Abstract
The current study characterizes the mechanism by which the Amaranthus spinosus (Amaranthaceae) decreases mast cell-mediated anaphylactic reactions. Anaphylaxis is a typical hypersensitivity Type I reaction, sharing common mechanisms with asthma in its early and late phases. Mast cells are key as effector cells in hypersensitivity Type I reactions. A. spinosus has been traditionally used in the treatment of allergic bronchitis and asthma, but its role in mast cell-mediated anaphylactic reactions has not fully been investigated. This report investigated the potential effects of the ethyl acetate fraction of A. spinosus leaves (EAFAS) against a Compound 48/80 (potent secretagogue)-induced systemic anaphylactic shock paradigm in a mouse model. In addition, rat peritoneal mast cells (RPMC) were used in in vitro studies to investigate the effect of EAFAS on Compound 48/80-induced peritoneal mast cell degranulation and histamine release. When administration by the oral route—1 h before Compound 48/80 injection—EAFAS (at dose from 0.001–1 g/kg) completely inhibited the induced anaphylactic shock. EAFAS at concentrations ranging 0.25–1 mg/ml dose-dependently attenuated rates of mast cell degranulation and histamine release from RPMC that were evoked by Compound 48/80. The results of the present investigation indicated that EAFAS stabilizes the mast cell lipid bilayer membrane, thereby preventing the perturbation of membrane and the release of histamine. As a result of these anti-degranulating and anti-histaminic effects, it can be suggested that EAFAS may have a potential use in the prophylaxis and management of anaphylactic reactions.
Introduction
Amaranthus spinosus Linn. (Amaranthaceae) is commonly known as spiny amaranth or pig weed. It is an annual or perennial herb native to tropical America and distributed throughout India (Senthil Kumar et al., Citation2010). According to Ayurveda (Indian traditional system of medicine) the plant is useful in conditions of bronchial asthma; it is also used as a digestive aide, laxative, diuretic, stomachic, and anti-pyretic, to improve appetite, biliousness, blood diseases, burning sensation, leprosy, bronchitis, rat bite, piles, and leucorrhoea, while the boiled leaves and root are given to children as a laxative, emollient, and poultice for abscesses, boils, and burns (Kirtikar and Basu, Citation1996; Zeashan et al., Citation2009a). In Malaysia, the plant is used as an expectorant and to relieve breathing in acute bronchitis (Ashok Kumar et al., Citation2010). The tribal inhabitants like Bhils, Pardhis, Pawaras, Tadavis, and Wanjaris from Jalgaon district located on northern border of the state of Maharashtra, India uses decoction (15–20 ml) of whole plant in allergic bronchitis and asthma (CitationPatil et al., 2008). A. spinosus has several biologically active constituents like alkaloids, flavonoids, glycosides, phenolic acids, steroids, amino acids, terpe-noids, lipids, saponins, anthriquinone derivatives, volatile oils, organic acids, betalains, β-sitosterol, stigmasterol, linoleic acid, catechuic tannins, polyuronides, and carotenoids (Zeashan et al., Citation2009a).
It has been reported that this plant (and its natural by-products) possess immunomodulatory (Tatiya et al., Citation2007), anti-apoptotic (Lin et al., Citation2008), anti-diabetic, anti-hyperlipidemic, spermatogenic (Sangameswaran and Jayakar, Citation2008), anti-nociceptive, hepatoprotective, anti-oxidant (Zeashan et al., Citation2009b), anthelmintic, and anti-inflammatory activities (CitationBaral et al., 2011). So far, no systematic study has closely examined the anti-anaphylactic activity of A. spinosus. The present study sought to establish the scientific validity for such anti-anaphylactic activity.
