Abstract
Multiple myeloma has been considered a weakly immunogenic malignancy that can cause profound defects in the immune system. An important issue for the immunotherapy of myeloma is the identification of appropriate tumor-associated antigens (TAAs). Recently, hTERT (human telomerase reverse transcriptase) was detected on a majority of human malignancies. In the studies reported here, we studied antigen-specific and HLA-A2-restricted cytotoxic activity against an ARH77 myeloma cell line in vitro. An HLA-A2-specific hTERT-derived nonapeptide (540ILAKFLHWL548) was used as a TAA. Myeloma-specific cytotoxic activity of hTERT-reactive cytotoxic lymphocytes (CTLs) was established by repeated stimulation of the CTLs via dendritic cells loaded with hTERT-derived nonapeptide. These studies were able to demonstrate that hTERT-reactive T-lymphocytes can be identified and expanded using relatively simple in vitro techniques consisting of antigen-specific stimulation, immunomagnetic sorting, and then induction of rapid expansion.
Keywords::
Introduction
Multiple myeloma is a hematologic cancer arising from post-germinal matured B-lymphocytes. The disease is characterized by an excess of monotypic plasma cells in the bone marrow secreting monoclonal immunoglobulins into the serum, a concomitant decrease in normal immunoglobulin levels, and an increase in the incidence of lytic bone lesions (Kuehl and Bergsagel, Citation2002). Multiple myeloma is a malignancy that is weakly immunogenic and that causes some dysfunctional responsiveness by the immune system. For example, patients with multiple myeloma may exhibit a variety of numerical and functional abnormalities of their T-lymphocytes, a reduction in the number of circulating precursors of myeloid and plasmacytoid dendritic cells (DCs), and a suppression of CD19+ B-lymphocytes (Pratt et al., Citation2007; Martín-Ayuso et al., Citation2008). This immune dysfunction is an important feature of the disease and may help to promote tumor growth and resistance to chemotherapy.
Telomeres are located at the distal ends of the chromosomes; the shortening of these with successive cycles of cell division leads to cell senescence and cell death (Blackburn, Citation1991; De Lange, Citation1994). Human telomerase is a ribonucleoprotein that consists of two components: the human telomerase reverse transcriptase (hTERT) and an RNA template (Shay et al., Citation2001). Except for male germ cells, activated lymphocytes, and certain stem cell populations (i.e., hema topoietic progenitor cells, basal keratinocytes), most adult somatic cells do not express hTERT (Keith et al., Citation2002). In cells where telomerase is activated, hTERT synthesizes a TTAGGG sequence from the RNA template that is added to the end of the shortening chromosome (Morin, Citation1989), saving the cells from death. This mechanism is exploited by tumor cells to maintain their immortality (Shay et al., Citation2001; Keith et al., Citation2002).
hTERT has been recognized as one of the universal tumor-associated antigens (TAAs), since >85% of all human cancers have telomerase activity (Kim et al., Citation1994). hTERT has also been proposed as a candidate for use in anti- tumor vaccinations (Huo et al., Citation2006). In fact, vaccinations using DCs pulsed with different TAAs have been shown to be effective in patients with different cancer types, including multiple myeloma, B-cell lymphoma, and malignant melanoma (Hsu et al., Citation1996; Nestle et al., Citation1998; Bendandi et al., Citation1999; Thurner et al., Citation1999; Timmerman and Levy, Citation1999). DCs are the most potent antigen-presenting cells (APCs) and have a unique ability to initiate primary immune responses of T-lymphocytes (Steinman, Citation1991; Porgador and Gilboa, Citation1995; Paglia et al., Citation1996; Zitvogel et al., Citation1996; Brossart et al., Citation1997; Banchereau and Steinman, Citation1998). Pilot studies have shown that the application of hTERT-specific DCs might induce immune responses in patients with renal cell carcinoma (Nair et al., Citation2000; Huo et al., Citation2006). Nair and colleagues also demonstrated that autologous hTERT-transfected DCs caused an inhibitory effect on the metastasis of other tumors (lung, breast, and bladder). Furthermore, the hTERT-specific CD8+ cytotoxic T-lymphocyte (CTL) response was recognized in HLA-A2-positive cancer patients (Filaci et al., Citation2006). The identification of these types of TAAs has opened up new opportunities for potential immunotherapies for multiple myeloma patients.
