Abstract
Human natural killer (NK) cells are a first-line immune defense against tumor cells and virally-infected cells. If their function is impaired, it leaves an individual more susceptible to cancer development or viral infection. The ability of compounds that contaminate the environment to suppress the function of NK cells could contribute to the increased risk of cancer development. There are a wide spectrum of compounds that significantly contaminate water and food that are consumed by humans, leading to accumulation of some of these compounds in human tissues. In the current study, we examined the ability of three such compounds to diminish the function of human NK cells. Triclosan (TC) is an antimicrobial agent used in a large number of antibacterial soaps. Nonylphenol (NP) is a degradation product of compounds used as surfactants and as stabilizers in plastics. 4,4′-Dichlorodiphenyltrichloroethane (DDT) is a pesticide that is mainly used to control mosquitoes. The compounds were examined for their ability to suppress NK function following exposures of 1 h, 24 h, 48 h, and 6 days. Each agent was able to substantially decrease NK lytic function within 24 h. At a concentration of 5 µM, both TC and NP inhibited NK lytic function by 87 and 30%, respectively; DDT decreased function by 55% at 2.5 µM. The negative effects of each of these compounds persisted and/or intensified following a brief (1 h) exposure to the compounds, indicating that the impairment of function cannot be eliminated by removal of the compound under in vitro conditions.
Introduction
Natural killer (NK) cells are the initial immune defense against development of cancers and viral infections. They are defined by the presence of the proteins CD56 and/or CD16 on their cell surface (Lotzova, Citation1993; Vivier et al., Citation2004). NK cells appear to be the predominant defense against tumor cells (Lotzova, Citation1993); they limit the spread of blood borne metastases and the development of primary tumors (Kiessling and Haller, Citation1978; Hanna, Citation1980). The important role of NK cells in protecting against viral infections has been demonstrated by the increased incidence of viral infection seen in individuals where this subset of lymphocytes was completely absent (Fleisher et al., Citation1982; Biron et al., Citation1989). Impairment of NK function by any agent could then leave an individual with increased risk of tumor development and/or viral infections. Previously, we have shown that a number of man-made chemicals that contaminate the environment are capable of suppressing the target-cell-destroying function (i.e., lytic function) of NK cells. These include organotin agents (Whalen et al., Citation1999, Citation2002, Citation2003a), carbamate pesticides (Whalen et al., Citation2003b; Wilson et al., Citation2004), and brominated flame-retardants (Hinkson and Whalen, Citation2009; Kibakaya et al., Citation2009), among others (Reed et al., Citation2004).
Triclosan (TC) is used widely in antibacterial soap products and other personal hygiene products, such as toothpaste (Bhargava and Leonard, Citation1996). It contaminates the aquatic environment (Koplin et al., Citation2002; Singer et al., Citation2002) and is found in human blood (Allmyr et al., Citation2006, Citation2008), breast milk (Allmyr et al., Citation2006), and urine (Calafat et al., Citation2008). TC enters the human system through absorption through the skin or by ingestion (Moss et al., Citation2000; Sandborgh-Englund et al., Citation2006). TC has been shown to disrupt the thyroid hormone–dependent aspects of metamorphosis in frogs (Veldhoen et al., Citation2006). It has also been shown to interfere with thyroid hormone regulation in rats (Crofton et al., Citation2007) and to act as an inhibitor of sulfotransferase and a glucuronosyltransferase enzymatic activity in human liver cytosolic and microsomal fractions (Wang et al., Citation2004).
Nonylphenol polyethoxylates (NPEOs) are used as surfactants in a variety of applications, such as in cleaning products, paints, and in herbicide and pesticide formulations (Chen et al., Citation2005). 4-Nonylphenol (NP) is one degradation product of NPEOs (Naylor et al., Citation1992) and a by-product of an antioxidant stabilizer in plastic products; as such, it can leach from plastics (Howe et al., Citation2001). As NP is very stable, it persists in the environment as evidenced by its presence in aquatic sediment and in rivers and lakes (Naylor et al., Citation1992). Consequently, NP has been found in drinking water (Clark et al., Citation1992). NP has been found in human blood samples at levels as high as 1 µM (Chen et al., Citation2005, Citation2008). In an in vitro study, NP was shown to have weak estrogenic activity in trout hepatocytes and in human breast cancer cell lines (White et al., Citation1994). NP has also been shown to have effects on immune system cells from exposed mice (Lee et al., Citation2003) and rats (Karrow et al., Citation2004), on ex vivo–exposed rat immune cells (Karrow et al., Citation2004), and on a human lymphocyte cell line (Yao et al., Citation2007).
DDT is used to control mosquitoes. Though it has been banned for use in the United States, it continues to be used in many countries (CDC, Citation2003). DDT can still be found in measureable quantities in blood samples from the US population (Thornton et al., Citation2002; Patterson et al., Citation2009). In Mexico, where DDT is still used, serum DDT levels as high as 23,169 ng/g of lipid have been noted (Koepke et al., Citation2004; Trejo-Acevedo et al., Citation2009). An association between blood levels of DDT and decreased numbers of NK cells as well as decreased NK function has been reported in humans (in vivo) (Svensson et al., Citation1994). Additionally, an association of DDT levels with interleukin-4 levels has been shown in humans (in vivo) (Huber et al., Citation2002). Previous studies have also shown that DDT could inhibit the lytic function of highly-purified human NK cells (Reed et al., Citation2004).
