Abstract
Ultrafine nanoparticles of zinc oxide (ZnO) recently became available as a substitute for larger-size fine ZnO particles. However, the biological activity of ultrafine ZnO currently remains undefined. In the present study, we investigated the effect of ultrafine ZnO on oral tolerance that plays an important role in the prevention of food allergy. Oral tolerance was induced in mice by a single oral administration (i.e., gavage) of 25 mg of ovalbumin (OVA) 5 days prior to a subcutaneous immunization with OVA (Day 0). Varying doses of ultrafine (diameter: ≈ 21 nm) as well as fine (diameter: < 5 µm) ZnO particles were given orally at the same time during the OVA gavage. The results indicated that a single oral administration of OVA was followed by significant decreases in serum anti-OVA IgG, IgG1, IgG2a, and IgE antibodies and in the proliferative responses to the antigen by these hosts’ spleen cells. The decreases in these immune responses to OVA were associated with a marked suppression of secretion of interferon (IFN)γ, interleukin (IL)-5, and IL-17 by these lymphoid cells. Treatment with either ultrafine or fine ZnO failed to affect the oral OVA-induced suppression of antigen-specific IgG, IgG1, IgG2a, and IgE production or lymphoid cell proliferation. The suppression induced by the oral OVA upon secretion of IFNγ, IL-5, and IL-17 was also unaffected by either size of ZnO. These results indicate that ultrafine particles of ZnO do not appear to modulate the induction of oral tolerance in mice.
Keywords::
Introduction
It is long recognized that oral administration of antigen induces immunological unresponsiveness to the antigen termed oral tolerance (Mowat, Citation1987; Weiner et al., Citation1994). Although the exact mechanism underlying induction of oral tolerance still remains obscure, possibilities include deletion (Chen et al., Citation2002), anergy of antigen-specific lymphocytes (Whitacre et al., Citation1991), and suppression by inhibitory cytokines including transforming growth factor (TGF)-β and interleukin (IL)-10 secreted from regulatory T-lymphocytes (Khoury et al., Citation1992; Chen et al., Citation1994). Oral tolerance is thought to contribute to the prevention of food allergy (Mowat, Citation1994). It was previously demonstrated that oral tolerance was modulated by environmental factors. For instance, diesel-exhaust particles (DEP) that have stimulatory effects as an adjuvant on immune responses (Yoshino and Sagai, Citation1999) have the ability to block induction of oral tolerance (Yoshino et al., Citation1998).
Fine particles of zinc oxide (ZnO) have been used for cosmetics, drugs, and paints and categorized as a nontoxic chemical according to the Globally Harmonized Classification System for the classification of chemicals (Scientific Committee on Cosmetic Products and Non-food Products, Citation2003). Recently, ultrafine ZnO consisting of nanoparticles was developed as a substitute for fine ZnO. However, the biological activities of the nanoparticles have not been defined well. There is a possibility that ultrafine ZnO might affect the mucosal immune system when it is exposed to humans since nanoparticles appear to be readily absorbed from mucosal tissues (Jani et al., Citation1990; Cross et al., Citation2007; Gulson et al., Citation2008). This suggests that oral tolerance might be modulated by such very small size of particles. Moreover, our recent studies showed that ultrafine ZnO had an ability to enhance immune responses in mice (Matsumura et al., Citation2010) as DEP did. These results encouraged us to investigate the effect of ultrafine ZnO on induction of orally-induced immunological unresponsiveness.
In the present study, we unexpectedly found that ultrafine as well as fine ZnO lacked the ability to modulate oral tolerance. Our results suggest that ultrafine ZnO appears to be a relatively safe compound as fine ZnO in terms of the induction of food allergy.
Materials and methods
Animals
Male 6-week-old BALB/c mice were used in all experiments. The mice were purchased (at 5 weeks-of-age) from Charles River Laboratories Japan, Inc. (Shiga, Japan) and acclimated for 1 week prior to use in the experiments herein. Throughout these studies, the mice were maintained in polycarbonate cages held within an on-site temperature- (20–26°C) and light-controlled (12 h/12 h on/off) pathogen-free environment, and provided free access to standard rodent chow (CRF-1; Oriental Yeast Co., Ltd., Tokyo, Japan) and filtered-and UV-irradiated-water. All experiments outlined herein were performed according to the Ethical Guidelines established by Shionogi & Co., Ltd. and approved by the Institutional Animal Care and Use Committee (IACUC).