In this study, we analyzed antigen-specific HLA-A2-restricted cytotoxic activity against the ARH77 myeloma cell line in vitro using an HLA-A2-specific hTERT-derived nonapeptide (540ILAKFLHWL548) as a TAA. Activation of CTLs was then performed via hTERT-pulsed DCs.
Materials and methods
Cell isolation and cultures
Peripheral blood was collected from five HLA-A2+ healthy volunteers after they each had signed the informed consent form approved by our local Ethics Committee. Peripheral blood mononuclear cells (PBMCs) were isolated from the buffy coats of healthy donors using Histopaque 1077 (Sigma-Aldrich, Prague, Czech Republic) density gradient centrifugation. Isolated PBMCs were cultivated in X-VIVO 10 (BioWhittaker, Walkersville, MD) supplemented with 10% human AB-serum (Sigma-Aldrich), 80 U/mL DNAse (Boehringer, Mannheim, Germany), and 1 mM L-glutamine (Sigma-Aldrich). After 2 hours of culture at 37°C in an incubator with 5% CO2, cells were separated into adherent and nonadherent fractions. The nonadherent fraction that was rich in T-lymphocytes was incubated in complete medium containing X-VIVO 15 (BioWhittaker), 10% human AB-serum, and 10 IU interleukin (IL)-2/mL (Proleukin, Chiron, Amsterdam, The Netherlands). The 10 IU IL-2/mL was added to the cells every 2–3 days.
The adherent fraction with DC precursors was cultured for 6 days in X-VIVO 10 supplemented with 20 ng IL-4/mL (CellGenix, Freiburg, Germany) and 100 U GM-CSF/mL (CellGenix). The DC medium with cytokines was replaced every 2 days, and cell morphology was monitored with a light microscope (Bendandi et al., Citation1999; Thurner et al., Citation1999). After 6 days of DC culture, maturation agents were added: 10 ng tumor necrosis factor (TNF)-α/mL (Bender Medsystems Diagnostics, Vienna, Austria), 10 ng IL-1β/mL (CellGenix), 250 ng prostaglandin-E2/mL (Prostin, Pfizer Inc., New York), 1 μg TLR-ligand R848/mL (Invitrogen, Carlsbad, CA), and 5000 IU interferon (IFN)-γ/mL (Gentaur, Brussels, Belgium) (Vonderheide et al., Citation1999; Büchler et al., Citation2003; Jarnjak-Jankovic et al., Citation2007; Zobywalski et al., Citation2007). Cells were then harvested after overnight incubation.
Peptide antigens
An HLA-A2-specific hTERT-derived synthetic peptide (amino acids 540ILAKFLHWL548) (Proimmune, Oxford, UK) was used as a TAA. An HLA-A2-binding HIV-1 reverse transcriptase synthetic peptide (amino acids 476ILKEPVHGV484) was used as a negative control (Proimmune) (Brossart et al., Citation2000; Shay et al., Citation2001). Matured DCs were pulsed for 2 hours with 1 μg peptide/mL on Day 7 of the DC culture, as previously described (Brossart et al., Citation2000).
Stimulation and re-stimulation of T-lymphocytes
On Day 7, peptide-pulsed DCs were mixed with T-lymphocytes at the ratio 20:1 (T-lymphocytes:DCs). The remaining DCs were frozen and used for T-lymphocyte re-stimulation at the ratio 2:1 (T-lymphocytes:DCs) 7 days after the initial stimulation, as previously described (Ocadlikova et al., Citation2006).
