in vitro without a supporting mesenchymal niche, though with a rather low clonogenicity (CitationSato et al., 2009). Subsequently, the same laboratory found that the niche's physical support and signals are provided by the terminally differentiated Paneth cells which reside in close proximity to crypt base columnar cells and can greatly enhance the in vitro capacity of Lgr5 + cells to form crypt-villus organoids (CitationSato et al., 2011). Notably, cell division of this highly proliferative stem cell population seems to occur symmetrically rather than asymmetrically, following a pattern of neutral drift (CitationSnippert et al., 2010; CitationLopez-Garcia,Klein, Simons, & Winton, 2010).
Notwithstanding the strong and elegant experimental evidence supporting the central role of Lgr5+ stem cells in the hierarchical structure of the intestinal epithelium, additional studies pointed out the existence of further stem cell types within the crypt of Lieberkühn. Based on the assumption that some stem cells are slow cycling in order to protect their genome from accumulating errors during DNA replication, Potten and colleagues developed a BrdU-based method to label infrequently dividing LRCs both in intestinal homeostasis and following radiation-induced injury. The BrdU pulse is followed by a period of chase in which the label is progressively diluted out of proliferating but retained in slow cycling cells (see above). Through this analysis, a long-term label-retaining cell population was described that resides at position + 4 from the base of the crypt and undergoes cell division approximately once a week (CitationPotten, 1998). Upon irradiation, these cells become activated and as such possibly underlie the clonogenic regenerative response of the crypt (CitationPotten, 1998; CitationPotten, Owen, & Booth, 2002). However, these pivotal studies were limited by the absence of specific markers to allow isolation and lineage tracing of the + 4 LRCs.
Multiple proteins have been identified to mark the allegedly quiescent+4 cell. The RNA-binding protein Musashi (encoded by the Msi-1 gene) represents a promising candidate though it also marks progeny cells (CitationPotten et al., 2003). The + 4 marker Dcamkl1 (doublecortin-like kinase 1 or Dckl1) is predominantly expressed in BrdU label retaining cells in the lower two thirds of the crypt and, occasionally, in crypt base columnar cells. In support of their stem-like features, Dcamkl1+ cells were shown to form spheroids in suspension culture. When injected subcutaneously, these spheroids give rise to glandular epithelial structures of the intestinal lineage (CitationMay et al., 2008; CitationMay et al., 2009). Yet, these studies fall short of direct lineage tracing of the Dcamkl1+ cells to definitely draw conclusions on their stem cell identity.
The polycomb complex gene Bmi1 was also shown to mark cells at position+4 from the base of the crypt, though by in situ hybridization (ISH) only (CitationSangiorgi & Capecchi, 2008). In the same study, lineage-tracing experiments were conducted in Rosa-lacZ reporter mice expressing an inducible variant of the C-recombinase (Cre) under control of the Bmi1 promoter. Five days after tamoxifen induction, only 1–2 LacZ-labelled cells could be observed in the duodenum, which is suggestive of their slow-cycling nature. Nonetheless, the Bmi1+ cells give rise to all differentiated lineages and their progeny could be detected even one year after tamoxifen induction (CitationSangiorgi & Capecchi, 2008). However, it is not clear whether Bmi1 expression is restricted to the + 4 position or is more widespread throughout the crypt. In fact, quantitative RT-PCR analysis of Lgr5+ cells revealed that Bmi1 is also expressed in crypt base columnar cells (Citationvan der Flier et al., 2009; CitationRoth et al., 2011). Most likely, the cells marked by Bmi1-CreERT2 and by ISH are those expressing Bmi1 at highest levels.
Relative resistance to senescence also represents a common feature of stem cells, though not specifically of their quiescence. As loss of telomeric DNA beyond a critical threshold induces senescence, it is thought that telomerase activity may earmark stem cell compartments. Indeed, It was shown that adult stem cell niches harbour the longest telomeres (CitationFlores et al., 2008). Following this line of reasoning, expression of mouse telomerase reverse transcriptase (mTert) correlates with telomerase activity, is down-regulated upon differentiation in most somatic cells, and is thought to play a central role in adult stem cells. Accordingly, mTert-GFP reporter mice were developed which show GFP-expression in long-term BrdU-retaining single cells located in the lower portion of the intestinal crypts (CitationBreault et al., 2008). More recently, lineage tracing analysis of mTert+ quiescent cells confirmed their identity as slow-cycling, intestinal stem cells (CitationMontgomery et al., 2011).
Our laboratory has applied the in vivo H2B-GFP pulse chase approach () to label and prospectively isolate infrequently dividing cells from the upper GI tract of the mouse (CitationRoth et al., 2009; CitationRoth et al., 2011). Notably, a specific subset of cells were identified which — 1) localize within the lower third of the crypt, 2) can retain the label for more than 80–90 days, 3) have morphological and gene expression features reminiscent of the Paneth lineage, and 4) can respond to tissue insults by cell cycle activation and Bmi1 expression (CitationRoth et al., 2011). Also in this case, progressive dilution of the H2B-GFP label upon multiple rounds of cell divisions does not allow in vivo lineage tracing. Nevertheless, although it is not yet clear what the exact relationship is among Bmi1+, mTert+, Dcamkl1+ and the H2B-GFP-retaining cells, and whether the latter encompass a true stem cell population, the above data suggest that the intestinal stem cell niche encompasses a rather heterogeneous population in terms of marker expression, position along the crypt-villus axis and cycling rates. The dichotomy between the fast cycling crypt base columnar cells (Lgr5+) and a more, yet poorly defined, quiescent stem cell population suggests parallels to other stem cell niches where cycling SCs are responsible for daily tissue turnover while their quiescent counterparts are only activated to underlie the regenerative response in situations of tissue injury.
Notably, the existence of the quiescent and cycling stem cell dichotomy has also been a matter of controversy in the fruit fly Drosphila melanogaster. In particular, whereas a constantly cycling stem cell population was shown to control homeostasis in the adult Drosophila midgut, i.e. the equivalent of the mammalian upper GI tract (CitationMicchelli & Perrimon, 2006; CitationOhlstein & Spradling, 2006), the identity and cycling rate of stem cells within the Drosophila hindgut (equivalent to the mammalian large intestine) have been debated (CitationXie, 2009). According to the original observation, the hindgut is maintained by a population of actively cycling stem cells confined within the HPZ (hindgut proliferation zone) (CitationTakashima, Mkrtchyan, Younossi-Hartenstein, Merriam, & Hartenstein, 2008). Subsequently, this model was argued against by the results by Fox and Spradling who convincingly suggested that hindgut stem cells are generally quiescent whereas their more active counterparts are virtually absent in the hindgut of adult flies (CitationFox & Spradling, 2009). By means of BrdU labelling assays, it was shown that LRCs are distributed sporadically along the proximal part of the hindgut (pylorus and ileum) during larval development whereas in adult flies only few BrdU-retaining cells are restricted to the pylorus. However, the hindgut quiescent stem cells are able to become cycling and move in response to tissue injury to underlie the regenerative response and repair the damaged tissue (CitationFox & Spradling, 2009).