Induction of oral tolerance
Ovalbumin (OVA; Sigma-Aldrich Co., St. Louis, MO; 25 mg dissolved in 0.5 mL phosphate-buffered saline (PBS)) was orally administered once by gastric intubation (i.e., gavage) on Day −5 before immunization with OVA (Day 0). As a nonspecific protein antigen control, 0.5 mL of PBS alone or 0.5 mL of PBS containing 25 mg of hen egg lysozyme (HEL; Sigma) was utilized in place of the OVA.
Administration of ZnO
Ultrafine (Ishihara Sangyo Kaisha, Ltd., Osaka, Japan) and fine (Wako Pure Chemical Industries, Ltd., Osaka) particles of ZnO had a diameter of 21 nm and < 5 µm, respectively. The shapes and sizes of both the ultrafine and fine particles in the administration solution (PBS containing 50 mg/mL OVA) were analyzed by transmission electron microscopy (TEM). The samples were mounted on Formvar-coated copper grids (Okenshoji Co., Ltd., Tokyo, Japan) and then examined on a JEOL JEM-1010 transmission electron microscope (JEOL; Japan Electronics Company, Tokyo) at an accelerating voltage of 80 kV (). Ultrafine (0.3, 3, and 30 mg) and fine (30 mg) ZnO particles were given orally immediately after they were suspended in PBS containing OVA on Day −5, i.e., at the time of oral administration of the antigen. PBS (0.5 mL) alone was given as a control. There were no significant differences in body weight gains between the control and ZnO-treated mice (data not shown).
Immunization
On Day 0, all mice were immunized subcutaneously at the base of the tail with 100 µg of OVA dissolved in 50 µL of PBS (pH 7.2) and emulsified with an equal volume of complete Freund’s adjuvant (CFA; Difco laboratories, Detroit, MI).
Measurement of OVA-specific antibodies
Blood was collected in SEPACLEAN A-5 tubes (Eiken Chemical Co., Ltd., Tokyo, Japan) on Day 21 and the tubes were centrifuged to separate serum that was then harvested and stored at −20°C until analysis. IgG, IgG1, IgG2a, and IgE antibodies specific for OVA were measured using an enzyme-linked immunosorbent assay (ELISA). For the measurement of IgG, IgG1, and IgG2a, 96-well flat-bottomed microtiter plates were incubated with 100 µL/well of OVA (100 µg/mL) at 4°C overnight and washed three times with PBS. The wells were then blocked by incubation with 200 µL of PBS containing 1% (w/v) casein (Sigma) at 37°C for 1 h. After washing, the plates were incubated with 100 µL of 1:50,000 (for IgG measures), 1:50,000 (for IgG1), or 1:1000 (for IgG2a) dilutions of each serum sample at 37°C for 1 h. The plates were then washed, and 100 µL/well of a 1:1000 dilution of goat anti-mouse IgG conjugated with alkaline phosphatase (Sigma), or rat anti-mouse IgG1 (or IgG2a) labeled with alkaline phosphatase (BD Pharmingen, San Diego, CA), was added and incubated at 37°C for 1 h. After washing, 100 µL of 3 mM p-nitrophenyl phosphate solution (Bio-Rad Laboratories, Hercules, CA) was added to each well and the plates were incubated in the dark (at room temperature) until a bright yellow reaction product was noted. The absorbance was then measured at 405 nm using a VersamMax microplate reader (MDS Inc., Sunnyvale, CA). All results were expressed in terms of mean absorbance units at OD405 (A405; ± SEM).
For the measurement of IgE, 96-well flat-bottomed microtiter plates were incubated with 100 µL of 1 µg/mL rat anti-mouse IgE (Monosan, Uden, the Netherlands) in each well at 37°C for 1 h and washed three times with SuperBlock Blocking Buffer (Pierce Biotechnology, Inc., Rockford, IL). The plates were incubated with 100 µL of a 1:5 dilution of each serum sample at 37°C for 1 h. The plates were washed with PBS containing 0.05% (v/v) Tween 20 detergent, and 100 µL/ well of 1 µg biotinylated OVA/mL was added and incubated at 37°C for 1 h. After washing, 100 µL of a 1 µg/mL solution of streptavidin conjugated with horseradish peroxidase (Vector Laboratories, Inc., Burlingame, CA) was added to each well and incubated at 37°C for 1 h. After washing, 100 µL of the 3,3′,5,5′-tetramethylbenzidine (TMB) peroxide substrate solution (Pierce) was added to each microplate well and the plates were incubated at room temperature for 30 min. The reaction was stopped by adding 100 µL of 2 M sulfuric acid to each well. The absorbance was then measured at 450 nm using a VersamMax microplate reader (MDS Inc.). All results were expressed as mean absorbance units at OD450 (A450; ± SEM).