IFNγ detection and immunomagnetic separation
Assessment of IFNγ production was performed using a Secretion Assay Cell Enrichment and Detection Kit (MACS Reagents, Miltenyi Biotec, Bergisch Gladbach, Germany) for both the experimental and control groups. Cell separation was performed 24 hours after re-stimulation of T-lymphocytes with peptide-pulsed DCs (hTERT or pol HIV-1). Following the manufacturer’s instructions, immunomagnetic beads were used for sorting within a VarioMACS cell separation system (MACS Reagents). The IFNγ+ T-lymphocytes were labeled with paramagnetic beads from the Secretion Assay Cell Enrichment and Detection Kit (Vonderheide et al., Citation1999). All solutions were kept on ice to prevent capping and patching of antibodies on the surface of labeled cells. To increase the purity, the IFNγ+ fraction containing the antigen-reactive T-lymphocytes was separated twice. The IFNγ+ fraction was than expanded in vitro. Cell purity was checked by flow cytometry.
Flow cytometry
Cells were stained using fluorescein isothiocyanate (FITC)-, phycoerythrin-cyanine 7 (PC7)-, or phycoerythrin (PE)-conjugated monoclonal antibodies against CD4, CD8, CD3, CD80, CD86, CD83, HLA-DR (all purchased from Immunotech, Marseille, France), and IFNγ (Miltenyi Biotec). Flow cytometry analyses were performed using a CytomicsTM FC 500 system (Beckman Coulter, Miami, FL).
Expansion of IFNγ+ hTERT-reactive T-lymphocytes
The IFNγ+ hTERT-reactive T-lymphocytes were expanded in vitro by treatment with 75 μg/mL of anti-CD3/anti-CD28 monoclonal antibodies (Dynabeads CD3/CD28 T-cell Expander, Invitrogen). Cultivated cells were plated (50 × 103 T-lymphocytes per well) into 96-well plates in complete medium supplemented with 500 IU IL-2/mL (Proleukin). Cultures were fed with fresh medium as needed, and IL-2 was added twice a week. Irradiated allogenic PBMCs (30 Gy) were added weekly.
Cytotoxicity assay
A T-lymphocyte-mediated cytotoxicity assay was performed with a LIVE/DEAD® Cell-Mediated Cytotoxicity Kit (Invitrogen). In order to assess their killing activity, hTERT-reactive T-lymphocytes (effector [E] cells) were mixed with different amounts of target [T] cells (to yield E:T ratios of 20:1 and 40:1) and then incubated for 4 hours at 37°C. The hTERT-positive ARH77 myeloma cell line (pre-labeled with 3,3′-dioctadecyloxacarbocyanine [DiOC], as previously described; Chang et al., Citation1993) was used as the specific target. To verify the specificity of the effector cells, as a negative control, third-party PBMCs were utilized as a non-specific target for the hTERT-specific CTLs. Dead target cells were detected using propidium iodide (PI) staining. Maximum target cell lysis was measured after irradiation (60 Gy) of ARH77 cells, and spontaneous target cell lysis was measured after incubation without effectors. The percentage cell-mediated cytotoxicity was calculated as: cytotoxicity (%) = 100 × (experimental target cell lysis − spontaneous target cell lysis)/(maximum target cell lysis − spontaneous target cell lysis)
Statistical analysis
Statistical analysis was performed with the software package SPSS 16.0© (SPSS Inc., Chicago, IL). Results were submitted with descriptive statistics. If not specified differently, all the results were reported as mean ± SD. Significance was estimated by nonparametric tests. Two independent sample analyses were performed with Mann–Whitney U-test. A Wilcoxon signed-rank test was used to compare the distributions of two related variables.
