Abstract
Measurements of antibodies in bodily fluids (e.g., by ELISA) have provided robust and reproducible results for decades and such assays have been validated for monitoring of B-cell immunity. In contrast, measuring T-cell immunity has proven to be a challenge due to the need to test live cells in functional assays ex vivo. Several previous efforts looking into the reproducibility of ex vivo T-cell assays between different laboratories, or even within the same laboratory, have provided rather discouraging results. The hypothesis we tested in this study is that those poor results are due to the lack of assay and data analysis standardization, rather than the inherent complexity of T-cell assays. In this study, 11 laboratories across Europe and the United States were provided identical reagents and were asked to follow the same protocol while testing aliquots of the same three cryopreserved peripheral blood mononuclear cells (PBMC) in an interferon-γ (IFNγ) ELISPOT assay measuring the antigen-specific T-cell response to a CMV peptide. All individuals performing the assays were ELISPOT novices. At their first attempt, while three of these individuals failed with the basic logistics of the trial, eight detected the peptide-specific CD8+ T-cells in frequencies approximating the values established by the Reference Laboratory. The data show that ELISPOT assays provide reproducible results among different laboratories when the assay procedure and data analysis is standardized. Since ELISPOT assays have been qualified and validated for regulated studies, they are ideal candidates for robust and reproducible monitoring of T-cell activity in vivo.
Introduction
T-cell immunity is critically involved in combating infections and cancer, as well as playing pathogenic roles in autoimmune disease and allergies. Therefore, monitoring antigen-specific T-cells and their effector functions is critical for the understanding of these diseases and for proper assessments of the efficacies of specific immune therapies. Yet, unlike the detection of antibodies, reliable measurement of T-cell-mediated immunity has, until now, continued to be a major challenge, this resulting from several factors. One such factor is that the antigen-specific T-cells of interest typically occur in very low frequencies in test samples such as peripheral blood. In addition, for an assay to reliably measure T-cell function(s), it needs to be warranted that the test conditions are such that the function of T-cells in vitro remains unimpaired relative to in vivo. The many variables that can affect T-cell functionality have earned T-cell assays the reputation of being rather fragile, with even minor changes of test conditions potentially having a major impact on the test results.
Further complicating the field of T-cell diagnostics, such assays traditionally have been carried out with freshly-isolated T-cells. While working with freshly-isolated cells may assure unimpaired functionality of the T-cells, it has the distinct disadvantage that assay results cannot be reproduced with the same cell material. Even in cases where the same subject is bled serially, the peripheral T-cell compartment will not be identical. Circadian rhythms and environmental influences such as nutrition, infections, and stress will change the cellular composition of the peripheral blood mononuclear cells (PBMC) and, subsequently, the functionality of the T-cells when tested in different bleeds of the same donor. Thus, when using freshly-isolated cell material, T-cell assay standardization is challenging even within a single laboratory, and assay harmonization among different laboratories has been close to impossible due to the difficulties inherent in transporting live cell material.
A major breakthrough in the field of T-cell monitoring has been the introduction of protocols that facilitate cryopreservation of PBMC such that, upon thawing, the cells retain their full functionality (Kreher et al., Citation2003). Thus, it has become possible to freeze multiple aliquots of PBMC obtained from a single bleed of a donor, thereby generating “reference PBMC.” In this way, one can use identical cell material for repeated testing in the same laboratory, or potentially in several remote facilities. Such cryopreserved PBMC are ideal tools for assay development and standardization, as well as providing the perfect platform for the comparison of T-cell assay performance in different laboratories.
Using such cryopreserved PBMC, it has recently been shown that T-cell assays can be used to precisely and stably quantify antigen-specific T-cell responses in PBMC involving highly trained personnel in tightly regulated laboratories that strictly adhere to standard operating procedures (SOPs) (Maecker et al., Citation2008). Several attempts have been undertaken to address the question of whether various different facilities are capable of generating reproducible data while testing the same PBMC reference samples (Cox et al., Citation2005; Janetzki et al., Citation2008; Britten et al., Citation2009). All of these efforts showed quite substantial variations in test results supporting the notion that harmonization efforts are needed before T-cell assays become robust enough to generate reproducible data across laboratories, and thus become suitable for immune monitoring in multicenter clinical trials (Britten et al., Citation2007).