Mast cells can be important effector cells in examples of innate or acquired immunity that contribute to host defense. The inappropriate or excessive activation of mast cells can contribute to the development of allergic or autoimmune diseases like asthma, anaphylaxis, allergic rhinitis, and urticaria. Mast cells can be found in almost every tissue but they are preferentially localized in organs that are in contact with the environment, that is, the skin, gut and airways. In response to the IgE-dependent and IgE-independent stimuli, mast cells elicit degranulation and cause release of spasmogenic, vasoactive, and pro-inflammatory mediators; these, in turn, can enhance airway smooth muscle contraction, vascular permeability, and inflammatory cell recruitment at sites of antigen challenge (Metz and Maurer, Citation2007; Nakae et al., Citation2007). These mediators can be granule-stored or preformed, e.g., histamine, proteoglycans, and neutral proteases, and certain cytokines, such as tumor necrosis factor-α (TNFα). Mediators can also be newly synthesized following activation, e.g., numerous cytokines, chemokines, lipid mediators, and growth and angiogenic factors that mediate various pro-inflammatory, anti-inflammatory, and immunoregulatory effects (Metz and Maurer, Citation2007).
Amongst these, histamine remains the most implicated and most potent vasoactive mediator and exerts many effects related to the immediate phase of allergic inflammation, including vasodilation, increased vascular permeability, tissue edema, bronchial and intestinal smooth muscle contraction, and increased mucus production (Kim et al., Citation2003). Mast cell degranulation can be elicited non-immunologically by the potent synthetic secretagogue Compound 48/80. This agent is a condensation product of N-methoxyphenylamine with formaldehyde and has been used as a direct convenient reagent to study mechanisms of anaphylaxis in situ (Shin et al., Citation1999). Modulation of the histamine and these cytokines secretion from mast cells can provide a useful therapeutic strategy for allergic anaphylactic reactions.
In light of the above information, the present study aimed to evaluate the effect of the ethyl acetate fraction of A. spinosus leaves (EAFAS) on Compound 48/80-induced systemic anaphylactic shock in mice, Compound 48/80-induced peritoneal mast cell degranulation, and inhibition of histamine release from rat peritoneal mast cells (RPMC).
Materials and methods
Reagents
Compound 48/80 and o-phthaldialdehyde (OPT) were purchased from Sigma-Aldrich (St. Louis, MO). Toluidine blue dye (analytical grade) was bought from Loba Chemicals (Mumbai, India).
Animals
Wistar rats (200–250 g) and Swiss Albino mice (25–30 g) of either sex were procured from the breeding centre of the R. C. Patel Institute of Pharmaceutical Education and Research, Shirpur (Maharashtra, India). Upon arrival, all animals were maintained in approved housing facilities of the institutional premises. Animals received a standard laboratory diet (certified Amrut brand rodent feed, Pranav Agro Industries, Pune, India) and water ad libitum; animals were maintained at ambient temperature (22 ± 2°C), at a relative humidity (55 ± 2°C), and with a 12 h light–dark cycle. All research was conducted as per a study protocol approved by the Institutional Animal Ethics Committee (IAEC) and as per Indian norms laid down by the Committee for the Purpose of Control and Supervision of Experiments on Animals (CPCSEA), New Delhi. During exposure regimens, animals were only given freshly prepared solutions of drugs or chemicals.
Procurement of plant material and fractionation procedures
Fresh Amaranthus spinosus plants (red [purple] variety) were collected from local areas of Bhalej in the Anand District of Gujarat, India. The plant material was authenticated by Dr M. M. Prajapati, Senior Botanist, The H.N.S.B Ltd Science College, Himatnagar, Gujarat, India; a voucher specimen (accession number A090) of the plant was deposited in the institutional herbarium. The leaves were separated from plant, dried, and coarsely powdered. The coarse powder (≈ 200 g) was extracted with methanol using a Soxhlet apparatus. The extract was filtered, concentrated, and dried. The dried extracted sample was then further fractionated successively using ethyl acetate. The resultant fraction was again concentrated and dried; the final ethyl acetate fraction of the A. spinosus leaves (EAFAS) material was then stored in an air-tight container in a cool place until utilized for phytochemical and pharmacological screening.
Preliminary phytochemical analysis
Preliminary phytochemical studies of the extract were performed for qualitative estimation of the presence of alkaloids, flavonoids, saponins, glycosides, phenols, steroids, tannins, and terpenoids. For these analyses, a small portion of the dry extract was used and analyses for the individual classes of components were performed in accordance with the methods of CitationHarbone (1998) and of Khandelwal (Citation2008)—and following the precise protocols outlined in Raaman (Citation2006) for performing each individual type of test (for some classes of agents, multiple types of tests for confirmatory purposes) indicated in .