Results
Activation of T-lymphocytes by hTERT-pulsed dendritic cells
Dendritic cells were generated from PBMCs using GM-CSF and IL-4 and were activated by addition of TNFα, as previously described. Maturation of DCs with TNFα led to a high expression of CD80, CD86, and HLA-DR (≥87%) and to at least an intermediate expression of CD83 (≥26%) molecules on the cells. Matured DCs were loaded with nonapeptide antigen (540ILAKFLHWL548) derived from hTERT protein, and these hTERT-pulsed DCs served as stimulators of autologous CD4+ and CD8+ T-lymphocytes. After repeated stimulation of T-lymphocytes, the mean (±SD) levels of IFNγ+ subsets among all CD3+CD4+ and CD3+CD8+ populations reached 1.17% (±0.21%) and 1.16% (±0.24%), respectively. In the control group with HIV-1 peptide, the corresponding levels among all CD3+CD4+ and CD3+CD8+ cells was 0.28% (±0.019%) and 0.16% (±0.037%), respectively. These differences between the experimental and control groups were statistically very significant (at p < 0.01) ().
Figure 1. IFNγ production by hTERT-reactive T-lymphocytes. Matured dendritic cells were loaded with nonapeptide antigen (139TSAPDTRPA147) derived from hTERT protein. Autologous T-lymphocytes were stimulated with those antigen-pulsed DCs. Seven days after the initial stimulation, T-lymphocytes were re-stimulated with the same antigen-pulsed DCs. HIV-1-derived peptide (476ILKEPVHGV484) was used as a negative control. Activation of (A) CD3+CD4+ and (B) CD3+CD8+ T-lymphocytes in an experimental (hTERT) and control (HIV-1) group was measured by IFNγ production. Flow cytometry analysis was performed after 24 h of re-stimulation with hTERT- or HIV-1-pulsed DCs. Data are reported as mean (±SD). Significant differences between groups (at p < 0.01) are denoted with an asterisk.
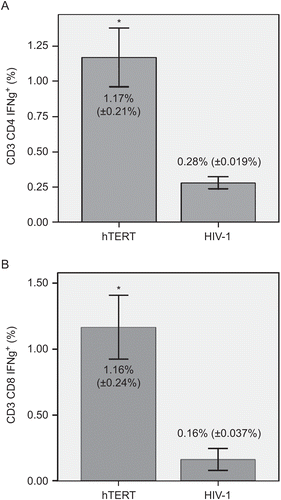
Immunomagnetic separation of hTERT-reactive T-lymphocytes
To enrich the hTERT-reactive population of T-lymphocytes, the IFNγ+ T-lymphocytes were separated in a magnetic field. Pre-purification levels of the IFNγ+ subsets of CD3+CD4+ and CD3+CD8+ T-lymphocytes were, as noted above, 1.17% and 1.16%, respectively. To increase the purity of hTERT-reactive T-lymphocytes, the IFNγ+ fraction was separated twice. After the double separation, the mean (±SD) levels of hTERT-reactive T-lymphocytes were very significantly (at p < 0.01) enriched to 63.59% (±10.8%) IFNγ+ CD3+CD4+ and 59.24% (±11.56%) IFNγ+ CD3+CD8+ T-lymphocytes. The negative fraction contained only < 0.6% IFNγ+ T-lymphocytes after these double immunomagnetic separations ().
Figure 2. Separation of hTERT-reactive T-lymphocytes. Comparison of IFNγ+ subsets of (A) CD3+CD4+ and (B) CD3+CD8+ T-lymphocytes in positive and negative fractions. Immunomagnetic separation was performed twice. Panels show percentages of IFNγ-producing cells of each subset. Data are shown as mean (±SD). Significant differences between groups (at p < 0.01) are denoted with an asterisk.
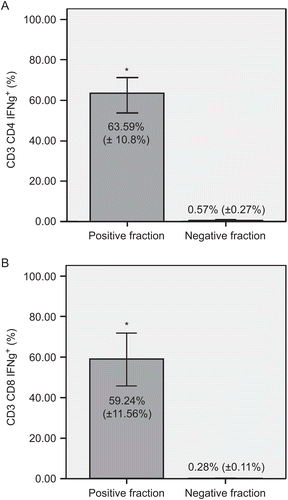
Expansion of IFNγ+ hTERT-reactive T-lymphocytes
Expansion of hTERT-reactive IFNγ+ T-lymphocytes after immunomagnetic separation was started with 0.1–0.3 × 106 T-lymphocytes. In all experiments, T-lymphocytes were successfully expanded to at least 1.0 × 107 T-lymphocytes within 8–12 days with treatments with anti-CD3/anti-CD28 beads.