Proliferation assay
Spleens were removed on Day 21 and cell suspensions prepared using protocols outlined in Yamaki et al. (Citation2003). Erythrocytes in the suspension were first lysed with Tris–NH4Cl, and then the remaining cells counted. From this suspension, a total of 5 × 105 cells (in 0.1 mL of RPMI 1640 (Sigma) containing 1 mM l-glutamine, 100 U penicillin/mL, 100 µg streptomycin, 40 µM 2-mercaptoethanol, and 10% (v/v) heat-inactivated fetal bovine serum) was placed into each microwell of a 96-well plate that then did or did not receive an OVA bolus (i.e., at 500 µg OVA/mL final concentration in well). After 48 h of culture at 37°C (under a 5% CO2 atmosphere), each well was pulsed with 0.5 µCi of [3H]-thymidine (GE Healthcare UK Ltd., Buckinghamshire, UK) and the cells were cultured for a further 6 h. The cells in each well were then harvested onto fiberglass filters using a multi-harvester (PerkinElmer. Inc., Waltham, MA) and counted using standard liquid scintillation techniques. All results were expressed as the average total counts per minute (cpm) from triplicate cultures of cells pooled from six mice.
Measurement of cytokines
One milliliter of RPMI 1640 containing a total of 5 × 106 spleen cells was cultured in 24-well tissue culture plates in medium alone or medium with 100 µg OVA/mL. After 4 days, the supernatants were harvested and stored at −20°C until assayed. Secretion of interferon (IFN)-γ, IL-5, and IL-17 was quantified using ELISA kits (Endogen, Inc., Woburn, MA for IFNγ and IL-5 measures; R&D Systems Inc., Minneapolis, MN for IL-17 measures).
Statistics
To analyze data statistically, one-way ANOVA followed by the Dunnett’s parametric multiple t-test was used to compare the data obtained with samples from each group against those from mice in the PBS control group.
Results
Effect of ZnO on the suppression by oral OVA of production of anti-OVA IgG antibodies and proliferative responses of spleen cells to the antigen
To induce oral tolerance, mice were each provided OVA by gavage (once) 5 days prior (i.e., Day −5) to immunization with OVA (Day 0). As shown in , the oral administration of OVA was followed by a significant suppression of anti-OVA IgG antibody production. In contrast, HEL gavaged in place of OVA (as a control) failed to modulate the antibody production. Mice orally given 0.3, 3, or 30 mg of ultrafine ZnO, or 30 mg of fine ZnO, concomitant with the OVA had similar levels of anti-OVA antibodies as those noted in the mice that had been orally-tolerized (i.e., exposed to OVA alone on Day −5). Both ultrafine and fine ZnO particles also failed to affect the suppression of proliferative responses of the hosts’ spleen cells to OVA that were associated with the oral administration of this antigen ().
Figure 2. Effect of zinc oxide (ZnO) on the suppression of anti-ovalbumin (anti-OVA) antibody production and spleen cell proliferation by cells from hosts that had been gavaged with the test antigen. All mice were immunized with OVA on Day 0. To induce oral tolerance, mice were gavaged once with 25 mg of OVA dissolved in phosphate-buffered saline (PBS) on Day −5. As controls, PBS or 25 mg hen egg lysozyme (HEL) were provided in place of OVA at the time of gavage. To examine the effect of ZnO on oral tolerance, PBS, 0.3, 3, or 30 mg of ultrafine ZnO, or 30 mg of fine ZnO were orally administered at the same time when the mice were gavaged. (A) Effect of ZnO on the suppression of anti-OVA IgG antibody production due to earlier oral exposure to the antigen. Serum samples were collected on Day 21 and assayed for anti-OVA IgG antibodies by enzyme-linked immunosorbent assay (ELISA). Values are expressed as mean (± SEM) of samples from six mice/regimen. (B) Effect of ZnO on the suppression of spleen cell proliferative responses to OVA among cells from hosts that had been gavaged with the antigen. On Day 21, spleens were recovered from the mice, and their cells isolated, pooled, and then incubated with OVA (500 µg/mL) for 48 h. Background values for cell proliferation in the absence of OVA ranged from 6000 to 9000 counts per minute (cpm). Values are expressed as mean (± SEM) of triplicate samples from culture supernatants of cells pooled from six mice. Y-axis tags indicate what the mice received during gavage on Day −5. **p < 0.01 and ***p < 0.001 versus PBS treatment group (Dunnett’s test).