Overall, although the published data are still partially controversial and incomplete, it appears that the intestinal stem cell niche does not represent an exception to the tortoise and hair model where slow-cycling stem cells coexist with their more actively dividing counterparts in homeostasis and tissue injury (CitationFuchs, 2009).
Chronic inflammation, metaplasia and cancers of the digestive tract
Chronic inflammation along the digestive tract is invariably accompanied by metaplasia, i.e. the abnormal transformation of an adult, fully differentiated cell type into a distinct, differentiated cell type that does not normally occur in the tissue in question (CitationSlack, 2009). Pathologists have reported metaplastic changes already for a long time in a broad spectrum of tissues and organs, especially in response to chronic stress, inflammation, and/or tissue damage, often in association with dysplastic changes and cancer (CitationSlack, 2009; CitationHerfs, Hubert, & Delvenne, 2009). Along the digestive tract, intestinal metaplasia has been observed in the oesophagus in association with gastric oesophageal reflux disease (GERD) and Barrett's oesophagus (CitationReid, Li, Gaipeau, & Vaughan, 2010); in the stomach upon Helicobacter pyloris infection (CitationYuasa, 2003), and even in inflammatory bowel diseases (IBD) in the form of Paneth cell metaplasia (see below)(CitationPaterson & Watson, 1961; CitationSommers, 1966).
IBD include conditions such as Crohn's disease and colitis ulcerosa characterized by chronic inflammation of the distal ileum and the colorectum (CitationBaumgart & Carding, 2007). In these pathologies, mucosal tissue is constantly ulcerated, crypt architecture is lost and extensive lymphocyte infiltration can be observed. Loss of integrity of the mucosal barrier leads to a deregulated immune response against the normal commensal microflora. Chronic inflammation is thus characterized by continuous tissue destruction followed by repair and by a persistent activation of immune cells. A striking morphologic observation in IBD is represented by Paneth cell metaplasia, i.e. the presence of Paneth cells in the non-neoplastic colonic tissue adjacent to these tumours (CitationPaterson & Watson, 1961). While Paneth cells form an essential part of the small intestinal stem cell niche, they are rarely observed in the colon, especially in young, healthy individuals. Paneth cell metaplasia is of interest in view of the observations according to which these terminally differentiated cells were shown to function as niche for Lgr5 + stem cells (CitationSato et al., 2011), and, allegedly, as quiescent stem-like cells (CitationRoth et al., 2011).
Apart from the morbidity associated with chronic inflammation of the intestinal mucosa, both Crohn's disease and colitis ulcerosa are strongly associated with increased risk of small bowel and colorectal carcinoma. In ulcerative colitis patients, the duration and severity of inflammation correlate with the cumulative probability of colorectal cancer, namely from 2 % at 10 years to 18 % after 30 years (CitationEaden,Abrams, & Mayberry, 2001). Even more strikingly, Crohn's patients with an inflammation restricted to the small intestine have a 12 to 60-fold increased risk of developing adenocarcinoma of the small intestine compared to healthy individuals (CitationBernstein, Blanchard, Kliewer, & Wajda, 2001). However, the molecular and cellular mechanisms underlying the causal relationship between inflammation and bowel cancer are still poorly understood.
Mouse models represent valuable tools for studying the connection between intestinal inflammation and cancer. IBD can be induced both genetically as well as chemically (CitationWestbrook, Szakmary, & Schiestl, 2010). Colitis and colitis-associated cancers can be triggered by the administration of dextran sodium sulfate (DSS), a non-genotoxic sulfated polysaccharide, in the drinking water. Chronic DSS treatment induces a ulcerative colitis pathology, including inflammation of the colorectum, epithelial cell erosions, crypt distortions, epithelial proliferation and dysplasia (CitationCooper,Murthy, Shah, & Sedergran, 1993). Administering DSS to tumour-prone animals such as ApcMin/ + leads to a strong increase of colorectal carcinogenesis due to the chronic inflammation (CitationTanaka et al., 2006). This is thought to occur in a stepwise progression from epithelial hyperplasia to dysplasia, adenoma, and adenocarcinoma. Over time, cells acquire DNA damage caused by inflammation-associated high levels of reactive oxygen and nitrogen species (CitationItzkowitz & Yio, 2004; CitationMeira et al., 2008).
A role for quiescent stem cells in inflammation-associated tumorigenesis?
Although frequently interpreted as a form of trans-differentiation, the metaplastic changes often observed in the context of chronic inflammatory conditions are most likely the result of reprogramming of the differentiation pattern of resident stem cells (CitationSlack, 2009). The connection between stem cell reprogramming and metaplasia is best illustrated in embryonic development where different tissues arise through the combinatorial expression levels of specific morphogens (code). In general, adult tissue stem cells are thought to retain this morphogen code. However, environmental changes and/or (epi-)genetic modifications may alter their code and trigger aberrant differentiation into different tissue types. If the metaplastic tissue entails a growth advantage within the altered environment, it will persist and possibly overgrow the endogenous tissue (CitationSlack, 2009). Likewise, Paneth cell metaplasia in the colorectum of IBD patients is most likely the result of reprogramming of the resident colonic tissue stem cell caused by chronic inflammation and continuous tissue repair. Morphologically, the metaplastic Paneth cells do not differ from the ones present in the small intestine. However, mutation and loss of heterozygosity (LOH) analysis of human Paneth cell metaplasia revealed both KRAS mutations and aneuploidy (CitationWada, Yamaguchi, & Tadokoro,, 2005). Neoplastic Paneth cells can also be observed within inflammation-related colorectal adenocarcinomas (CitationImai et al., 2007). These observations led to the development of a new hypothesis on the sequence of events underlying colorectal carcinogenesis in IBD, i.e. epithelial inflammation, followed by colorectal Paneth cell metaplasia and neoplasia (CitationWada, 2009).