Test of cytotoxicity
To verify the specificity of the hTERT-reactive T-lymphocytes, a cytotoxicity test with the ARH77 hTERT-positive myeloma cell line as target cells was performed. Here, the expanded hTERT-reactive T-lymphocytes were used as effector cells, and the specific cytotoxicity of the hTERT-reactive lymphocytes was measured using a flow cytometry–based system. At a ratio of 20:1 (E:T cells), the cytotoxic activity of the IFNγ+ T-lymphocytes against ARH77 targets reached a mean (±SD) value of 25.0% (±9.88%), compared with a level of nonspecific killing of allogenic PBMCs equal to 0.79% (±0.39%). At a ratio of 40:1, the IFNγ+ T-lymphocytes were able to kill 36.54% (±15.29%) of ARH77 hTERT- positive target cells, and cytotoxicity against allogenic PBMCs remained negligible (0.77% [±0.38%]) (). To further verify the specificity of the reactive T-lymphocytes, assays with a presumptive negative control (third-party PBMCs) were also performed. The specificity of the hTERT-specific CTLs was confirmed in that the level of killing in these studies was found never to exceed 1.18% (data not shown). In summary, the differences in cytolytic activity against the ARH77 hTERT-positive myeloma cell targets noted here between the experimental and control groups demonstrated the specific cytotoxic potential of the expanded hTERT-reactive T-lymphocytes in that they were able to recognize hTERT and kill target cells carrying this particular antigen.
Figure 3. Cytotoxic activity of hTERT-specific CTLs against the ARH77 myeloma cell line. T-lymphocyte-mediated cytotoxicity was measured using the expanded hTERT-reactive T-lymphocytes as effector (E) cells. DiOC-labeled hTERT-positive ARH77 myeloma cells were used as the target cells (T). To validate the specificity of the effector cells, PBMCs were used as a negative control target. Data are shown as mean (±SD) cytotoxic activities at the E:T ratios shown. Significant (at p < 0.05) differences between results with the different target cell types are denoted with an asterisk.
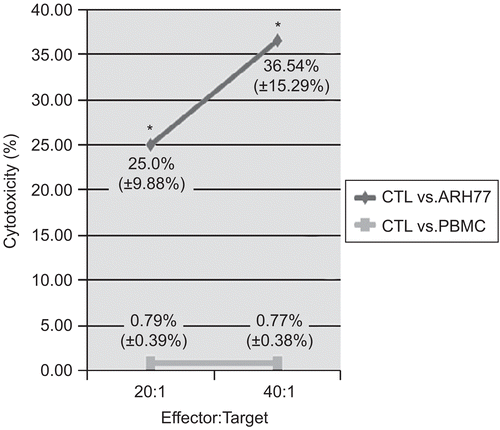
Discussion
Multiple myeloma has been regarded as a cancer with weak immunogenicity that causes defective functioning of the immune system, including DCs and T-lymphocytes (Pratt et al., Citation2007; Martín-Ayuso et al., Citation2008). Functional DCs are critical for optimal antigen presentation and for initiation of T-lymphocyte response. A significant increase of T-lymphocyte IFNγ production confirmed the immunogenic activity of hTERT-pulsed DCs.
CD8+ T-lymphocytes can differentiate into CTLs that are able to lyse TAA-expressing cells. Activation of CD8+ T-lymphocytes requires two steps: presentation of antigenic peptides on professional APCs and a helper function that is provided by CD4+ T-lymphocytes (Becker et al., Citation2001; Brossart et al., Citation2001; Bryant and Ploegh, Citation2004). In our study, the anti-tumor activity of hTERT-specific IFNγ+ T-lymphocytes was evaluated in vitro. Importantly, no signs of autoimmunity have been described in connection with the introduction of immune responses against hTERT. In this regard, hTERT-specific CTLs are not lysing either telomerase-positive CD34+ hematopoietic progenitor cells or activated T-lymphocytes in vitro (Vonderheide et al., Citation1999; Minev et al., Citation2000; Danet-Desnoyers et al., Citation2005).