The study reported here examined the capacity of TC, NP, and DDT to disrupt the lytic function of human NK cells. The effects of continuous exposures (for up to as long as 6 days) to these compounds were investigated. Additionally, NK cells were exposed to TC, NP, or DDT for 1 h and then incubated as long as 6 days in compound-free media to determine whether any of the agents produced persistent or progressive loss of lytic function following a single brief exposure. Lasting (or developing) negative effects of such a brief exposure to any of these compounds would suggest a potential for greater toxicologic risk from exposure to these environmental contaminants.
Materials and methods
Isolation of NK cells
Peripheral blood from healthy adult (male and female) volunteer donors was used for this study. Buffy coats (source leukocytes) obtained from the American Red Cross (Portland, OR) or Key Biologics, LLC (Memphis, TN) were used to prepare NK cells. Highly-purified NK cells were obtained using a rosetting procedure. Buffy coats were mixed with 0.8 mL of RosetteSep human NK cell enrichment antibody cocktail (StemCell Technologies, Vancouver, British Columbia, Canada) per 45 mL of buffy coat. The mixture was incubated for 20 min at room temperature (∼25°C). Following the incubation, 7–8 mL of the mixture was layered onto 4 mL of Ficoll-Hypaque (ρ = 1.077 g/mL; MP Biomedicals, Irvine, CA) and centrifuged at 1200 x g for 30–40 min. The cell layer was then collected and washed twice with phosphate-buffered saline (pH 7.2) and stored in complete media [RPMI-1640 supplemented with 10% heat-inactivated bovine calf serum (BCS), 2 mM L-glutamine, and 50 U penicillin G/50 µg streptomycin/mL] at a concentration of 1 million cells/mL (Whalen et al., Citation2002). Freshly-isolated NK cells were used rather than a cell line; this was due to the fact that cell lines (being normally derived from cancerous cells) are inherently not “normal” and our interest was in examining the effects of these contaminants in/on normal cells.
Chemical preparation
TC (Calbiochem, La Jolla, CA), NP (Alpha Aesar, Ward Hill, MA), and DDT (Supelco, Bellefonte, PA) were each dissolved in dimethyl sulfoxide (DMSO; Sigma-Aldrich, St. Louis, MO) to yield 100 mM stock solutions. Desired concentrations of each compound were then prepared by dilution of the stock into complete media containing 0.5% gelatin (in place of 10% bovine serum). The final concentration of DMSO in any of the exposures did not exceed 0.01%.
As noted here, in these studies, gelatin was used in place of the serum. This was done because serum albumin has a well-known capacity to bind hydrophobic compounds, an effect that would interfere with delivery/diminish the amount of each test agent that might be available for interaction(s) with the NK cells. Nevertheless, in order to confirm that: any effects of each agent noted in the gelatin-utilizing studies might also potentially occur under in vivo scenarios (wherein albumin would be present); the effects reported in the gelatin-utilizing protocols are not misleading; and, any agent-induced effects are demonstrably comparatively skewed due to the expected binding effects of albumin, several of the studies herein were also performed using 10% bovine serum.
Cell treatments
NK cells (at a concentration of 1.5 × 106 cells/mL) were exposed at 37°C (in an air:CO2 (19:1) incubator) to compounds or control media for 1 h, 24 h, 48 h, or 6 days and then assayed for tumor-destroying function. In some studies, NK cells were also exposed to compounds for 1 h and, following this period, the media was removed and replaced with fresh compound-free media. The cells were then incubated in compound-free media for 24 h, 48 h, or 6 days before being assayed for tumor-destroying function.
Cell viability
Cell numbers and viability were assessed at the beginning and end of each exposure period by trypan blue exclusion. Treated and control cells that had been exposed to trypan blue were counted using a hemocytometer. Viability was determined at each concentration for each of the exposure periods. The viability of treated cells was then compared to that of control cells at each length of exposure (Whalen et al., Citation2003b). Only those concentrations where viability was unaffected were used at a given length of exposure.
Cytotoxicity assay
The ability of NK cells to lyse tumor cells was measured using a [51Cr] release assay (Whalen et al., Citation1999). The target cell in all cytotoxicity assays was the NK-susceptible K562 (human chronic myelogenous leukemia) cell line. Briefly, K562 cells were incubated with [51Cr] (as Na2[51Cr]O4; Perkin-Elmer Life Sciences, Boston, MA) in 0.2–0.5 mL of unadulterated BCS (i.e., BCS containing no additions) for 1.0–1.5 h at 37°C in an air:CO2 (19:1) incubator. Following this incubation, the target cells were washed twice with gelatin media. NK (effector) cells (1.2 × 105/100 µL for 12:1 ratio with target cells) were added to the wells of round-bottom micro-well plates. The effector cells were diluted to a 6:1 (0.6 × 105/100 µL) and a 3:1 ratio (0.3 × 105/100 µL); each ratio was tested in triplicate. Target cells were added (1 × 104/100 µL) to each well of the micro-well plate; the plate was then centrifuged at 300 × g for 3.5 min and incubated for 2 h at 37°C in air:CO2 (19:1). After this incubation, a 0.1 mL aliquot of supernatant was collected from each well to assess radioactivity (in 60 sec) in a Packard COBRA γ-radiation counter (Packard Instrument Co., Meriden, CT). Target lysis was then calculated as: 100 × [test cpm − spontaneous cpm]/[maximum cpm − spontaneous cpm]. Maximum release was produced by adding 100 µL of 10% Triton X-100 to wells containing only the labeled target cells; spontaneous release was assessed from wells containing cells plus medium only.
