Abstract
Human natural killer (NK) cells are lymphocytes that destroy tumor cells, virally-infected cells, and antibody-coated cells. Tetrabromobisphenol A (TBBPA) is used both as a reactive and as an additive flame retardant in a variety of materials and appears to contaminate the environment. TBBPA has been found in human blood samples and if it interferes with NK cell function, this could increase the risk of tumor development and/or viral infection. The present study examines the effects of exposure to various concentrations of TBBPA for 24 hr, 48 hr, and 6 days on the lytic function, tumor-target-binding function, and ATP levels of NK cells. These same parameters were also monitored in NK cells that were exposed to TBBPA for 1 h followed by 24 hr, 48 hr, and 6 days in TBBPA-free media. A 24-h exposure of NK cells to 5 μM TBBPA caused a >95% decrease in NK lytic function, a 70% decrease in binding function, and a 34% decrease in ATP levels in NK cells. Exposure to 2.5 μM TBBPA for 24 h decreased lytic function by 76%, binding function by 20%, and had no effect on ATP levels. Exposure of NK cells to 5 μM TBBPA for 1 h followed by 24 h in TBBPA-free media caused a progressive and persistent loss of lytic function (41%) while not affecting either binding ability or ATP levels. The results indicate that TBBPA exposures decrease the lytic function of human NK cells and that an initial brief (1 hr) exposure can cause a progressive loss of function. In addition, the data also indicate that TBBPA-induced loss of NK lytic function can occur at a concentration of TBBPA that does not affect target-binding ability and ATP levels of NK cells.
Introduction
Tetrabromobisphenol A (TBBPA) is used as a flame retardant in a wide variety of materials including plastics, paper, and textiles (IPCS/WHO, Citation1995; HSBD, Citation2001). TBBPA has been used as a replacement for the polybrominated diphenylethers (PBDEs), whose persistence in the environment and potential for negative effects on health have prompted concerns (Sjodin et al., Citation1999; Eriksson et al., Citation2001). The main use of TBBPA is as a reactive flame retardant in epoxy resin circuit boards (IPCS/WHO, Citation1995; HSDB, Citation2001). However, it is also applied to carpeting and upholstery (Gain, Citation1997). TBBPA has additional uses as a plasticizer and as an intermediate in the production of other flame retardants (IUPCS/WHO, Citation1995; HSBD, Citation2001). In 1998, the annual United States; production of TBBPA was more than 1 million pounds with estimates ranging between 100 and 500 million pounds, (U.S. EPA, Citation2000a; Environ Defense, Citation2001). Its production and use have the potential to release TBBPA into the environment. It is a highly hydrophobic compound and would be expected to adsorb onto suspended solids and sediment in streams (U.S. EPA, Citation1999, 2002b). Its half-life in water is expected to be 48–84 days (U.S. EPA, Citation1999, Citation2000b). TBBPA is found in sewage sludge (Sellstrom and Jannson, Citation1995; de Boer et al., Citation2002; de Wit, Citation2002) and appears to bioaccumulate (IPCS/WHO, Citation1995; HSBD Citation2001; Birnbaum and Staskal, Citation2004).
TBBPA has been found in human serum samples (Hagmar et al., Citation2000; Nagayama et al., Citation2001; Thomsen et al., Citation2002). Levels reported were 0.24–0.71 ng/g lipid in serum samples from Norway (∼1.8–5.3 pM, using a conversion factor of 400 mg lipid/100 mL serum) (Thomsen et al., Citation2002) and averaged 4.5 ng/g lipid (∼33.8 pM) in a Japanese study (Nagayama et al., Citation2001). A toxicokinetic study following a single oral dose of 300 mg/kg TBBPA to adult humans showed a peak plasma concentration of 103 μM within 3 h that declined, with an elimination half-life of 13 h (Schauer et al., Citation2006). Human exposure to TBBPA is believed to occur by inhalation, dermal contact, and ingestion (IPCS/WHO, Citation1995; Peterman et al., Citation2000). It has been found in commercial drinking water that was stored in polycarbonate containers (Peterman et al., Citation2000) and in seafood (IPCS/WHO, Citation1995). There has been little study of the toxicological effects of TBBPA on humans. However, there have been studies of its effects in rodents and rainbow trout. TBBPA was shown to increase the activity of glutathione reductase in rainbow trout (Ronisz et al., Citation2004). In mice, it has been shown to cause decreases in serum proteins and red blood cells, as well as increases in spleen weight (IPCS/WHO, Citation1995). The administration of TBBPA to newborn rats caused polycystic kidney lesions (Fukuda et al., Citation2004). The administration of TBBPA to Wistar rats showed effects on circulating thyroid hormone T4 levels (Van der Ven et al., 2008). This same study showed that there were no effects of TBBPA exposure on the function of splenic natural killer (NK) cells in exposed rats or their offspring (Van der Ven et al., 2008). In vitro studies of TBBPA showed that it was able to compete with thyroid hormone T4 for binding to human transthyretin (thyroid hormone transport protein) (Meerts et al., Citation2000).
