Abstract
Toll-like receptor (TLR)-mediated signaling is proposed as an immunotherapeutic target against tumorigenesis. Natural killer (NK) cells play a critical role in host defense against tumors. Specifically, formation of tumor metastasis in various organs can be suppressed by the local activity of NK cells. In this study, we present a novel TLR7 agonist (termed SC-1) that induces pro-inflammatory cytokines in human blood cells, activates NK cell function, and is highly efficient in preventing lung metastases in a pulmonary metastatic Renca model. Furthermore, a second compound (termed SC-2), acting as dual-specific TLR7 and TLR8 agonist, was evaluated with respect to its immunostimulatory and NK cell–activating capacities. The release of pro-inflammatory cytokines was shown to be even more pronounced with this compound. Additional experiments showed a significant up-regulation of activation marker CD69 on NK cells and increased cytolytic activity of peripheral blood cells compared to the effect of a monospecific TLR7 agonist SC-1. Normally, TLR7 and TLR8 are expressed on different immune cell subpopulations. TLR7 expression on antigen-presenting cells is detected in plasmacytoid dendritic cells, CD34+-derived dendritic cells, and B-cells, whereas TLR8 is mainly expressed on cells of the myeloid lineage, such as monocytes, macrophages, and myeloid dendritic cells. Therefore, a compound that activates both TLR7 and TLR8 would result in a highly efficient immune system activation and may give rise to an enhanced anti-tumor activity in vivo compared to that elicited by a monospecific TLR7 agonist.
Introduction
Established tumors are a consequence of the selective pressure of the immune system which eliminates susceptible tumor clones. The process results in the selection of tumor cell variants that are able to resist, avoid, or suppress the anti-tumor response (reviewed in Swann and Smyth, Citation2007). A promising approach to improve the anti-tumor response involves boosting of the innate immune response with the so-called pathogen-associated molecular patterns (PAMPs). The PAMPs are ligands for Toll-like receptors (TLR), a group of receptors belonging to the innate immune system.
Thirteen members of the TLR family have been reported so far. Their function is to sense pathogen structures from different origins, such as bacteria, viruses, fungi, or protozoan parasites (reviewed in Takeda and Akira, Citation2005). TLR 1, 2, 4, 5, 6, and 11 are expressed on the cell surface and recognize external pathogen structures such as lipopeptides (TLR 1, 2, and 6) (Takeuchi et al., Citation2001, Citation2002), lipopolysaccharide (TLR2 and TLR4) (Hoshino et al., Citation1999; Qureshi et al., Citation1999; Werts et al., Citation2001), flagellin (TLR5) (Hayashi et al., Citation2001), and profilin (TLR11) (Yarovinsky et al., Citation2005). TLR 3, 7, 8, and 9 form a group of intracellular TLR and recognize bacterial or viral nucleic acids. TLR3 is responsible for the recognition of double-stranded RNA (Alexopoulou et al., Citation2001), whereas DNA with non-methylated CpG motifs is recognized by TLR9 (Hemmi et al., Citation2000; Bauer et al., Citation2001; Takeshita et al., Citation2001; Klinman et al., Citation2002). The natural ligand for TLR7 and TLR8 is single-stranded RNA that is rich in guanosine and/or uridine (Diebold et al., Citation2004; Heil et al., Citation2004; Lund et al., Citation2004).
TLR7 and TLR8 are also activated by small synthetic compounds. Primarily, the imidazoquinoline derivates imiquimod (R837) and resiquimod (R848) were described as TLR7 ligands in mice (Hemmi et al., Citation2002). A guanosine analogue, loxoribine, was the next immune system activator identified as a TLR7 ligand (Heil et al., Citation2003; Lee et al., Citation2003). To date, several other compounds have been developed as ligands for TLR7 and/or TLR8, that is, CL097 (3M-001) and 852A are mainly TLR7 agonists, whereas CL075 preferably activates TLR8 (Gorden et al., Citation2005; Harrison et al., Citation2007).
Despite the structural similarities between TLR7 and TLR8, their activation has distinct consequences on the innate immune cells and subsequent production of cytokines. Gorden et al. (Citation2005) demonstrated that TLR8 agonists were much more effective than TLR7 agonist at inducing pro-inflammatory cytokines and chemokines, such as tumor necrosis factor (TNF)-α, interleukin (IL)-12, and macrophage inflammatory protein (MIP)-1α, in peripheral blood mononuclear cells (PBMC), probably due to the expression of TLR8 on myeloid-derived dendritic cells. In contrast, the TLR7-specific agonist 3M-001—but not the TLR8-specific agonist 3M-002—directly activated plasmacytoid dendritic cells and induced the production of interferon (IFN)-α.
So far, the only approved TLR ligand in the clinical setting is imiquimod (as 5% cream, Aldara®; Graceway Pharmaceuticals). It is used for the treatment of genital warts, caused by human papilloma virus infection, and for the treatment of malignant tumors of the skin. Cutaneous tumors that have responded well to topical treatment with imiquimod include basal cell carcinomas (Sterry et al., Citation2002; Geisse et al., Citation2004; Schulze et al., Citation2005), actinic keratosis (Stockfleth et al., Citation2002; Szeimies et al., Citation2004; Korman et al., Citation2005; Hadley et al., Citation2006), keratoacanthomas (Dendorfer et al., Citation2003), and cutaneous metastases of melanoma (Bong et al., Citation2002; Ugurel et al., Citation2002). Another imidazoquinoline, resiquimod, has also been evaluated in a clinical Phase II study on actinic keratosis, with positive results (Szeimies et al., Citation2008).
Until now, TLR7 and TLR8 agonists were predominantly administered topically due to the side effects reported after oral and systemic therapy (i.e., with imiquimod) (Goldstein et al., Citation1998). Systemic administration with resiquimod was even associated with transient peripheral blood leukocyte depletion (Gunzer et al., Citation2005). Intravenously administered TLR7 agonist 852A led to similar adverse effects, but was better tolerated overall (Dummer et al., Citation2008). However, the anti-tumor efficacy with 852A monotherapy was less convincing in that study; this outcome was probably due to the advanced cancer status of enrolled patients.