Statistical analysis
The data from treated cells at a given concentration were compared to control data by pair-wise analysis, using Student’s t-test at a specific length of incubation. One-way analysis of variance followed by a post hoc Bonferroni correction was used to compare data from different time points or different concentrations of the compounds.
Results
Lytic function of NK cells exposed to TC for 1 h, 24 h, 48 h, and 6 days
NK cells were exposed to 2.5–10 µM TC for 1 h and then tested for their ability to lyse tumor cells. Both 5 and 10 µM TC caused very significant reductions in NK cell lytic function after only 1 h [46.3 (± 20.6)% and 91.7 (± 5.7)%, respectively] (P < 0.0001; ). The concentration range used for the 24-h exposures to TC was 0.5–5.0 µM. There was a decrease in NK cell viability with exposure to 10 µM TC for 24 h, and thus it was not used to analyze lytic function. The decrease in lytic function after a 24-h exposure to 2.5 µM TC was 64.3 (± 11.0)% (P < 0.0001), whereas that at 5 µM was 87.4 (± 5.6)% (P < 0.0001; ). When NK cells were exposed to these same TC levels for 48 h, there were again very substantial decreases in lytic function at 2.5 and 5 µM TC [65.7 (± 26.8)% and 87.2 (± 9.6)%, respectively] (P < 0.0001; ). The 6-day exposures of NK cells to TC were carried out at concentrations of 0.5–5.0 µM. There was a significant decrease in tumor cell–lysing function with a 6-day exposure to all concentrations of TC except 0.5 µM (). Decreases in lytic function were 32.2 (± 10.4)% at 1 µM, 76.7 (± 10.8)% at 2.5 µM, and 85.6 (± 9.4)% at 5 µM TC (P < 0.0001; ). NK cells treated with 2.5 µM TC for 24 h in the presence of 10% bovine serum showed a 25.4 (± 5.7)% decrease in lytic function compared to control cells (data not shown—available on request).
Figure 1. Effects of triclosan (TC) exposures on the ability of natural killer (NK) cells to lyse tumor cells. NK cells were exposed to 0.5 – 10 µM TC for 1 h, 24 h, 48 h, or 6 days. To combine results from separate experiments (using cells from different donors) the levels of lysis were normalized as the percentage of the lytic function of the control cells in a given experiment. Results were from three separate experiments using different donors (triplicate determinations for each experiment; n = 9, mean ± SD). *Statistically significant change as compared to control cells at that same length of incubation (P < 0.05).
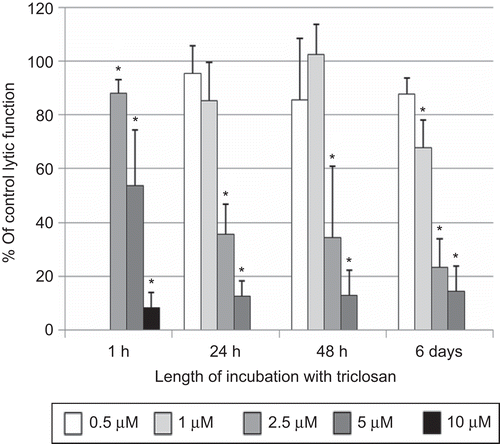
Lytic function of NK cells exposed to TC for 1 h, followed by 24 h, 48 h, and 6 days in TC-free medium
In order to assess whether a brief exposure of NK cells to TC could cause lasting and/or ensuing effects on lytic or binding function, NK cells were exposed to 2.5–10 µM TC for 1 h, which was removed, and the NK cells were then suspended in TC-free media for 24 h, 48 h, or 6 days before assaying for lytic function. As described above, NK cells exposed to 10 µM TC for 1 h and then tested immediately for lytic function showed decreases in lytic function of 46.3 (± 20.6)% at 5 µM TC and 91.7 (± 5.7)% at 10 µM TC. Although 10 µM TC caused a decrease in NK cell viability when the exposure was 24 h or more, there was no decrease in NK cell viability with a 1-h exposure. When NK cells were exposed to 5 and 10 µM TC for 1 h, and then incubated in TC-free media for 24 h before assaying lytic function, there was no significant decrease at 5 µM TC. However, there remained a very significant decrease at the 10 µM level [67.0 (± 21.2)%; ]. At 48 h after a 1-h exposure to TC, there was still a significant decrease from the 10 µM exposure [57.3 (± 25.0)%]; 6 days following a 1-h exposure, there were significant decreases at both the 5 and 10 µM exposures [28.9 (± 9.4)% and 74.9 (± 16.2)%, respectively; ].
Figure 2. Effects of 1-h exposures to triclosan (TC) followed by 24 h, 48 h, or 6 days in TC-free media on the ability of natural killer (NK) cells to lyse tumor cells. NK cells were exposed to 2.5–10 µM TC for 1 h. Results were from at least three separate experiments using different donors (triplicate determinations for each experiment; n = 9, mean ± SD), as described in . *Statistically significant change as compared to control cells at that same length of incubation (P < 0.05).