Human NK cells are immune system cells (lymphocytes) that are capable of destroying tumor cells, virally-infected cells, and antibody-coated cells. NK cells have the markers CD56 and/or CD16 on their cell surface and lack the T-lymphocyte receptor/CD3 complex (Lotzova, Citation1993). NK cells are a crucial immune defense against malignant neoplasms and viral infections (Lotzova, Citation1993; Vivier et al., Citation2004). Their role in protecting against viral infections is demonstrated by increased incidences of viral infection in individuals that lack NK cells (Fleisher et al., Citation1982; Biron et al., Citation1989). Unlike T-lymphocytes, NK cells lyse (destroy) appropriate target cells without prior sensitization, making them a frontline immune defense. Thus, if a compound such as TBBPA were to alter NK cell function, this could result in increased risk of viral infection and tumor formation. A recent study has shown that another flame retardant inhibits the function of human NK cells (Hinkson and Whalen, Citation2009). Suppression of human NK cell function by contaminants such as these may account, in part, for increases in cancer incidence especially those seen in lymphomas and leukemias (CitationSEER, 2009). Thus, it is important to address the capacity of TBBPA and other environmental contaminants to diminish the function of human NK cells.
In the present study, TBBPA was examined for its potential to disrupt the lytic function of NK cells. Both acute (i.e., 1 h) and chronic (as long as 6 days) exposures were examined. The brief (1 h) exposures were followed by periods of time in TBBPA-free media before assessing NK function (1-h exposures, followed by up to 6 days in TBBPA-free media). These types of studies were important in that lasting (or developing) negative effects from a brief exposure to TBBPA would suggest greater risk from exposure to the compound. Finally, the effects of TBBPA on the ability of NK cells to bind to their target cells and on the intracellular ATP levels in NK cells were studied. Binding of NK cells to targets is a necessary step in the lytic process and interference with binding could lead to loss of lytic function (Lotzova et al., Citation1993). In addition, NK lytic function is an ATP-dependent process (Andzelm et al., Citation2007), and decreases in ATP could lead to loss of lytic function.
Materials and methods
Isolation of NK cells
Peripheral blood from healthy adult (male and female) volunteer donors was used for this study. Buffy coats (source leukocytes) obtained from the American Red Cross (Portland, OR) or Key Biologics, LLC (Memphis, TN) were used to prepare NK cells. Highly purified NK cells were obtained using a rosetting procedure. Buffy coats were mixed with 0.8 mL of RosetteSep human NK cell enrichment antibody cocktail (StemCell Technologies, Vancouver, British Columbia, Canada) per 45 mL of buffy coat. The mixture was incubated for 20 min at room temperature (∼25°C). Following the incubation, 7–8 mL of the mixture was layered onto 4 mL of Ficoll-Hypaque (1.077 g/mL; MP Biomedicals, Irvine, CA) and centrifuged at 1200 xg for 30–40 min. The cell layer was then collected and washed twice with phosphate-buffered saline (PBS; pH 7.2) and stored in complete media (RPMI-1640 supplemented with 10% heat-inactivated bovine calf serum [BCS], 2 mM L-glutamine, and 50 U penicillin G/50 μg streptomycin/mL) at 1 million cells/mL (Whalen et al., Citation2002).
Chemical preparation
TBBPA (purchased from Fisher Scientific, 97% pure) was dissolved in dimethyl sulfoxide (DMSO) (Sigma-Aldrich, St. Louis, MO) to yield a 100 mM stock solution. Desired concentrations of TBBPA were then prepared by dilution of the stock into complete media containing 0.5% gelatin in the place of 10% bovine serum. Gelatin was used in place of bovine serum to avoid binding of TBBPA to the serum’s albumin, which could interfere with its delivery to the cells. The final concentration of DMSO in any of the TBBPA exposures did not exceed 0.01%.
Cell treatments
NK cells (at a concentration of 1.5 × 106 cells/mL) were exposed to TBBPA or control (DMSO-bearing medium) for 24 h, 48 h, or 6 days. Following exposures, the cells were assayed for tumor-destroying function, binding function, and/or ATP levels. In addition, NK cells were exposed to TBBPA for 1 h; following the 1-h exposure period, the TBBPA-containing or control media were removed and replaced with fresh TBBPA-free media, and the cells were incubated in compound-free media for 24 h, 48 h, or 6 days before being assayed for tumor-destroying function, binding function, and/or ATP levels. The concentration range examined was 0.05–10 μM TBBPA.
Cell viability
Cell viability was determined by trypan blue exclusion. Cell numbers and viability were assessed at the beginning and end of each exposure period. Viability was determined at each concentration for each of the exposure periods. The viability of treated cells was then compared to that of control cells at each length of exposure (Whalen et al., Citation2003). Only those concentrations where viability was unaffected were used at a given length of exposure. Viability was 100% of that seen in the control NK cells at the concentrations used at a specific exposure time.
Cytotoxicity assay
The ability of NK cells to lyse tumor cells was measured using a 51Cr release assay (Whalen et al., Citation1999). The target cell in all cytotoxicity assays was the NK-susceptible K562 (human chronic myelogenous leukemia) cell line. Briefly, K562 cells were incubated with 51Cr (Perkin-Elmer Life Sciences, Boston, MA) in 0.2–0.5 mL of unadulterated BCS (BCS containing no additions) for 1–1.5 h at 37°C in an air/CO2 (19:1) incubator. Following this incubation, the target cells were washed twice with gelatin media. NK (effector) cells (1.2 × 105/100 μL, for 12:1 ratio with target cells) were added to the wells of round-bottom microwell plates. The effector cells were diluted to a 6:1 (0.6 × 105/100 μL) and a 3:1 ratio (0.3 × 105/100 μL); each ratio was tested in triplicate. Target cells were added (1 × 104/100 μL) to each well of the microwell plate; the plate was then centrifuged at 300 xg for 3.5 min and incubated for 2 h at 37°C (air/CO2, 19:1). After this incubation, a 0.1 mL aliquot of supernatant was collected from each well and each assessed for radioactivity (in 60 sec) in a Packard COBRA γ-radiation counter (Packard Instrument Co., Meriden, CT). Target lysis was then calculated as follows: 100 × [test cpm − spontaneous cpm]/[maximum cpm − spontaneous cpm]. Maximum release was produced by adding 100 μL of 10% Triton X-100 to wells containing only the labeled target cells; spontaneous release was assessed from wells containing cells plus medium only.