Ligands for TLR9, CpG-containing oligonucleotides, were also investigated for cancer monotherapy against melanoma (Pashenkov et al., Citation2006), non-Hodgkin’s lymphoma (both studies with PF-3512676) (Link et al., Citation2006), and recurrent glioblastoma (CpG-28) (Carpentier et al., Citation2006). These clinical studies showed an acceptable safety profile, but the frequency of objective responses was relatively low (reviewed in Krieg, Citation2007). Therefore, the focus of ongoing clinical trials with TLR9 agonists has shifted to combination therapies with chemotherapeutics; in many instances, these trials have yielded promising results (Wang et al., Citation2006; Manegold et al., Citation2008). For TLR7/8 ligands, similar constellations could be considered.
Since an intact immune system is prerequisite to determine the anti-tumor effects of immunostimulatory agents, tumor models must be performed in immunocompetent animals. Syngeneic murine models can be used for this purpose, usually B16-F10 tumor model in C57BL/6 mice or Renca tumor model in Balb/c mice were utilized (Heckelsmiller et al., Citation2002; Whitmore et al., Citation2004). If injected intravenously, both B16-F10 and Renca cells produce a high number of pulmonary metastasis, allowing the evaluation of new therapeutic approaches for antimetastatic treatment.
In the present study, we analyzed a novel small TLR7-stimulating molecule — SC-1—in terms of its anti-tumor activity. SC-1 induced pro-inflammatory cytokines in human blood cells, activated NK cell functions, and was highly efficient in preventing metastasis in a Renca pulmonary metastatic model. A second cytokine inducing compound — SC-2—was found to be specific for TLR7 and TLR8, and showed an increased NK cell activating potency compared to the monospecific TLR7 agonist SC-1.
In conclusion, novel synthetic TLR7/8 agonists with improved pharmacological features for treatment of a broader range of tumors are still in demand. It is possible that a combination therapy with established chemotherapeutics will be necessary to achieve the full clinical potential of TLR7/8 agonists.
Material and methods
Reagents
Resiquimod (R848) and lipopolysaccharide (LPS, from Escherichia coli serotype O111:B4) were obtained from Alexis Biochemicals (Axxora Deutschland, Lörrach, Germany). TLR plasmids were purchased from InvivoGen (Toulouse, France). Prelabeled CD69 and NKp46 antibodies were from BD Bioscience (Heidelberg, Germany). All other chemicals were obtained from Sigma-Aldrich (Taufkirchen, Germany).
Animals
Balb/c mice (female, 8 week-of-age) for use in the metastatic model studies were obtained from Janvier (Le Genest Saint Isle, France). All mice were provided a pellet diet produced by sniff Spezialdiäten GmbH (Soest, Germany) and water ad libitum, and were kept as groups of five in well-ventilated cages under standard conditions of humidity (55 ± 5%), temperature (22 ± 2°C), and light (12/12 hr light-dark cycles) in on-site animal housing facilities. The care and all of the animal studies here were conducted in compliance with guidelines of the German Animal Health and Welfare Act (Tierschutzgesetz).
Reporter gene assay
HEK293 cells (3 × 106), stably expressing an NF-κB-dependent luciferase construct, were resuspended in 400 μL medium, mixed with 15 μg plasmid, transferred to a 4-mm electroporation cuvette (BioRad, Munich, Germany), and electroporated at 200 V and 960 μF using a BTX 600 electroporator (VWR International GmbH, Darmstadt, Germany). The cells were seeded into wells of a 96-well plate (at 3 × 104 cells/well), allowed to adhere over 6 hr, and then treated overnight as indicated. Luciferase expression was then measured using OneGlo reagent from Promega (Mannheim, Germany) and a Sunrise Model microplate reader (Tecan, Crailsheim, Germany).
Cells stimulation, cytokine measurement, and FACS analysis
Peripheral blood mononuclear cells (PBMC) from healthy human donors were purified by centrifugation over Ficoll-Hypaque (Sigma-Aldrich, Taufkirchen, Germany). Purified PBMC were then washed twice with phosphate-buffered saline (PBS) and resuspended in RPMI1640 culture medium supplemented with 10% heat-inactivated fetal calf serum, 1.5 mM L-glutamine, 100 U penicillin/mL, and 100 mg streptomycin/mL (all from PAN Biotech, Aidenbach, Germany). For immune stimulation, PBMC were seeded at 1 × 105 cells/ well and treated as indicated for 24 hr. Concentrations of the select cytokines of interest in the culture supernatants were then determined using a Luminex BioPlex system, following the manufacturer’s instructions (BioRad, Munich, Germany). Expression of the early activation marker CD69 on NK cells was analyzed using flow cytometry (FACSCalibur, BD Bioscience, Heidelberg, Germany); for this, stimulated PBMC were stained with fluorescein isothiocyanate (FITC)-labeled CD69 and phycoerythrin (PE)-labeled NKp46 antibodies using standard protocols described in Shevach (Citation2005).
Cytolytic assay with HEK293 cells
Freshly-isolated human PBMC (described earlier) were seeded into a 96-well plate at 6 × 105, 4 × 105, or 2 × 105 cells/well, and treated as indicated for 20 hr. Luciferase-producing HEK293 cells (3 × 104/well) were then added to the pre-stimulated PBMC and the incubation extended for a further 4 hr. Luciferase released from lysed HEK293 cells in the supernatant was determined using OneGlo reagent and a microplate reader (as described earlier). The luciferase release was then reported as relative luminescence units (RLU) per well after subtraction of background (i.e., representative of spontaneous lysis).
Renca metastatic model
Renca cells (1 × 105) expressing β-galactosidase (Renca-lacZ) were inoculated by intravenous injection into Balb/c mice (n = 10 per group). On Days 1–4, animals were treated intravenously with 30 mg/kg once per Day of compound SC-1 formulated in 20% PEG (Polyethylene glycol 400) or the according amount of vehicle (10 mL/kg). One group was not inoculated and was left untreated as blank control. To determine the metastatic burden, the lungs were taken out and weighed on Day 28. Isolated organs were stained with 5-bromo-4-chloro-3-indoxyl-β-D-galactopyranoside to enhance the visibility of the metastasis. Mice were weighed on Days 1–4, 7, 10, 16, 18, 22, 25, and 28.
Statistics
A one-tailed Student’s t-test was used for assessing the significance of tumor volume differences among the compound treated and the vehicle group in the Renca model.
