Abstract
To develop a rat T-cell-dependent antibody response (TDAR) model evaluating both primary and secondary antibody responses, keyhole limpet hemocyanin (KLH) was used to immunize rats twice during a 14-day course of study, a pattern closely linked to that of a short-term general toxicity study. Female rats of four representative strains (e.g., Sprague-Dawley, Wistar, Fischer, and Lewis) were immunized twice with intravenous administrations of KLH (300 µg/rat) on Days 5 and 9 during a 14-day treatment regimen with cyclophosphamide (CPA) at 1, 3, or 6 mg/kg/day. The primary and secondary immunizations of KLH markedly elevated serum anti-KLH IgM and IgG levels in all strains on Days 9 and 15. Remarkable higher levels of anti-KLH IgG (≈ 1000 µg/ml) were noted in all strains, which were more than 4-times compared with those of anti-KLH IgM levels at Day 9, indicating that predominant IgG reactions were induced by the dual immunizations. A large inter-individual variability in KLH-specific IgM and IgG production was observed in all strains. However, levels of the KLH-specific antibodies were considered sufficient for the evaluation, even in Sprague-Dawley and Wistar rats reported as strains with a wide range of variability since immunosuppression of CPA on responses in both anti-KLH IgM and IgG were observed in all strains to the same extent. In addition, the sensitivity of the KLH-ELISA assay system detecting the immunosuppressive effects of CPA was comparable to other assay systems with PFC assay or ELISA using SRBC. The results here demonstrated that these experimental designs could provide valuable information about the influence on both the primary and secondary humoral immune responses in rats when exposed to potential immunomodulatory drugs. Furthermore, the design of the presented TDAR study would support comprehensive evaluation together with the outcome of the conventional general toxicity study.
Introduction
A T-cell-dependent antibody response (TDAR) assay is recommended as a first choice for an immune function test to evaluate the potential immunotoxicity on new chemicals (ICH S8 Guideline, Citation2006). As the traditional models of TDAR assays were developed in the last era, the primary immune response to sheep red blood cells (SRBC) measured by a splenic plaque-forming cell (PFC) or an enzyme-linked immunosorbent assay (ELISA) had been widely used (Luster et al., Citation1992; Institoris et al., Citation2002). However, these assay systems with SRBC have inherent drawbacks, including complicated procedures of PFC assay and several variations come primarily from the lots of SRBC employed and the different procedures of SRBC preparation for plate coating among laboratories.
Recently, TDAR assay using a soluble immunogenic protein, keyhole limpet hemocyanin (KLH) instead of SRBC, has become an experimental trend (Smith et al., Citation2003; Gore et al., Citation2004; Ulrich et al., Citation2004; Finco-Kent and Kawabata, Citation2005; Piccotti et al., Citation2005), since KLH is easily applied to ELISA and a lyophilized KLH which can be preserved to remain stable for the long-term is commercially available (Stenzel-Poore and Rittenberg, Citation1989; Friedman et al., Citation2002). The primary antibody response to KLH in rats measured by an ELISA has been reported to be a reliable and readily standardized method alternative to the conventional SRBC PFC assay (Gore et al., Citation2004). Gore et al. (Citation2004) have recommended that evaluation of the IgG response, in addition to the IgM response, provides more comprehensive assessment of the primary response and increases the likelihood of identifying a ‘true’ potential. They have also suggested that evaluation of the secondary antibody response could be included in the TDAR assay to identify the potential immunotoxicity of new medicinal candidates, although the secondary antibody response, consisting predominantly of IgG, would require additional immunizations with the antigen or a longer dosing period (Gore et al., Citation2004). Despite the important nature of the secondary response, no standardized protocol for TDAR assay incorporating both primary and secondary responses has been established to date.
To develop a practical study design incorporating both the primary and secondary responses in TDAR assay in rats, we evaluated both serum KLH-specific IgM and IgG levels by ELISA following primary and secondary immunizations with KLH (300 µg/rat, IV) during a 14-day study period. Although the ICH S8 Guideline stated that the 28-day study period was generally accepted to assess drug-induced immunotoxicity in rodents, the study period was selected to be closely-linked to the period routinely used in short-term 14-day general toxicity studies used to support short-term clinical trials for human pharmaceutical candidate drugs (which also last up to 14-days, as per the ICH M3(R2) Guideline [2009]). In the present study, four representative strains of rats (Sprague-Dawley (SD), Wistar, Fischer, and Lewis rats) were employed since the differences in strains and inter-individuals have been pointed out as practical issues in TDAR assays (Gore, Citation2006). Additionally, the utility of this model to detect immunosuppressive effects was confirmed in the above four strains with the reference immunosuppressive drug, cyclophosphamide (CPA) by a 14-day exposure. Further, the results obtained from the experiment with CPA were compared to those of the primary response obtained by PFC assay or SRBC-ELISA in order to validate the sensitivity of this TDAR assay.