Table 1. Preliminary qualitative phytochemical analyses of EAFAS.
HPLC analysis
Here, HPLC was used for the determination of quercetin in EAFAS, according to the method described by Zu et al. (Citation2006) with slight modifications. Chromatographic analysis was carried out by using C18 reverse-phase column (150 × 4.6 mm) packed with 5-µm diameter particles. The mobile phase used was methanol:acetonitrile:water (40:15:45 v/v/v) containing 0.1 ml acetic acid. Flow rate and injection volume were 0.7 ml/min and l0 µl, respectively. Quercetin was quantified at 340 nm. The chromatographic peak of the quercetin in EAFAS was confirmed by comparing the retention time (RT) with that of the reference standard quercetin.
Compound 48/80-induced systemic anaphylactic shock in mice
Compound 48/80-induced systemic anaphylactic shock was examined as previously described (Kim et al., Citation2005) with slight modification in the protocol. Mice (n = 10/group) were given an intraperitoneal injection (i.p.) of the mast cell degranulator Compound 48/80 (8 mg/kg body weight (BW)). This particular dose of Compound 48/80 was selected as previous investigators (Kim et al., Citation2006; CitationJudstrom et al., 2010) had demonstrated that it is a dose (intraperitoneal) that induces a systemic fatal allergic reaction within 1 h in mice.
EAFAS was dissolved in saline and administered orally at doses of 0.001–1 g/kg BW 1 h before the injection of Compound 48/80. In the time-dependent experiment, EAFAS (1 g/kg) was administered 5 and 10 min after Compound 48/80 (n = 10/group) injection. Mortality was then monitored for 1 h after induction of anaphylactic shock. In each of these studies, the doses of EAFAS were selected on the basis of preliminary studies that optimized the model.
Preparation of RPMC suspension
RPMC were isolated as previously described (Mahajan and Mehta, Citation2007). In brief, rats were anesthetized by ether and injected with 20 ml of Tyrode buffer B (150 mM NaCl, 0.1% glucose, 3 mM NaHCO3, 3.7 mM KCl, and 3.5 mM NaH2PO4 containing 0.1% gelatin) into the peritoneal cavity. After gentle massaging, the cavity was carefully opened and the fluid recovered by Pasteur pipette. The peritoneal cells present in the fluid were sedimented at 150 × g (10 min, room temperature) and resuspended in Tyrode buffer B. Mast cells were separated from the other major cell types present (i.e., macrophages and small lymphocytes) according to the method of Yurt et al. (Citation1977). Peritoneal cells suspended in 1 ml Tyrode buffer B were layered on 2 ml metrizamide (22.5% w/v, density = 1.120 g/ml) and centrifuged at 400 × g (15 min, room temperature). The cells remaining at the buffer-metrizamide interface were aspirated and discarded; the cells in the pellet were washed and resuspended in 1 ml Tyrode buffer A containing calcium for their use in further assays.
Peritoneal mast cell degranulation
In vitro mast cell degranulation by Compound 48/80 was utilized to assess the effects of EAFAS and ketotifen on peritoneal mast cell degranulation. Ketotifen is a second-generation H1 anti-histamine and a mast cell stabilizer. In these assays, RPMC were pre-incubated with EAFAS (0.25–1.00 mg/ml) and ketotifen (0.1 mg/ml) at a constant temperature of 37°C for 15 min and then incubated with 0.1 ml Compound 48/80 (10 µg/ml) for 10 min. The doses of EAFAS used here were selected to cover the range of doses successfully utilized by Rivera et al. (Citation2006) and Patel et al. (Citation2009) in their analyses of these particular mitigating effects by other natural product extracts (specifically, Mangifera indica L. (Vimang) stem bark and Taxus baccata Linn. (AET) leaves, respectively). The RPMC were then stained with toluidine blue and examined microscopically to estimate the percentage (%) of degranulated mast cells. A total of 100 cells/sample were analyzed for each treatment regimen.