Results
Two novel synthetic immune system activators signal by stimulation of TLR7 or TLR7 and TLR8
Two novel small organic molecules activating cytokine production — compound SC-1 and compound SC-2—were identified in a cellular screen of PBMC. Treatment of PBMC with SC-1 led to the secretion of IL-6, IL-12p40, and IL-1β (). The agent SC-2 was found to be more potent and induced IL-6, IL-12p40, IL-1β, and TNFα () at levels comparable to that due to the resiquimod (R848) that was used as a control. Interestingly, SC-2-induced production of IL-1β and TNFα was highest at 3–10 μM and decreased at 30 μM. No decreasing effect of higher concentration of SC-2 could be observed on IL-6 production. Thus, it is unlikely that this effect is due to the unspecific toxicity of SC-2 at 30 μM. This bell-shaped dose–response curve has often been found for other TLR ligands, for example, for CpG-DNA (Vollmer et al., Citation2004a,Citationb), and may be explained by negative feedback mechanisms. However, in human clinical studies of systemically administered CpG-DNA, linear dose–response curves were observed (Krieg et al., Citation2004), indicating that the observed in vitro dose–response of a TLR ligand might be of limited significance in the clinical setting.
Figure 1. Novel synthetic immune system activator ability to stimulate immune cells via TLR7 or TLR7 and TLR8. (A) Human PBMC were treated with indicated stimuli for 24 hr and cytokine production was determined from measures of the culture supernatant by Luminex. The cytokines levels are in pg/mL. One representative experiment out of three using PBMC from different donors is shown. (B) HEK293 cells stably expressing an NF-κB-dependent luciferase construct were transfected with a TLR7, TLR8, TLR9, or mock, and stimulated as indicated. After 16 hr, luciferase activity was determined in cell lysates. As controls, 1 μg/mL LPS, 10 μM R848, or 10 μM ODN2006 was employed.
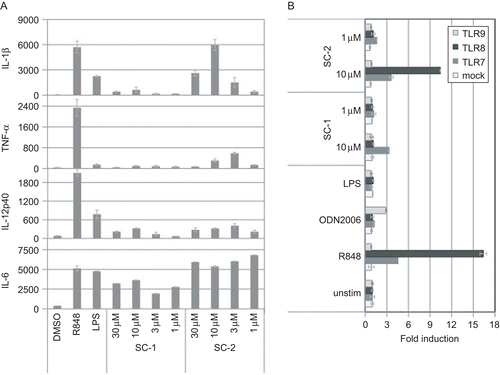
Chloroquine inhibits the maturation of endosomes by buffering their acidification. Since endosomal maturation is crucial for signaling through endosomal TLR, chloroquine is often used to ensure the dependency of the immune stimulation on TLR 3, 7, 8, and 9. The cytokine production induced by the test compounds here was also abrogated by chloroquine addition (data not shown), suggesting that these compounds may be ligands for endosomal TLR.
Among the endosomal TLR, only TLR7 and TLR8 are known to recognize synthetic small molecules such as resiquimod, loxoribine, or imiquimod. Therefore, it was decided to examine if the test compounds activated these toll-like receptors first. For this purpose, a reporter gene assay with an NF-κB-dependent luciferase gene and TLR7 or TLR8 was constructed. In fact, the results in the present study showed that SC-1-induced NF-κB activation was TLR7-dependent, whereas SC-2 activated both TLR7 and TLR8 (). Moreover, neither compound was active on TLR9 (). Furthermore, the lack of noticeable TNFα production by a specific TLR7 agonist SC-1 is consistent with the report of Gorden et al. (Citation2005) that a TLR8 agonist (i.e., 3M-002) is a better TNFα inducer than a specific TLR7 agonist (i.e., 3M-001).
Novel TLR ligands stimulate NK cell activation and enhance their cytolytic activity
One field of application in cancer immunotherapy discussed with regard to TLR ligands is the inhibition of metastasis formation. Natural killer (NK) cells play a crucial role in preventing metastasis (Wiltrout et al., Citation1985). Although human NK cells do not express TLR7 or TLR8 and are not directly responsive to their ligands, they become indirectly activated through cytokine release by TLR7- or TLR8- responsive immune cells (Hornung et al., Citation2002; Gorski et al., Citation2006). Therefore, the activation of NK cells upon agonist stimulation was analyzed in the PBMC populations here. Both compounds, SC-1 and SC-2, induced up-regulation of an early activation marker CD69 on NK cells at low μM concentrations, with SC-2 being more potent than SC-1 ().
Figure 2. Novel TLR7/8 agonist effects on NK cell activity. (A and B) Human PBMC were treated as indicated in legend of , and the up-regulation of an early activation marker CD69 on NK cells was determined 24 h after stimulation. (C) To estimate functionality of activated NK cells, cytolytic activity against HEK293 cells was measured. Specifically, pre-stimulated human PBMC were incubated for 4 hr with luciferase-producing HEK293 cells and luciferase activity was then assessed in the supernatant. Test compounds were used in indicated concentrations; 10 μM R848 was used as control. PBMC from the same donor were used to compare the effect of both compounds. Note that due to the relatively low number of NK cells in PBMC, the cytolytic effect at 13:1 ratio is subject to more variation compared to a ratio of 20:1.
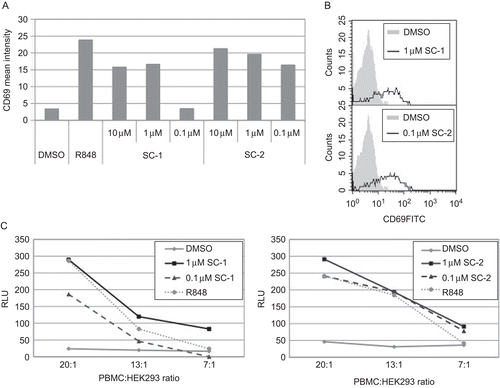
One function of NK cells is to lyse tumor and virus-transformed cells. To demonstrate the correlation of increased CD69 expression with the activity of NK cells, a non-radioactive cytolytic assay was designed. Instead of labeling of target cells with 51Cr, luciferase-producing HEK293 cells were used. HEK293 cells are susceptible to lysis by activated NK and can be used to demonstrate NK cell activity. PBMC pre-stimulated with the test compounds were used as effector cells. Luciferase-induced luminescence resulting from lysed target cells corresponded to the cytolytic activity of PBMC. The internal control resiquimod (R848) induced the lysis of target cells at effector/target ratio of 20:1 and 13:1, but was not effective at 7:1 ratio. The test compounds used in the present study were able to enhance cytolytic activity to the similar extent, with SC-2 again being more efficient at lower concentrations than SC-1 (). When incubated with the target cells alone, both compounds had no effect on luciferase release, therefore lacking unspecific cytotoxicity (data not shown).