Materials and methods
Animals
Female SD, Wistar, Fischer, and Lewis rats (6-weeks-old) were obtained from Charles River Laboratories Japan, Inc. (Kanagawa, Japan). Rats were housed individually in stainless steel wire mesh bracket cages for at least 5 days for acclimation to the laboratory environment (temperature of 23 [±3]°C; relative humidity of 55 [±15]%; lighting cycle of 12 h/day). Commercial rodent diet (CRF-1, Oriental Yeast Co., Ltd., Tokyo, Japan) and tap water were available ad libitum. All animal procedures were performed in accordance with our institutional guide for the care and use of laboratory animals.
Chemicals and antibodies
KLH, bovine serum albumin (BSA) and sodium dodecyl sulfate (SDS) were obtained from Wako Pure Chemical Industries, Ltd. (Osaka, Japan). Cyclophosphamide (CPA) and Sigma Fast™ o-phenylenediamine (OPD) dihydrochloride tablet sets were purchased from Sigma (St. Louis, MO). Horseradish peroxidase (HRP)-conjugated anti-rat IgM and anti-rat IgG, as well as guinea pig complement were supplied as lyophilized products by Rockland Immunochemicals, Inc. (Gilbertsville, PA). Anti-KLH rat IgM (purified rat IgM [κ isotype] control) and anti-KLH rat IgG (purified rat IgG1 [κ isotype] control) antibodies were purchased from BioLegend (San Diego, CA). Sheep whole blood (in sterile Alsevers solution) was obtained from Nippon Bio-Supp. Center (Tokyo, Japan). RPMI medium was purchased from Nissui Pharmaceutical Co., Ltd. (Tokyo). Fetal bovine serum (FBS) was obtained from Invitrogen (Carlsbad, CA). The BCA protein assay reagent kit was purchased from Thermo Scientific Pierce (Rockford, IL).
Treatment protocol
CPA dissolved in distilled water was orally administered to rats on 14 consecutive days at 5 ml/kg body weight (BW) and ). The dose levels were set at 0 (vehicle control), 1, 3, or 6 mg CPA/kg/day, based on a preliminary study (data not shown). An intravenous (IV) dose of KLH-saline solution delivering 300 µg KLH antigen/rat was selected based on the earlier studies of Gore et al. (Citation2004) and White et al. (Citation2007). The results of the preliminary study which included a time-course response revealed that IV dosing of 300 µg KLH/rat produced sufficient levels of anti-KLH specific antibody that were, in turn, considered to be utilizable for detecting the immunosuppressive potential of the test articles, even in the absence of any other overt toxicity findings. In the study here, the rats were immunized twice, i.e., on Days 5 and 9 of the CPA dosing regimen. Blood was collected from the jugular veins of individual rats on Days 1, 9, and 15 in order to perform a TDAR assay ). On Day 9, the blood was collected prior to the secondary KLH immunization to avoid affecting the primary TDAR outcome.
Figure 1. Study designs of TDAR assays. Cyclophosphamide (CPA) was dosed orally to female rats for 14 consecutive days (1, 3, and 6 mg/kg). (A) Primary and secondary KLH-TDAR assay: Animals were immunized twice by IV injection of keyhole limpet hemocyanin (KLH, 300 μg/rat) on Days 5 and 9 during the 14-day CPA treatment. ELISA was used to determine serum anti-KLH IgM and anti-KLH IgG levels on Days 1, 9, and 15. (B) Traditional SRBC-PFC or SRBC-TDAR assay: Animals were immunized with IV injection of SRBC (2 × 108 cells/body) on Day 11 during 14-day CPA treatment. Serum anti-SRBC IgM levels or anti-SRBC IgM producing cell counts were determined on Day 15 by ELISA or PFC assay, respectively.