Inhibition of histamine release
RPMC suspensions (2 × 105 cells/ml) were pre-incubated for 10 min at 37°C before the addition of Compound 48/80 for stabilization. The cells were pre-incubated with EAFAS (0.25–1 mg/ml) for 20 min and then incubated for 15 min with 0.1 ml Compound 48/80 (10 µg/ml). The reaction was stopped by cooling the tubes in ice. The cells were separated from the released histamine by centrifugation at 400 × g for 5 min at 4°C. Residual histamine in the cells was released by disrupting the cells with perchloric acid and centrifugation at 400 × g for 5 min at 4°C. The histamine content was measured by the o-phthaldialdehyde spectrofluorometric procedure of Shore et al. (Citation1959). The fluorescent intensity was measured at 440 nm (excitation of 360 nm) in a spectrofluorometer (FL600 Microplate Fluorescence Reader, Bio-Tek, Winooski, VT). The inhibition percentage of histamine release was then calculated using the following equation:
In the equation, A is the level of histamine release in the absence of EAFAS treatment and B the release when EAFAS was present.
Statistical analyses
All data are reported as the mean ± the standard error of the mean (SEM) in each of the different experiments. The Dunnett’s test was used to make a statistical comparison between the various treatment groups. Results with p-values < 0.05 were considered statistically significant. All statistical analyses were performed using Graph Pad software (Version 4; GraphPad Software Inc., La Jolla, CA).
Results
Preliminary phytochemical study
The preliminary qualitative phytochemical screening of the EAFAS revealed the presence of amino acids/proteins, flavonoids, tannins, and saponins (like steroids and triterpenoids) (). The material was found to not contain detectable amounts of alkaloids, carbohydrates, or glycosides. In all cases, verification of any given outcome was done using at least one other well-known confirmatory test per class of component (in some cases, up to four other tests, each outlined in great detail in Raaman (Citation2006)).
HPLC analysis
HPLC study revealed the presence of quercetin in EAFAS. The retention time (RT) for standard quercetin sample was found to be 4.8338 min (). The RT for quercitin in EAFAS was 4.8667 min, a value that was comparable with the reference standard ().
Effect of EAFAS on Compound 48/80-induced systemic anaphylactic shock
To investigate the effect of EAFAS on anaphylactic reactions, initially we used an in vivo model of systemic anaphylactic reaction using Compound 48/80. After the i.p. injection of Compound 48/80 (8 mg/kg BW) into mice, mortality over a period of 1 h was examined. As shown in , injection of Compound 48/80 into saline-treated mice induced fatal anaphylactic shock in 100% of the animals. When EAFAS was orally administered at levels ranging from 0.01–1.00 g/kg BW before the Compound 48/80 injection, mortality was dose-dependently reduced. Of note, EAFAS completely inhibited anaphylactic reactions at a 1 g/kg concentration. The mortality of mice injected i.p. with EAFAS (1 g/kg BW) 5 and 10 min after Compound 48/80 injection increased time-dependently ().
Table 2. Effect of EAFAS on Compound 48/80-induced systemic anaphylactic shock in mice.
Table 3. Time-dependent effect of EAFAS on Compound 48/80-induced systemic anaphylactic shock in mice.
Effect of EAFAS on Compound 48/80-induced peritoneal mast cell degranulation
The percent numbers of degranulated mast cells in Compound 48/80 (10 µg/ml)-treated cells were ≈ 77% as compared to among saline-only-treated cells. EAFAS induced significant dose-dependent protection against Compound 48/80-induced mast cell degranulation. Ketotifen (0.01 mg/ml) also markedly protected the mast cells against degranulation. The mast cell stabilization effect as shown by ketotifen was comparable with that from EAFAS ().
Figure 2. Effect of EAFAS on Compound 48/80-induced peritoneal mast cell degranulation in vitro. RPMC were pre-incubated with EAFAS (0.25–1 mg/ml) and ketotifen (0.1 mg/ml) at 37°C for 15 min and then treated with 0.1 ml of 10 µg Compound 48/80/ml for 10 min. Each bar represents mean (± SEM) of six independent experiments. *Value significantly different from saline-treated group (p < 0.01); **values significantly different from Compound 48/80 only-treated group (p < 0.01).