The novel TLR7 ligand was highly efficient in preventing lung metastasis in the Renca metastasis model
The murine syngeneic renal cell carcinoma (i.e., Renca) model allows evaluation of therapy on metastasis as well as on primary tumors if implanted under the renal capsule of Balb/c mice (Hillman, Citation2002). To increase the incidence and number of pulmonary metastasis, Renca cells can be injected intravenously (Chakrabarty et al., Citation1994). In this pulmonary metastatic model, the inhibiting effect of the TLR7 ligand SC-1 on the colonization of the lung with the metastasis was investigated. The once per day treatment started 1 day after the injection of 1 × 105 tumor cells into the tail vein of Balb/c mice and went for 4 days. A dose of 30 mg/kg SC-1 was then intravenously administered in the SC-1-treated group, while the vehicle group received an according volume of vehicle (20% polyethylene glycol [PEG]). One group was not inoculated with tumor cells and was left untreated as a blank control. After 28 days, the mice were killed and the metastatic burden in their lungs was assessed via weighing of the excised organs. The lungs of the animals in the group treated with SC-1 displayed, on average, the same weight when compared to the lungs from the control group. On the other hand, the mean weight of the lungs in the vehicle group nearly doubled (from ∼370 to ∼670 mg; ). Differences between the vehicle and SC-1-treated group were highly significant judged by a one-tailed Student’s t-test (P = 0.00009).
Figure 3. SC-1 effects on preventing metastasis in the Renca lung metastasis model. A total of 1 × 105 Renca cells were injected intravenously into Balb/c mice (n = 10 per group); on Days 1–4, animals were treated once a Day with 30 mg/kg SC-1 or an equal amount of vehicle (20% PEG, 10 mL/kg). One group was not inoculated and was left untreated as blank control. (A) To determine metastatic burden, lungs were removed and weighed on Day 28. Statistical significance of the difference between the vehicle and the SC-1-treated group was analyzed using a one-tailed Student’s t-test (***P=0.00009). A representative lung from vehicle and from SC-1-treated group is shown in (B) and (C), respectively. For better visibility, Renca metastases were stained with 5-bromo-4-chloro-3-indoxyl-β-D-galactopyranoside. (D) To estimate adverse effects, mice were weighed on Days 1–4 and then at least two times a week thereafter.
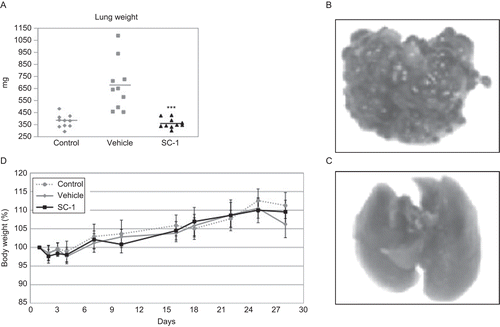
To visualize the metastasis, the lungs were stained with bromo-4-chloro-3-indoxyl-β-D-galactopyranoside. Virtually no metastasis could be found in the lungs from the SC-1-treated group (), whereas the lungs from the vehicle group were highly infested (). Body weight as a marker of adverse effects in cancer models was not affected by treatment with SC-1 (). As a result, we could show that a novel synthetic TLR7 agonist SC-1 was able to completely prevent the formation of metastasis without apparent adverse effects and that a relatively short treatment period of 4 days was sufficient to achieve this effect.
Discussion
After stimulation of a Toll-like receptor, a cascade of intracellular signaling events is activated that culminates in the production of pro-inflammatory cytokines such as TNFα, IL-6, IL-1β, and IL-12. This series of events is mediated by activation of NF-κB transcription factor that acts as a master switch for inflammation, and of the MAP kinases p38 and JNK that participate in increased transcription of inflammatory genes (reviewed in O’Neill, Citation2006). In addition, some TLR agonists can lead to the Type I interferon (IFN) production via IFN regulatory factor (IRF)-5 or -7 (Honda et al., Citation2004; Kawai et al., Citation2004; Takaoka et al., Citation2005). TLR stimulation of professional antigen-presenting cells also leads to increased expression of co-stimulatory molecules (such as CD80 and CD86) and chemokines. These innate immunity effects can promote tumor regression either directly through the anti-tumor activity of Type I IFNs (Pestka et al., Citation2004), or indirectly through the activation of NK cell–mediated tumor killing that is followed by a tumor antigen-specific T-cell response (Liu et al., Citation2008).
In the present study, we demonstrated that the treatment of human peripheral blood immune cells with a novel TLR7 stimulating small molecule induced the production of pro-inflammatory cytokines as well as up-regulation of NK cell function in vitro. In vivo this compound showed a potent anti-metastatic activity in a murine metastatic renal cell carcinoma model. Furthermore, a second compound with an even more pronounced cytokine inducing capacity was evaluated with respect to its immunostimulatory and NK cell activation capacities. This compound activated TLR8 as well as TLR7 and showed an increased NK cell cytolytic activity compared to the monospecific TLR7 agonist.
TLR7 and TLR8 are expressed on different immune cell subpopulations. TLR7 is produced in plasmacytoid dendritic cells, CD34+-derived dendritic cells, B-cells, and eosinophils. In contrast, TLR8 is mainly expressed in cells of the myeloid lineage, such as monocytes, macrophages, and myeloid dendritic cells (Hornung et al., Citation2002; Ito et al., Citation2002; CitationNagase et al., 2003; Larangé et al., Citation2009). Thus, a compound that activates TLR8 as well as TLR7 would result in a highly efficient immune system activation and may show an enhanced anti-tumor activity in vivo. However, singular systemic administration of the TLR7/8 ligand resiquimod resulted in a transient depletion of leukocytes from the blood (Gunzer et al., Citation2005), and sustained treatment with resiquimod induced an immunopathology reminiscent of progressive lymphoid destruction in HIV disease in mice (Baenziger et al., Citation2009). Consequently, it may be possible that the stronger immune activation by triggering both TLR7 and TLR8 is inseparable from the observed adverse effects. On the other hand, cytokine profile induced by different TLR7/8 agonists can vary (Gorden et al., Citation2005, and personal observations). Thus, it may be feasible to find a TLR7/8 agonist with a safer immunostimulatory profile than resiquimod without loss of efficacy.