The above-mentioned multicenter studies left open a fundamental question: Are results of functional T-cell assays inherently difficult to reproduce among laboratories due to the complexity of these assays and because of the live cell material being tested, or does the variability result from the different test conditions in the participating laboratories? A common feature of these trials was that expert laboratories were invited to participate and each laboratory was permitted to use their preferred reagents, locally established protocols, and favored data analysis methods. Because many experimental details of T-cell assays have not yet been standardized, these individual laboratories followed substantially variant protocols while performing the assays, possibly explaining the variability in the results.
We tested herein the hypothesis that T-cell assays will provide reproducible and robust data from different laboratories when all pertinent test conditions are standardized. In addition to standardizing the protocol as well as all reagents used in the assays, we also made an attempt to standardize the expertise level of the individuals performing the assays, using a somewhat unconventional approach: the personnel performing the tests for this trial were all selected based on the criterion that they had no previous experience with the T-cell assay in question, this being ELISPOT (although the laboratories in which they work may or may not have such expertise). In this way, we wanted to minimize the chance that habitual variations of trained investigators could influence the results, and to ensure that the protocol provided is indeed closely followed.
The results presented here show that antigen-specific T-cells can be detected with astounding precision by the interferon-γ (IFNγ) ELISPOT assay in different laboratories—even by inexperienced personnel—if the same protocol is followed, using the same reagents, and a user-independent platform for data analysis. Thus, the variability in previously reported studies involving all types of T-cell assays are not inherent consequences of working with live cell material in complex assay systems, but must have resulted from variations in the different approaches within the laboratories.
Materials and methods
Peripheral blood mononuclear cells
The human PBMC used for this study were selected from the ePBMC™ library of Cellular Technology Ltd. (CTL, Cleveland, OH). The PBMC in this library of donors are high-resolution HLA typed, and precharacterized for T-cell reactivity to common recall antigens. The cells had been obtained by leukapheresis, and were frozen using serum-free freezing reagents (CTL-Cryo™, by CTL) in up to 2000 identical aliquots. While the material used for this study has been a donation to facilitate this study, these cells are commercially available, permitting anyone to reproduce the results reported here. To minimize the chance of damage to the PBMC during transit, the cells were shipped to the participating laboratories in liquid nitrogen shippers (MVE Vapor Cryoshipper, Chart Industries, Marietta, GA) which maintain their temperature for more than 2 weeks, and the laboratories were instructed to transfer the cells to liquid nitrogen tanks upon receipt, where they would be stored until tested. The cells were thawed according to the detailed protocol provided by CTL using the serum-free thawing reagent CTL Anti-Aggregate™ Wash Supplement (from CTL), and were counted and re-suspended in the serum-free testing medium, CTL-Test™ (from CTL). Alternatively, as specified in the Results section, the cells were processed and tested with the respective laboratories’ serum of choice.
Antigen
The antigen used for this study was the HLA-A-2-restricted cytomegalovirus peptide NLVPMVATV (CMV pp65) (Wills et al., Citation1996). This peptide was provided as part of the commercially available Reference Sample QC Set from Cellular Technology Ltd.. The antigen was used at the concentration(s) specified in the Results section.
ELISPOT assay
All study participants used the human IFNγ ELISPOT kit by BD Biosciences (San Diego, CA, USA) and followed the manufacturer’s instruction sheet for using the kit. The PBMC were tested within 3 hr after thawing (without an overnight resting period). The PBMC were plated at 400,000 cells per well, except for where they were titrated in serial dilution, as specified.
Antigen-induced cytokine production was measured in quadruplicate wells, except for where single wells were tested. The assay results were analyzed on an ImmunoSpot® Series 5 UV Reader (CTL) using its automated software features for user-independent setting of counting parameters (SmartCount™) and gates (AutoGate™).