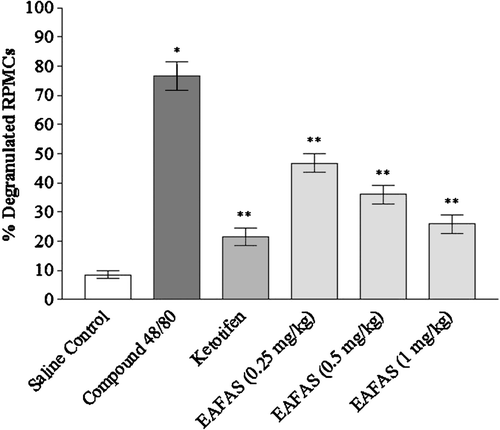
Effect of EAFAS on RPMC histamine release
To confirm that the effect of EAFAS on anaphylactic reaction was due to the inhibition of histamine release, we analyzed the histamine content from RPMC by using Compound 48/80 as a degranulator. EAFAS significantly and dose-dependently inhibited Compound 48/80 induced histamine release from RPMC at the concentrations of 0.25–1 mg/ml. The inhibitory effect of EAFAS on Compound 48/80-induced histamine release from RPMC is shown in .
Figure 3. Effect of EAFAS on Compound 48/80-induced histamine release from RPMC in vitro. Cells (2 × 105 RPMC/ml) were pre-incubated with EAFAS for 20 min at 37°C prior to incubation with 10 µg Compound 48/80/ml for a further 15 min. Each bar represents the mean (± SEM) of four independent experiments. **Value significantly different from saline-treated group (p < 0.01).
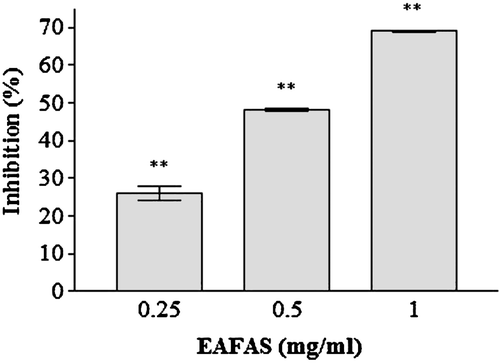
Discussion
Anaphylactic shock is an extreme and fatal allergic reaction induced by the sudden systemic release of inflammatory mediators from mast cells. A drug capable of preventing or restraining it is normally entitled with a very potent anti-allergic action. The anti-anaphylactic activity of natural substances has been reported by in vivo, in animals treated with the mast cell degranulator Compound 48/80 and in vitro models of mast cell degranulation (Cruz et al., Citation2008).
In the present investigation, we used the model of non-immune-mediated anaphylactic shock where mice die when challenged with the potent synthetic secretagogue Compound 48/80. In the in vivo studies here, treatment with EAFAS significantly suppressed systemic anaphylactic shock, probably by interfering with the mast cell/histamine system. EAFAS at the dose 1 g/kg prevented death in 100% of mice; this suggested a rapid and potent anti-allergic action compatible with possible anti-degranulating/anti-histaminic effects on mast cells.
Compound 48/80 induces signal transduction pathways, which leads to exocytosis, and degranulation of mast cells causing histamine release (Kim et al., Citation2005). The intracellular calcium (Ca2+) pathways are critical to the degranulation of mast cells. The activation of the Ca2+ signal by 1,4,5-triphosphate (IP3) elicits exocytosis in mast cells. In mast cells, the rise of the cytosolic free Ca2+ concentration is a biphasic process: emptying of the intracellular stores and influx through the plasma membrane. It is well established that intracellular Ca2+ mobilization is induced by the production of IP3. Binding of IP3 to its receptors, which are ligand-activated Ca2+-selective channels on the endoplasmic reticulum membrane, increases the probability of channel opening and Ca2+ flow into the cytoplasm. Compound 48/80, can cause degranulation of mast cells by releasing Ca2+ from the intracellular pool. Emptying of the Ca2+ stores produces a retrograde signal that activates Ca2+ influx across the plasma membrane. Thus, translocation of Ca2+, whether from outside or inside the cell, has been assumed to lead to an increase in free cytosol Ca2+ concentration as a signal to trigger degranulation (Beaven et al., Citation1984; Metcalfe et al., Citation1997).