In the evaluation of immunotherapy approaches, the murine syngeneic renal cell carcinoma (Renca) model in immunocompetent Balb/c mice has been commonly used since the 1980s, initially to assess cytokine mono- and co-therapies (Hillman, Citation2002). With the discovery of CpG-DNA as a TLR9 agonist, the model was also used to estimate the effectivity of CpG-DNA as a innate immune system stimulus with promising results, illustating the suitability of this model to evaluate the anti-tumor response of TLR agonists on solid tumors (Heckelsmiller et al., Citation2002).
In 1994, Chakrabarty et al. presented a modified pulmonary metastatic Renca model that was suitable for quantitative evaluation of new therapeutic approaches for the treatment of lung metastasis. Since TLR-induced activation of the immune system can increase resistance to metastasis formation (Kawarada et al., Citation2001; Yang et al., Citation2006), it was decided to test the efficacy of the novel TLR7 agonist in this model first. Recently, the anti-tumor effect of another TLR7 agonist, imiquimod, was also assessed in the metastatic Renca model. In this case, the treatment started 1 week after the injection of Renca cells, and the mice were treated orally with imiquimod every other day for 2 weeks. In these settings, imiquimod significantly reduced the number of pulmonary metastasis compared to placebo, and 30% of the animals were completely free of metastasis in the lung (Schwartz et al., Citation2009). However, other models in immunocompetent animals are also available and should be examined to demonstrate the efficacy of our compounds against cells other than Renca tumor cells. The B16-F10 melanoma model in C57BL/6 mice, for instance, has been used to assess anti-tumor effects of loxoribine, CpG oligonucleotide 1826, poly(I:C), and a TLR7/8 ligand 3M-011 (Pope et al., Citation1994; Whitmore et al., Citation2004; Dumitru et al., Citation2009). In addition to adverse effects usually surveyed in cancer models, specific adverse effects entailed by TLR stimulation, such as fever, lymphopenia, and splenomegaly should be monitored in any prospective animal models.
In summary, because of their ability to boost the immune system, TLR7/8 agonists are proposed as immunotherapeutic target against tumorigenesis. In addition to their use as a standalone immunotherapeutic agent, TLR7/8 agonists can be combined with standard chemotherapeutics (as proposed for TLR9 agonists) and hold a promise as potential adjuvants in cancer vaccinations (reviewed in Krieg, Citation2007; Smits et al., Citation2008). The present study described the effects of two novel agonists for TLR7 or TLR7/8 that displayed a potent immunostimulatory activity in vitro. Furthermore, for the novel TLR7 agonist SC-1, anti-tumor in vivo efficacy was demonstrated in a metastatic Renca model. Despite these encouraging findings, further investigation of these promising compounds is required and already scheduled.
Acknowledgments
4SC is grateful to Nycomed/Altana Pharma staff for performing the Renca model. We also thank Sylvia Lemstra for her critical reading of the manuscript.
Declaration of interest: All of the authors are employees of 4SC AG and may have financial interest in the development of novel TLR7/8 agonists for cancer therapies.
References
- Alexopoulou, L., Holt, A.C., Medzhitov, R., and Flavell, R. A. 2001. Recognition of double-stranded RNA and activation of NF-κB by Toll-like receptor 3. Nature 413:732–738.
- Baenziger, S., Heikenwalder, M., Johansen, P., Schlaepfer, E., Hofer, U., Miller, R. C., Diemand, S., Honda, K., Kundig, T. M., Aguzzi, A., and Speck, R. F. 2009. Triggering TLR7 in mice induces immune activation and lymphoid system disruption, resembling HIV-mediated pathology. Blood 113:377–388.
- Bauer, S., Kirschning, C. J., Hacker, H., Redecke, V., Hausmann, S., Akira, S., Wagner, H., and Lipford, G. B. 2001. Human TLR9 confers responsiveness to bacterial DNA via species-specific CpG motif recognition. Proc. Natl. Acad. Sci. USA 98:9237–9242.
- Bong, A. B., Bonnekoh, B., Franke, I., Schon, M. P., Ulrich, J., and Gollnick, H. 2002. Imiquimod, a topical immune response modifier, in the treatment of cutaneous metastases of malignant melanoma. Dermatology 205:135–138.
- Carpentier, A., Laigle-Donadey, F., Zohar, S., Capelle, L., Behin, A., Tibi, A., Martin-Duverneuil, N., Sanson, M., Lacomblez, L., Taillibert, S., Puybasset, L., Van Effenterre, R., Delattre, J. Y., and Carpentier, A. F. 2006. Phase 1 trial of a CpG oligodeoxynucleotide for patients with recurrent glioblastoma. Neurol. Oncol. 8:60–66.
- Chakrabarty, A., Hillman, G. G., Maughan, R. L., Ali, E., Pontes, J. E., and Haas, G. P. 1994. Radiation therapy enhances the therapeutic effect of immunotherapy on pulmonary metastases in a murine renal adenocarcinoma model. In Vivo 8:25–31.
- Dendorfer, M., Oppel, T., Wollenberg, A., and Prinz, J. C. 2003. Topical treatment with imiquimod may induce regression of facial keratoacanthoma. Eur. J. Dermatol. 13:80–82.
- Diebold, S. S., Kaisho, T., Hemmi, H., Akira, S., and Reise Sousa C. 2004. Innate antiviral responses by means of TLR7-mediated recognition of single-stranded RNA. Science 303:1529–1531.
- Dumitru, C. D., Antonysamy, M. A., Gorski, K. S., Johnson, D. D., Reddy, L. G., Lutterman, J. L., Piri, M. M., Proksch, J., McGurran, S. M., Egging, E. A., Cochran, F. R., Lipson, K. E., Tomai, M. A., and Gullikson, G. W. 2009. NK1.1+ cells mediate the anti-tumor effects of a dual Toll-like receptor 7/8 agonist in the disseminated B16-F10 melanoma model. Cancer Immunol. Immunother. 58:575–587.