Binding assay
The percentage of target cells with bound NK cells was determined at two effector/target ratios, i.e., 12:1 and 6:1. The NK cells were treated as described earlier for the cytotoxicity assay. Tumor cells were then incubated with an appropriate number of control or TBBPA-exposed lymphocytes in round-bottomed microwell plates (20,000 tumor cells/well). Each condition was tested in triplicate. The cells were incubated at 37°C (air/CO2, 19:1) for 10 min and then placed on ice. Following the incubation period, the cells were gently resuspended with a micropipette, placed in a hemocytometer, and viewed under a light microscope. The number of target cells with two or more lymphocytes bound to their surface was counted as well as the total number of targets to determine the percentage of tumor cells with lymphocytes bound. The minimum number of targets counted per determination was 50–100 (Whalen et al., Citation1999).
ATP assay
Following exposures of cells to the various concentrations of TBBPA, 250,000 NK cells were added to 300 μL of somatic cell ATP releasing reagent (Sigma-Aldrich). To measure ATP, 100 μL of lysed cell suspension was added to 100 μL of a Luciferin/Luciferase mixture (Sigma). The light emission was then measured using a Fluoroskan Ascent FL luminescence plate reader (Thermo Electron Corp., Vantaa, Finland). ATP levels were then determined by extrapolation from a standard curve (Dudimah et al., Citation2007b).
Statistical analysis
The data from TBBPA-treated cells at a given concentration were compared to control data by pairwise analysis using Student’s t-test (, , , and ). Two-way ANOVA and post-hoc linear regression were used to evaluate the effect of length of exposure at a given concentration of TBBPA in and .
Results
Lytic function and binding function of NK cells exposed to TBBPA for 24 h, 48 h, and 6 days
Exposures of NK cells to 2.5 and 5 μM TBBPA for 24 h caused very significant reductions in NK cell lytic function of 75.8 ± 6.6% and 96.2 ± 3.1%, respectively (P < 0.0001) (). The decrease in lytic function after a 24-h exposure to 1 μM TBBPA was 38.6 ± 10.7% (P < 0.001), while that at 0.5 μM was 23.3 ± 11.2% (P < 0.001). The binding function of NK cells exposed to 2.5 and 5 μM TBBPA for 24 h was reduced by 19.1 ± 14.3% and 69.9 ± 16.6%, respectively (P < 0.0001) (). NK cells exposed to 1 μM TBBPA for 24 h showed no loss of binding function.
NK cells were exposed to concentrations of 0.25–2.5 μM TBBPA for 48 h because higher concentrations caused decreased viability of the NK cells. Exposure at all of the concentrations tested except 0.25 μM TBBPA caused significant decrease in the lytic function of NK cells. The decreases were 31.0 ± 9.8% at 0.5 μM, 56.0 ± 7.1% at 1 μM, and 86.1 ± 6.3% at 2.5 μM (P < 0.0001) (). NK cells that were exposed to concentrations below 1 μM TBBPA showed no loss of binding function. A 48-h exposure to 1 μM TBBPA caused a small loss of binding function of 12.2 ± 11.0% (P < 0.05). Exposure to 2.5 μM TBBPA for 48 h caused a decrease in binding function of 51.2 ± 18.7% (P < 0.0001) ().
The 6-day exposures of NK cells to TBBPA were carried out at concentrations of 0.05–1 μM. There was a significant decrease in tumor-cell-lysing function with a 6 days exposure to all of the concentrations of TBBPA except the 0.05 μM (). TBBPA caused decreases in lytic function of 20.3 ± 16.0% at 0.1 μM, 33.2 ± 19.1% at 0.25 μM, 63.7 ± 21.4% at 0.5 μM, and 89.2 ± 16.0% at 1 μM (P < 0.01) (). NK cells exposed to 0.25–1 μM TBBPA for 6 days showed a significant decrease in binding function (33.2 ± 23.7%, P < 0.01) only with the 1 μM exposure ().
Figure 1. Effects of TBBPA exposures on the ability of NK cells to lyse or bind tumor cells. NK cells were exposed to 0.05–5 μM TBBPA for (A) 24 h, (B) 48 h, or (C) 6 days, and the tested for lytic function (closed diamonds) or binding function (closed squares). To combine results from separate experiments (using cells from different donors), levels of lysis or binding were normalized as the percentage of the lytic or binding function of the control cells (untreated cells from the same donor) in a given experiment. Results were pooled from three separate experiments using different donors (triplicate determinations for each experiment; n = 9; mean ± SD). Significant decreases in Lytic Function as compared to control: (A) 0.5, 1, 2.5, and 5 μM (P < 0.001); (B) 0.5, 1, and 2.5 μM (P < 0.0001); and (C) 0.1, 0.25, 0.5, and 1 μM at (P < 0.01). Significant decreases in Binding Function as compared to control: (A) 2.5 and 5 μM (P < 0.0001); (B) 1 and 2.5 μM (P < 0.05); and (C) 1 μM (P < 0.01).