RPMC can also generate intracellular reactive oxygen species (ROS) following incubation with Compound 48/80 by A-kinase inactivation via decreases in intracellular cAMP concentrations. Compound 48/80 has been shown to penetrate cellular membranes and directly activate G proteins. This activation involves insertion of an aromatic ring of Compound 48/80 into the membrane and interaction of a positively-charged domain of the molecule with the COOH-terminal portion of the α-sub-unit of G protein (Fukuishi et al., Citation1997; Brooks et al., Citation1999; Suzuki et al., Citation2003). In the in vitro studies performed here, EAFAS attenuated the rate of mast cell degranulation in a dose-related manner as observed microscopically by toluidine blue staining. It is proteoglycan that imparts the metachromatic staining characteristic of mast cells when exposed to certain basic dyes like toluidine blue. Proteoglycan has two functions, packaging histamine and basic proteins into secretory granules of mast cells and regulating the stability of tryptase protease. Neutral proteases, which account for the vast majority of the granule proteins, serve as markers of mast cells (Metcalfe et al., Citation1997; Choudhary, Citation2010).
As expected based on its known stabilizing effect on mast cell membranes (Dyson and Mackay, Citation1980), ketotifen possesed a mast cell stabilizing potential in the in vitro RPMC system here; of great note was the fact that the measured potential here was comparable to that from the EAFAS. The exact mechanisms by which EAFAS can influence the cell membrane remain to be elucidated. However, it is very tempting to speculate that EAFAS might have anti-degranulating potential as a result of an ability to stabilize the lipid bilayer membrane of cells, including RPMC, thereby preventing the perturbation of membranes as well as decreases in ROS and Ca2+ translocation (via inhibiting IP3 or GTP-induced Ca2+ release).
In the mast cell, the tissue hormones histamine and serotonin are bound to the sulfated heteroglycan heparin. The recognition of the allergen somehow causes a conformational change in the heparin, perhaps due to a change in ionic strength or to neutralization of the sulfate groups by Ca2+ ions entering the cell. The result is an abrupt release of a cloud of histamine and serotonin from the mast cells by exocytosis of granules (CitationHavsteen, 2002). As the in vitro studies here also showed that EAFAS inhibited the histamine burst from RPMC normally evoked by Compound 48/80, we attribute this outcome to be due, in great part, to the above-discussed anti-degranulating effect of this natural product.
Amaranthus spinosus is a rich source of different phytoconstituents with a variety of potential biological activities. Preliminary phytochemical screening of EAFAS proved the presence of flavonoids, steroids, triterpenoids, tannins, amino acids, and proteins, which may be responsible for anti-anaphylactic activity of the plant. HPLC analysis also revealed the presence of flavonoid quercetin in EAFAS. Flavonoids possess anti-oxidant activity (CitationHavsteen, 2002), and inhibit the secretion of histamine from mast cells. Flavonoid quercetin also inhibits the Ca2+ pump (CitationHavsteen, 2002). Steroids and triterpenes are also found to inhibit the histamine release from RPMC and thus are helpful in treating hypersensitivity type I reactions (Desai et al., Citation2009; Rios, Citation2010). The biological properties of these phytoconstituents support the results of the present pharmacological investigation.
Hence, a conclusion can be drawn that Amaranthus spinosus may have beneficial effects in the prevention or treatment of mast cell-mediated anaphylactic reactions due to its anti-degranulating and anti-histaminic potential. However, the effort to identify active components from EAFAS is ongoing in our laboratory, as the present findings of the in vivo and in vitro studies have given a hopeful picture for the future.
Acknowledgments
The authors are thankful to R. C. Patel Institute of Pharmaceutical Education and Research, Shirpur for providing facility for research work.
Declaration of interest
The authors report no conflict of interest. The authors alone are responsible for the content and writing of the paper.