- Dummer, R., Hauschild, A., Becker, J. C., Grob, J. J., Schadendorf, D., Tebbs, V., Skalsky, J., Kaehler, K. C., Moosbauer, S., Clark, R., Meng, T. C., and Urosevic, M. 2008. An exploratory study of systemic administration of the toll-like receptor-7 agonist 852A in patients with refractory metastatic melanoma. Clin. Cancer Res. 14:856–864.
- Geisse, J., Caro, I., Lindholm, J., Golitz, L., Stampone, P., and Owens, M. 2004. Imiquimod 5% cream for the treatment of superficial basal cell carcinoma: Results from two Phase III, randomized, vehicle-controlled studies. J. Am. Acad. Dermatol. 50:722–733.
- Goldstein, D., Hertzog, P., Tomkinson, E., Couldwell, D., McCarville, S., Parrish, S., Cunningham, P., Newell, M., Owens, M., and Cooper, D. A. 1998. Administration of imiquimod, an interferon inducer, in asymptomatic human immunodeficiency virus-infected persons to determine safety and biologic response modification. J. Infect. Dis. 178:858–861.
- Gorden, K. B., Gorski, K. S., Gibson, S. J., Kedl, R. M., Kieper, W. C., Qiu, X., Tomai, M. A., Alkan, S. S., and Vasilakos, J. P. 2005. Synthetic TLR agonists reveal functional differences between human TLR7 and TLR8. J. Immunol. 174:1259–1268.
- Gorski, K. S., Waller, E. L., Bjornton-Severson, J., Hanten, J. A., Riter, C. L., Kieper, W. C., Gorden, K. B., Miller, J. S., Vasilakos, J. P., Tomai, M. A., and Alkan, S. S. 2006. Distinct indirect pathways govern human NK-cell activation by TLR-7 and TLR-8 agonists. Int. Immunol. 18:1115–1126.
- Gunzer, M., Riemann, H., Basoglu, Y., Hillmer, A., Weishaupt, C., Balkow, S., Benninghoff, B., Ernst, B., Steinert, M., Scholzen, T., Sunderkotter, C., and Grabbe, S. 2005. Systemic administration of a TLR7 ligand leads to transient immune incompetence due to peripheral-blood leukocyte depletion. Blood 106:2424–2432.
- Hadley, G., Derry, S., and Moore, R. A. 2006. Imiquimod for actinic keratosis: Systematic review and meta-analysis. J. Invest. Dermatol. 126:1251–1255.
- Harrison, L.I., Astry, C., Kumar, S., and Yunis, C. 2007. Pharmacokinetics of 852A, an imidazoquinoline Toll-like receptor 7-specific agonist, following intravenous, subcutaneous, and oral administrations in humans. J. Clin. Pharmacol. 47:962–969.
- Hayashi, F., Smith, K. D., Ozinsky, A., Hawn, T. R., Yi, E. C., Goodlett, D. R., Eng, J. K., Akira, S., Underhill, D. M., and Aderem, A. 2001. The innate immune response to bacterial flagellin is mediated by Toll-like receptor 5. Nature 410:1099–1103.
- Heckelsmiller, K., Rall, K., Beck, S., Schlamp, A., Seiderer, J., Jahrsdorfer, B., Krug, A., Rothenfusser, S., Endres, S., and Hartmann, G. 2002. Peritumoral CpG DNA elicits a coordinated response of CD8 T-cells and innate effectors to cure established tumors in a murine colon carcinoma model. J. Immunol. 169:3892–3899.
- Heil, F., Ahmad-Nejad, P., Hemmi, H., Hochrein, H., Ampenberger, F., Gellert, T., Dietrich, H., Lipford, G., Takeda, K., Akira, S., Wagner, H., and Bauer, S. 2003. The Toll-like receptor 7 (TLR7)-specific stimulus loxoribine uncovers a strong relationship within the TLR7, 8 and 9 subfamily. Eur. J. Immunol. 33:2987–2997.
- Heil, F., Hemmi, H., Hochrein, H., Ampenberger, F., Kirschning, C., Akira, S., Lipford, G., Wagner, H., and Bauer, S. 2004. Species-specific recognition of single-stranded RNA via toll-like receptor 7 and 8. Science 303:1526–1529.
- Hemmi, H., Kaisho, T., Takeuchi, O., Sato, S., Sanjo, H., Hoshino, K., Horiuchi, T., Tomizawa, H., Takeda, K., and Akira, S. 2002. Small anti-viral compounds activate immune cells via the TLR7 MyD88-dependent signaling pathway. Nat. Immunol. 3:196–200.
- Hemmi, H., Takeuchi, O., Kawai, T., Kaisho, T., Sato, S., Sanjo, H., Matsumoto, M., Hoshino, K., Wagner, H., Takeda, K., and Akira, S. 2000. A Toll-like receptor recognizes bacterial DNA. Nature 408:740–745.
- Hillman, G. G. 2002. Experimental animal models for renal cell carcinoma. In: Tumor Models in Cancer Research (Teicher, B. A., Ed.), Heidelberg: Springer, pp. 494–498.
- Honda, K., Yanai, H., Mizutani, T., Negishi, H., Shimada, N., Suzuki, N., Ohba, Y., Takaoka, A., Yeh, W.C., and Taniguchi, T. 2004. Role of a transductional-transcriptional processor complex involving MyD88 and IRF-7 in Toll-like receptor signaling. Proc. Natl. Acad. Sci. USA 101:15416–15421.
- Hornung, V., Rothenfusser, S., Britsch, S., Krug, A., Jahrsdorfer, B., Giese, T., Endres, S., and Hartmann, G. 2002. Quantitative expression of toll-like receptor 1-10 mRNA in cellular subsets of human peripheral blood mononuclear cells and sensitivity to CpG oligodeoxynucleotides. J. Immunol. 168:4531–4537.
- Hoshino, K., Takeuchi, O., Kawai, T., Sanjo, H., Ogawa, T., Takeda, Y., Takeda, K., and Akira, S. 1999. Cutting edge: Toll-like receptor 4 (TLR4)-deficient mice are hyporesponsive to lipopolysaccharide: Evidence for TLR4 as the Lps gene product. J. Immunol. 162:3749–3752.
- Ito, T., Amakawa, R., Kaisho, T., Hemmi, H., Tajima, K., Uehira, K., Ozaki, Y., Tomizawa, H., Akira, S., and Fukuhara, S. 2002. Interferon-alpha and interleukin-12 are induced differentially by Toll-like receptor 7 ligands in human blood dendritic cell subsets. J. Exp. Med. 195:1507–1512.