Statistical analysis
Except for , where single wells were tested, for each test condition the test was performed in quadruplicate wells. Means and standard deviations (SD) were calculated for the quadruplicate wells, and are shown in the figures. and show different representations of the same data set—the means and SD for this dataset are shown in . Spot counts represent spots counted per well, as counted, without the spot count in the medium control subtracted. When tested serum-free, the spot counts in the medium control were less than 10 spots per well for all figures—raw data for the serum-free medium spot counts are shown in . Stimulation indices (SI) were calculated as follows: SI = mean spot number in quadruplicate antigen-containing wells/mean spot number in quadruplicate medium containing negative control wells.
The full-length instructions and protocols provided to the study participants are available as supplementary material accompanying the online version of this article. The participants were asked to run two assays—the first one was intended for practice and the second one was the actual assay. In this paper, we report the results of the first “practice run” because it already produced impressive results—the results of the second assay were essentially the same as of the first one.
Results and discussion
Overall design of trial
In preparation for this trial, we placed particular emphasis on the thorough characterization of reference PBMC distributed to the participating laboratories. The PBMC were high-resolution HLA, typed. From the PBMC library of Cellular Technology Ltd. (CTL), 38 donors bearing the HLA-A2*0201 allele were selected. The PBMC of all 38 donors were tested in an IFNγ ELISPOT assay for reactivity to the A-2-restricted cytomegalovirus peptide NLVPMVATV (CMV pp65) (Wills et al., Citation1996), at 1 μg/mL. The response level, measured as spot number in ELISPOT, for the different donors ranged from zero to “too numerous to count” (TNTC) (data not shown). From this library, we selected three PBMC for further characterization, one each with a relatively high, medium, and low reactivity level. Antigen dose–response curves were established for these three donors to select a peptide concentration for plateau-level activation, and the frequencies of CMV pp65-reactive cells were defined by serial dilution of the cells in an IFNγ ELISPOT assay (see later). Moreover, we verified through cell separation experiments and intracytoplasmic IFNγ staining that the CMV pp65-induced IFNγ-producing cells are CD8 positive (data not shown).
The cells were repeatedly tested in IFNγ ELISPOT assays in CTL’s Reference Laboratory to establish inter- and intra-assay variation within a single laboratory when the test is performed under GLP-compliant conditions under strict adherence to established SOPs. The “reference value” was established as the mean spot number from the different tests performed in the Reference Laboratory.
Cryopreserved PBMC of the three CMV pp65-reactive donors were then shipped to all participating laboratories. The cells of each PBMC donor were from the same blood draw, thereby assuring that identical cell material was tested by all laboratories. The participating laboratories were then asked to defrost and test the cells in an IFNγ ELISPOT assay using the provided protocol and reagents, and the results obtained were compared with reference value as established in the CTL Reference Laboratory.
Antigen dose responsiveness of the reference PBMC
T-cell activation is antigen dose-dependent, whereby the dose–response curve reflects the functional avidity of the T-cell population to the antigen: high-avidity T-cells are stimulated by low concentrations of antigen, low-avidity T cells are stimulated by high concentrations of antigen (Targoni and Lehmann, Citation1998). In ELISPOT assays, titrating peptide-antigen typically results in a characteristic sigmoidal dose–response curve; the peptide concentration that induces 50% maximal activation representing the Keff value (Hesse et al., Citation2001). We tested the dose–response curves of three PBMC to CMV pp65 (), permitting us to select a peptide concentration for this study that induces maximal stimulation in all three PBMC: this was determined at 0.1 μg/mL.
Figure 1. Dose−response curves of the three reference PBMC after stimulation with CMV pp65. The cryopreserved cells were thawed and processed under serum free conditions and plated at 4 × 105 cells per well into an IFNγ ELISPOT assay. CMV peptide pp65 was titrated as specified. One well was tested at each peptide concentration. The number of spots induced is shown for each concentration and each sample.