References
- Ashok Kumar B. S., Lakshman K., Jayaveera K. N., Khan S., Manoj B., and Narayan Swamy V. B. 2010. Evaluation of the anti-oxidant activity of Amaranthus spinosus Linn. By non-enzymatic haemoglycosylation. Sains Malaysiana 39:413–415.
- Baral M., Chakraborty S., and Chakraborty P. 2010. Evaluation of anthelmintic and anti-inflammatory activity of Amaranthus spinosus Linn. Int. J. Curr. Pharm. Res. 2:44–47.
- Beaven M. A., Rogers J., Moore J. P., Hesketh T. R., Smith G. A., and Metcalfe J. C. 1984. Mechanism of the calcium signal and correlation with histamine release in 2H3 cells. J. Biol. Chem. 259:7129–7136.
- Brooks A. C., Whelan C. J., and Purcell W. M. 1999. Reactive oxygen species generation and histamine release by activated mast cells: Modulation by nitric oxide synthase inhibition. Br. J. Pharmacol. 128:585–590.
- Choudhary G. P. 2010. Mast cell stabilizing activity of Ocimum sanctum leaves. Int. J. Pharm. Bio. Sci. 1:1–11.
- Cruz E. A., Da Silva S. A. G., Muzitano M. F., Silva P. M., Costa S. S., and Rossi-Bergmann B. 2008. Immunomodulatory pretreatment with Kalanchoe pinnata extract and its quercitin flavonoid effectively protects mice against fatal anaphylactic shock. Int. Immunopharmacol. 8:1616–1621.
- Desai S. D., Desai D. G., and Kaur H. 2009. Saponins and their biological activities. Pharma Times 41:13–16.
- Dyson A. J., and Mackay A. D. 1980. Ketotifen in adult asthma. Br. Med. J. 280:360–361.
- Fukuishi N., Sakaguchi M., Matsuura S., Nakagawa C., Akagi R., and Akagi M. 1997. The mechanisms of Compound 48/80-induced superoxide generation mediated by A-kinase in rat peritoneal mast cells. Biochem. Mol. Med. 61:107–113.
- Harborne J. B. (Ed.) 1998. Phytochemical Methods - A Guide to ModernTechniques of Plant Analysis. London: Chapman and Hall.
- Havsteen, B. H. 2002. The biochemistry and medical significance of the flavonoids. Pharmacology and Therapeutics 96:67–202.
- Judstrom I., Jukkola H., Metso J., Jauhiainen M., Kovanen P. T., and Lee-Rueckert M. 2010. Mast cell-dependent proteolytic modification of HDL particles during anaphylactic shock in the mouse reduces their ability to induce cholesterol efflux from macrophage foam cells ex vivo. Atherosclerosis 208:148–154.
- Khandelwal K. R. (Ed.) 2008. Preliminary phytochemical screening. In: Practical Pharmacognosy Techniques and Experiments. 9th Edition. Pune, India: Nirali Prakashan, pp. 149–157.
- Kim M. S., Na H. J., Han S. W., Jin J. S., Song U. Y., Lee E. J., Song B. K., Hong S. H., and Kim H. M. 2003. Forsythia fructus inhibits the mast-cell-mediated allergic inflam-matory reactions. Inflammation 27:129–135.
- Kim S. H., Choi C. H., Kim S. Y., Eun J. S., and Shin T. Y. 2005. Anti-allergic effects of Artemisia iwayomogi on mast cell-mediated allergy model. Exp. Biol. Med. 230:82–88.
- Kim S. H., Kim S. H., Kim S. H., Moon J. Y., Park W. H., Kim C. H., and Shin T. Y. 2006. Action of Dracocephalum argunense on mast cell-mediated allergy model. Biol. Pharm. Bull. 29:494–498.
- Kirtikar K. R., and Basu B. D. (Eds.) 1996. Indian Medicinal Plants, Vol. 3. Dehradun, India: International Book Distributors, pp. 2057–2059.
- Lin J. Y., Li C. Y., and Lin B. F. 2008. Amaranthus spinosus L. inhibits spontaneous and dexamethasone-induced apoptosis in murine primary splenocytes. J. Food Drug Anal. 16:52–61.