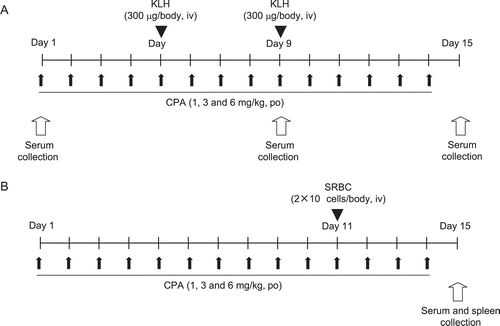
For the SRBC-PFC assay or SRBC-TDAR assay, rats were sensitized once with an IV SRBC injection (2 × 108 cells/animal) just after the dosing of CPA on Day 11. On Day 15, the rats were sacrificed by exsanguination after blood collection from the abdominal aorta, under anesthesia. The spleen was then removed for use in the PFC assay ). Serum samples were prepared by centrifugation and then stored at −80°C until analysis.
Preparation of KLH and SRBC antigens
KLH was dissolved in physiological saline at a concentration of 1.5 mg/ml. The solution was filtered using a membrane filter (0.45 µm, Sartorius Stedim Biotech GmbH, Goettingen, Germany) prior to injection. Sheep whole blood (in Alsever’s sterile solution) was washed three times with saline and the SRBC were then re-suspended in saline at 1 × 106 cells/µl.
ELISAs with anti-KLH antibodies
KLH (10 µg/ml) dissolved in coating (50 mM NaHCO3) buffer was applied to a 96-well microplate (Nalge Nunc Intl., Roskilde, Denmark) and incubated overnight at 4°C. After blocking with 5% BSA in 0.05% Tween-20 (v/v) in PBS (TPBS) at 37°C for 2 h, anti-KLH rat IgM or anti-KLH rat IgG in duplicate was added to the plate for standard curves (4-parameter logistic model). The assay range for the IgM- and IgG-specific ELISAs was 1.56–100.00 ng/ml and 7.80–500.00 ng/ml, respectively. To evaluate each serum sample within the assay range, each serum sample was diluted (≥ 1:1000) with the diluent (1% BSA in TPBS). Each diluted serum sample in duplicate was added to the plate and was equilibrated at 37°C for 2 h. After gentle washing to remove non-adherent antibody/other serum factors, the plates were then treated with anti-rat IgM or IgG detection antibody conjugated with HRP at pre-determined optimal dilutions in the diluent and incubated at 37°C for 2 h. The optical density (OD) after stopping the OPD color reaction with 50 µl 1 M sulfuric acid was measured at 492 nm in a SpectraMax Pro micro-plate reader (Molecular Devices LLC, Sunnyvale, CA). Standard curves and anti-KLH Ig levels for each serum sample were calculated using SoftMax Pro software (Molecular Devices LLC).
PFC assay
A PFC assay was conducted according to the methods in Cunningham (Citation1965) and Cunningham and Szenberg (Citation1968). Briefly, the excised spleen was put in a dish with 10% FBS-RPMI medium, and a portion of the spleen was gently minced with tweezers. A single-cell suspension was obtained by passage through a cell strainer (70 µm, BD Falcon™). After two washes, the cells were re-suspended in 10% FBS-RPMI medium (cell viability was > 90% in trypan blue dye exclusion tests). Subsequently, 400 µl of the suspension, 50 µl of 40% (v/v) SRBC, and 50 µl of guinea pig complement were mixed in a test tube, and the mixture was put in a Cunningham chamber. The sealed chamber was incubated at 37°C for 1.5 h before the number of PFC was counted microscopically; results were then calculated and expressed in terms of specific activity (PFC/106 splenocytes). In addition, total spleen activity (PFC/whole spleen) was calculated based upon the whole/partial spleen weight ratio. In this paper, only specific activity (PFC/106 splenocytes) is reported because the outcomes were comparable to those for total spleen activity.
Extraction of SRBC membrane proteins
Erythrocyte ghosts were prepared according to the reported method (Dodge et al., Citation1963; Hanahan and Ekholm, Citation1974), with some modification. After centrifugation of sheep whole blood (in Alsever’s sterile solution), the SRBC pellets were washed with saline and then re-suspended in 1 mM Na2-EDTA/5 mM Tris-HCl (pH 7.0). After washing, the pellet was re-suspended with 3 M KCl, the solution sonicated for 1 h at 4°C, and then stored for 2 days at 4°C. After centrifugation, the pellet was re-suspended with 0.1% SDS (w/v) and then stored at 4°C for 2 days. The solution was centrifuged and the supernatant dialyzed against PBS at 4°C overnight. The collected SRBC membrane extract solution was diluted with PBS and protein concentration measured with the BCA Protein Assay Reagent Kit. The protein concentration of the solution was adjusted to 1 mg/ml with PBS and the diluted protein samples stored at −20°C until use.