Recently, it has been demonstrated that CTLs activated by specific tumor antigen and producing IFNγ can be captured and used for targeted immunotherapy of cancer. In our previous study we adapted this strategy further to demonstrate that activated IFNγ+ T-lymphocytes can be expanded to large numbers without loss of tumor specificity using phytomegglutinin (PHA) (Ocadlikova et al., Citation2006). PHA serves as a potent stimulus for T-lymphocyte expansion, but it has been also demonstrated that long-term repeated PHA stimulation of T-lymphocytes may lead to their exhaustion (Jaruga et al., Citation2000). Thus, short-term PHA stimulation or other strategies that employ anti-CD3/CD28 beads can be used for rapid clinical-grade T-lymphocyte expansion (Kalamasz et al., Citation2004).
Conclusion
In this study, we were able to demonstrate that hTERT-specific T-lymphocytes can be identified and expanded using a relatively simple in vitro technique consisting of antigen-specific stimulation via DCs, immunomagnetic sorting, and rapid expansion. Such an approach can be used clinically even in poorly immunogenic diseases such as multiple myeloma. In summary, hTERT-pulsed DCs could be a good candidate for future clinical trials. In the case of multiple myeloma, the hTERT-specific vaccination can be a great benefit, alerting the immune system to the tumor antigen and possible myeloma progression.
Acknowledgment
This study was graciously supported with funding from grants MSMT LC06027 and IGA MZCR 1A8709-5.
Declaration of interest: The authors report no conflicts of interest and are fully responsible for the content and writing of this paper.
References
- Banchereau, J., and Steinman, R. M. 1998. Dendritic cells and the control of immunity. Nature 392:245–252.
- Becker, C., Pohla, H., Frankenberger, B., Schuler, T., Assenmacher, M., Schendel, D. J., and Blankenstein, T. 2001. Adoptive tumor therapy with T-lymphocytes enriched through an IFNγ capture assay. Nat. Med. 7:1159–1162.
- Bendandi, M., Gocke, C. D., Kobrin, C. B., Benko, F. A., Sternas, L. A., Pennington, R., Watson, T. M., Reynolds, C. W., Gause, B. L., Duffey, P. L., Jaffe, E. S., Creekmore, S. P., Longo, D. L., and Kwak, L. W. 1999. Complete molecular remissions induced by patient-specific vaccination plus granulocyte-monocyte colony-stimulating factor against lymphoma. Nat. Med. 5:1171–1177.
- Blackburn, E. H. 1991. Structure and function of telomeres. Nature 350:569–573.
- Brossart, P., Goldrath, A. W., Butz, E. A., Martin, S., and Bevan, M. J. 1997. Adenovirus mediated delivery of antigenic epitopes into DC by a means of CTL induction. J. Immunol. 158:3270–3276.
- Brossart, P., Wirths, S., Stuhler, G., Reichardt, V. L., Kanz, L., and Brugger, W. 2000. Induction of cytotoxic T-lymphocyte responses in vivo after vaccinations with peptide-pulsed dendritic cells. Blood 96:3102–3108.
- Brossart, P., Schneider, A., Dill, P., Schammann, T., Grünebach, F., Wirths, S., Kanz, L., Bühring, H. J., and Brugger, W. 2001. The epithelial tumor antigen HTERT is expressed in hematological malignancies and is recognized by HTERT-specific cytotoxic T-lymphocytes. Cancer Res. 61:6846–6850.
- Bryant, P., and Ploegh, H. 2004. Class II MHC peptide loading by the professionals. Curr. Opin. Immunol. 16:96–102.
- Büchler, T., Hájek, R., Bourková, L., Kovarova, L., Musilova, R., Bulikova, A., Doubek, M., Svobodnik, A., Mareschova, I., Vanova, P., Tuzova, E., Vidlakova, P., Vorlicek, J., and Penka, M. 2003. Generation of antigen-loaded cells in a serum-free medium using different cytokine combinations. Vaccine 21:877–882.