Judging from their early presence during metaplasia and tumorigenesis, Paneth cells are seemingly of functional relevance and represent one of the first differentiation lineages resulting from the alleged reprogramming of the resident colonic stem cell. In view of the above mentioned data pointing at the existence of intestinal quiescent stem-like cells closely related to the Paneth lineage and capable of cycling upon tissue injury (CitationRoth et al., 2011), and to the supporting role of mature Paneth cells as niche of Lgr5+ crypt base columnar cells (CitationSato et al., 2011), it is likely that their early appearance in IBD plays a functional role in the regenerative response to inflammation-induced tissue damage and in cancer onset. We thus hypothesize a mechanism in which chronic inflammation triggers the activation of quiescent stem cells of the gut that increase their turnover rate in order to facilitate tissue repair. This increase in division rate, together with a constant exposure to mutagenic inflammatory agents, predisposes the quiescent stem cell population to acquire somatic mutations and/or epigenetic alterations. Alterations within the quiescent stem cells will eventually lead to transformation and thus tumour development. In this scenario, quiescent stem cells of the colon could be the cell of origin of inflammation-related malignancies ().
Barker and colleagues provided evidence for the cycling stem cell as the cell of origin of intestinal tumourigenesis by showing that Apc mutations lead to the formation of multiple adenomas in the small intestine and colon exclusively when induced in Lgr5+ crypt base columnar cells (CitationBarker et al., 2009). When the same Apc mutation was induced in the transit amplifying cell compartment, microadenomas were observed which do not progress into bona fide tumours. In a second study, the endogenous promoter of the cancer stem cell marker CD133 (prominin, Prom1) was used to drive inducible Cre-expression (CitationZhu et al., 2009) and to demonstrate that Prom1 expression largely overlap with that of Lgr5 at the base of the small intestinal crypt, albeit absent in the colon. Accordingly, Wnt signalling activation within the Prom1+ cells leads to massive dysplasia in the small intestine but not in the colon (CitationZhu et al., 2009).
Thus, alterations in tissue stem cells are the origin of some types of cancer, amongst others colon cancer. In the specific case of the digestive tract, a scenario can be envisaged where cycling stem cells represent the cell of origin of sporadic cancers (i.e. not associated with chronic inflammation) whereas their more quiescent equivalents are likely to play a similar role in cancers arising in individuals affected by inflammatory conditions.
Cancer stem cell quiescence
The cancer stem cell (CSC) model postulates that multipotent, self-renewing cancer stem cells co-exist with more differentiated and mature cells within the tumour mass. In this scenario, the tumour recapitulates the hierarchical structure of normal, self-renewing tissues. By further extending this parallel between self-renewing tissues and tumour masses, the dichotomy of cycling versus quiescent stem cells may also apply for the CSC niche. Do quiescent cancer stem cells (qCSCs) co-exist together with their more actively cycling and tumour-propagating counterparts? If validated, the very existence of qCSCs may have profound clinical implications, as malignant cells with quiescent characteristics are more likely to be resistant to conventional cytotoxic therapeutic agents whose mode of action relies on the introduction of DNA damage into dividing cells. Moreover, qCSCs may represent the elusive dormant cancer cells, which underlie metastasis in cancer patients even decades after removal of the primary tumour (CitationAguirre-Ghiso, 2007, Muller, Alix-Panabieres, & CitationPantel, 2010).
The existence of quiescent CSCs has now been demonstrated in several tumour types (). Using the membrane labelling dyes PKH26 and PKH67, ovarian adenocarcinoma cell lines were studied for their label retention properties when injected subcutaneously into NOD-SCID mice (CitationKusumbe & Bapat, 2009). Notably, LRCs encompassed a population of stem-like cells, which were shown to be reversibly quiescent and refractory to therapy. Following chemotherapy, a fraction of these cells acquires the potential to proliferate in a drug-refractory manner (Kusumbe and Bapat, 2009).
Table 2. Overview of quiescent cancer stem cells characterized to date.
Similarly, slow cycling stem-like cells were isolated from pancreas adenocarcinoma cell lines by using the long term lipophilic tracer dye similar to PKH26, DiI (C59H97ClN2O4) (CitationDembinski and Krauss, 2009). Notwithstanding the only partial overlap between the DiI + slow-cycling cells (DiI+ /SCCs) and other CD markers commonly employed to enrich CSCs from pancreatic adenocarcinomas (CD133+, CD24+ /CD44+), they were shown to have increased in vivo tumorigenic potential and a fibroblast-like morphology suggestive of an epithelial-mesenchymal transition (EMT). Indeed, several EMT markers were found to be increased among DiI +/SCCs (CitationDembinski and Krauss, 2009).
A subpopulation of slow cycling, PKH26 label retaining melanoma cells was also identified in primary melanoma cell lines (CitationRoesch et al., 2010). These cells express the H3K4 methylase JARID1B whose knockdown results in tumour exhaustion, thus suggesting that the JARID1B-positive cells are essential for continuous tumour growth. Notably, both the melanoma and pancreas cancer studies revealed a dynamic process affecting the qCSCs and their progenies as indicated by the capacity of LRCs to generate non-LRCs and, more surprisingly, of non-LRCs to give rise to LRCs (CitationDembinski & Krauss, 2009; CitationRoesch et al., 2010). This dynamic regulation of tumour cells is in apparent contradiction with the hierarchical CSC model (CitationQuintana et al., 2008) and could be explained by cancer cells undergoing EMT and MET in response to environmental cues (CitationMani et al., 2008).
PKH26 label retention was also employed to define a molecular signature of human normal mammary stem cells (hNMSC) more commonly represented in high vs low-grade breast cancers. Accordingly, mammospheres derived from high grade tumours displayed a higher percentage of PKH26 label retaining cells and, vice versa, hNMSC+ breast cancer cells more efficiently formed mammospheres in vitro and tumours in vivo when compared with hNMSC cells (CitationPece et al., 2010).
In hepatocellular carcinoma (HCC), a side population fraction of cells was identified that is partly marked by the surface marker CD13 (CitationHaraguchi et al., 2010). CD13-positive cells are semiquiescent as shown by Hoechst 33342 and by the RNA-binding dye Pyronin Y, and typically formed cell clusters within the tumour. In mouse xenograft models, the combination of the CD13-inhibitor ubenimex and a genotoxic agent such as 5-fluorouracil proved to be most efficient in reducing tumor size than either agent alone (CitationHaraguchi et al., 2010). CD13+ cells resist chemotherapy because of reduced reactive oxygen species (ROS) and, accordingly, acquire chemosensitivity upon ubenimex treatment and increase of ROS levels (CitationHaraguchi et al., 2010).
Possibly the most clinically relevant example of quiescent cancer stem cells comes from a mouse model of acute myelogenous leukaemia (AML), where patient-derived CSCs have been identified that engraft within the bone marrow endosteal region where they are protected from chemotherapy-induced apoptosis (CitationIshikawa et al., 2007). Subsequent characterization of these leukaemia stem cells showed that they are partly quiescent and can be induced to enter cell cycle through a pre-treatment with granulocyte colony-stimulating factor (G-CSF). Accordingly, a combined G-CSF and chemotherapy treatment enhances the induction of apoptosis and elimination of AML stem cells thus significantly increasing survival when compared to chemotherapy alone (CitationSaito et al., 2010).