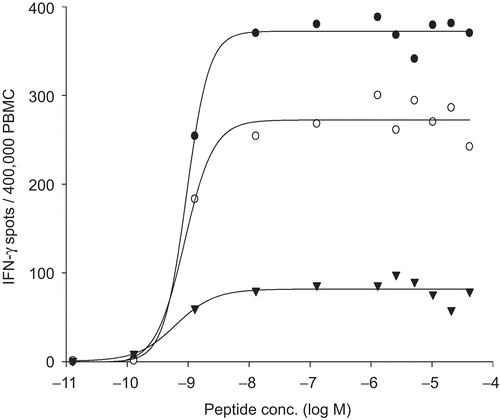
As this concentration lies along the “plateau” of the does–response curves for all three PBMC, we can thus assure that minor variations in peptide concentration due to operator variability would not affect the results. All subsequent data reported here were obtained using this peptide concentration. The choice of a single peptide antigen, as opposed to the complex Cytomegalovirus, Epstein-Barr virus, and Flu virus (CEF) control peptide pool (Currier et al., Citation2002) may have contributed to the reproducibility of the data reported in this study. The individual CEF peptides within the pool trigger T-cells with fundamentally different functional avidities (W. Zhang, unpublished data), and in addition, the individual peptides compete for MHC binding (Lehmann et al., Citation1989). Thus, while inter-experimental variations in the concentration of the CEF peptide pool used in the preceding multicenter studies might have contributed to the variable T-cell responses reported, in this study the potential stimulatory activity of the antigen was controlled.
Dependence of assay results on cell numbers plated
T-cell activation is dependent on the interaction of a T cell with an antigen-presenting cell. Ideal test conditions therefore will ensure that the two cell types are able to interact. In ELISPOT assays, such contacts are critically dependent on the density of the PBMC in the assay wells. To establish the dependence of ELISPOT assay results on PBMC numbers per well, we plated the reference samples in serial dilutions ranging from 1.6 × 106 to 5.0 × 105 PBMC per well. The peptide was tested at 0.1 μg/mL, and the number of IFNγ spots elicited was determined.
The results are summarized in . The number of PBMC plated was found to be in a direct linear relationship to the number of spots detected (R2 > 0.995) in the range between 5 × 104 and 4 × 105 cells per well for all three samples (the regression lines and correlation coefficients calculated for 400,000 to 50,000 PBMC per well are shown in ). On the basis of this result, a concentration of 4 × 105 cells per well was selected for this study. These data also established that cell counting or pipetting errors affecting the cell numbers plated will also affect the spot counts in direct proportionality. All subsequent data were obtained by testing 4 × 105 cells per well.
Figure 2. Relationship between PBMC number plated and IFNγ spots induced by peptide CMV pp65 in the reference PBMC. The three cryopreserved reference sample PBMC LP-51 (A) LP-37(B) LP-58(C) were thawed and plated in the specified cell numbers per well, in quadruplicate wells. Peptide CMV pp65 was added to each well at 0.1 μg/mL. The mean and standard deviation for each cell number is specified. The regression line was calculated for 4 × 105 to 5 × 104 PBMC per well (red line), with the correlation coefficient shown.
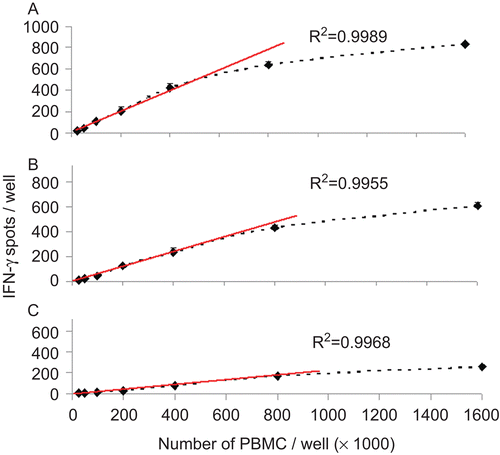
At cell concentrations higher than 8 × 105 per well, the linear relationship between cell and spot number was lost. One reason for this was an observed overlap or confluence of spots in samples where reactivity was high. shows raw as well as counted images for such wells.
Figure 3. Upper detection limit of IFNγ spot counting. Representative images are shown for data obtained with LP-51 when tested at the cell numbers specified. The test conditions are as described in . The top row shows the raw images, the bottom row the spot counts with the overlay of the counted spots over the raw image; the green outlines are generated by the ImmunoSpot software to mark spots that have been automatically recognized/counted by the software. The data illustrate how individual spots can be discretely discerned up ≈ 500 spots per well. Note also the linearity of the counts in . At higher numbers, confluence of spots and ELISA effects interfere with the countability of the data.