Anti-SRBC antibody ELISA
Serum anti-SRBC IgM was measured by ELISA using the method of Temple et al. (Citation1993), with some modification. Extracted SRBC membranes (20 µg/ml) suspended in coating buffer (0.1 M NaHCO3) were applied to a 96-well microplate and incubated at 4°C overnight. After blocking with 1% skimmed milk (in water) at room temperature (RT) for 2 h, plates were incubated at RT for 2 h with serial dilutions (1:4 to 1:65,536) of the serum samples. The plates were then incubated with an anti-rat IgM detection antibody conjugated with HRP at RT for 2 h. After gentle washing with buffer, each well received 100 µl OPD substrate and the plate was incubated for 8 min at RT. The color reaction was then stopped by addition of 50 µl of 1 M sulfuric acid to each well and the absorbance in each was measured at 492 nm with the micro-plate reader. The 4-fold dilution of serum vs the absorbance was used to fit a 4-parameter curve. A dilution factor (which indicates dilution of serum needed to produce an OD = 1.0) was extrapolated from the equation resulting from the 4-parameter curve using SOFTmaxPRO software (Molecular Devices LLC).
Statistical analysis
The mean and standard deviations for each parameter were calculated at each timepoint. They were statistically compared between a vehicle control and treated groups. First, the parameter was analyzed by a Bartlett’s test (significance level = 1%) to evaluate homogeneity of variance. The parameter was further analyzed by a Dunnett’s test when the variance was homogenous and by a Dunnett’s rank test when not. The analyses were each performed using a 5% significance level.
Results
Primary and secondary TDAR to KLH in four rat strains
During the course of the study, the primary injection of KLH (300 μg/rat) on Day 5 produced detectable serum amounts of anti-KLH IgM antibodies on Day 9 in the control animals of all strains ). However, serum amounts of anti-KLH IgG antibodies were less than those of the IgM type. The second injection of KLH at the same dose on Day 9 led to further increases in anti-KLH IgM antibody production on Day 15 in SD and Wistar rats (1.8- and 2.2-times, respectively, compared to Day 9 levels), but slight decreases in the Fischer and Lewis hosts (0.6-times in both strains). In contrast, anti-KLH IgG productions markedly increased in all strains (ranging from 17–53-times).
Figure 2. Primary and secondary antibody response to KLH in rats. Female rats were immunized twice with IV injection of KLH (300 μg/rat) on Days 5 and 9 during the course of the study. Left panel: Serum anti-KLH IgM levels, Right panel: Serum anti-KLH IgG levels, (A) and (E): SD rats; (B) and (F): Wistar rats; (C) and (G): Fischer rats; (D) and (H): Lewis rats. Values shown represent mean of eight control animals in each strain.
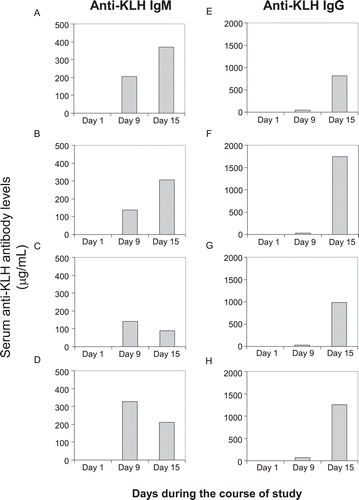
A large inter-individual variability in anti-KLH antibody responses was observed in the control animals of all strains ). The coefficients of variance (CV) in serum anti-KLH IgM levels on Day 9 were 52.6, 56.7, 21.9, and 16.6% in SD, Wistar, Fischer, and Lewis rats, respectively. The CV in serum anti-KLH IgG levels on Day 9 were 54.3, 51.4, 17.7, and 22.5%, respectively. The CV in serum anti-KLH IgM levels on Day 15 were 37.2, 62.0, 29.4, and 17.9%, respectively. Lastly, the CVs in serum anti-KLH IgG levels on Day 15 were 56.5, 49.5, 36.1, and 48.6%, respectively. A tendency for a wide range of CVs in the primary responses was noted in SD and Wistar rats compared to Fischer and Lewis rats with respect to both anti-KLH IgM and IgG productions on Day 9.