Lastly, in human ovarian cancers, the surface molecule CD24 marks a subpopulation of cancer cells that is enriched for CSCs characterized by a lower proliferation rate than the bulk of the tumour (Gao, Choi, Kang, Youn & Cho, 2010). The CD24 + qCSCs were able to form tumour xenografts in nude mice at low multiplicities, whereas equal number of CD24− cells remained non-tumorigenic. Notably, a number of ‘stemness’ genes, including Nestin, β-catenin, Bmi1, and Oct3/4 were found to be expressed in CD24+ cells at higher level than bulk tumour cells (CitationGao et al., 2010).
These results are of interest as CD24 is up-regulated in the regenerating intestinal mucosa from patients affected by inflammatory bowel disease (CitationAhmed et al., 2010) and could represent a marker of quiescent stem-like cells activated by tissue insults. Notably, CD24 also earmarks cells of the Paneth lineage in the mouse (CitationSato et al., 2011), previously shown to have label-retaining capacities and to undergo specific changes upon inflammation, irradiation and chemotherapy including hyperplasia and metaplasia (CitationPorter, Bevins, Ghosh, & Ganz, 2002). These observations, together with our own preliminary data showing that quiescent cells of the upper GI tract share features of the Paneth cell lineage (CitationRoth et al., 2011), point to a potentially relevant role of Paneth cells in the regeneration of intestinal mucosa.
Overall, quiescent cancer stem cells seem to be common to a broad spectrum of malignancies and are likely to be of clinical relevance both because of their intrinsic resistance to chemo- and radiotherapy and due to their alleged identity as dormant tumour cells causing relapses and distant metastases in cancer patients.
Quiescent CSCs: clinical relevance
Cancer treatments have become more efficient and successful over the last decades. However, metastases occurring from tumour cell dissemination after a period of dormancy still represent the main cause of morbidity and mortality among individual cancer patients. Tumour dormancy is a widely used term to describe the state in which tumour cells exist for long periods of time following treatment and/or surgical removal of the primary tumour. From their dormant state, quiescent cancer cells can subsequently switch to a state of active cycling and underlie tumour relapse. In the clinic, tumours are considered dormant if they recur after at least 5 years from surgical treatment. Whereas 85% of recurrences of colon tumours already occur within the first three years, patients with breast cancer are at risk for up to 12 years after surgery (CitationSargent et al., 2007, CitationSaphner, Tormey, & Gray, 1996). The notion that (quiescent) cancer stem cells may be resistant to therapy implies that many cytotoxic regimens would result in an initial shrinkage of the tumour mass but also in the relative enrichment in the CSCs (CitationReya, Morrison, Clarke, & Weissmen, 2001). Apart from their slow cycling rates, additional mechanisms are likely to underlie CSCs’ innate resistance to therapy: up-regulation of cellular efflux pumps e.g. the multidrug resistance transporter MDR-1 (CitationKnutsen et al., 1998; CitationMickley, Spengler, Knutson, Biedler, & Fojo et al., 1997), activation of DNA damage response (CitationBao et al., 2006), and lower concentrations of critical mediators of ionizing-radiation-induced cell killing such as ROS (CitationDiehn et al., 2009). Understanding the biology of tumour dormancy and the molecular and cellular mechanisms underlying both its maintenance and the triggers of cell cycle activation leading to metastatic growth will be crucial for the future development of targeted therapies.
Metastasis can be viewed as a rather inefficient process (CitationWeiss and Ward, 1990) where tumour cells need to undergo epithelial to mesenchymal transitions in order to detach from the primary tumour, enter the blood stream to be spread throughout the body, extravasate from the blood vessels, and eventually home in a distant organ and establish a metastatic outgrowth by mesenchymal to epithelial transition (CitationBrabletz, Jung, Spaderna, Hlubek & Kirchner, 2005). In various types of cancer, disseminated and circulating tumour cells (DTCs, CTCs) are commonly observed in the bone marrow and blood of cancer patients, respectively. Notably, dissemination can occur even at very early stages as shown both for colorectal adenoma and breast cancer patients (CitationSteinert, Veith, Hantschick, & Reymond, 2005; CitationHusemann et al., 2008). DTCs are mainly detected by means of immunocytochemical staining or PCR-based analyses of bone marrow samples collected from the iliac crest (CitationPantel & Brakenhoff, 2004; CitationPantel,Brakenhoff, & Brandt, 2008). On the other hand, peripheral blood represents a more easily accessible site of sampling for monitoring circulating tumour cells (CTCs). Overall, DTCs and, to a lesser extent, CTCs were shown to have prognostic significance and predict unfavourable clinical outcome especially in breast cancer (CitationBraun et al., 2005; CitationPantel et al., 2008). However, the relationship between DTCs/CTCs and (quiescent) cancer stem cells is still correlative in nature (CitationMuller et al., 2010). DTCs and CTCs are viable tumour cells of epithelial origin that exist in a non-proliferating, quiescent state and have been shown to resist to systemic chemotherapy (CitationBecker et al., 2006; CitationBecker, Solomeyer-Pergola, Wallruiener, & Fehn, 2007). Moreover, DTCs from early stage breast cancer patients encompass considerable numbers of cells expressing the CD44 + CD24-/low markers characteristic of CSCs (CitationBalic et al., 2006). Also, breast DTCs show increased angiogenic capacity and the expression of the CXCR4 receptors likely to facilitate their dissemination to distant organs (CitationBalic et al., 2006).
The expression of receptors such as CXCR4 and even HER2 previously found in CSCs (CitationGalie et al., 2008) is of good auspices for the future development of antibodies-based targeted therapies and may explain the positive results obtained by trastuzumab in preventing metastasis in breast cancer patients (CitationBrufsky, 2009). Likewise, the inefficacy of conventional cytotoxic chemotherapy (doxorubicin) on the number of dormant breast CTCs (CitationNaumov et al., 2003) highlights the need for tailor-made intervention strategies aimed at quiescent cancer cells.
Conclusions
Future directions
The intestine, like many other adult stem cell niches, is a hierarchically organized, self-renewing tissue characterized by a dichotomy of cycling and quiescent stem cells. While the actively cycling stem cells are responsible for daily tissue turnover, their quiescent counterparts are likely to become activated upon tissue injury in the regenerative response underlying tissue repair. In conditions such as IBD, the chronic inflammation continuously stimulates the quiescent stem cell population to cycle. We here propose that, because of the presence of mutagenic substances in the inflammatory tissue and of their high turnover rate, quiescent tissue stem accumulate somatic mutations. In parallel, constant exposure to inflammation leads to stem cell reprogramming resulting in metaplastic changes. In the colon, the presence of Paneth cell within the inflamed epithelium indicates a pre-cancerous condition characterized by the activation of otherwise quiescent stem cells and the presence of metaplastic and dysplastic lesions. Prolonged exposure to chronic inflammation will eventually result in the accumulation of additional somatic mutations and further progression towards invasive colorectal carcinomas.