- Chang, L., Gusewitch, G. A., Chritton, D. B., Folz, J. C., Lebeck, L. K., and Nehlsen-Cannarella, S. L. 1993. Rapid flow cytometric assay for the assessment of natural killer cell activity. J. Immunol. Meth. 166:45–54.
- Danet-Desnoyers, G. A., Luongo, J. L., Bonnet, D. A., Domchek, S. M., and Vonderheide, R. H. 2005. Telomerase vaccination has no detectable effect on SCID-repopulating and colony-forming activities in the bone marrow of cancer patients. Exp. Hematol. 33:1275–1280.
- De Lange, T. 1994. Activation of telomerase in a human tumor. Proc. Natl. Acad. Sci. USA 91:2882–2885.
- Filaci, G., Fravega, M., Setti, M., Traverso, P., Millo, E., Fenoglio, D., Negrini, S., Ferrera, F., Romagnoli, A., Basso, M., Contini, P., Rizzi, M., Ghio, M., Benatti, U., Damonte, G., Ravetti, J. L., Carmignani, G., Zanetti, M., and Indiveri, F. 2006. Frequency of telomerase-specific CD8+ T-lymphocytes in patients with cancer. Blood 107:1505–1512.
- Hsu, F. J., Benike, C., Fagnoni, F., Liles, T. M., Czerwinski, D., Taidi, B., Engleman, E. G., and Levy, R. 1996. Vaccination of patients with B-cell lymphoma using autologous antigen-pulsed dendritic cells. Nat. Med. 2:52–58.
- Huo, L. F., Tang, J. W., Huang, J. J., Huang, P. T., Huang, C. F., Kung, H. F., and Lin, M. C. 2006. Cancer immunotherapy targeting the telomerase reverse transcriptase. Cell. Mol. Immunol. 3:1–11.
- Jarnjak-Jankovic, S., Hammerstad, H., Saebøe-Larssen, S., Kvalheim, G., and Gaudernack, G. 2007. A full-scale comparative study of methods for generation of functional dendritic cells for use as cancer vaccines. BMC Cancer 7:119.
- Jaruga, E., Skierski, J., Radziszewska, E., and Sikora, E. 2000. Proliferation and apoptosis of human T-cells during replicative senescence—a critical approach. Acta Biochim. Pol. 47:293–300.
- Kalamasz, D., Long, S. A., Taniguchi, R., Buckner, J. H., Berenson, R. J., and Bonyhadi, M. 2004. Optimization of human T-cell expansion ex vivo using magnetic beads conjugated with anti-CD3 and anti-CD28 antibodies. J. Immunother. 27:405–418.
- Keith, W. N., Bilsland, A., Evans, J. T. R., and Glasspool, R. M. 2002. Telomerase-directed molecular therapeutics. Exp. Rev. Mol. Med. 4(10):1–25. http://www.expertreviews.org.
- Kim, N. W., Piatyszek, M. A., Prowse, K. R., Harley, C. B., West, M. D., Ho, P. L., Coviello, G. M., Wright, W. E., Weinrich, S. L., and Shay, J. W. 1994. Specific association of human telomerase activity with immortal cells and cancer. Science 266:2011–2015.
- Kuehl, W. M., and Bergsagel, P. L. 2002. Multiple myeloma: Evolving genetic events and host interactions. Nat. Rev. Cancer 2:175–187.
- Martín-Ayuso, M., Almeida, J., Pérez-Andrés, M., Cuello, R., Galende, J., González-Fraile, M. I., Martín-Nuñez, G., Ortega, F., Rodríguez, M. J., San Miguel, J. F., and Orfao, A. 2008. Peripheral blood dendritic cell subsets from patients with monoclonal gammopathies show an abnormal distribution and are functionally impaired. Oncologist 13:82–92.