Figure 3. Inter-individual variation of anti-KLH antibody responses in rats. Female rats were immunized twice with IV injection of KLH (300 μg/rat) on Days 5 and 9 during the course of the study. (A) Serum anti-KLH IgM levels on Day 9. (B) Serum anti-KLH IgG levels on Day 9. (C) Serum anti-KLH IgM levels on Day 15. (D) Serum anti-KLH IgG levels on Day 15. Each circle and bar represent the individual value and mean of eight control animals in each strain. Each value in parentheses means the coefficient of variance.
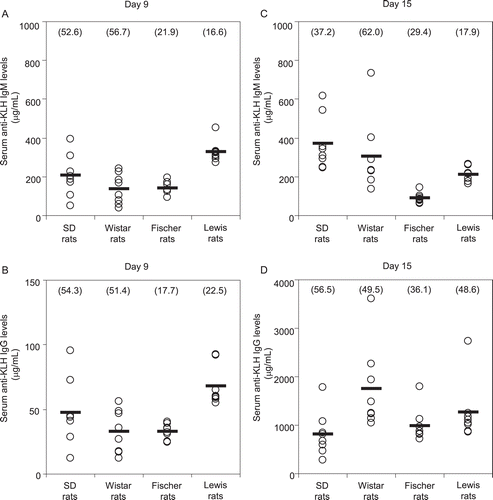
Immunosuppressive effects on primary and secondary TDAR to KLH in four rat strains during 14-day CPA exposure
On Day 9 in all strains, suppressed serum anti-KLH IgM antibody production was noted with the 6 mg/kg/day dose; serum anti-KLH IgG antibodies were reduced with both the 3 and 6 mg/kg/day doses ). On Day 15 in all strains, CPA at 3 and 6 mg/kg/day suppressed the anti-KLH IgM antibody production; serum anti-KLH IgG antibodies formation was maximally suppressed with 6 mg CPA/kg/day. Although the data shown in seem to suggest to us that the suppressive effects on CPA occur in a dose-related manner, this outcome was not apparent across the strains (no consistent dose–trend relationship in Wistar rats) or as a function of time (see loss of apparent dose-relatedness for IgG from Day 9 to Day 15).
Figure 4. Effect of CPA treatment on anti-KLH antibody responses in rats. CPA (1, 3, and 6 mg/kg) was dosed orally to female rats for 14 consecutive days. Animals were immunized twice with IV injection of KLH (300 μg/rat) on Days 5 and 9 during the CPA treatment. (A) Serum anti-KLH IgM levels on Day 9. (B) Serum anti-KLH IgG levels on Day 9. (C) Serum anti-KLH IgM levels on Day 15. (D) Serum anti-KLH IgG levels on Day 15. Each column and bar represents the mean (± SD) of eight animals. # p < 0.05, ## p < 0.01: Significantly different from respective control group in each strain (Dunnett test), * p < 0.05: Significantly different from respective control group in each strain (Dunnett rank test).
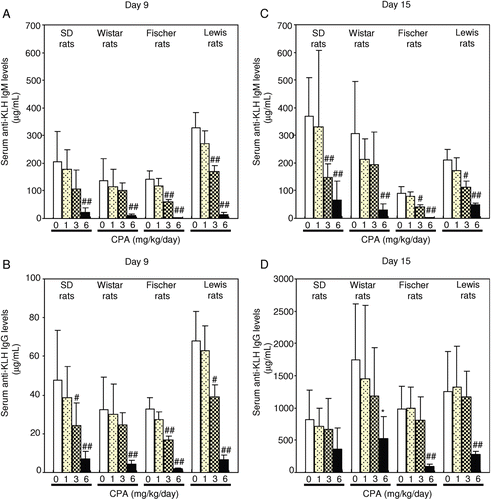
Immunosuppressive effects on primary antibody response to SRBC in four rat strains during 14-day CPA exposure
A single IV injection of SRBC (2 × 108 cells/body) on Day 11 resulted in the presence of anti-SRBC IgM-producing cells in the spleen and serum anti-SRBC IgM antibody on Day 15 in the control animals of all strains ). CPA treatments of 3 and 6 mg/kg/day significantly suppressed PFC production and serum anti-SRBC IgM antibody levels at the same time. In this case, the data more clearly suggested there was a dose-related effect for the CPA on these parameters.