Apart from their putative role as cell of origin of inflammation-associated tumours, quiescent stem cells are also likely to play a highly relevant role from within the tumours. Analogous to the normal stem cell niches, malignancies encompass both cycling and quiescent cancer stem cells. While the former fuel the tumour mass and underlie its growth, quiescent cancer stem cells are likely to play a central role in dissemination and metastasis formation at distant organ sites. In view of their intrinsic resistance to radio- and chemotherapy future therapeutic strategies will need to tackle disseminated dormant cancer cells to prevent recurrence in cancer patients.
Acknowledgements
The authors are grateful to Mr. Frank van der Panne for his assistance with the artwork.
Declaration of interest: The authors declare no conflict of interest. This study was supported among others by grants from the Dutch Cancer Society, the BSIK program of the Dutch Government grant 03038 (www.stemcells.nl), and the EU FP6 and FP7 consortia Migrating Cancer Stem Cells program (MCSCs; www.mcscs.eu) and TuMIC (integrated concept of tumor metastasis (http://itgmv1.fzk.de/www/tumic/tumic_main.htm).
References
- Aguirre-Ghiso JA (2007). Models, mechanisms and clinical evidence for cancer dormancy. Nat Rev Cancer. 7: 834–846.
- Ahmed MA, Jackson D, Seth R, Robins A, Lobo Dn, Tomlinson Ip, and Ilyas M (2010). CD24 is upregulated in inflammatory bowel disease and stimulates cell motility and colony formation. Inflamm Bowel Dis. 16: 795–803.
- Balic M, Lin H, Young L, Hawes D, Giuliano A, Mcnamara G, Datar Rh, and Cote Rj (2006). Most early disseminated cancer cells detected in bone marrow of breast cancer patients have a putative breast cancer stem cell phenotype. Clin Cancer Res. 12: 5615–21.
- Bao S, Wu Q, Mclendon RE, Hao Y, Shi Q, Hjelmeland Ab, Dewhirst Mw, Bigner Dd, and Rich Jn (2006). Glioma stem cells promote radioresistance by preferential activation of the DNA damage response. Nature. 444: 756–60.
- Barker N, Huch M, Kujala P, Van De Wetering M, Snippert HJ, Van Es JH, Sato T, Stange De, Begthel H, Van Den Born M, Danenberg E, Van Den Brink S, Korving J, Abo A, Peters PJ, Wright N, Poulsom R, and Clevers H (2010). Lgr5(+ ve) stem cells drive self-renewal in the stomach and build long-lived gastric units in vitro. Cell Stem Cell. 6: 25–36.
- Barker N, Ridgway RA, Van Es JH Van De Wetering M, Begthel H, Van Den Born M, Danenberg E, Clarke AR, Sansom Oj, and Clevers H (2009). Crypt stem cells as the cells-of-origin of intestinal cancer. Nature. 457: 608–611.
- Barker N, Van Es JH, Kuipers J, Kujala P, Van Den Born M, Cozijnsen M, Haegebarth A, Korving J, Begthel H, Peters PJ, and Clevers H (2007). Identification of stem cells in small intestine and colon by marker gene Lgr5. Nature. 449: 1003–1007.
- Baumgart DC And Carding SR (2007). Inflammatory bowel disease: cause and immunobiology. Lancet. 369: 1627–1640.
- Becker S, Becker-Pergola G, Wallwiener D, Solomayer EF, and Fehm T (2006). Detection of cytokeratin-positive cells in the bone marrow of breast cancer patients undergoing adjuvant therapy. Breast Cancer Res Treat. 97: 91–96.
- Becker S, Solomayer E, Becker-Pergola G, Wallwiener D, and Fehm T (2007). Primary systemic therapy does not eradicate disseminated tumor cells in breast cancer patients. Breast Cancer Res Treat. 106: 239–243.
- Bernstein CN, Blanchard JF, Kliewer E, and Wajda A (2001). Cancer risk in patients with inflammatory bowel disease: a population-based study. Cancer. 91:854–862.
- Bickenbach JR (1981). Identification and behavior of label-retaining cells in oral mucosa and skin. J Dent Res. 60 Spec No C: 1611–1620.
- Bjerknes M And Cheng H (1981). The stem-cell zone of the small intestinal epithelium. III. Evidence fromcolumnar, enteroendocrine, and mucous cells in the adult mouse. Am J Anat. 160: 77–91.
- Brabletz T, Jung A, Spaderna S, Hlubek F, and Kirchner T (2005). Opinion: migrating cancer stem cells - an integrated concept of malignant tumour progression. Nat Rev Cancer. 5: 744–9.
- Braun S, Vogl Fd, Naume B, Janni W, Osborne MP, Coombes RC, Schlimok G, Diel IJ, Gerber B, Gebauer G, Pierga JY, Marth C, Oruzio D, Wiedswang G, Solomayer Ef, Kundt G, Strobl B, Fehm T, Wong Gy, Bliss J, Vincent-Salomon A, and Pantel K (2005). A pooled analysis of bone marrow micrometastasis in breast cancer. N Engl J Med. 353: 793–802.
- Breault Dt, Min IM, Carlone DL, Farilla LG, Ambruzs Dm, Henderson De, Algra S, Montgomery RK, Wagers AJ, and Hole N (2008). Generation of mTert-GFP mice as a model to identify and study tissue progenitor cells. Proc Natl Acad Sci U S A. 105: 10420–10425.
- Brufsky A (2009). Trastuzumab-based therapy for patients with HER2-positive breast cancer: from early scientific development to foundation of care. Am J Clin Oncol. 33: 186–195.
- Challen GA and Goodell MA (2008). Promiscuous expression of H2B-GFP transgene in hematopoietic stem cells. PLoS One. 3: e2357.
- Cheng H And Leblond Cp (1974). Origin, differentiation and renewal of the four main epithelial cell types in the mouse small intestine. V. Unitarian Theory of the origin of the four epithelial cell types. Am J Anat. 141: 537–561.
- Cooper HS, Murthy SN, Shah RS, and Sedergran DJ (1993). Clinicopathologic study of dextran sulfate sodium experimental murine colitis. Lab Invest. 69: 238–249.