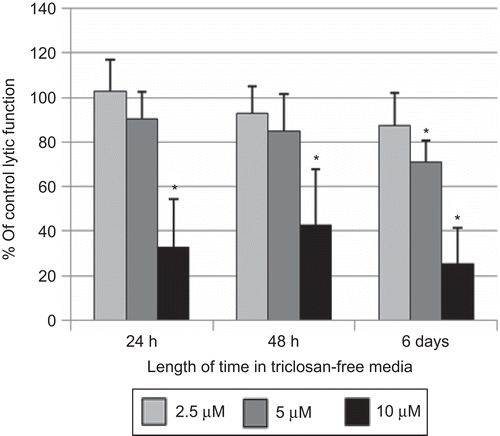
Lytic function of NK cells exposed to NP for 1 h, 24 h, 48 h, and 6 days
Exposure of NK cells to 10 µM NP for 1 h significantly decreased the lytic function of NK cells by 39.0 (± 30.4)% (P < 0.01; ). The 24-h exposures to 5 and 10 µM NP decreased lytic function by 30.1 (± 19.3)% and by 50.6 (± 9.5)%, respectively (P < 0.01; ). The concentration range used for the 48-h exposures to NP were no greater than 5 µM, as there was a decrease in NK cell viability with exposure to 10 µM NP. There was a decrease in lytic function after a 48-h exposure to 5 µM NP of 27.1 (± 21.7)% (P < 0.01; ). NK cells exposed to NP for 6 days showed significant decreases in lytic function at all of the concentrations of NP. Decreases in lytic function were 21.0 (± 10.7)% at 1 µM, 37.6 (± 27.2)% at 2.5 µM, and 56.2 (± 20.8)% at 5 µM NP (P < 0.01; ). NK cells treated with 5 µM NP for 24 h in the presence of 10% bovine serum showed a 26.1 (± 6.4)% decrease in lytic function compared to control cells (data not shown—available on request).
Figure 3. Effects of nonylphenol (NP) exposures on the ability of natural killer (NK) cells to lyse tumor cells. NK cells were exposed to 1–10 µM NP for 1 h, 24 h, 48 h, or 6 days. Results were from three separate experiments using different donors (triplicate determinations for each experiment, n = 9, mean ± SD), as described in . *Statistically significant change as compared to control cells at that same length of incubation (P < 0.05).
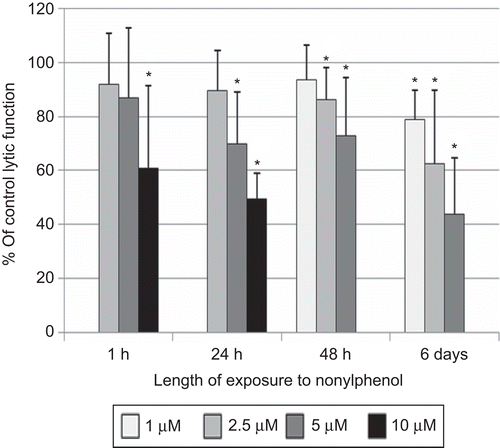
Lytic function of NK cells exposed to NP for 1 h, followed by 24 h, 48 h, and 6 days in NP-free medium
Exposure of NK cells to 10 µM NP for 1 h caused ≈40% decrease in lytic function (). Cells exposed to 10 µM NP for 1 h and then incubated for 24 h in NP-free media before being tested for lytic function showed a 44.9 (± 18.3)% (P < 0.01) decrease in lytic function (). Although exposure of NK cells to 5 µM NP for 1 h caused no significant loss of lytic function () there was an 15.8 (± 12.8)% (P < 0.01) decrease in lytic function 24 h following a 1-h exposure to 5 µM NP (). When NK cells were exposed to 5 and 10 µM NP for 1 h and then incubated in NP-free media for 48 h before assaying for lytic function, there were significant decreases at 5 µM [37.4 (± 27.5)%, P < 0.001] and 10 µM [64.3 (± 29.9)%, P < 0.001] NP (). Six days following a 1-h exposure to 2.5 µM NP, there was a decrease in lytic function of 47.7 (± 27.5)% (P < 0.001), whereas those seen with 5 and 10 µM were 48.4 (± 15.6) and 82.4 (± 8.0)%, respectively (P < 0.001; ).
Figure 4. Effects of 1-h exposures to nonylphenol (NP) followed by 24 h, 48 h, or 6 days in NP-free media on the ability of natural killer (NK) cells to lyse tumor cells. NK cells were exposed to 2.5–10 µM NP for 1 h. Results were from at least three separate experiments using different donors (triplicate determinations for each experiment; n = 9, mean ± SD), as described in . *Statistically significant change as compared to control cells at that same length of incubation (P < 0.05).
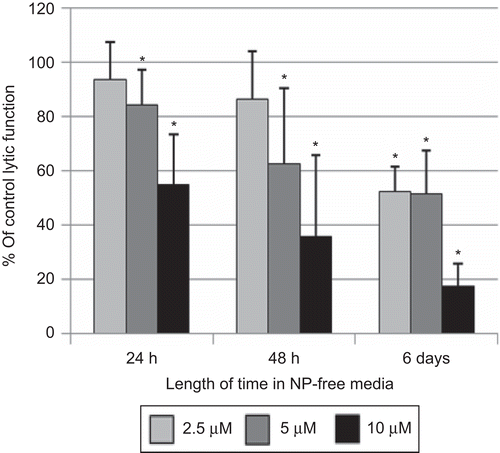
Lytic function of NK cells exposed to DDT for 1 h, 24 h, 48 h, and 6 days
Exposure of NK cells to DDT for 1 h (at concentrations as high as 10 µM) had no effect on the lytic function of NK cells (). However, a 24-h exposure to 2.5 µM DDT decreased lytic function by 55.4 (± 18.0)% (P < 0.001; ). Concentrations > 2.5 µM caused decreased viability of NK cells at 24 h. After a 48-h exposure to 2.5 µM DDT, lytic function was decreased by 68.9 (± 21.6)% (P < 0.001; ); after a 6-day exposure, the decrease from the 2.5 µM dose was 86.4 (±8.1)% (P < 0.001). There were also significant decreases at 0.25, 0.50, and 1.00 µM DDT—after a 6-day exposure—of 19.3 (± 15.0)%, 22.1 (± 8.8)%, and 22.2 (± 9.8)%, respectively (P < 0.01; ). NK cells treated with 2.5 µM DDT for 24 h in the presence of 10% bovine serum showed a 19.5 (± 6.7)% decrease in lytic function compared to control cells (data not shown—available on request).