Figure 5. Effect of CPA treatment on plaque-forming cells (PFC) assay and anti-SRBC IgM response by SRBC immunization in rats. CPA (1, 3, and 6 mg/kg) was dosed orally to female rats for 14 consecutive days. Animals were immunized with single intravenous injection of SRBC (2 × 108 cells/rat) on Day 11 during the CPA treatment. (A) PFC on Day 15. (B) Serum anti-SRBC IgM levels on Day 15. Each column and bar represents the mean (± SD) of four-to-eight animals. ** p < 0.01, * p < 0.05: Significantly different from respective control group in each strain (Dunnett rank test).
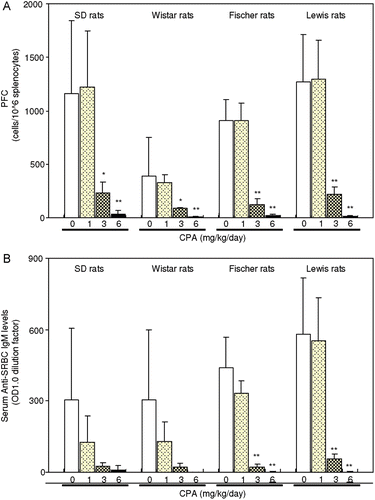
Discussion
In recent years, the utility of the primary response to KLH (i.e., formation of anti-KLH IgM antibody) has been demonstrated to serve as a toxicological endpoint to detect any immunosuppressive potential of new drug entities. Additional endpoints such as the secondary response (consisting predominantly of IgG) still remain to be addressed. To develop a practical study design incorporating both the primary and secondary responses in a TDAR assay, we characterized anti-KLH IgM and IgG antibody responses following primary and secondary immunizations with KLH (300 µg/rat) during a 14-day course of study in four rat strains. Under these conditions, the primary injection of KLH markedly elevated serum anti-KLH IgM levels 4 days after the injection (in a range from 137–327 µg/ml in all strains); however, the secondary injection caused only slight changes in the anti-KLH IgM responses (≈ 2-times in SD and Wistar rats or ≈ 0.6-times in Fischer and Lewis rats, compared with counterpart primary responses). In contrast, remarkable increases in anti-KLH IgG levels (17- to 53-times compared with primary response) were observed 6 days after the secondary KLH injection in all rat strains. In addition, all strains yielded serum levels of ≈ 1000 µg/ml of anti-KLH IgG antibodies; these levels were > 4-times those of the anti-KLH IgM antibodies, clearly indicating that IgG predominant reactions were induced by the secondary immunization.
Gore et al. (Citation2004) demonstrated that the production of anti-KLH IgM antibody in SD rats reached the maximum level (i.e., 388 µg/ml) 5 days after a single intravenous immunization of KLH (300 µg/kg), whereas the production of anti-KLH IgG production was slight (~ 10 µg/ml). In that study, anti-KLH IgG antibody production reached the maximum level (230 µg/ml) 14 days after the immunization, despite the level still being lower than that for anti-KLH IgM. Consistent with that report, the present study indicated that a single immunization with KLH induced an IgM-predominant response, with the IgG response being induced to a lesser extent. From an immunology point of view, the main antibody involved in a primary response is IgM, which eliminates pathogens in early stages of humoral immunity without sufficient IgG. Generally, IgG antibodies, which are involved predominantly in the secondary immune response, are known to play important roles in complement-mediated activation, opsonization of antigens for phagocytosis, and antibody-dependent cell-mediated cytotoxicity (ADCC). A presence of specific IgG corresponds to maturation of the antibody responses that are regulated by multiple mechanisms such as Ig class switch and effectors of memory cells. Therefore, incorporating both the primary and secondary responses in a TDAR assay would be crucial to comprehensively evaluate the immunotoxic effect of a new drug.
IgG analysis was demonstrated to show greater sensitivity for the detection of immuno-suppression than IgM analysis in several TDAR studies. An immunosuppressant, FK506, inhibited the production of anti-KLH IgG in KLH-immunized rats with no significant change in the IgM response (Ulrich et al., Citation2004). The immunosuppressant cyclosporine also reduced antigen-specific IgG responses more than IgM responses in rats after a single immunization with KLH (Gore et al., Citation2004) or dual immunizations with nitrophenyl-chicken γ-globulin (Smith et al., Citation2003). To address this point, we examined the immunosuppressive effects of CPA in the present study. In our study, CPA at ≥ 3 mg/kg suppressed anti-KLH IgM and IgG production in all four rat strains. In three of the test strains, these outcomes appeared to occur in a dose-related manner. Although the mechanisms causing these differences between IgM and IgG responses in the immunosuppressive effects remain unclear, the sensitivity in the IgG suppression of the drug may be associated with its pharmacological properties and dosing duration. FK506 and cyclosporine predominantly suppress T-cell activity, resulting in decreasing cytokine production related to B-cell differentiation and IgG class switch (Henry, Citation1999). This specificity of these drugs may provide profiles different from CPA, which simply affects all immune cells as a cytotoxin.