- Cotsarelis G, Cheng SZ, Dong G, Sun TT, and Lavker Rm (1989). Existence of slow-cycling limbal epithelial basal cells that can be preferentially stimulated to proliferate: implications on epithelial stem cells. Cell. 57: 201–209.
- Cotsarelis G, Sun TT, and Lavker RM (1990). Label-retaining cells reside in the bulge area of pilosebaceous unit: implications for follicular stem cells, hair cycle, and skin carcinogenesis. Cell. 61:1329–1337.
- Dembinski Jl And Krauss S (2009). Characterization and functional analysis of a slow cycling stem cell-like subpopulation in pancreas adenocarcinoma. Clin Exp Metastasis. 26: 611–623.
- Diehn M, Cho Rw, Lobo Na, Kalisky T, Dorie Mj, Kulp An, Qian D, Lam Js, Ailles Le, Wong M, Joshua B, Kaplan Mj, Wapnir I, Dirbas Fm, Somlo G, Garberoglio C, Paz B, Shen J, Lau Sk, Quake Sr, Brown Jm, Weissman Il, and Clarke Mf (2009). Association of reactive oxygen species levels and radioresistance in cancer stem cells. Nature. 458:780–783.
- Eaden Ja, Abrams Kr, and Mayberry Jf (2001). The risk of colorectal cancer in ulcerative colitis: a meta-analysis. Gut. 48: 526–535.
- Flores I, Canela A, Vera E, Tejera A, Cotsarelis G, and Blasco Ma (2008). The longest telomeres: a general signature of adult stem cell compartments. Genes Dev. 22: 654–67.
- Foudi A, Hochedlinger K, Van Buren D, Schindler Jw, Jaenisch R, Carey V, and Hock H (2009). Analysis of histone 2B-GFP retention reveals slowly cycling hematopoietic stem cells. Nat Biotechnol. 27: 84–90.
- Fox Dt And Spradling AC (2009). The Drosophila hindgut lacks constitutively active adult stem cells but proliferates in response to tissue damage. Cell Stem Cell. 5: 290–297.
- Fuchs E (2009). The tortoise and the hair: slow-cycling cells in the stem cell race. Cell. 137: 811–819.
- Galie M, Konstantinidou G, Peroni D, Scambi I, Marchini C, Lisi V, Krampera M, Magnani P, Merigo F, Montani M, Boschi F, Marzola P, Orru R, Farace P, Sbarbati A, and Amici A (2008). Mesenchymal stem cells share molecular signature with mesenchymal tumor cells and favor early tumor growth in syngeneic mice. Oncogene. 27: 2542–251.
- Gao MQ, Choi YP, Kang S, Youn JH, and Cho NH (2010). CD24 + cells from hierarchically organized ovarian cancer are enriched in cancer stem cells. Oncogene. 29: 2672–2680.
- Haraguchi N, Ishii H, Mimori K, Tanaka F, Ohkuma M, Kim HM, Akita H, Takiuchi D, Hatano H, Nagano H, Barnard GF, Doki Y, and Mori M (2010). CD13 is a therapeutic target in human liver cancer stem cells. J Clin Invest. 120: 3326–3339.
- Herfs M, Hubert P, and Delvenne P (2009). Epithelial metaplasia: adult stem cell reprogramming and (pre)neoplastic transformation mediated by inflammation? Trends Mol Med. 15: 245–253.
- Horan Pk, Melnicoff Mj, Jensen Bd, and Slezak Se (1990). Fluorescent cell labeling for in vivo and in vitro cell tracking. Methods Cell Biol. 33: 469–490.
- Hsu YC, Pasolli HA, and Fuchs E (2011). Dynamics between Stem Cells, Niche, and Progeny in the Hair Follicle. Cell. 144: 92–105.
- Husemann Y, Geigl JB, Schubert F, Musiani P, Meyer M, Burghart E, Forni G, Eils R, Fehm T, Riethmuller G, and Klein CA (2008). Systemic spread is an early step in breast cancer. Cancer Cell. 13: 58–68.
- Imai T, Fukuta K, Hasumura M, Cho YM, Ota Y, Takami S, Nakagama H, and Hirose M (2007). Significance of inflammation-associated regenerative mucosa characterized by Paneth cell metaplasia and beta-catenin accumulation for the onset of colorectal carcinogenesis in rats initiated with 1,2-dimethylhydrazine. Carcinogenesis. 28: 2199–2206.
- Ishikawa F, Yoshida S, Saito Y, Hijikata A, Kitamura H, Tanaka S, Nakamura R, Tanaka T, Tomiyama H, Saito N, Fukata M, Miyamoto T, Lyons B, Ohshima K, Uchida N, Taniguchi S, Ohara O, Akashi K, Harada M, and Shultz LD (2007). Chemotherapy-resistant human AML stem cells home to and engraft within the bone-marrow endosteal region. Nat Biotechnol. 25: 1315–21.
- Itzkowitz SH and Yio X (2004). Inflammation and cancer IV. Colorectal cancer in inflammatory bowel disease: the role of inflammation. Am J Physiol Gastrointest Liver Physiol. 287: G7–G17.
- Jaks V, Barker N, Kasper M, Van EE JH, Snippert HJ, Clevers H, and Toftgard R (2008). Lgr5 marks cycling, yet long-lived, hair follicle stem cells. Nat Genet. 40: 1291–1299.
- Knutsen T, Mickley LA, Ried T, Green ED, Du Manoir S, Schrock E, Macville M, Ning Y, Robey R, Polymeropoulos M, Torres R, and Fojo T (1998). Cytogenetic and molecular characterization of random chromosomal rearrangements activating the drug resistance gene, MDR1/P-glycoprotein, in drug-selected cell lines and patients with drug refractory ALL. Genes Chromosomes Cancer. 23: 44–54.
- Kusumbe AP and Bapat SA (2009). Cancer stem cells and aneuploid populations within developing tumors are the major determinants of tumor dormancy. Cancer Res. 69: 9245–53.
- Lopez-Garcia C, Klein AM, Simons BD, and Winton DJ (2010). Intestinal stem cell replacement follows a pattern of neutral drift. Science. 330: 822–825.
- Lyle S, Christofidou-Solomidou M, Liu Y, Elder DE, Albelda S, and Cotsarelis G (1998). The C8/144B monoclonal antibody recognizes cytokeratin 15 and defines the location of human hair follicle stem cells. J Cell Sci. 111 (Pt 21): 3179–3188.
- Lyle S, Christofidou-Solomidou M, Liu Y, Elder DE, Albelda S, and Cotsarelis G (1999). Human hair follicle bulge cells are biochemically distinct and possess an epithelial stem cell phenotype. J Investig Dermatol Symp Proc. 4: 296–301.