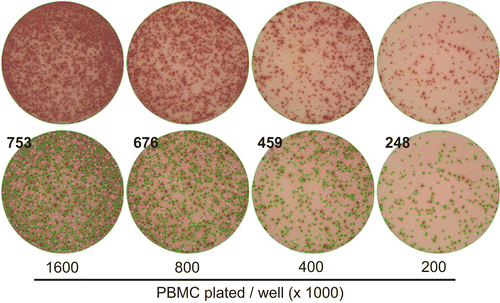
As is apparent from the images, discrete spots are observed at frequencies of up to 400 spots per well. Spot confluence and elevation of background due to “ELISA effects” start to occur at 8 × 105 cells per well in the high responding donor, making spot counts unreliable when they are above 600 spots per well. In this study, spot counts higher than 600 per well were considered “too numerous to count” or TNTC.
Establishing levels of intralaboratory variability for test results and determining a reference value
The PBMC of all three donors were tested in the Reference Laboratory of the CTL (in three different experiments) by a single ELISPOT-trained investigator in a GLP-compliant facility, following established SOPs (). Subsequently, the three PBMC samples were tested by three different ELISPOT- and GLP-trained investigators in the same laboratory following the same SOPs, with each investigator independently processing the samples through all steps of the assay (). In a separate experiment, we attempted to mitigate the effects of variability introduced during the processing of cells and in doing so, assess any potential variability in the performance of the ELISPOT assay itself. For this experiment, the three PBMC were thawed, washed, and counted by a single individual, and then the cells were split for independent testing by three investigators. The results are shown in .
Figure 4. Intra-laboratory reproducibility of data under GLP conditions. The three reference samples as specified by shading were tested in three independent experiments by a single individual (A) or by three different individuals, whereby each of them thawed and processed the cells independently (B). In (C), the cells were thawed in a separate experiment and processed by a single individual and subsequently split into three identical aliquots for handling by three independent investigators. The results obtained by each individual are represented by the grey bars (with the SD for triplicate wells) shown; the mean ± SD of the three independently-obtained data points are specified by the black bar. All the data were obtained in the Reference Laboratory while testing under GLP-compliant conditions.
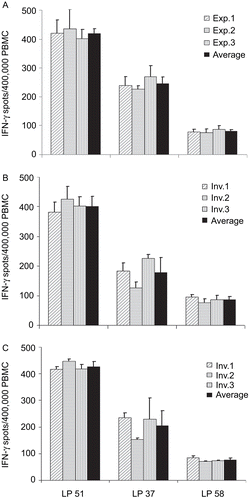
Since each of the five experiments shown in , , and represent an independent frequency measurement in the Reference Laboratory, we used the results of all five individual experiments to establish the reference values for each of the PBMC as the mean ± SD of the spot counts from the five experiments. The mean ± 3SD for the each of the three reference samples are shown on the left-hand side in . Medium controls are not shown, as they were < 10 spots per well in each of these experiments.
Figure 5. Inter-laboratory variability of the test results. Three reference samples shown in the left three panels (A: LP-51, B: LP-37, C: LP-58) were tested independently in eight different laboratories following identical protocols and using identical reagents, including the use of serum free media. Each dot on the right represents the test result obtained by one of these laboratories, with the mean for all laboratories shown by the red bar. The results of the individual tests performed in the Reference Laboratory (from , , and ) are represented by the dots on the right, with the mean shown by the red bar (representing the Reference Value for this study), and ± 3 SD shown by the hatched red line. Panel D shows the ranking hierarchy, whereby the results obtained by each laboratory are represented by a different color and are connected by a line in that color.