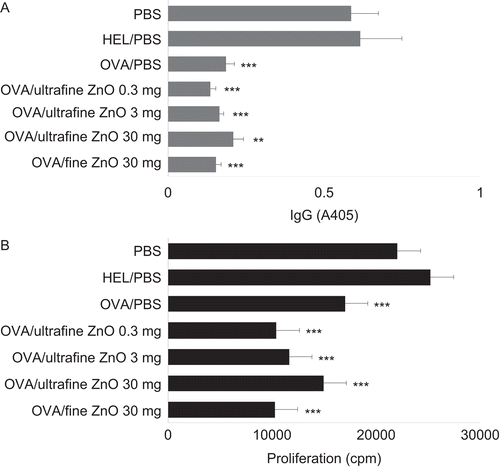
Effect of ZnO on the suppression by oral OVA of production of TH1 (IgG2a) and TH2 (IgG1 and IgE) cell-dependent anti-OVA antibodies
Next, whether ZnO is able to modulate lower levels of anti-OVA IgG2a (TH1 cell-dependent) and IgG1 and IgE (TH2 cell-dependent) in tolerized mice was investigated. As shown in , the levels of anti-OVA IgG2a (A), IgG1 (B), and IgE (C) in mice gavaged with OVA were unaffected by any of the doses of ultrafine and ultra ZnO tested.
Figure 3. Effect of zinc oxide (ZnO) on the suppression of anti-ovalbumin (anti-OVA) IgG2a, IgG1, and IgE antibody production by cells from hosts that had been gavaged with the test antigen. All mice were immunized with OVA on Day 0. To induce oral tolerance, mice were gavaged once with 25 mg of OVA dissolved in phosphate-buffered saline (PBS) on Day −5. As controls, PBS or 25 mg hen egg lysozyme (HEL) were provided in place of OVA at the time of gavage. To examine the effect of ZnO on oral tolerance, PBS, 0.3, 3, or 30 mg of ultrafine ZnO, or 30 mg of fine ZnO were administered at the same time when the mice were gavaged. Serum samples were collected Day 21 and individually assayed for anti-OVA (A) IgG2a, (B) IgG1, and (C) IgE antibodies by enzyme-linked immunosorbent assay (ELISA). Values are expressed as mean (± SEM) of six mice. *p < 0.05 and ***p < 0.001 versus PBS treatment group (Dunnett’s test). Y-axis tags indicate what the mice received during gavage on Day −5.
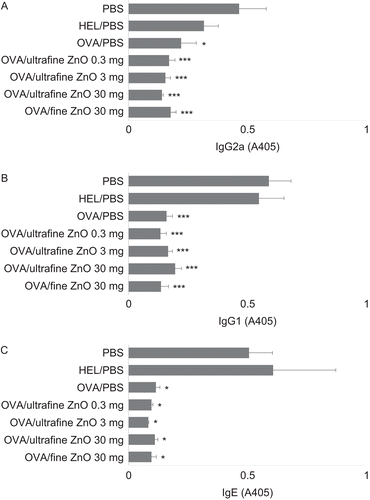
Effect of ZnO on the suppression by oral OVA of cellular cytokine secretion
The effects of both sizes of ZnO on the suppression of secretion of TH1 (IFNγ) and TH2 (IL-5) cytokines as a result of the OVA gavage were also investigated. Furthermore, any effect of ZnO co-treatment on TH17 cell-dependent IL-17 secretion was also examined. The exposure to either ultrafine or fine ZnO failed to modulate the “lower” levels of IFNγ () and IL-5 () while that was seen with cells from mice that had been given OVA alone on Day −5. Both sizes of ZnO also failed to affect the suppression of IL-17 secretion associated with the Day −5 oral OVA treatment alone ().