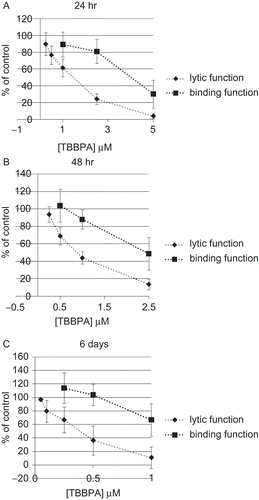
plots the lytic function of NK cells exposed to 0.5 and 1 μM TBBPA as a function of length of exposure (using the data from ). Only the 0.5 and 1 μM TBBPA concentrations are shown as these are the only concentrations that were tested at all three lengths of exposure. This is done to show that there appears to be an increased loss of lytic function with length of exposure at a given concentration of TBBPA, when comparing the 24-h exposures to the 6-day exposures (P < 0.01).
Lytic function and binding function of NK cells exposed to TBBPA for 1 h, followed by 0 h, 24 h, 48 h, and 6 days in TBBPA-free medium
To assess whether a brief exposure of NK cells to TBBPA could cause lasting and/or ensuing affects on lytic or binding function, NK cells were exposed to 0.5–10 μM TBBPA for 1 h which was removed and the NK cells were then suspended in TBBPA-free media for 0 h, 24 h, 48 h, or 6 days before assaying for lytic function or binding function. NK cells exposed to 10 μM for 1 h and then tested immediately for lytic function (0 h in TBBPA-free media) exhibited a statistically significant decrease in lytic function of 30.1 ± 12.5% (P < 0.001), lower concentrations caused no significant decreases in lytic function. When NK cells were exposed to 0.5–10 μM, TBBPA for 1 h and then incubated in TBBPA-free media for 24 h before assaying for lytic function, decreases in lytic function of 22.4 ± 9.9% (1 μM), 27.0 ± 11.3% (2.5 μM), 41.4 ± 10.9% (5 μM), and 53.9 ± 15.0% (10 μM) (P < 0.001) were seen (). The binding function of NK cells exposed to TBBPA for 1 h, followed by 24 h in TBBPA-free media before assaying for binding function was unaffected by any of the concentrations tested ().
NK cells exposed to these same concentrations of TBBPA and incubated for 48 h in TBBPA-free media before assay showed reductions in lytic function of 18.3 ± 1 8.9% (2.5 μM), 22.6 ± 14.7% (5 μM), and 37.4 ± 11.7% (10 μM) (P < 0.05) (). The binding function of NK cells exposed to TBBPA for 1 h, followed by 48 h in TBBPA-free media before assaying for binding function was unaffected by any of the concentrations tested ().
In NK cells exposed to these same concentrations of TBBPA for 1 h, followed by a 6-day period in TBBPA-free media, lytic function decreased 33.6 ± 23.5% (2.5 μM), 41.2 ± 9.9% (5 μM), and 64.3 ± 6.7% (10 μM) (P < 0.01) (). NK cells that were exposed to 10 μM TBBPA for 1 h and then given 6 days in TBBPA-free media before assaying for binding function showed a loss of binding function of 49.6 ± 8.4% (P < 0.0001) (). All other concentrations tested 6 days following 1-h exposures to TBBPA showed no loss of binding function ().
Figure 3. Effects of a 1-h exposure to TBBPA followed by various periods in TBBPA-free media on the ability of NK cells to lyse or bind tumor cells. NK cells were exposed to 0.5–10 μM TBBPA for 1 h, and then tested for lytic function (closed diamonds) or binding function (closed squares) after (A) 24 h, (B) 48 h, or (C) 6 days. Results were normalized as described in . Significant decreases in Lytic Function as compared to control: (A) 1, 2.5, 5, and 10 μM (P < 0.001); (B) 2.5, 5, and 10 μM (P < 0.05); (C) 2.5, 5, and 10 μM at (P < 0.01). Significant decreases in Binding Function as compared to control: (A) none; (B) none; and (C) 10 μM (P < 0.0001).
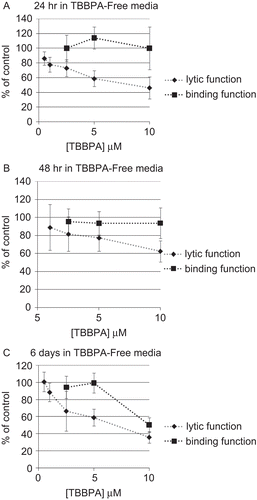
plots the results of 1-h exposures to 5 and 10 μM TBBPA followed by 0 h, 24 h, 48 h, and 6 days in TBBPA-free media as a function of length of time in TBBPA-free media (such as was carried out in ). There again appears to be an increasing loss of lytic function with time after a 1-h exposure (P < 0.01).
ATP levels in NK cells exposed to TBBPA for 24 h, 48 h, and 6 days
NK cells exposed to TBBPA for 24 h showed no significant decreases in ATP levels except at 5 μM where ATP was decreased by 33.5 ± 12.6% (P < 0.0001) (). Lytic function data from were placed on the plots of ATP changes () in order to facilitate the comparison of changes in ATP levels with those of lytic function. Reduction in ATP levels of 16.8 ± 9% and 32.7 ± 8.4% were seen with 48-h exposures to 1 and 2.5 μM hexabromocylododecane (HBCD), respectively (P < 0.0001) (). A 6-day exposure of NK cells to 0.25, 0.5, and 1 μM HBCD led to decreases in ATP levels of 38.4 ± 15%, 55.6 ± 29.6%, and 78.3 ± 27.2%, respectively (P < 0.001) ().