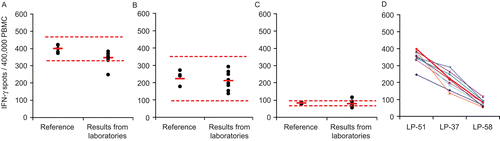
Establishing interlaboratory variability of test results
The three cryopreserved PBMC reference samples were distributed to 11 laboratories in 8 countries (e.g., Bulgaria, France, Greece, Latvia, Norway, Poland, Spain, the United Kingdom, and the United States). In addition, all participating laboratories were provided with identical serum-free thawing/washing, and testing media (CTL-Anti-Aggregate-Wash™ and CTL-Test™, respectively), as well as CTL’s detailed protocols for thawing and processing the cells. The laboratories received CMV pp65 peptide in a ready-to-use concentration, and were provided a human IFNγ ELISPOT kit (from BD Biosciences). Each participant performed the assays according to the manufacturer’s specifications. Importantly, all individuals performing the assay were ELISPOT-inexperienced. The plates were scanned and analyzed centrally at Nottingham Trent University, United Kingdom, using the automated analysis parameters of a CTL ImmunoSpot S5 UV Reader. The results obtained in eight laboratories are shown in and . Note that three laboratories failed to provide results: one of these reported that the cells were inadvertently thawed and refrozen before the assay was performed; one switched the washing and testing media during the preparation of the cells for the assay; and the third laboratory reported having mixed up the reference samples.
Figure 6. Assay performance in serum free test conditions versus serum-containing media. All participating laboratories tested the PBMC reference samples in serum free CTL-Test™ medium (SF, the black bars) or with the serum of their choice (S, the grey bars—these media contained 10% of the respective serum plus 1% L-glutamine in RPMI 1640). Panel (A) shows the CMV pp 65-induced spot counts. Panel (B) shows the spot counts in the respective media alone. In each of the panels, the data for the three reference PBMC (as specified) are grouped per laboratory being separated by the hatched line. Thus, within each hatched line a different serum was tested. Means (±1SD) are shown for each test condition done in quadruplicate wells. The last two rows show the stimulation index (SI) for each test condition serum-free or with the respective serum. SI was calculated as spot counts induced by antigen divided by spot count in the medium control. The data in this graph for the SF media are the same as in .
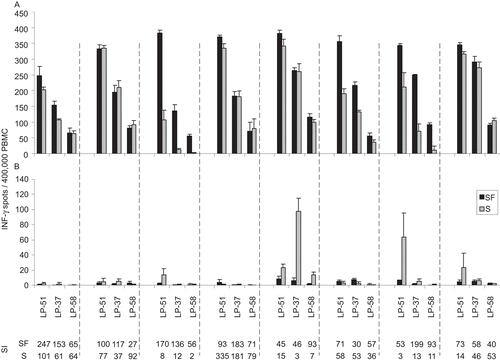
In all eight laboratories, for all three PBMC, the medium background was < 10 spots per well (when tested under serum-free conditions, see ) and all detected very clear-cut CMV pp65-induced responses (all stimulation indices were >27, shown and discussed later in ). Most of the spot counts obtained in the different laboratories were within three standard deviations (SD) of the Reference Value provided by the Reference Laboratory (). Moreover, each of the laboratories correctly established the response hierarchy/rank among the PBMC samples, with spot counts for LP-51 > LP-37 > LP-58 ().
Furthermore, deviations from the reference value tended to be systematic, that is, if one laboratory detected, for example, a lower spot number for one PBMC sample relative to another laboratory, the values for the other two PBMC tended to be also lower (). A likely explanation for such systematic deviations is to be sought in cell counting. Therefore, it would seem that, with more standardization for cell counting, and some practice with the technique, these already impressive data on interlaboratory reproducibility of results will move yet closer to agreement with each other. It should be reiterated that the individuals performing the assays were ELISPOT-inexperienced, and that these results represent their very first attempt at performing the assay.
Previous attempts to show reproducibility of T-cell assays using ELISPOT, Tetramers/Pentamers, ICS, and CFSE dilution indicated that there were substantial inter-laboratory variations (Cox et al., Citation2005; Janetzki et al., Citation2008; Britten et al., Citation2009). Because in those attempts each participating laboratory was permitted to use its favorite reagents and test conditions, it remained unclear whether T-cell assays are inherently hard to reproduce, or whether the variable outcomes resulted from the different test conditions used in the participating laboratories. The study here clearly answers this question for ELISPOT, and invites similar comparisons for other T-cell assays.