Figure 4. Effect of zinc oxide (ZnO) on the suppression of interferon (IFN)γ, interleukin (IL-5), and IL-17 secretion by cells from hosts that had been gavaged with the test antigen. All mice were immunized with ovalbumin (OVA) on Day 0. To induce oral tolerance, mice were gavaged once with 25 mg of OVA dissolved in phosphate-buffered saline (PBS) on Day −5. As controls, PBS or 25 mg hen egg lysozyme (HEL) were provided in place of OVA at the time of gavage. To examine the effect of ZnO on oral tolerance, PBS, 0.3, 3, or 30 mg of ultrafine ZnO, or 30 mg of fine ZnO were administered at the same time when the mice were gavaged. On Day 21, spleens were removed and splenocytes were incubated with (solid columns) or without (open columns) 100 µg OVA/mL followed by measurement of IFNγ (A), IL-5 (B), and IL-17 (C) in the culture supernatant. Values are expressed as mean (± SEM) concentrations (of each cytokine) of triplicate samples from culture supernatants of cells pooled from six mice. Y-axis tags indicate what the mice received during gavage on Day −5. *p < 0.05, **p < 0.01, and ***p < 0.001 versus PBS treatment group (Dunnett’s test).
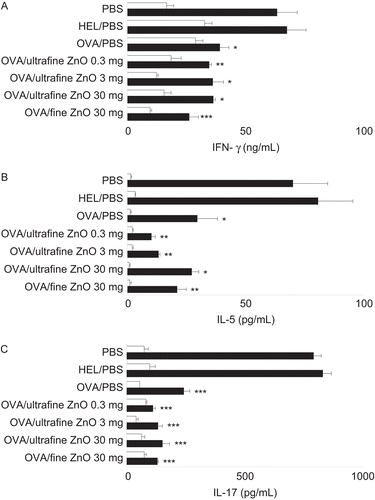
Discussion
The present study demonstrates that ultrafine ZnO does not appear to affect induction of oral tolerance that is critically involved in the prevention of food allergy (Mowat, Citation1994) since the treatment of mice with ultrafine as well as fine ZnO failed to modulate the suppression of anti-OVA IgG, IgG2a, IgG1, and IgE antibody production as a result of oral administration of OVA. The suppression of proliferative responses of spleen cells to OVA in orally-tolerized animals was also unaffected by both sizes of ZnO. The failure of modulation of oral tolerance by these ZnO was associated with that of cellular secretion of IFNγ, IL-5, and IL-17.
Ultrafine ZnO consisting of nanoparticles were recently developed as a substitute for the larger size of fine ZnO. However, the biological activities of the newly-developed ultrafine ZnO have not been defined well. There is evidence that nanoparticles are readily absorbed from mucosal tissues (Jani et al., Citation1990; Cross et al., Citation2007; Gulson et al., Citation2008). This suggests that oral tolerance induced by the mucosal immune system in the gut might be modulated by such very small size of particles. In addition, our recent studies revealed that treatment of mice with ultrafine ZnO was followed by the enhancement of immune responses, especially on TH2 responses (Matsumura et al., Citation2010). The similar adjuvant effect was observed when mice were exposed to DEP (Yoshino and Sagai, Citation1999). At least some of compounds that have adjuvant effects have been demonstrated to break immune tolerance, causing autoimmune diseases. For instance, CFA has been widely used as an adjuvant for induction of experimental autoimmune diseases including adjuvant arthritis (Shahrara et al., Citation2008), collagen-induced arthritis (Notley et al., Citation2009), and experimental autoimmune encephalomyelitis (Farez et al., Citation2009). More importantly, DEP is able to block the induction of oral tolerance (Yoshino et al., Citation1998). However, the treatment with ultrafine ZnO unexpectedly failed to modulate induction of oral tolerance.