The differences among rat strains have been claimed to be one of the confounders in TDAR assays. In fact, two immunosuppressive compounds, e.g., 2-methoxyethanol and 2-methoxy-acetic acid, have shown different susceptibility in the PFC assay among rat strains (Smialowicz et al., Citation1994). Similar strain differences in anti-KLH IgM responses have been reported to exist in a KLH-ELISA-based TDAR assay using mice and rats (White et al., Citation2007). In addition, the inherent inter-individual variability of the antibody responses—especially in the out-bred strains—has been assumed to be affected by variations in analytical methods, i.e., ELISA (Gore, Citation2006). Under our sensitizing conditions of KLH to rats, steady responses of IgM and IgG to KLH were observed in all strains without non-responders, although the primary responses of IgM and IgG in the outbred strains, SD rats and Wister rats, exhibited relatively larger inter-individual variability than those in the inbred strains, Fischer and Lewis rats. Our results indicated that these sensitizing conditions with KLH could practically apply these typical rat strains for induction of the primary and secondary responses enough to detect the immunosuppression by CPA.
The differences in the sensitivity of TDAR methodologies have also long been a topic for discussion (Herzyk and Holsapple, Citation2007; White et al., Citation2007). Traditional primary SRBC plaque responses have been reported to be more sensitive to detect the immunosuppressive effects of CPA and cyclosporine A than the primary KLH-ELISA responses in rodents (White et al., Citation2007). In the present study, CPA inhibited both KLH and SRBC-induced primary antibody responses at the same dose in either assay system. The data demonstrated that the KLH-ELISA under our experimental conditions has similar capability to detect CPA-induced immunosuppression with regards to the primary IgM response as compared to the primary response in the PFC assay or the SRBC-ELISA. According to a meta-analysis to compare data from several laboratories using SRBC and KLH with different assay formats, both antigens and assay formats showed the same pattern of responses to strong immunotoxicants, indicating that standardizing the choice of an antigen or assay format may not be critical in evaluation of immunotoxicity potential (Bugelski and Kim, Citation2007).
The duration of a study is another important point for the evaluation of pharmaceutical immunotoxicity. The non-clinical immunotoxicity guideline for human pharmaceuticals (ICH S8 Guideline, Citation2006) is based on a cause of concern approach using a weight-of-evidence review of various factors, which includes findings in standard toxicity studies. Consequently, in many cases, a 28-day study period could be employed for the TDAR assay, consistent with the conventional general toxicity studies. In the present work, we selected the 14-day study period in order to be able to eventually link outcomes here to those in short-term 14-day general toxicity studies used to support short-term clinical trials for human pharmaceutical candidate drugs (which also last up to 14-days, as per the ICH M3(R2) Guideline [2009]). We also believe that our study design with the KLH immunization is applicable to the 28-day repeated dose study design recommended by the ICH S8 Guideline. We feel this is the case because our recent work had also shown that the primary and secondary responses to KLH immunizations on Days 14 and 23 were sufficient to detect immunosuppressive effects of CPA on Days 20 and 29 (Kawai et al., Citation2010).
Acknowledgments
We would like to thank Satomi Komatsu, Masae Yagi, and Motoko Ajioka for their technical assistance.
Declaration of interest
The authors report no conflicts of interest. The authors alone are responsible for the content and writing of the paper.
References
- Bugelski, P. J. Kim, C. 2007. T-Dependent antigen response (TDAR) Tests: Meta-analysis of results generated across multiple laboratories. J. Immunotoxicol. 4:159–164.
- Cunningham, A. J. 1965. A method of increased sensitivity for detecting single antibody-forming cells. Nature 207:1106–1107.
- Cunningham, A. J. Szenberg, A. 1968. Further improvements in the plaque technique for detecting single antibody-forming cells. Immunology 14:599–600.
- Dodge, J. T., Mitchell, C. Hanahan, D. J. 1963. The preparation and chemical characteristics of hemoglobin-free ghosts of human erythrocytes. Arch. Biochem.Biophys. 100:119–130.