Influence of serum on the test results
Cellular assays in general, and T-cell assays in particular, have been traditionally performed using serum-containing media that rely on serum to provide the essential microenvironment for maintaining the viability and functionality of PBMC in culture. Only recently have serum-free media become available that are suited for cryopreservation and ex vivo testing of PBMC, such as the medium used to generate the above data. In addition to testing the samples on this common platform, with the same serum-free reagents used for thawing, washing (CTL Anti-Aggregate Wash™), and testing of the PBMC (CTL-Test™), the participating laboratories were asked to do a parallel experiment in which the cells were processed and tested using the serum of their choice—a serum which had been used in their respective laboratories for cell culture/T-cell assays. Thus, since in the same experiment, under otherwise identical conditions, the cells were tested with eight different sera vs. in the same serum-free medium, one can directly compare the assay performance under these conditions. As shown in , the spot counts in CTL-Test™ (the black bars) were either similar (Laboratories 1, 2, 4, 5, and 7) or significantly higher (Laboratories 3, 6, and 7) than those induced in the different serum-containing media. The medium background tended to be higher in the serum-containing media, and was disturbingly high in the serum of Laboratories 5 and 7 (). This resulted for most laboratories in an increased signal-to-noise ratio of assay performance in favor of the serum-free medium, expressed as stimulation index (SI = spot counts induced by antigen/spot counts in the medium control; see last two rows in ). These data establish that—while reproducible results among laboratories can be obtained using serum-free media—different sera used in the different laboratories can introduce variability in the assay results. The fact that serum can be a major assay variable might explain why this study, performed using serum-free conditions, showed much higher reproducibility of test results among laboratories than was seen in the previous attempts, in which each laboratory was permitted to use its own favored serum.
The higher reproducibility of results in serum-free media could benefit clinical trials in which there are multiple testing sites. For example, while the spot counts for LP-37 in serum-free media ranged from 130 to 300 spots, due to the low medium background, all laboratories reported strongly positive SI (ranging from 30 to 199; see ). In contrast, for the serum-containing media one of the laboratories recorded for LP-37 a borderline (SI = 3) and two laboratories a weakly-positive result with SI =12 and 13, respectively ().
Concluding remarks
For the scientific community, the notion that T-cell assays can be reliably performed, only in expert laboratories by highly-trained personnel, has become accepted wisdom. This notion, however, also implies that T-cell assays are inherently complex and their results hard to reproduce, which caused a large segment of the non-T-cell specialized research community to refrain from utilizing T-cell monitoring techniques. Pharmaceutical industry and governmental agencies have generally been reluctant to include T-cell assays in their immune monitoring efforts as well because it was believed to be very difficult—if not impossible—to validate and qualify T-cell assays. Regulatory agencies will only accept qualified assays for use in development of new drugs, or as surrogate clinical end points (van der Burg, Citation2008). Recently, ELISPOT assays have been qualified and validated in laboratories adhering to GLP, including in the reference laboratory of this study. The data reported here, showing reproducibility of T-cell assay results between different laboratories, may boost the confidence that, in the near future, T-cell assays will join antibody measurements for robust assessments of immunity both by regulatory agencies and a broader, non-T-cell-specialized scientific community.
Acknowledgments
We thank Cellular Technology Ltd. (CTL) for donating their reference PBMC, CMV pp65 peptide, and the serum-free wash- (CTL Anti-Aggregate-Wash) and test-media (CTL-Test). We also thank BD Biosciences for donating their human IFNγ ELISPOT kits. Most of all, we thank the directors of the laboratories who supported this project by assigning an ELISPOT inexperienced member of their staff to this project, namely Drs. C. Baxevanis, A. DeLeo, G. Gaudernack, F. Garrido, F. Jotereau, P. Laidler, A. Line, E. Tartour, E. Naumova, and R. Rees.