Although the reason why ultrafine ZnO failed to modulate oral tolerance is unknown at present, it may be simply explained by the difference in the mechanism between adjuvant action and induction of oral tolerance. The ability of CFA to break immune tolerance stated above is shown when it is given systemically but not orally. CFA breaks already existing immunological unresponsiveness when it is administered with self-antigens. It is unknown whether CFA given orally is able to block induction of oral tolerance. Another explanation of the failure of the modulation of oral tolerance by ZnO is that the adjuvant effect of ultrafine ZnO might be weaker than DEP so that the ability of ZnO to block induction of oral tolerance was not sufficient while that of DEP is greater enough to prevent the orally-induced unresponsiveness. Yoshino and Sagai (Citation1999) demonstrated that mice exposed to DEP had enhanced production of HEL-specific IgG2a and IgG1 that was associated with increased secretion of IFNγ and IL-4, respectively, suggesting that DEP appears to have adjuvant activity on both TH1 and TH2 immune responses. On the other hand, Matsumura et al. (Citation2010) revealed that treatment of mice with OVA plus ZnO was followed by greater increases in anti-OVA IgG1/-IgE and IL-4/IL-5, but not in anti-OVA IgG2a and IFNγ compared with that with OVA alone, indicating that ZnO seems to selectively stimulate TH2 but not TH1 responses. The blockade of induction of oral tolerance by DEP was associated with marked prevention of the suppression of both TH1 and TH2 secretion by oral antigen.
ZnO failed to affect lower levels of such cytokines in orally tolerized animals. Furthermore, the difference in toxicity between ZnO and DEP might also explain the difference in the effect on oral tolerance since the toxic effect of chemical compounds might be related to the damage of the mucosal immune system, resulting in the modulation of induction of oral tolerance. DEP has been shown to contain a number of organic toxic compounds that enhance lung injury via the production of proinflammatory cytokine and chemokines (Takano et al., Citation2002). In contrast, especially fine ZnO has been utilized as a safe chemical (Scientific Committee on Cosmetic Products and Non-food Products, Citation2003). Ultrafine ZnO might also be a relatively safe compound, at least less toxic than DEP.
In the present study, we observed that oral administration of OVA was followed by decreased levels of IL-17 that had been recently found as a cytokine produced by TH17 cells (Weaver et al., Citation2007). Although a role of IL-17 in oral tolerance has not been defined, our results appear to support the study by Ehirchiou et al. (Citation2007) who demonstrated that CD11b-deficient mice were more resistant to oral tolerance and that the resistance to the immunological unresponsiveness was associated with higher secretion of IL-17, suggesting that decreased secretion of IL-17 appears to be associated with induction of oral tolerance. Furthermore, our studies also showed that ZnO failed to modulate the decreased levels of IL-17 as well as IFNγ and IL-5 in orally -tolerized mice.
Conclusions
The recently developed ultrafine nanoparticles of ZnO do not appear to modulate the induction of oral tolerance that plays a critical role in the prevention of food allergy. The failure of modulation of orally induced immunological unresponsiveness by the ultrafine ZnO was associated with that of the secretion of IFNγ, IL-5, and IL-17. Ultrafine ZnO may be a relatively safe compound as fine ZnO used so far in terms of the induction of food allergy.
Declaration of interest
This work was in part supported by a Grant-in-Aid for Scientific Research (C) from the Ministry of Education, Science, Sports, and Culture of Japan.
References
- Chen, Y., Inobe, J., Marks, R., Gonnella, P., Kuchroo, V. K., and Weiner, H. L. 2002. Peripheral deletion of antigen-reactive T-cells in oral tolerance. Nature 376:177–180.
- Chen, Y., Kuchroo, V. K., Inobe, J., Hafler, D. A., and Weiner, H. L. 1994. Regulatory T-cell clones induced by oral tolerance: Suppression of autoimmune encephalomyelitis. Science 265:1237–1240.
- Cross, S. E., Innes, B., Roberts, M. S., Tsuzuki, T., Robertson, T. A., and McCormick, P. 2007. Human skin penetration of sunscreen nanoparticles: In vitro assessment of a novel micronized zinc oxide formulation. Skin Pharmacol. Physiol. 20:148–154.
- Ehirchiou, D., Xiong, Y., Xu, G., Chen, W., Shi, Y., and Zhang, L. 2007. CD11b facilitates the development of peripheral tolerance by suppressing TH17 differentiation. J. Exp. Med. 204:1519–1524.
- Farez, M. F., Quintana, F. J., Gandhi, R., Izquierdo, G., Lucas, M., and Weiner, H. L. 2009. Toll-like receptor 2 and poly (ADP-ribose) polymerase 1 promote central nervous system neuro-inflammation in progressive EAE. Nat. Immunol. 10:958–964.