Figure 5. Effects of DDT exposures on the ability of natural killer (NK) cells to lyse tumor cells. NK cells were exposed to 0.25–10 µM DDT for 1 h, 24 h, 48 h, or 6 days. Results were from three separate experiments using different donors (triplicate determinations for each experiment; n = 9, mean ± SD), as described in . *Statistically significant change as compared to control cells at that same length of incubation (P < 0.05).
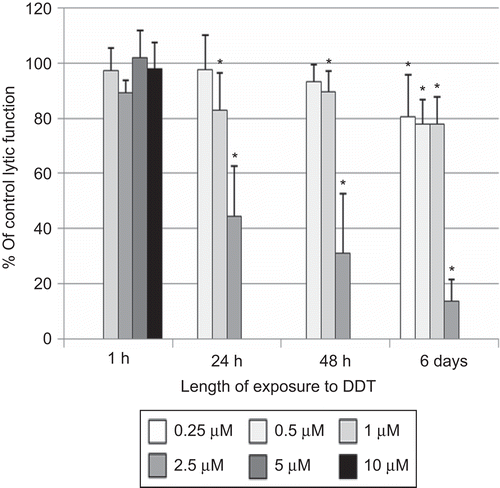
Lytic function of NK cells exposed to DDT for 1 h, followed by 24 h, 48 h, and 6 days in DDT-free medium
When NK cells were exposed to concentrations of DDT as high as 10 µM for 1 h, there was no decrease in lytic function (). However, if those cells were then incubated for 24 h in DDT-free media following the 1-h exposure, a loss of lytic function ensued (). Exposure to 2.5 µM DDT for 1 h caused a 34.8 (± 7.8)% decrease in lytic function 24 h after the compound had been removed; 5 µM DDT caused an 84.5 (± 12.2)% loss of function. There was no decrease in NK cell viability when the cells were exposed to 5 µM DDT for 1 h, which was subsequently removed. When NK cells were examined for lytic function 48 h following 1-h exposures to 2.5 and 5 µM DDT, decreases in lytic function were the same as those seen after 24 h in DDT-free media [33.7 (10.1)% and 83.5 (± 9.3)%, respectively; ]. A 6-day period in DDT-free media following a 1-h exposure to DDT caused decreases of 30.9 (± 31.0)% at 1 µM, 72.9 (± 15.6)% at 2.5 µM, and 95.1 (± 9.1)% at 5 µM DDT (P < 0.01) ().
Figure 6. Effects of 1 h exposures to DDT followed by 24 h, 48 h, or 6 days in DDT-free media on the ability of natural killer (NK) cells to lyse tumor cells. NK cells were exposed to 1–5 µM DDT for 1 h. Results were from at least three separate experiments using different donors (triplicate determinations for each experiment; n = 9, mean ± SD), as described in . *Statistically significant change as compared to control cells at that same length of incubation (P < 0.05).
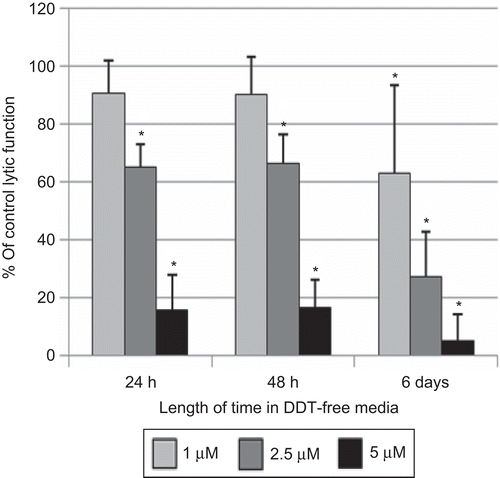
Discussion
The agents TC and NP are both very significant environmental contaminants. Both TC (Allmyr et al., Citation2006, Citation2008) and NP (Chen et al., Citation2005, Citation2008) are found in human blood and thus have the potential to interact with immune system cells that exist in the peripheral blood. NK cells are required for immune responsiveness to tumor cells and virally-infected cells and compromise of their function by any agent could result in increased occurrences of cancer/viral infection (Kiessling and Haller, Citation1978; Hanna, Citation1980; Fleisher et al., Citation1982; Biron et al., Citation1989; Lotzova, Citation1993).
The detrimental effects of these compounds in humans have not been thoroughly investigated. It is important to investigate the ability of these compounds to interfere with the essential immune function of human NK cells. The current study examines whether either of these compounds is capable of interfering with the lytic function of NK cells. Additionally, it examines whether a brief exposure to TC or NP can cause lasting and or increasing loss of lytic function. Previous studies have shown that other environmental contaminants can produce a lasting and/or progressive loss of lytic function following a brief (1 h) exposure in vitro (Whalen et al., Citation1999, Citation2002, Citation2003a; Taylor et al., Citation2005; Hinkson and Whalen, Citation2009; Kibakaya et al., Citation2009).