Figure 5. Effects of TBBPA exposures on the ATP levels and lytic function of NK cells. NK cells were exposed to 0.05–10 μM TBBPA for (A) 24 h, (B) 48 h, or (C) 6 days, and then tested for ATP levels (closed squares) or lytic function (closed diamonds). Results were normalized as described in . Significant decreases in ATP levels as compared to control: (A) 5 μM (p < 0.0001); (B) 1 and 2.5 μM (P < 0.0001); and (C) 0.25, 0.5, and 1 μM (P < 0.001). Significant decreases in Lytic Function as compared to control: (A) 0.5, 1, 2.5, and 5 μM (P < 0.001); (B) 0.5, 1, and 2.5 μM (P < 0.0001); and (C) 0.1, 0.25, 0.5, and 1 μM at (P < 0.01).
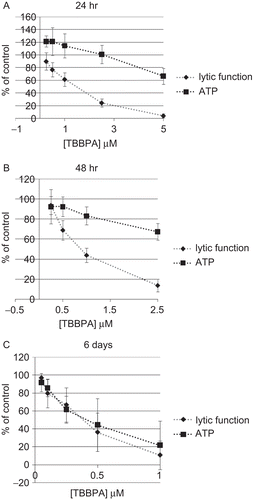
Effects of exposures to TBBPA for 1 h followed by 24 h, 48 h, and 6 days in TBBPA-free media on ATP levels in NK cells
As with lytic function and binding function, it is possible that an initial exposure to TBBPA for 1 h could cause changes in NK cell ATP levels that would manifest later, even though the compound has been removed. Thus, we examined whether the effects of exposures to TBBPA intensified following the removal of the compound. To address this possibility, NK cells were exposed to 0.5–10 μM TBBPA for 1 h that was then removed, and the NK cells were then suspended in TBBPA-free media for 24 h, 48 h, or 6 days before assaying for ATP levels. NK cells exposed to TBBPA for 1 h and then incubated for 24 h in TBBPA-free media before measuring ATP levels showed no significant decreases in ATP levels (). As was performed earlier, lytic function data from were placed on the plots of ATP changes () in order to facilitate comparison of changes in ATP levels with those of lytic function. NK cells exposed to 2.5, 5, and 10 μM TBBPA for 1 h and given a 48-h period in TBBPA-free media before assay showed very small decreases in ATP levels (P < 0.05) (). A 1-h exposure of NK cells to 1–10 μM TBBPA followed by 6 days in TBBPA-free media lead to decreases in ATP of about 30% at the 1–5 μM concentrations of TBBPA and of 43.6 ± 39.6% at 10 μM (P < 0.01) ().
Figure 6. Effects of TBBPA exposures on the ATP levels and lytic function of NK cells. NK cells were exposed to 0.5–10 μM TBBPA for 1 h, followed by periods of time in TBBPA-free media before assaying for ATP levels (closed squares) or lytic function (closed diamonds). (A) 24 h, (B) 48 h, or (C) 6 days. Results were normalized as described in . Significant decreases in ATP Levels as compared to control: (A) none; (B) 2.5, 5, and 10 μM (P < 0.05); and (C) 1, 2.5, 5, and 10 μM (P < 0.01). Significant decreases in Lytic Function as compared to control: (A) 1, 2.5, 5, and 10 μM (P < 0.001); (B) 2.5, 5, and 10 μM (P < 0.05); (C) 2.5, 5, and 10 μM (P < 0.01).
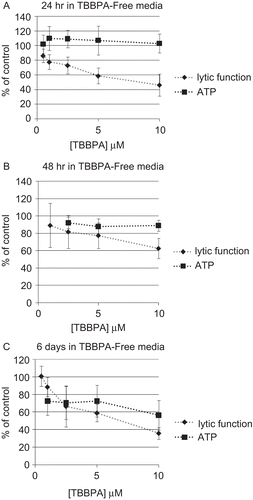
Discussion
Chemically-induced diminution of human NK cell immune function could leave an individual vulnerable to malignant neoplasias and/or viral infection. Previous studies have indicated that humans with a defect that leaves their NK cells unable to lyse target cells have a predisposition to development of certain tumors (Ortaldo et al., Citation1992) and that individuals lacking NK cells had severe herpes virus infection (Biron et al., Citation1989) and Epstein–Bar infections (Fleisher et al., Citation1982). Studies in mice have shown that a decrease in NK cell activity of 80% results in an increase in tumor formation upon challenge with melanoma cells (Wilson et al., Citation2001). Decreased NK cell function and increased tumor metastases have been demonstrated in mice exposed to cigarette smoke (Lu et al., Citation2007) and exposure of mice to the chemical urethane causes tumors and has been shown to suppress NK function (Luebke et al., Citation1986).