- Mahajan S. G., and Mehta A. A. 2007. Inhibitory action of ethanolic extract of seeds of Moringa oleifera Lam. on systemic and local anaphylaxis. J. Immunotoxicol. 4:287–294.
- Metcalfe D. D., Baram D., and Mekori Y. A. 1997. Mast cells. Physiol. Rev. 77:1033–1079.
- Metz M., and Maurer M. 2007. Mast cells—key effector cells in immune responses. Trends Immunol. 28:234–241.
- Nakae S., Lien H., Yu M., Monteforte R., Likura M., Suto H., and Galli S. J. 2007. Mast cell-derived TNF contributes to airway hyperreactivity, inflammation, and TH2 cytokine production in an asthma model in mice. J. Allergy Clin. Immunol. 120:48–55.
- Patel P. K., Patel K. V., and Gandhi T. R. 2009. Evaluation of effect of Taxus baccata leaves extract on bronchoconstriction and bronchial hyperreactivity in experimental animals. Global J. Pharmacol. 3:141–148.
- Patil G. G., Mali P. Y., and Bhadane V. V. 2008. Folk remedies used against respiratory disorders in Jalgaon district, Maharashtra. Nat. Prod. Radiance 7:354–358.
- Raaman N. (Ed.). 2006. Phytochemical Techniques. New Delhi: New India Publishing.
- Rios J. L. 2010. Effects of triterpenes on the immune system. J. Ethnopharmacol. 128:1–14.
- Rivera D. G., Balmaseda I. H., Leon A. A., Hernandez B. C., Montiel L. M., Garrido G. G., Cuzzocrea S., and Hernandez R. D. 2006. Anti-allergic properties of Mangifera indica L. extract (Vimang) and contribution of its glucosylxanthone mangiferin. J. Pharm. Pharmacol. 58:385–392.
- Sangameswaran B., and Jayakar B. 2008. Anti-diabetic, anti-hyperlipidemic and spermatogenic effects of Amaranthus spinosus Linn. on streptozotocin-induced diabetic rats. J. Nat. Med. 62:79–82.
- Senthil Kumar M. K., Rajesh V., Suresh Kumar S., and Perumal P. 2010. Evaluation of analgesic activity of Amaranthus spinosus Linn. leaves in mice. J. Pharm. Res. 3:3088–3089.
- Shin T. Y., Park J. H., and Kim H. M. 1999. Effect of Cryptotympana atrata extract on Compound 48/80-induced anaphylactic reactions. J. Ethnopharmacol. 66:319–325.
- Shore P. A., Burkhalter A., and Cohn V. H. 1959. A method for fluorometric assay of histamine in tissues. J. Pharmacol. Exp. Ther. 127:182–186.
- Suzuki Y. M., Yoshimaru T., Matsui T., Inoue T., Niide, O., Nunomura S., and Ra, C. 2003. Fcϵ-RI signaling of mast cells activates intracellular production of hydrogen peroxide: Role in the regulation of calcium signals. J. Immunol. 171:6119–6127.
- Tatiya A. U., Surana S. J., Khope S. D., Gokhale S. B., and Sutar M. P. 2007. Phytochemical investigation and immunomodulator activity of Amaranthus spinosus linn. Indian J. Pharm. Educ. Res. 41:337–341.
- Yurt R. W., Leid R. W., and Austen K. F. 1977. Native heparin from rat peritoneal mast cells. J. Biol. Chem. 252:518–521.
- Zeashan H., Amresh G., Singh S., and Rao C. V. 2009a. Anti-diarrheal and anti-ulcer activity of Amaranthus spinosus in experimental animals. Pharm. Biol. 47:932–939.
- Zeashan H., Amresh G., Singh S., and Rao C. V. 2009b. Hepatoprotective and anti-oxidant activity of Amaranthus spinosus against CCl4-induced toxicity. J. Ethnopharmacol. 125:364–366.
- Zu Y., Li C., Fu Y., and Zhao C. 2006. Simultaneous determination of catechin, rutin, quercetin, kaempferol, and isorhamnetin in extract of sea buckthorn (Hippophae rhamnoides L.) leaves by RP-HPLC with DAD. J. Pharm. Biomed. Anal. 41:714–719.