- Gulson, B., Wong, H., McCall, M., Casey, P., Trotter, J., McCulloch, M., Greenoak, M., and Stauber, J. 2008. Dermal absorption of ZnO nanoparticles in sunscreen using the stable isotope approach. Toxicol. Lett. 180:S222.
- Jani, P., Halbert, G. W., Langridge, J., and Florence, A. T. 1990. Nanoparticle uptake by the rat gastrointestinal mucosa: Quantitation and particle size dependency. J. Pharm. Pharmacol. 42:821–826.
- Khoury, S. J., Hancock W. W., and Weiner, H. L. 1992. Oral tolerance to myelin basic protein and natural recovery from experimental autoimmune encephalomyelitis are associated with down-regulation of inflammatory cytokines and differential up-regulation of transforming growth factor beta, interleukin-4, and prostaglandin E expression in the brain. J. Exp. Med. 176:1355–1364.
- Matsumura, M., Nagata, M., Nakamura, K., Kawai, M., Baba, T., Yamaki, K., and Yoshino, S. 2010. Adjuvant effect of zinc oxide on TH2 but not TH1 immune responses in mice. Immunopharmacol. Immunotoxicol. 32:56–62.
- Mowat, A. M. 1994. Oral tolerance and regulation of immunity to dietary antigens. In: Handbook of Mucosal Immunology (Ogra, P. L., Lamm, M. E., McGhee, J. R., Mestecky, J., Strober, W., and Bienenstock, J., Eds.), San Diego, CA: Academic Press, pp. 587–618.
- Mowat, A. M. 1987. The regulation of immune responses to dietary protein antigens. Immunol. Today 8:93–98.
- Notley, C. A., McCann, F. E., Inglis, J. J., and Williams, R. O. 2009. Anti-CD3 therapy expands the number of CD4+ and CD8+ Treg cells and induces sustained amelioration of collagen-induced arthritis. Arthritis Rheum. 62:171–178.
- Scientific Committee on Cosmetic Products and Non-food Products (SCCNFP). 2003. Evaluation and Opinion on Zinc Oxide. 24th Plenary Meeting, Brussels.
- Shahrara, S., Proudfoot, A. E., Park, C. C., Volin, M. V., Haines, G. K., Woods, J. M., Aikens, C. H., Handel, T. M., and Pope, R. M. 2008. Inhibition of monocyte chemoattractant protein-1 ameliorates rat adjuvant-induced arthritis. J. Immunol. 180:3447–3456.
- Takano, H., Yanagisawa, R., Ichinose, T., Sadakane, K., Yoshino, S., Yoshikawa, T. and Morita, M. 2002. Diesel exhaust particles enhance lung injury related to bacterial endotoxin through expression of pro-inflammatory cytokines, chemokines, and intercellular adhesion molecule-1. Am. J. Respir. Crit. Care Med. 165:1329–1335.
- Weaver, C. T., Hatton, R. D., Mangan, P. R., and Harrington, L. E. 2007. IL-17 family cytokines and the expanding diversity of effector T-cell lineages. Annu. Rev. Immunol. 25:821–852.
- Weiner, H. L., Friedman, A., Miller, A., Khoury, S. J., al-Sabbagh, A., Santos, L., Sayegh, M., Nussenblatt, R. B., Trentham, D. E., and Hafler, D. A. 1994. Oral tolerance: immunologic mechanisms and treatment of animal and human organ-specific autoimmune diseases by oral administration of auto-antigens. Annu. Rev. Immunol. 12:809–837.
- Whitacre, C. C., Gienapp, I. E., Orosz, C. G., and Bitar, D. M. 1991. Oral tolerance in experimental autoimmune encephalomyelitis. III. Evidence for clonal anergy. J. Immunol. 147:2155–2163.
- Yamaki, K., Uchida, H., Harada, Y., Yanagisawa, R., Takano, H., Hayashi, H., Mori, Y., and Yoshino, S. 2003. Effect of the non-steroidal anti-inflammatory drug indomethacin on TH1 and TH2 immune responses in mice. J. Pharm. Sci. 92:1723–1729.
- Yoshino, S., Ohsawa, M., and Sagai, M. 1998. Diesel exhaust particles block induction of oral tolerance in mice. J. Pharmacol. Exp. Ther. 287: 679–683.
- Yoshino, S., and Sagai, M. 1999. Induction of systemic TH1 and TH2 immune responses by oral administration of soluble antigen and diesel exhaust particles. Cell. Immunol. 192:72–78.