- Kawai, T., Sato, S., Ishii, K.J., Coban, C., Hemmi, H., Yamamoto, M., Terai, K., Matsuda, M., Inoue, J., Uematsu, S., Takeuchi, O., and Akira, S. 2004. Interferon-alpha induction through Toll-like receptors involves a direct interaction of IRF7 with MyD88 and TRAF6. Nat. Immunol. 5:1061–1068.
- Kawarada, Y., Ganss, R., Garbi, N., Sacher, T., Arnold, B., and Hammerling, G. J. 2001. NK- and CD8+ T-cell-mediated eradication of established tumors by peritumoral injection of CpG-containing oligodeoxynucleotides. J. Immunol. 167:5247–5253.
- Klinman, D. M., Takeshita, F., Gursel, I., Leifer, C., Ishii, K. J., Verthelyi, D., and Gursel, M. 2002. CpG DNA: Recognition by and activation of monocytes. Microbes Infect. 4:897–901.
- Korman, N., Moy, R., Ling, M., Matheson, R., Smith, S., McKane, S., and Lee, J. H. 2005. Dosing with 5% imiquimod cream 3 times per week for the treatment of actinic keratosis: Results of two Phase 3, randomized, double-blind, parallel-group, vehicle-controlled trials. Arch. Dermatol. 141:467–473.
- Krieg, A. M., Efler, S. M., Wittpoth, M., Al Adhami, M. J., and Davis, H. L. 2004. Induction of systemic TH1-like innate immunity in normal volunteers following subcutaneous but not intravenous administration of CPG 7909, a synthetic B-class CpG oligodeoxynucleotide TLR9 agonist. J. Immunother. 27:460–471.
- Krieg, A. M. 2007. Development of TLR9 agonists for cancer therapy. J. Clin. Invest. 117:1184–1194.
- Larangé, A., Antonios, D., Pallardy, M., and Kerdine-Romer, S. 2009. TLR7 and TLR8 agonists trigger different signaling pathways for human dendritic cell maturation. J. Leukocyte Biol. 85:673–683.
- Lee, J., Chuang, T. H., Redecke, V., She, L., Pitha, P. M., Carson, D. A., Raz, E., and Cottam, H. B. 2003. Molecular basis for the immunostimulatory activity of guanine nucleoside analogs: Activation of Toll-like receptor 7. Proc. Natl. Acad. Sci. USA 100:6646–6651.
- Link, B. K., Ballas, Z. K., Weisdorf, D., Wooldridge, J. E., Bossler, A. D., Shannon, M., Rasmussen, W. L., Krieg, A. M., and Weiner, G. J. 2006. Oligodeoxynucleotide CpG 7909 delivered as intravenous infusion demonstrates immunologic modulation in patients with previously treated non-Hodgkin lymphoma. J. Immunother. 29:558–568.
- Liu, C., Lou, Y., Lizee, G., Qin, H., Liu, S., Rabinovich, B., Kim, G.J., Wang, Y. H., Ye, Y., Sikora, A. G., Overwijk, W. W., Liu, Y. J., Wang, G., and Hwu, P. 2008. Plasmacytoid dendritic cells induce NK cell-dependent, tumor antigen-specific T-cell cross-priming and tumor regression in mice. J. Clin. Invest. 118:1165–1175.
- Lund, J. M., Alexopoulou, L., Sato, A., Karow, M., Adams, N. C., Gale, N. W., Iwasaki, A., and Flavell, R. A. 2004. Recognition of single-stranded RNA viruses by Toll-like receptor 7. Proc. Natl. Acad. Sci. USA 101:5598–5603.
- Manegold, C., Gravenor, D., Woytowitz, D., Mezger, J., Hirsh, V., Albert, G., Al-Adhami, M., Readett, D., Krieg, A. M., and Leichman, C. G. 2008. Randomized Phase II trial of a toll-like receptor 9 agonist oligodeoxynucleotide, PF-3512676, in combination with first-line taxane plus platinum chemotherapy for advanced-stage non-small-cell lung cancer. J. Clin. Oncol. 26:3979–3986.
- Nagase, H., Okugawa, S., Ota, Y., Yamaguchi, M., Tomizawa, H., Matsushima, K., Ohta, K., Yamamoto, K., and Hirai, K. (2003). Expression and function of Toll-like receptors in eosinophils: Activation by Toll-like receptor 7 ligand. J. Immunol. 171:3977–3982.
- O’Neill, L. A. 2006. How Toll-like receptors signal: What we know and what we don’t know. Curr. Opin. Immunol. 18:3–9.
- Pashenkov, M., Goess, G., Wagner, C., Hormann, M., Jandl, T., Moser, A., Britten, C. M., Smolle, J., Koller, S., Mauch, C., Tantcheva-Poor, I., Grabbe, S., Loquai, C., Esser, S., Franckson, T., Schneeberger, A., Haarmann, C., Krieg, A. M., Stingl, G., and Wagner, S. N. 2006. Phase II trial of a Toll-like receptor 9-activating oligonucleotide in patients with metastatic melanoma. J. Clin. Oncol. 24:5716–5724.
- Pestka, S., Krause, C. D., and Walter, M. R. 2004. Interferons, interferon-like cytokines, and their receptors. Immunol. Rev. 202:8–32.
- Pope, B. L., Sigindere, J., Chourmouzis, E., MacIntyre, P., and Goodman, M. G. 1994. 7-Allyl-8-oxoguanosine (loxoribine) inhibits the metastasis of B16 melanoma cells and has adjuvant activity in mice immunized with a B16 tumor vaccine. Cancer Immunol. Immunother. 38:83–91.
- Qureshi, S. T., Lariviere, L., Leveque, G., Clermont, S., Moore, K. J., Gros, P., and Malo, D. 1999. Endotoxin-tolerant mice have mutations in Toll-like receptor 4 (Tlr-4). J. Exp. Med. 189:615–625.
- Schulze, H. J., Cribier, B., Requena, L., Reifenberger, J., Ferrandiz, C., Garcia Diez, A., Tebbs, V., and McRae, S. 2005. Imiquimod 5% cream for the treatment of superficial basal cell carcinoma: Results from a randomized vehicle-controlled Phase III study in Europe. Br. J. Dermatol. 152:939–947.