- Finco-Kent, D. Kawabata, T. T. 2005. Development and validation of a canine T-cell-dependent antibody response model for immunotoxicity evaluation. J. Immunotoxicol. 2:197–201.
- Friedman, E. M., Becker, K. A., Overstreet, D. H. Lawrence, D. A. 2002. Reduced primary antibody responses in a genetic animal model of depression. Psychosom. Med. 64:267–273.
- Gore, E R. 2006. Immune function tests for hazard identification: A paradigm shift in drug development. Basic Clin. Pharmacol. Toxicol. 98:331–335.
- Gore, E. R., Gower, J., Kurali, E., Sui, J. L., Bynum, J., Ennulat, D. Herzyk, D. J. 2004. Primary antibody response to keyhole limpet hemocyanin in rat as a model for immunotoxicity evaluation. Toxicology 197:23–35.
- Hanahan, D. J. Ekholm, J. E. 1974. The preparation of red cell ghosts (membranes). Methods Enzymol. 31:168–172.
- Henry, M. L. 1999. Cyclosporine and tacrolimus (FK506): A comparison of efficacy and safety profiles. Clin. Transplant. 13:209–220.
- Herzyk, D. J. Holsapple, M. 2007. Immunotoxicity evaluation by immune function tests: Focus on the T-dependent antibody response (TDAR) [Overview of a Workshop Session at the 45th Annual Meeting of the Society of Toxicology (SOT) March 5-9, 2006 San Diego, CA]. J. Immunotoxicol. 4:143–147.
- ICH (International Conference on Harmonization of Technical Requirements for Registration of Pharmaceuticals for Human Use) M3(R2) Guideline. 2009. Guidance on Nonclinical Safety Studies for the Conduct of Human Clinical Trials and Marketing Authorization for Pharmaceuticals M3(R2).
- ICH S8 Guideline. 2006. Immunotoxicity Studies for Human Pharmaceuticals S8.
- Institoris, L., Siroki, O., Undeger, U., Basaran, N. Desi, I. 2002. Immunotoxicological investigation in rats dosed repeatedly with combinations of cypermethrin, As(III), and Hg(II). Toxicology 172:59–67.
- Kawai, R., Nagayama, Y., Mori, K. Kouchi, Y. 2010. The inter-laboratory KLH-TDAR assay validation study II– investigation using an immunosuppressive agent. Proceedings of the 17th Annual Meeting of The Japanese Society of Immunotoxicology, Tsukuba-city in Japan, pp. 71–76.
- Luster, M. I., Portier, C., Pait, D. G., White, K. L. Jr., Gennings, C., Munson, A. E. Rosenthal, G. J. 1992. Risk assessment in immunotoxicology. I. Sensitivity and predictability of immune tests. Fundam. Appl. Toxicol. 18:200–210.
- Piccotti, J. R., Alvey, J. D., Reindel, J. F. Guzman, R. E. 2005. T-cell-dependent antibody response: Assay development in cynomolgus monkeys. J. Immunotoxicol. 2:191–196.
- Smialowicz, R. J., Riddle, M. M., Williams, W. C.-P. 1994. Species and strain comparisons of immunosuppression by 2-methoxyethanol and 2-methoxyacetic acid. Int. J. Immunopharmacol. 16:695–702.
- Smith, H. W., Winstead, C. J., Stank, K. K., Halstead, B. W. Wierda, D. 2003. A predictive F344 rat immunotoxicology model: Cellular parameters combined with humoral response to NP-CγG and KLH. Toxicology 194:129–145.
- Stenzel-Poore, M. P. Rittenberg, M. B. 1989. Clonal diversity, somatic mutation, and immune memory to phosphocholine-keyhole limpet hemocyanin. J. Immunol. 143:4123–4133.
- Temple, L., Kawabata, T. T., Munson, A. E. White, K.L. Jr. 1993. Comparison of ELISA and plaque-forming cell assays for measuring the humoral immune response to SRBC in rats and mice treated with benzo[a]pyrene or cyclophosphamide. Fundam. Appl. Toxicol. 21:412–419.
- Ulrich, P., Paul, G., Perentes, E., Mahl, A. Roman, D. 2004. Validation of immune function testing during a 4-week oral toxicity study with FK506. Toxicol. Lett. 149:123–131.
- White, K. L. Jr., Sheth, C. M. Peachee, V. L. 2007. Comparison of primary immune responses to SRBC and KLH in rodents. J. Immunotoxicol. 4:153–158.