- Mani SA, Guo W, Liao MJ, Eaton EN, Ayyanan A, Zhou Ay, Brooks M, Reinhard F, Zhang CC, Shipitsin M, Campbell LI, Polyak K, Brisken C, Yang J, and Weinberg RA (2008). The epithelial-mesenchymal transition generates cells with properties of stem cells. Cell. 133: 704–715.
- May R, Riehl TE, Hunt C, Sureban SM, Anant S, and Houchen CW (2008) Identification of a novel putative gastrointestinal stem cell and adenoma stem cell marker, doublecortin and CaM kinase-like-1, following radiation injury and in adenomatous polyposis coli/multiple intestinal neoplasia mice. Stem Cells, 26:630–637.
- May R, Sureban SM, Hoang N, Riehl Te, Lightfoot SA, Ramanujam R, Wyche JH, Anant S, and Houchen CW (2009). Dcamkl-1 and LGR5 Mark Quiescent and Cycling Intestinal Stem Cells Respectively. Stem Cells, 10: 2571–2579.
- Meira LB, Bugni JM, Green Sl, Lee CW, Pang B, Borenshtein D, Rickman BH, Rogers AB, Moroski-Erkul CA, Mcfaline Jl, Schauer DB, Dedon PC, Fox JG, and Samson LD (2008) DNA damage induced by chronic inflammation contributes to colon carcinogenesis in mice. J Clin Invest. 118: 2516–2525.
- Micchelli CA And Perrimon N (2006). Evidence that stem cells reside in the adult Drosophila midgut epithelium. Nature. 439: 475–479.
- Mickley La, Spengler BA, Knutsen Ta, Biedler JI, and Fojo T (1997). Gene rearrangement: a novel mechanism for MDR-1 gene activation. J Clin Invest. 99: 1947–1957.
- Montgomery RK, Carlone DI, Richmond CA, Farilla L, Kranendonk Me, Henderson DE, Baffour-Awuah NY, Ambruzs DM, Fogli Lk, Algra S, and Breault Dt (2011). Mouse telomerase reverse transcriptase (mTert) expression marks slowly cycling intestinal stem cells. Proc Natl Acad Sci U S A. 108: 179–184.
- Morshead Cm, Reynolds BA, Craig CG, Mcburney MW, Staines Wa, Morassutti D, Weiss S, And Van Der Kooy D (1994). Neural stem cells in the adult mammalian forebrain: a relatively quiescent subpopulation of subependymal cells. Neuron. 13: 1071–1082.
- Muller V, Alix-Panabieres C, and Pantel K (2010). Insights into minimal residual disease in cancer patients: implications for anti-cancer therapies. Eur J Cancer. 46: 1189–1197.
- Naumov Gn, Townson JI, Macdonald IC, Wilson Sm, Bramwell Vh, Groom Ac, and Chambers Af (2003). Ineffectiveness of doxorubicin treatment on solitary dormant mammary carcinoma cells or late-developing metastases. Breast Cancer Res Treat. 82: 199–206.
- Nishimura S, Wakabayashi N, Toyoda K, Kashima K, and Mitsufuji S (2003). Expression of Musashi-1 in human normal colon crypt cells: a possible stem cell marker of human colon epithelium. Dig Dis Sci. 48: 1523–1529.
- Ohlstein B and Spradling A (2006). The adult Drosophila posterior midgut is maintained by pluripotent stem cells. Nature. 439: 470–474.
- Pantel K and Brakenhoff RH (2004). Dissecting the metastatic cascade. Nat Rev Cancer. 4: 448–456.
- Pantel K, Brakenhoff RH, and Brandt B (2008). Detection, clinical relevance and specific biological properties of disseminating tumour cells. Nat Rev Cancer. 8: 329–340.
- Paterson JC and Watson SH (1961). Paneth cell metaplasia in ulcerative colitis. Am J Pathol. 38: 243–249.
- Pece S, Tosoni D, Confalonieri S, Mazzarol G, Vecchi M, Ronzoni S, Bernard L, Viale G, Pelicci PG, and Di Fiore PP (2010). Biological and molecular heterogeneity of breast cancers correlates with their cancer stem cell content. Cell. 140: 62–73.
- Porter EM, Bevins CI, Ghosh D, and Ganz T (2002). The multifaceted Paneth cell. Cell Mol Life Sci. 59: 156–170.
- Potten CS (1998). Stem cells in gastrointestinal epithelium: numbers, characteristics and death. Philos Trans R Soc Lond B Biol Sci. 353: 821–830.
- Potten CS, Booth C, Tudor GI, Booth D, Brady G, Hurley P, Ashton G, Clarke R, Sakakibara S-I, and Okano H (2003). Identification of a putative intestinal stem cell and early lineage marker; musashi-1. Differentiation. 71: 28–41.
- Potten CS, Owen G, and Booth D (2002). Intestinal stem cells protect their genome by selective segregation of template DNA strands. J Cell Sci. 115: 2381–2388.
- Qiao XT, Ziel JW, Mckimpson W, Madison BB, Todisco A, Merchant JL, Samuelson LC, and Gumucio DL (2007). Prospective identification of a multilineage progenitor in murine stomach epithelium. Gastroenterology. 133: 1989–1998.
- Quintana E, Shackleton M, Sabel MS, Fullen DR, Johnson TM, and Morrison SJ (2008). Efficient tumour formation by single human melanoma cells. Nature. 456: 593–598.
- Reid BJ, Li X, Galipeau PC, and Vaughan TL (2010). Barrett’s oesophagus and oesophageal adenocarcinoma: time for a new synthesis. Nat Rev Cancer. 10: 87–101.
- Reya T, Morrison SJ, Clarke MF, and Weissman IL (2001). Stem cells, cancer, and cancer stem cells. Nature. 414: 105–11.
- Roesch A, Fukunaga-Kalabis M, Schmidt EC, Zabierowski SE, Brafford PA, Vultur A, Basu D, Gimotty P, Vogt T, and Herlyn M (2010). A temporarily distinct subpopulation of slow-cycling melanoma cells is required for continuous tumor growth. Cell. 141: 583–594.
- Roth S, Franken P, Van Veelen W, Blonden L, Raghoebir L, Beverloo B, Van Drunen E, Kuipers EJ, Rottier R, Fodde R, and Smits R (2009). Generation of a tightly regulated doxycycline-inducible model for studying mouse intestinal biology. Genesis. 47: 7–13.
- Roth S, Franken PF, Sacchetti A, and Fodde R (2011). Identification of quiescent and multipotent intestinal Paneth-like cells that are activated in response to tissue injury. submitted.