A large number of chemicals currently contaminate the environment and are found in human tissues (Thornton et al., Citation2002). The use of TBBPA as a flame retardant and plasticizer (IPCS/WHO, Citation1995; HSBD, Citation2001) has resulted in its being found in human blood samples (Hagmar et al., Citation2000; Nagayama et al., Citation2001; Thomsen et al., Citation2002). While it appears to be eliminated to some extent from the human system (Schauer et al., Citation2006), its detection in human blood samples indicates that a certain fraction accumulates in the body. Levels of TBBPA in human serum of 4.5 ng/g lipid (33.8 pM, using 400 mg lipid per 100 mL serum) have been reported (Nagayama et al., Citation2001). We examined the effects of TBBPA exposures on the lytic function of human NK cells. Further, the ability of an initial exposure to TBBPA to cause lasting and/or ensuing disruption of lytic function was examined, as a lasting disruption of NK function by a brief encounter with TBBPA would indicate a greater level of potential harm from exposure to this compound. The mechanism of TBBPA-induced decreases in lytic function was also addressed by examining the effects of TBBPA on the ability of NK cells to bind to tumor target cells and on ATP levels in NK cells. NK cells must first bind to target cells before they can initiate the process by which the target is lysed (Lotzova, Citation1993; Vivier et al., Citation2004). ATP plays an important role in lysis of tumor cells by NK cells (Andzelm et al., Citation2007).
A 24-h exposure to as little as 0.5 μM TBBPA caused a significant loss of lytic function in NK cells. When the concentration was 5 μM there was a nearly complete loss of lytic function at 24 h. These decreases in lytic function were not accompanied by comparable losses in binding function. While exposure to 2.5 μM TBBPA for 24 h caused a loss of lytic function of about 76% the loss of binding function was only 19%. Thus, these studies indicate that the TBBPA-induced loss of lytic function cannot be accounted for simply by TBBPA causing reduced ability of the NK cells to bind to their tumor targets.
An important finding of the current study was that a brief exposure to TBBPA could cause alteration(s) of the NK cell such that the loss of lytic function persisted and/or developed after the compound was removed. An exposure to 10 μM TBBPA for 1 h caused a 30% loss of lytic function while lower concentrations had no effect on lytic function. When cells were tested 24 h following the 1-h exposures, there was a 54% loss of lytic function at 10 μM TBBPA. A 1-h exposure to 5 μM TBBPA caused no loss of lytic function; however, 24 h after that exposure, the NK cells had lost 41% of their lytic ability. There was no loss of ability of NK cells to bind to target cells 24 h after a 1-h exposure to any of the concentrations of TBBPA that were tested. Thus, as was seen with continuous exposures to TBBPA loss of lytic function was not accompanied by a concomitant loss of binding function.
Since a loss of binding function could not account for the losses of lytic function seen with TBBPA exposures, we went on to examine whether TBBPA caused decreases in the intracellular ATP levels of NK cells. As mentioned earlier, ATP is required for the release of cytotoxic proteins from the NK cells onto target cells (Andzelm et al., Citation2007). As with binding function we found that a decrease in ATP levels did not necessarily accompany a loss of lytic function in TBBPA-exposed NK cells. For instance, a 24-h exposure to 2.5 μM TBBPA caused a nearly 80% loss of lytic function without having any effect on ATP levels. We did find that after a 6-day exposure to TBBPA the loss of lytic function was associated with a decrease in ATP levels.
The present study indicates that exposures to TBBPA can cause very significant losses of human peripheral blood NK cell lytic function. However, a recent study in Wistar rats found that in vivo exposure to TBBPA had no effect on the function of splenic NK cells in exposed rats or their offspring (Van der Ven et al., 2008). As mentioned, this study examined the function of NK cells from the spleen and did not examine peripheral blood NK cells. A previous study indicated that in vivo exposure of rats to the chemical carcinogens, diethylnitrosamine and 2-acetylaminofluorene, decreased the function of peripheral blood NK cells, but had no effect on splenic NK cells (Leu et al., Citation1985). Thus, the difference between splenic and peripheral blood NK cells may be a potential explanation for the fact that TBBPA dramatically decreased human NK cell lytic function but did not decrease the NK function in rats. In addition, species differences in susceptibility to TBBPA as well as exposure levels may also account for the differing results between rats and humans.
Previous studies have shown that a variety of environmental contaminants cause decreases in NK cell functions that persist once the compound is removed. These include another brominated flame retardant, hexabromocylododecane (HBCD) (Hinkson and Whalen, Citation2009); several organotin compounds (tributyltin [TBT], dibutyltin [DBT], and triphenyltin [TPT]) (Whalen et al., Citation2002, Citation2003; Dudimah et al., Citation2007a,Citationb); an organochlorine compound, pentacholorphenol (PCP); and a carbamate compound, ziram (Taylor et al., Citation2005). As with TBBPA, neither a decrease in target binding nor a decrease in ATP levels could fully account for the losses of lytic function seen with many of these compounds (HBCD, TBT, DBT, PCP, ziram) (Whalen et al., Citation1999, Citation2002; Taylor et al., Citation2005; Dudimah et al., Citation2007a,Citationb; Hinkson and Whalen, Citation2009; Taylor and Whalen, Citation2009). However, as seen with TBBPA a decrease in ATP appears to be associated with the loss of function seen after 6 days with some of these compounds (HBCD, TBT, DBT, and PCP) (Dudimah et al., Citation2007a,Citationb; Nnodu and Whalen, Citation2008; Hinkson and Whalen, Citation2009).
While significant decreases in NK lytic function were seen in human NK cells exposed to TBBPA, the concentrations at which these losses of function were seen were high compared to what has been measured in human serum (Nagayama et al., Citation2001). However, because the effects of TBBPA exposure do not subside once the compound is removed, there may be potential for cumulative effects over time.