DDT has previously been shown to decrease the lytic function of NK cells both in vitro (Reed et al., Citation2004) and in vivo (Svensson et al., Citation1994). However, its capacity to produce lasting and/or ensuing negative effects on NK lytic function was not addressed. It is found in human blood and in some cases at very high levels (Thornton et al., Citation2002; Koepke et al., Citation2004; Patterson et al., Citation2009; Trejo-Acevedo et al., Citation2009). Thus, it was important to assess whether there was a persistent and/or progressive loss of NK lytic function with brief exposures to DDT.
The results of the current study indicated that both TC and NP have the capacity to decrease the lytic function of human NK cells. TC (10 µM) was able to block NK function by > 90% within 1 h. The negative effects of TC on NK lytic function appeared to increase with length of exposure to the compound; for instance, 2.5 µM TC had no effect on lytic function at 1 h but blocked roughly 65% of lytic function after 24 h and > 75% after 6 days. As little as 1 µM TC caused a > 30% loss of lytic function at 6 days. In comparison, NP was less effective at decreasing lytic function than TC, exemplified by the fact that TC blocked ≈77% and NP blocked ≈38% of lytic function after a 6-day exposure at the equivalent 2.5 µM dose. The effectiveness of each of these compounds (as well as that of DDT) was diminished when the exposure to the compounds occurred in the presence of serum; however, they nevertheless were still able to cause decreases in NK function. The fact that DDT has also been shown to inhibit NK cell function in vivo is consistent with our observation that the compounds remain effective toxicants, even in the presence of serum.
An initial exposure to TC for 1 h, which was followed by 24 h, 48 h, or 6 days in TC-free media, caused a loss of lytic function that persisted but did lessen to some extent. However, NP caused a progressive loss of lytic function following a 1-h exposure. DDT was more effective than either TC or NP at blocking NK function and also caused very significant persistent and progressive loss of lytic function.
The persistent, and in the cases of NP and DDT progressive, negative effects of these compounds on NK lytic function indicate that even brief exposures can cause lasting and sometimes increasing loss of this important immune function. This type of effect on human NK lytic function has been seen with other categories of environmental contaminants including organotin compounds, brominated flame retardants, carbamates, and organochlorines. Tributyltin, dibutyltin (Whalen et al., Citation2002), and triphenyltin (Whalen et al., Citation2003a) each cause lasting and progressive loss of lytic function (in vitro) after an initial exposure. This is also true for the carbamate pesticide ziram (Taylor et al., Citation2005), the brominated flame retardants hexabromocyclododecane (Hinkson and Whalen, Citation2009) and tetetrabromobisphenol A (Kibakaya et al., Citation2009), and the organochlorine pentachlorophenol (Taylor et al. Citation2005).
The effects of each of the above-mentioned compounds on NK function were not due to a general toxicity that caused the cells to die, as evidenced by the lack of effect on cell viability at the doses employed in these studies. However, these agents each were able to prevent the NK cells from being able to kill their target at the tested doses. Thus, it appears that these compounds interfere with processes required for NK lytic function. Mitogen-activated protein kinases (MAPKs) are important in the NK lytic process (Trotta et al., Citation1998, Citation2000; Wei et al., Citation1998). We have shown in previous studies that organotin compounds were able to alter the activation of state of MAPKs in NK cells as part of the mechanism by which they decrease NK lytic function (Aluoch et al., Citation2006). Future investigations will examine whether compounds such as TC, NP, and DDT are also able to interfere with MAPK function in NK cells or whether their mechanism of interference is distinct from that seen with organotins.
The likelihood an individual having two or more environmental contaminants present in their bloodstream appears to be significant based on recent body burden studies (Thornton et al., Citation2002) as well as other studies showing significant blood levels of a number of different contaminants (Kannan et al., Citation1999; Koepke et al., Citation2004; Chen et al., Citation2005, Citation2008; Allmyr et al., Citation2006, Citation2008; Patterson et al., Citation2009; Trejo-Acevedo et al., Citation2009). Thus, it is essential to understand the effects of these contaminants on the function of human blood components such as NK lymphocytes whose proper functioning is vital in protecting individuals from cancers and viral infections. Taken together, the current study—in concert with past studies—suggests a wide range of environmental contaminants are capable of interfering with NK cell function and that the induced interference is not reversible, under in vitro conditions.
Declaration of interest
This research was supported by Grant 2S06GM-08092-34 from the National Institutes of Health.
References
- Allmyr, M., Adolfsson-Erici, M., McLachlan, M. S., and Sanborgh-Englund, G. 2006. Triclosan in plasma and milk from Swedish nursing mothers and their exposure via personal care products. Sci. Total Environ. 372:87–93.
- Allmyr, M., Harden, F., Toms, L. M., Mueller, J. F., McLachlan, M. S., Adolfsson-Erici, M., and Sandborgh-Englund, G. 2008. The influence of age and gender on triclosan concentrations in Australian human blood serum. Sci. Total Environ. 393:162–167.
- Aluoch, A. O., Odman-Ghazi, S. O., and Whalen, M. M. 2006. Alteration of an essential NK cell signaling pathway by low doses of tributyltin in human natural killer cells. Toxicology 224:229–237.
- Bhargava, H. N., and Leonard, P. A.1996. Triclosan: Applications and safety. Am. J. Infect. Control 24:209–218.
- Biron, C. A., Byron, K. S., and Sullivan, J. L. 1989. Severe herpes virus in an adolescent without natural killer cells. New Engl. J. Med. 320:1731–1735.