Declaration of interest: Dr. Lehmann is employed by CTL. CTL is the manufacturer of ELISPOT readers, of the PBMC, and serum-free media used in this study. Independent evaluation of the study was conducted by Dr. McArdle. The Authors alone are responsible for the content and writing of this paper. The Authors did not receive funding for this study.
References
- Britten, C. M., Janetzki, S., van der Burg, S. H., Gouttefangeas, C., and Hoos, A. 2007 Toward the harmonization of immune monitoring in clinical trials: Quo vadis? Cancer Immunol. Immunother. 57:285–288.
- Britten, C. M., Janetzki, S., Ben-Porat, L., Clay, T. M., Kalos, M., Maecker, H., Odunsi, K., Pride, M., Old, L., Hoos, A., and Romero, P. 2009. Harmonization guidelines for HLA-peptide multimer assays derived from results of a large-scale international proficiency panel of the Cancer Vaccine Consortium. Cancer Immunol. Immunother. DOI 10.1007/s00262-009-0681-z.
- Cox, J. H., Ferrari, G., Kalams, S. A., Lopaczynski, W., Oden, N., and D’souza, M. P. 2005. Results of an ELISPOT proficiency panel conducted in 11 laboratories participating in international human immunodeficiency virus type 1 vaccine trials. AIDS Res. Hum. Retrovirus. 21:68–81.
- Currier, J. R., Kuta, E. G., Turk, E., Earhart, L. B., Loomis-Price, L., Janetzki, S., Ferrari, G., Birx, D. L., and Cox, J. H. 2002. A panel of MHC Class I- restricted viral peptides for use as a quality control for vaccine trial ELISPOT assays. J. Immunol. Meth. 260:157–172.
- Hesse, M. D., Karulin, A. Y., Boehm, B. O., Lehmann, P. V., and Tary-Lehmann, M. 2001. A T-cell clone’s avidity is a function of its activation state. J. Immunol. 167:1353–1361.
- Janetzki, S., Panageas, K. S., Ben-Porat, L., Boyer, J., Britten, C. M., Clay, T. M., Kalos, M., Maecker, H. T., Romero, P., Yuan, J., Kast, W. M., and Hoos, A. 2008. Results and harmonization guidelines from two large-scale international ELISPOT proficiency panels conducted by the Cancer Vaccine Consortium (CVC/SVI). Cancer Immunol. Immunother. 57:303–315.
- Kreher, C. R., Dittrich, M. T., Guerkov, R., Boehm, B. O., and Tary-Lehmann, M. 2003. CD4+ and CD8+ cells in cryopreserved human PBMC maintain full functionality in cytokine ELISPOT assays. J. Immunol. Meth. 278:79–93.
- Lehmann, P. V., Cardinaux, F., Appella, E., Muller, S., Falcioni, F., Adorini, L., and Nagy, Z. A. 1989. Inhibition of T-cell response with peptides is influenced by both peptide-binding specificity of major histocompatibility complex molecules and susceptibility of T-cells to blocking. Eur. J. Immunol. 19:1071–1077.
- Maecker, H. T., Hassler, J., Payne, J. K., Summers, A., Comatas, K., Ghanayem, M., Morse, M. A., Clay, T. M., Lyerly, H. K., Bhatia, S., Ghanekar, S. A., Maino, V. C., Delarosa, C., and Disis, M. L. 2008. Precision and linearity targets for validation of an IFNγ ELISPOT, cytokine flow cytometry, and tetramer assay using CMV peptides. BMC Immunol. 9:9.
- Targoni, O. S., and Lehmann, P. V. 1998. Endogenous myelin basic protein inactivates the high avidity T-cell repertoire. J. Exp. Med. 187:2055–2063.
- van der Burg, S. H. 2008. Therapeutic vaccines in cancer: Moving from immunomonitoring to immunoguiding. Expert Rev. Vaccines 7:1–5.
- Wills, M., Carmichael, A., Mynard, K., Jin, X., Weekes, M., Plachter, B., and Sissons, J. 1996. The human cytotoxic T-lymphocyte (CTL) response to cytomegalovirus is dominated by structural protein pp65: Frequency, specificity, and T-cell receptor usage of pp65-specific CTL. J. Virol. 70:7569–7579.