In summary, the data indicate that the exposure of NK cells to TBBPA can cause very significant losses of NK lytic function. Further, loss of lytic function occurs after a brief (1 h) exposure, indicating that the process(es) is/are stimulated during a brief exposure that lead to a sustained loss of lytic function. TBBPA has effects on the ability of NK cells to bind to their targets and also is able to decrease intracellular levels of ATP under certain conditions. However, decreases in binding or in ATP levels cannot explain the losses of lytic function seen under several conditions. The current study shows that TBBPA interferes with the essential immune function of NK cells and thus has the potential for increasing the risk of cancer development in exposed individuals.
Acknowledgements
This research was supported by Grant 2S06GM-08092-34 from the National Institutes of Health.
Declaration of interest: The authors report no conflicts of interest. The authors alone are responsible for the content and writing of the paper.
References
- Andzelm, M. M., Chen, X., Krzewski, K., Orange, J. S., and Strominger, J. L. 2007. Myosin IIA is required for cytolytic granule exocytosis in human NK cells. J. Exp. Med. 204:2285–2291.
- Birnbaum, L. S., and Staskal, D. F. 2004. Brominated flame retardants: Cause for concern? Environ. Health Perspect. 112:9–17.
- Biron, C. A., Byron, K. S., and Sullivan, J. L. 1989. Severe herpes virus in an adolescent without natural killer cells. New Engl. J. Med. 320:1731–1735.
- de Boer, J., Allchin, C., Zegers, B., Boon, J. P., Brandsma, S. H., Morris, S., Kruijt, A. W., van der Hesselingen, J. M., and Haftka, J. J. 2002. HBCD and TBBP-A in Sewage Sludge, Sediments and Biota, Including Inter-laboratory Study. CO33/02. Wageningen, The Netherlands: RIVO-Netherlands Institute for Fisheries Research, p. 40.
- de Wit, C. A. 2002. An overview of brominated flame retardants in the environment. Chemosphere 46:583–624.
- Dudimah, F. D., Gibson, C., and Whalen, M. M. 2007a. Effect of dibutyltin on ATP levels in human natural killer cells. Environ. Toxicol. 22:117–123.
- Dudimah, F. D., Odman-Ghazi, S. O., Hatcher, F., and Whalen, M. M. 2007b. Effect of tributyltin (TBT) on ATP levels in human natural killer (NK) cells: Relationship to TBT-induced decreases in NK function. J. Appl. Toxicol. 27:86–94.
- Environ Defense. 2001. 2,2′,6,6′-Tetrabromo-4,4′-isopropylidenediphenol—Industrial uses. http://www.scorecard.org/chemical-profiles/uses.tcl?edf_substance_id=79%2d94%2d7.
- Eriksson, P., Jakobsson, E., and Fredriksson, A. 2001. Brominated flame retardants: A novel class of developmental neurotoxicants in our environment. Environ. Health Perspect. 109:903–908.
- Fleisher, G., Koven, N., Kamiya, H., and Henle, W. 1982. A non-X-linked syndrome with susceptibility to severe Epstein-Bar virus infections. J. Pediatr. 100:727–730.
- Fukuda, N., Ito, Y., Yamaguchi, M., Mitumori, K., Koizumi, M., Hasegawa, R., Kamata, E., and Ema, M. 2004. Unexpected nephrotoxicity induced by tetrabromobisphenol A in newborn rats. Toxicol. Lett. 150:145–155.
- Gain, B. 1997. Flame retardants’ Albemarle boosts capacity. Chem. Week (July 2, 1997, p. 88). Full text available from PROMT 97:369076.
- Hagmar, L., Jakobsson, K., Thuresson, K., Rylander, L., Sjodin, A., and Bergman, A. 2000. Computer technicians are occupationally exposed to polybrominated diphenyl ethers and tetrabromobisphenol A. Organohalogen Comp. 47:202–205.
- Hinkson, N. C., and Whalen, M. M. 2009. Hexabromocyclododecane decreases the lytic function and ATP levels of human natural killer cells. J. Appl. Toxicol. 2009 Jun 23. [Epub ahead of print] PMID: 19551757 [PubMed - as supplied by publisher].
- HSDB (Hazardous Substances Data Bank). 2001. 2,2′,6,6′-Tetrabromobisphenol A. Bethesda, MD: National Library of Medicine. http://www.toxnet.nlm.nih.gov/cgi-bin/sis/search.
- IPC/WHO (International Program on Chemical Safety/World Health Organization). 1995. Environmental Health Criteria 172: Tetrabromobisphenol A and Derivatives. Geneva: World Health Organization.
- Leu, R. W., Norton, T. R., Herriott, M. J., Ringer, D. P., and Kearns, R. J. 1985. Suppression of natural killer and lymphocyte functions associated with carcinogen-induced pre-malignant hyperplastic nodules in rat liver. Cancer Res. 45:3282–3287.
- Lotzova, E. 1993. Definition and function of natural killer cells. Nat. Immunol. 12:177–193.
- Lu, L. M., Zavitz, C. C., Chen, B., Kianpour, S., Wan, Y., and Stampfli, M. R. 2007. Cigarette smoke impairs NK cell-dependent tumor immune surveillance. J. Immunol. 178:936–943.
- Luebke, R. W., Riddle, M. M., Rogers, R. R., Rowe, D. G., Garner, R. J., and Smialowicz, R. J. 1986. Immune function in adult C57BL/6J mice following exposure to urethane pre- or postnatally. J. Immunopharmacol. 8:243–257.