- Calafat, A. M., Ye, X., Wong, L. Y., Reidy, J. A., and Needham, L. L. 2008. Urinary concentrations of triclosan in the U.S. population: 2003-2004. Environ. Health Perspect. 116:303–307.
- Centers for Disease Control and Prevention (CDC). 2003. 2nd National Report on Human Exposure to Environmental Chemicals. 2003. Atlanta, GA.
- Chen, M. L., Lee, W. P., Chung, H. Y., Guo, B. R., and Mao, I. F. 2005. Biomonitoring of alkylphenols exposure for textile and housekeeping workers. Int. J. Environ. Anal. Chem. 85:335–347
- Chen, M. L., Chang, C. I., Shen, Y. J., Hung, J. H., Gao, B. R., Chuang, H. Y., and Mao, I. F. 2008. Quantification of prenatal exposure and maternal-fetal transfer of nonylphenol. Chemosphere 73:5239–5245.
- Clark, L. B., Rosen, R. T., Hartman, T. G., Louis, J. B., Suffet, I. H., and Lippincott, R. L. 1992. Determination of alkylphenol ethoxylates and their acetic acid derivatives in drinking water by particle beam liquid chromatography/electrospray mass spectrometry. Int. J. Environ. Anal. Chem. 47:167–180
- Crofton, K. M., Paul, K., de Vito, M. J., and Hedge, J. M. 2007. Short-term in vivo exposure to the water contaminant triclosan: Evidence for disruption of thyroxine. Environ. Toxicol. Pharmacol. 24:194–197.
- Fleisher, G., Koven, N., Kamiya, H., and Henle, W. 1982. A non-X-linked syndrome with susceptibility to severe Epstein-Bar virus infections. J. Pediatr. 100:727–730.
- Hanna, N. 1980. Expression of metastatic potential of tumor cells in young nude mice is correlated with low levels of natural-killer cell-mediated cytotoxicity. Int. J. Cancer 26:675–690.
- Hinkson, N. C., and Whalen, M. M. 2009. Hexabromocyclododecane decreases the lytic function and ATP levels of human natural killer cells. J. Appl. Toxicol. 29:656–661.
- Howe, S. R., Surana, P., Jakupca, M. R., and Borodisky, L. 2001. Potential dietary exposure to p-nonylphenol from food contact use of tris (nonylphenyl) phosphate (TNPP). Food Addit. Contam. 18:1021–1039.
- Huber, D. V. Bauer, K. Suesal, C. Conradt, C., and Opelz, G. 2002. Associations of dichlorophenyltrichloroethane (DDT) 4.4. and dichlorodiphenyldichloroethylene (DDE) 4.4 blood levels with plasma IL-4. Arch. Environ. Health 57:541–547.
- Kannan, K., Senthilkumar, K., and Giesy, J. P. 1999. Occurrence of butyltin compounds in human blood. Environ. Sci. Technol. 33:1776–1779.
- Karrow, N. A., Guo, T. L., Declos, K. B., Newbold, R. R., Weis, C., Germolec, D. R., White, K. L., and McCay, J. A. 2004. Nonylphenol alters the activity of splenic NK cells and the numbers of leukocyte subpopulations in Sprague-Dawley rats: A two-generation feeding study. Toxicology 196:237–245.
- Kibakaya, E. C., Stephen, K., and Whalen, M. M. 2009. Tetrabromobisphenol A has immunosuppressive effects on human natural killer cells. J. Immunotoxicol. 6:285–292.
- Kiessling, R., and Haller, O. 1978. Natural killer cells in the mouse, an alternative surveillance mechanism? Contemp. Topics Immunobiol. 8:171–201.
- Koepke, R., Warner, M., Petreas, M., Cabria, A., Danis, R., Hernandez-Avila, M., and Eskenazi, B. 2004. Serum DDT and DDE levels in pregnant women of Chiapas. Mexico. Arch. Environ. Health 59:559–565.
- Koplin, D. W., Furlong, E. T., Meyer, M. T., Thurman, E. M., Zaugg, S. D., Barber, L. B., and Buxton, H. T. 2002. Pharmaceuticals, hormones, and other organic wastewater contaminants in U. S. Streams, 1999-2000: A national reconnaissance. Environ. Sci. Technol. 36:1201–1211.
- Lee, M. H., Chung, S. W., Kang, B. Y., Park, J., Lee, C. H., Hwang, S. Y., and Kim, T. S. 2003. Enhanced interleukin-4 production in CD4+ T-cells and elevated immunoglobulin E levels in antigen-primed mice by bisphenol A and nonylphenol, endocrine disruptors: Involvement of nuclear factor-AT and Ca2+. Immunology 109:76–86.
- Lotzova, E. 1993. Definition and function of natural killer cells. Nat. Immunol. 12:177–193.
- Naylor, C. G., Mieure, J. P., Adams, W. J., Weeks, J. A., Castaldi, F. J., Ogle, L. D., and Romano, R. R. 1992. Alkylphenol ethoxylates in the environment. J. Am. Oil Chem. Soc. 69:695–703.
- Moss, T., Howes, D., and Williams, F. M. 2000. Percutaneous penetration and dermal metabolism of triclosan (2,4,4′-tricloro-2′-hydroxydiphenyl ether). Food Chem. Toxicol. 38:361–370
- Patterson, D. G., Jr., Wong, L. Y., Turner, W. E., Caudill, S. P., Dipietro, E. S., McClure, P. C., Cash, T. P., Osterloh, J. D., Pirkle, J. L., Sampson, E. J., and Needham, L. L. 2009. Levels in the U. S. population of those persistent organic pollutants (2203-2004) included in the Stockholm Convention or in other long-range trans-boundary air pollution agreements. Environ. Sci. Technol. 43:1211–1218.