- Minev, B., Hipp, J., Firat, H., Schmidt, J. D., Langlade-Demoyen, P., and Zanetti, M. 2000. Cytotoxic T cell immunity against telomerase reverse transcriptase in humans. Proc. Natl. Acad. Sci. USA 97:4796–4801.
- Morin, G. B. 1989. Human telomerase terminal transferase enzyme is a ribonucleoprotein that synthesizes TTAGGG repeats. Cell 59:521–529.
- Nair, S. K., Heiser, A., Boczkowski, D., Majumdar, A., Naoe, M., Lebkowski, J. S., Vieweg, J., and Gilboa, E. 2000. Induction of cytotoxic T-cell responses and tumor immunity against unrelated tumors using telomerase reverse transcriptase RNA-transfected dendritic cells. Nat. Med. 6:1011–1017.
- Nestle, F. O., Alijagic, S., Gilliet, M., Sun, Y., Grabbe, S., Dummer, R., Burg, G., and Schadendorf, D. 1998. Vaccination of melanoma patients with peptide- or tumor lysate-pulsed dendritic cells. Nat. Med. 4:328–332.
- Ocadlikova, D., Zahradova, L., Kovarova, L., Penka, M., Zaloudik, J., Buchler, T., Hajek, R., and Michalek, J. 2006. Isolation and expansion of allogeneic myeloma-specific interferon-gamma producing T-cells for adoptive immunotherapy. Med. Oncol. 23:377–384.
- Paglia, P., Chiodoni, C., Rodolfo, M., and Colombo, M. P. 1996. Murine dendritic cells loaded in vitro with soluble protein prime cytotoxic T-lymphocytes against tumor antigen in vivo. J. Exp. Med. 183:317–322.
- Porgador, A., and Gilboa, E. 1995. Bone-marrow-generated dendritic cells pulsed with a Class I-restricted peptide are potent inducers of cytotoxic T lymphocytes. J. Exp. Med. 182:255–260.
- Pratt, G., Goodyear, O., and Moss, P. 2007. Immunodeficiency and immunotherapy in multiple myeloma. Br. J. Haematol. 138:563–579.
- Shay, J. W., Zou, Y., Hiyama, E., and Wright, W. E. 2001. Telomerase and cancer. Human Mol. Genet. 10:677–685.
- Steinman, R. M. 1991. The dendritic cell system and its role in immunogenicity. Annu. Rev. Immunol. 9:271–296.
- Thurner, B., Haendle, I., Roder, C., Dieckmann, D., Keikavoussi, P., Jonuleit, H., Bender, A., Maczek, C., Schreiner, D., von den Driesch, P., Brocker, E. B., Steinman, R. M., Enk, A., Kampgen, E., and Schuler, G. 1999. Vaccination with MAGE-3A1 peptide-pulsed mature, monocyte-derived dendritic cells expands specific cytotoxic T-cells and induces regression of some metastases in advanced Stage IV melanoma. J. Exp. Med. 190:1669–1678.
- Timmerman, J. M., and Levy R. 1999. Dendritic cell vaccines for cancer immunotherapy. Annu. Rev. Med. 50:507–529.
- Vonderheide, R. H., Hahn, W. C., Schultze, J. L., Nadler, L. M. 1999. The telomerase catalytic subunit is a widely expressed tumor-associated antigen recognized by cytotoxic T-lymphocytes. Immunity 10:673–690.
- Zitvogel, L., Mayordomo, J. I., Tjandrawan, T., DeLeo, A. B., Clarke, M. R., Lotze, M. T., and Storkus, W. J. 1996. Therapy of murine tumors with tumor peptide-pulsed dendritic cells: Dependence on T-cells, B7 co-stimulation, and T-helper cell 1-associated cytokines. J. Exp. Med. 183:87–97.
- Zobywalski, A., Javorovic, M., Frankenberger, B., Pohla, H., Kremmer, E., Bigalke, I., and Schendel, D. J. 2007. Generation of clinical grade dendritic cells with capacity to produce biologically-active IL-12p70. J. Transl. Med. 5:18.