- Meerts, I. A., van Zanden, J. J., Luijks, E. A., van Leeuwen-Bol, I., Marsh, G., Jakobsson, E., Bergman, A., and Brouwer, A. 2000. Potent competitive interactions of some brominated flame retardants and related compounds with human transthyretin in vitro. Toxicol. Sci. 56:95–104.
- Nagayama, J., Takasuga, T., and Tsuji, H. (Eds.). 2001. Contamination levels of brominated flame retardants, dioxins, and organochlorine compounds in the blood of Japanese adults. In: Human Levels and Trends, Part 4, pp. 218–221. http://www.kemi.se/aktuellt/BFR/bfr_del4.pdf.
- Nnodu, U., and Whalen, M. M. 2008. Pentachlorophenol decreases ATP levels in human natural killer cells. J. Appl. Toxicol. 28:1016–1020.
- Ortaldo, J. R., Glenn, G. M., Young, H. A., and Frey, J. L. 1992. Natural killer (NK) cell lytic dysfunction and putative NK cell receptor expression abnormality in members of a family with chromosome 3p-linked von Hippel-Lindau disease. J. Natl. Cancer Inst. 84:1897–1903.
- Peterman, P. H., Orazio, C. E., and Gale, R. W. 2000. Detection of tetrabromobisphenol A and formation of brominated [13C]-bisphenol A’s in commercial drinking water stored in reusable polycarbonate containers. ACS Div. Environ. Chem. Extended Abstr. 40:431–433.
- Ronisz, D., Farmen Finne, E., Karlsson, H., and Forlin, L. 2004. Effects of the brominated flame retardants hexabromocyclododecane (HBCDD), and tetrabromobisphenol A (TBBPA), on hepatic enzymes and other biomarkers in juvenile rainbow trout and feral eelpout. Aquat. Toxicol. 69:229–245.
- Schauer, U. M., Volkel, W., and Dekant, W. 2006. Toxicokinetics of tetrabromobisphenol A in humans and rats after oral administration. Toxicol. Sci. 91:49–58.
- SEER (Surveillance Epidemiology End Results) National Cancer Institute. U.S. National Institutes of Health. www.cancer.gov. April 2009.
- Sellstroem, U., and Jansson, B. 1995. Analysis of tetrabromobisphenol A in a product and environmental samples. Chemosphere 31:3085–3092.
- Sjödin, A., Hagmar, L., Klasson-Wehler, E., Kronholm-Diab, K., Jakobsson, E., and Bergman, A. 1999. Flame retardant exposure: polybrominated diphenyl ethers in blood from Swedish workers. Environ. Health Perspect. 107:643–648.
- Taylor, T. R., Tucker, T., and Whalen, M. M. 2005. Persistent inhibition of human natural killer cell function by ziram and pentachlorophenol. Environ. Toxicol. 20:418–424.
- Taylor, T. R., and Whalen, M. M. 2009. Effects of ziram on tumor-cell-binding capacity, cell-surface marker expression, and ATP levels of human natural killer cells. Cell Biol. Toxicol. 25:447–455.
- Thomsen, C., Lundanes, E., and Becher, G. 2002. Brominated flame retardants in archived serum samples from Norway: A study on temporal trends and the role of age. Environ. Sci. Technol. 36:1414–1418.
- Thornton, J. W., McCally, M., and Houlihan, J. 2002. Biomonitoring of industrial pollutants: Health and policy implications of the chemical body burden. Public Health Rep. 117:315–323. www.ewg.org/reports/bodyburden/findings.php.
- U.S. EPA. 1999. 40 CFR Part 372: Persistent Bioaccumulation Toxic (PBT) Chemicals; Final Rule.
- U.S. EPA. 2000a. Non-Confidential Production Volume Information Submitted by Companies for Chemicals under the 1998 Inventory Update Rule (IUR). CAS no. 79947. Washington, DC: U.S. EPA, Office of Pollution Prevention and Toxics (OPPT). http://www.epa.gov/cgi-bin/iursrch3.cgi.
- U.S., EPA. 2000b. Emergency Planning and Community Right-To-Know Act Section 313 Reporting Guidance for Rubber and Plastics Manufacturing. EPA 745-B-00-017. Washington, DC: Office of Environmental Information, pp. 2–16. http://www.epa.gov/tri/00rubber.pdf.
- Vivier, E., Nunes, J. A., and Vely, F. 2004. Natural killer cell signaling pathways. Science 306:1517–1519.
- Whalen, M. M., Loganathan, B. G., and Kannan, K. 1999. Immunotoxicity of environmentally-relevant concentrations of butyltins on human natural killer cells in vitro. Environ. Res. 81:108–116.
- Whalen, M. M., Green, S. A., and Loganathan, B. G. 2002. Brief butyltin exposure induces irreversible inhibition of the cytotoxic function on human natural killer cells in vitro. Environ. Res. 88:19–29.
- Whalen, M. M., Wilson, S., Gleghorn, C., and Loganathan, B. G. 2003. Brief exposure to triphenyltin produces irreversible inhibition of the cytotoxic function of human natural killer cells. Environ Res. 92:213–220.
- Wilson, S. D., McCay, J. A., Butterworth, L. F., Munson, A. E., and White, K. L.Jr., 2001. Correlation of suppressed natural killer cell activity with altered host resistance models in B6C3F1 mice. Toxicol. Appl Pharmacol. 177:208–218.