- Reed, A., Dzon, L., Loganathan, B. G., and Whalen, M. M. 2004. Immunomodulation of human natural killer cell cytotoxic function by organochlorine pesticides. Human Exp. Toxicol. 23:463–471.
- Sandborgh-Englund, G., Adolfsson-Ericic, M., Oldham, G., and Ekstrand, J. 2006. Pharmacokinetics of triclosan following oral ingestion in humans. J. Toxicol. Environ. Health 69:1861–1873.
- Singer, H., Muller, S., Tixier, C., and Pillonel, L. 2002. Triclosan: Occurrence and fate of a widely used biocide in the aquatic environment. Field measurements in wastewater treatment plants, surface waters, and lake sediments. Environ. Sci. Technol. 36:4998–5004.
- Svensson, B. G., Hallberg, T., Nilsson, A., Schütz, A., and Hagmar, L. 1994. Parameters of immunological competence in subjects with high consumption of fish contaminated with persistent organochlorine compounds. Int. Arch. Occup. Environ. Health 65:351–358.
- Taylor, T. R., Tucker, T., and Whalen, M. M. 2005. Persistent inhibition of human natural killer cell function by ziram and pentachlorophenol. Environ. Toxicol. 20:418–424.
- Thornton, J. W., McCally, M., and Houlihan, J. 2002. Biomonitoring of industrial pollutants: Health and policy implications of the chemical body burden. Public Health Rep. 117:315–323.
- Trejo-Acevedo, A., Diaz-Barriga, F., Carrizales, L., Dominguez, G., Costilla, R., Ize-Lema, I., Yarto-Ramirez, M., Gavilan-Garcia, A., Mejia-Saavedra, J. J., and Perez-Maldonado, I. N. 2009. Exposure assessment of persistent organic pollutants and metals in Mexican children. Chemosphere 74:974–980.
- Trotta, R., Puorro, K. A., Paroli, M., Azzoni, L., Abebe, B., Eisenlohr, L.C., and Perussia, B. 1998. Dependence of both spontaneous and antibody-dependent, granule exocytosis-mediated NK cell cytotoxicity on extracellular signal-regulated kinases. J. Immunol. 161:6648–6656.
- Trotta, R., Fettuciari, K., Azzoni, L., Abebe, B., Puorro, K.A., Eisenlohr, L.C., and Perussia, B. 2000. Differential role of p38 and c-Jun N-terminal kinase-1 mitogen-activated protein kinases in NK cell cytotoxicity. J. Immunol. 165:1782–1789.
- Veldhoen, N., Shirrow, R. C., Osachoff, H., Wigmore, H., Clapson, D. J., Gunderson, M. P., Van Aggelen, G., and Helbing, C. C. 2006. The bactericidal agent triclosan modulates thyroid hormone-associated gene expression and disrupts post-embryonic anuran development. Aquat. Toxicol. 80:217–227.
- Vivier, E., Nunes, J. A., and Vely, F. 2004. Natural killer cell signaling pathways. Science 306:1517–1519.
- Wang, L. Q., Falany, C. N., and James, M. O. 2004. Triclosan as a substrate and inhibitor of 3′-phosphoadenosine 5′-phosphosulfate-sulfotransferase and UDP-glucuronosyl transferase in human liver fractions. Drug Metab. Disp. 32:1162–1169.
- Wei, S., Gamero, A. M., Liu, J. H., Daulton, A. A., Valkov, N. I., Trapani, J. A., Larner, A. C., Weber, M. J., and Djeu, J. Y. 1998. Control of lytic function by mitogen-activated protein kinase/extracellular regulatory kinase-2 (ERK2) in a human natural killer cell line: Identification of perforin and granzyme B mobilization by functional ERK2. J. Exp. Med. 187:1753–1765.
- Whalen, M. M., Loganathan, B. G., and Kannan, K. 1999. Immunotoxicity of environmentally-relevant concentrations of butyltins on human natural killer cells in vitro. Environ. Res. 81:108–116.
- Whalen, M. M., Green, S. A., and Loganathan, B. G. 2002. Brief butyltin exposure induces irreversible inhibition of the cytotoxic function on human natural killer cells in vitro. Environ. Res. 88:19–29.
- Whalen, M. M., Wilson, S., Gleghorn, C., and Loganathan, B. G. 2003a. Brief exposure to triphenyltin produces irreversible inhibition of the cytotoxic function of human natural killer cells. Environ. Res. 92:213–220.
- Whalen, M. M., Loganathan, B. G., Yamashita, N., and Saito, T. 2003b. Immunomodulation of human natural killer cell cytotoxic function by triazine and carbamate pesticides. Chem.-Biol. Interact. 145:311–319.
- White, R., Jobling, S., Hoare, S. A., Sumpter, J. P., and Parker, M. G. 1994. Environmentally-persistent alkylphenolic compounds are estrogenic. Endocrinology 135:175–182.
- Wilson, S., Dzon, L., Reed, A., Pruitt, M., and Whalen, M. M. 2004. Effects of extended, in vitro, exposures to low levels of organotin and carbamate pesticides on human natural killer cell cytotoxic function. Environ. Toxicol. 19:554–563.
- Yao, G., Ling, L., Luan, J., Ye, D., and Zhu, P. 2007. Nonylphenol induces apoptosis of Jurkat cells by a caspase-8 dependent mechanism. Int. Immunopharmacol. 7:444–453.