- Saito Y, Uchida N, Tanaka S, Suzuki N, Tomizawa-Murasawa M, Sone A, Najima Y, Takagi S, Aoki Y, Wake A, Taniguchi S, Shultz LD, and Ishikawa F (2010). Induction of cell cycle entry eliminates human leukemia stem cells in a mouse model of AML. Nat Biotechnol. 28: 275–280.
- Sangiorgi E and Capecchi MR (2008). Bmi1 is expressed in vivo in intestinal stem cells. Nat Genet. 40: 915–920.
- Saphner T, Tormey DC, and Gray R (1996). Annual hazard rates of recurrence for breast cancer after primary therapy. J Clin Oncol. 14: 2738–2746.
- Sargent DJ, Patiyil S, Yothers G, Haller DG, Gray R, Benedetti J, Buyse M, Labianca R, Seitz JF, O’callaghan CJ, Francini G, Grothey A, O’connell M, Catalano PJ, Kerr D, Green E, Wieand HS, Goldberg RM, and De Gramont A (2007). End points for colon cancer adjuvant trials: observations and recommendations based on individual patient data from 20,898 patients enrolled onto 18 randomized trials from the ACCENT Group. J Clin Oncol. 25: 4569–4574.
- Sato T, Van Es JH, Snippert HJ, Stange DE, Vries RG, Van Den Born M, Barker N, Shroyer NF, Van De Wetering M, and Clevers H (2011). Paneth cells constitute the niche for Lgr5 stem cells in intestinal crypts. Nature. 469: 415–418.
- Sato T, Vries RG, Snippert HJ, Van De Wetering M, Barker N, Stange De, Van Es JH, Abo A, Kujala P, Peters PJ, and Clevers H (2009). Single Lgr5 stem cells build crypt-villus structures in vitro without a mesenchymal niche. Nature. 459: 262–265.
- Shackleton M, Vaillant F, Simpson KJ, Stingl J, Smyth GK, Asselin-Labat ML, Wu L, Lindeman GJ, and Visvader JE (2006). Generation of a functional mammary gland from a single stem cell. Nature. 439: 84–88.
- Slack JM (2009). Metaplasia and somatic cell reprogramming. J Pathol. 217: 161–168.
- Snippert HJ, Van Der Flier LG, Sato T, Van Es JH, Van Den Born M, Kroon-Veenboer C, Barker N, Klein AM, Van Rheenen J, Simons BD, and Clevers H (2010). Intestinal crypt homeostasis results from neutral competition between symmetrically dividing Lgr5 stem cells. Cell. 143: 134–44.
- Sommers SC (1966). Mast cells and paneth cells in ulcerative colitis. Gastroenterology. 51: 841–50.
- Spotl L, Sarti A, Dierich MP, and Most J (1995). Cell membrane labeling with fluorescent dyes for the demonstration of cytokine-induced fusion between monocytes and tumor cells. Cytometry. 21: 160–9.
- Steinert R, Vieth M, Hantschick M, and Reymond MA (2005). Epithelial cells disseminate into the bone marrow of colorectal adenoma patients. Gut. 54: 1045–6.
- Takashima S, Mkrtchyan M, Younossi-Hartenstein A, Merriam JR, and Hartenstein V (2008). The behaviour of Drosophila adult hindgut stem cells is controlled by Wnt and Hh signalling. Nature. 454: 651–5.
- Tanaka T, Kohno H, Suzuki R, Hata K, Sugie S, Niho N, Sakano K, Takahashi M, and Wakabayashi K (2006). Dextran sodium sulfate strongly promotes colorectal carcinogenesis in Apc(Min/ +) mice: inflammatory stimuli by dextran sodium sulfate results in development of multiple colonic neoplasms. Int J Cancer. 118: 25–34.
- Teng C, Guo Y, Zhang H, Zhang H, Ding M, and Deng H (2007). Identification and characterization of label-retaining cells in mouse pancreas. Differentiation. 75: 702–12.
- Tsujimura A, Koikawa Y, Salm S, Takao T, Coetzee S, Moscatelli D, Shapiro E, Lepor H, Sun TT, and Wilson EL (2002). Proximal location of mouse prostate epithelial stem cells: a model of prostatic homeostasis. J Cell Biol. 157: 1257–65.
- Tumbar T, Guasch G, Greco V, Blanpain C, Lowry WE, Rendl M, and Fuchs E (2004). Defining the epithelial stem cell niche in skin. Science. 303: 359–63.
- Van Der Flier LG, Van Gijn ME, Hatzis P, Kujala P, Haegebarth A, Stange DE, Begthel H, Van Den Born M, Guryev V, Oving I, Van Es JH, Barker N, Peters PJ, Van De Wetering M, and Clevers H (2009). Transcription factor achaete scute-like 2 controls intestinal stem cell fate. Cell. 136: 903–12.
- Wada R (2009). Proposal of a new hypothesis on the development of colorectal epithelial neoplasia: nonspecific inflammation–colorectal Paneth cell metaplasia—colorectal epithelial neoplasia. Digestion. 79: Suppl 1, 9–12.
- Wada R, Yamaguchi T, and Tadokoro K (2005). Colonic Paneth cell metaplasia is pre-neoplastic condition of colonic cancer or not? J Carcinog. 4: 5.
- Weiss L and Ward PM (1990). Contributions of vascularized lymph-node metastases to hematogenous metastasis in a rat mammary carcinoma. Int J Cancer. 46: 452–5.
- Welm BE, Tepera SB, Venezia T, Graubert TA, Rosen JM, and Goodell MA (2002). Sca-1(pos) cells in the mouse mammary gland represent an enriched progenitor cell population. Dev Biol. 245: 42–56.
- Westbrook AM, Szakmary A, and Schiestl RH (2010). Mechanisms of intestinal inflammation and development of associated cancers: lessons learned from mouse models. Mutat Res. 705: 40–59.
- Wilson A, Laurenti E, Oser G, Van Der Wath RC, Blanco-Bose W, Jaworski M, Offner S, Dunant CF, Eshkind L, Bockamp E, Lio P, Macdonald HR, and TRUMPP A (2008). Hematopoietic stem cells reversibly switch from dormancy to self-renewal during homeostasis and repair. Cell. 135: 1118–29.
- Xie T (2009). Stem cell in the adult Drosophila hindgut: just a sleeping beauty. Cell Stem Cell. 5: 227–8.
- YUASA Y (2003). Control of gut differentiation and intestinal-type gastric carcinogenesis. Nat Rev Cancer. 3: 592–600.
- Zhu L, Gibson P, Currle DS, Tong Y, Richardson RJ, Bayazitov IT, Poppleton H, Zakharenko S, Ellison DW, and Gilbertson RJ (2009). Prominin 1 marks intestinal stem cells that are susceptible to neoplastic transformation. Nature. 457: 603–7.