- Schwartz, M. J., Liu, H., Hwang, D. H., Kawamoto, H., and Scherr, D. S. 2009. Anti-tumor effects of an imidazoquinoline in renal cell carcinoma. Urology 73:1156–1162.
- Shevach, E. M. 2005. Immunofluorescence and cell sorting. In: Short Protocols in Immunology (Coligan, J. E., Marguiles, D. H., Shevach, E. M., and Strober, W., Eds.), Hoboken, NJ: John Wiley & Sons, pp. 4-1–4-15.
- Smits, E. L., Ponsaerts, P., Berneman, Z. N., and Van Tendeloo, V. F. 2008. The use of TLR7 and TLR8 ligands for the enhancement of cancer immunotherapy. Oncologist 13:859–875.
- Sterry, W., Ruzicka, T., Herrera, E., Takwale, A., Bichel, J., Andres, K., Ding, L., and Thissen, M. R. 2002. Imiquimod 5% cream for the treatment of superficial and nodular basal cell carcinoma: Randomized studies comparing low-frequency dosing with and without occlusion. Br. J. Dermatol. 147:1227–1236.
- Stockfleth, E., Meyer, T., Benninghoff, B., Salasche, S., Papadopoulos, L., Ulrich, C., and Christophers, E. 2002. A randomized, double-blind, vehicle-controlled study to assess 5% imiquimod cream for the treatment of multiple actinic keratoses. Arch. Dermatol. 138:1498–1502.
- Swann, J. B., and Smyth, M. J. 2007. Immune surveillance of tumors. J. Clin. Invest. 117:1137–1146.
- Szeimies, R. M., Bichel, J., Ortonne, J. P., Stockfleth, E., Lee, J., and Meng, T. C. 2008. A Phase II dose-ranging study of topical resiquimod to treat actinic keratosis. Br. J. Dermatol. 159:205–210.
- Szeimies, R. M., Gerritsen, M. J., Gupta, G., Ortonne, J. P., Serresi, S., Bichel, J., Lee, J. H., Fox, T. L., and Alomar, A. 2004. Imiquimod 5% cream for the treatment of actinic keratosis: Results from a Phase III, randomized, double-blind, vehicle-controlled, clinical trial with histology. J. Am. Acad. Dermatol. 51:547–555.
- Takaoka, A., Yanai, H., Kondo, S., Duncan, G., Negishi, H., Mizutani, T., Kano, S., Honda, K., Ohba, Y., Mak, T. W., and Taniguchi, T. 2005. Integral role of IRF-5 in the gene induction programme activated by Toll-like receptors. Nature 434:243–249.
- Takeda, K., and Akira, S. 2005. Toll-like receptors in innate immunity. Int. Immunol. 17:1–14.
- Takeshita, F., Leifer, C. A., Gursel, I., Ishii, K. J., Takeshita, S., Gursel, M., and Klinman, D. M. 2001. Cutting edge: Role of Toll-like receptor 9 in CpG DNA-induced activation of human cells. J. Immunol. 167:3555–3558.
- Takeuchi, O., Kawai, T., Muhlradt, P. F., Morr, M., Radolf, J. D., Zychlinsky, A., Takeda, K., and Akira, S. 2001. Discrimination of bacterial lipoproteins by Toll-like receptor 6. Int. Immunol. 13:933–940.
- Takeuchi, O., Sato, S., Horiuchi, T., Hoshino, K., Takeda, K., Dong, Z., Modlin, R. L., and Akira, S. 2002. Cutting edge: Role of Toll-like receptor 1 in mediating immune response to microbial lipoproteins. J. Immunol. 169:10–14.
- Ugurel, S., Wagner, A., Pfohler, C., Tilgen, W., and Reinhold, U. 2002. Topical imiquimod eradicates skin metastases of malignant melanoma but fails to prevent rapid lymphogenous metastatic spread. Br. J. Dermatol. 147:621–624.
- Vollmer, J., Rankin, R., Hartmann, H., Jurk, M., Samulowitz, U., Wader, T., Janosch, A., Schetter, C., and Krieg, A. M. 2004a. Immunopharmacology of CpG oligodeoxynucleotides and ribavirin. Antimicrob. Agents Chemother. 48:2314–2317.
- Vollmer, J., Weeratna, R., Payette, P., Jurk, M., Schetter, C., Laucht, M., Wader, T., Tluk, S., Liu, M., Davis, H. L., and Krieg, A. M. 2004b. Characterization of three CpG oligodeoxynucleotide classes with distinct immunostimulatory activities. Eur. J. Immunol. 34:251–262.
- Wang, H., Rayburn, E. R., Wang, W., Kandimalla, E. R., Agrawal, S., and Zhang, R. 2006. Chemotherapy and chemosensitization of non-small cell lung cancer with a novel immunomodulatory oligonucleotide targeting Toll-like receptor 9. Mol. Cancer Ther. 5:1585–1592.
- Werts, C., Tapping, R. I., Mathison, J. C., Chuang, T. H., Kravchenko, V., Saint Girons, I., Haake, D. A., Godowski, P. J., Hayashi, F., Ozinsky, A., Underhill, D. M., Kirschning, C. J., Wagner, H., Aderem, A., Tobias, P. S., and Ulevitch, R. J. 2001. Leptospiral lipopolysaccharide activates cells through a TLR2-dependent mechanism. Nat. Immunol. 2:346–352.
- Whitmore, M. M., DeVeer, M. J., Edling, A., Oates, R. K., Simons, B., Lindner, D., and Williams, B. R. 2004. Synergistic activation of innate immunity by double-stranded RNA and CpG DNA promotes enhanced anti-tumor activity. Cancer Res. 64:5850–5860.
- Wiltrout, R. H., Salup, R. R., Twilley, T. A., and Talmadge, J. E. 1985. Immunomodulation of natural killer activity by polyribonucleotides. J. Biol. Resp. Mod. 4:512–517.
- Yang, Q., Goding, S. R., Hokland, M. E., and Basse, P. H. 2006. Anti-tumor activity of NK cells. Immunol. Res. 36:13–25.
- Yarovinsky, F., Zhang, D., Andersen, J. F., Bannenberg, G. L., Serhan, C. N., Hayden, M. S., Hieny, S., Sutterwala, F. S., Flavell, R. A., Ghosh, S., and Sher, A. 2005. TLR11 activation of dendritic cells by a protozoan profilin-like protein. Science 308:1626–1629.