Abstract
Establishing an in vivo cell-mediated immunity (CMI) assay, such as the delayed-type hypersensitivity (DTH) assay, has been identified as an important gap and recommended to receive highest priority for new model development in several workshops on developmental immunotoxicity. A Candida albicans DTH model has recently been developed that has the advantage over other DTH models, which use alternative sensitizing antigens, in that antigen-specific antibodies, which may interfere with the assay, are not produced. In addition, the in vivo C. albicans DTH model was demonstrated to be more sensitive in detecting immunosuppression than DTH models using keyhole limpet hemocyanin (KLH) or sheep red blood cells as antigens, as well as some ex vivo CMI assays. While KLH and sheep red blood cells are non-physiological immunogens, C. albicans is an important human pathogen. The present studies were conducted in order to optimize and validate the C. albicans DTH model for use in developmental immunotoxicity studies using juvenile rats. Three known immunosuppressive compounds with different mechanisms of action were tested in this model, cyclosprorin A (CsA), cyclophosphamide (CPS), and dexamethasone (DEX). Animals were sensitized with formalin-fixed C. albicans on postnatal day (PND) 28 and challenged with chitosan on PND 38. Drug was administered beginning on PND 23 and continued until PND 37. Exposure to each of the three immunotoxicants resulted in statistically significant decreases in the DTH response to C. albicans-derived chitosan. Decreases in footpad swelling were observed at ≥10 mg CsA/kg/day, ≥5 mg CPS/kg/day, and ≥0.03 mg DEX/kg/day. These results demonstrate that the C. albicans DTH model, optimized for use in juvenile rats, can be used to identify immunotoxic compounds, and fills the need for a sensitive in vivo CMI model for assessments of developmental immunotoxicity.
Abbreviations
Ab, antibody
APC, antigen presenting cell
BSA, bovine serum albumin
C. albicans, Candida albicans
CI, challenge interval
CMI, cell-mediated immunity
CO, challenge only
CPS, cyclophosphamide
CsA, cyclosporin A
CTL, cytotoxic T lymphocyte
DEX, dexamethasone
DIT, developmental immunotoxicity
DTH, delayed-type hypersensitivity
ip, intraperitoneal
KLH, keyhole limpet hemocyanin
MLR, mixed lymphocyte reaction
OVA, ovalbumin
PBS, phosphate-buffered saline
PND, postnatal day
sc, subcutaneous
SEM, standard error of the mean
SRBC, sheep red blood cells
Introduction
Over the last two decades there has been increased focus on the effects of pharmaceuticals and agricultural/industrial chemicals on the developing immune system. The concern is that the immune system of neonates and juveniles is inherently different from that of the adult, and therefore their responses to environmental and/or pharmaceutical exposures may be different. Developmental immunotoxicity (DIT) focuses on the concern that early life exposure may result in enhanced susceptibility to immune-related disease (i.e. viral, bacterial, neoplastic) compared to adults, that there may be unique effects not observed in adults, or that effects are more persistent compared to those following adult exposure.
Given the complexity of the immune system, a battery of tests is often needed in determination of immunotoxicity in order to fully assess immune function, and the use of specific assays is based on the cause for concern and/or the mechanism of action of the chemical or drug. Even well established and validated holistic assays such as the T-dependent antibody response (TDAR) are incapable of detecting immunotoxicity in all cases. In addition, immunotoxicity assays that have been applied to studies using adult animals may not be fully optimized for use in assessments of DIT.
While various assays have been applied to DIT assessments of both pharmaceuticals and environmental chemicals, establishment of a cell-mediated immunity (CMI) assay, such as the delayed-type hypersensitivity (DTH) assay, has been identified as an important gap and recommended to receive highest priority for new model development in several recent DIT reviews and workshops (Burns-Naas et al., Citation2008; Collinge et al., Citation2012; Holsapple, Citation2002; Holsapple et al., Citation2005). Currently there is no standardized holistic assay to assess immunotoxicity with respect to CMI. Typically CMI is evaluated ex vivo using lymphoproliferation assays following stimulation with T-lymphocyte mitogens or anti-CD3 antibodies, cytotoxic T-lymphocyte (CTL) assays, or mixed lymphocyte responses (MLR) (Luster et al., Citation1988). However, ex vivo assays often require subsequent cell isolation from peripheral blood or immune organ tissue with the consequence of drug removal, potentially allowing cells to recover from drug effects if cells are maintained in culture for several days. In contrast, the DTH response is an in vivo holistic evaluation of CMI.
DTH reactions (Type IV hypersensitivity) are mediated by antigen-specific effector T-lymphocytes, with the consequence being local skin swelling, erythema, induration, and cellular infiltration. Development of a DTH response requires two separate phases, sensitization and elicitation. During the sensitization phase animals are exposed to antigen, and antigen-presenting cells (APCs) migrate to the draining lymph nodes and activate T-lymphocytes, with consequent production of memory T-lymphocytes. During the elicitation (challenge) phase the same antigen (or immunogenic component of the antigen) is injected locally, is presented by APC to memory T-lymphocytes leading to the release of inflammatory cytokines and chemokines, including interferon (IFN)-γ, tumor necrosis factor (TNF)-α and interleukin (IL)-3/GM-CSF (granulocyte-monocyte colony-stimulating factor). These processes in part lead to increased expression of vascular endothelial cell adhesion molecules, increase blood vessel permeability, and allow circulating blood cells and accessory cells (e.g. neutrophils, macrophages, and T-lymphocytes) to enter the site, causing visible swelling which is best observed 24–48 h post-challenge. The recruited macrophages are also able to present antigen and as a result amplify the response.
While the DTH response has been well characterized (Kaufmann & Schaible, Citation2005) and the response is known to be cell-mediated and antigen-specific (Black, Citation1999), the choice of sensitizing antigen is critically important. However, while many different antigens have been used to measure DTH responses there is no widespread agreement among immunotoxicologists as to the choice of sensitizing and challenge antigens to be used. Antigens have included tetanus toxoid, Candida albicans, Mycobacterium bovis (Bacillus Calmett-Guerin – BCG), sheep red blood cells (SRBC), bovine serum albumin (BSA), keyhole limpet hemocyanin (KLH) and ovalbumin (OVA) (Exon et al., Citation1990; Henningsen et al., Citation1984; Hurtrel et al., Citation1984; Lagrange & Mackaness, Citation1975; Nghiem et al., Citation2002; Vos, Citation1980; Whittingham et al., Citation1982). However, many of these antigens, including KLH, SRBC, OVA, and BSA are typical immunogens used in holistic assessments of humoral immunity for T-lymphocyte antigens (Jerne & Nordin, Citation1963; Plitnick & Herzyk, Citation2010; Temple et al., Citation1993; White et al., Citation2010). As such these antigens lead to the robust production of antigen-specific antibodies, and there are examples where increased antibody production correlates with a decreased DTH response (Lagrange et al., Citation1980; Mackaness et al., Citation1974; Morikawa et al., Citation1991). In addition to the potential of antigen-specific antibodies to alter the observed DTH response, the optimal antigen concentration required to elicit either a DTH and humoral response is typically different. Therefore, attempts to measure both assays within the same animal with the same antigen will likely be suboptimal for one response versus the other.
An ideal antigen for assessment of DTH would generate a robust cell-mediated response, but not produce antigen-specific antibodies, allowing evaluation of the effects of drugs or chemicals on CMI without the influence from confounding factors of antibody production. Recently, Smith and White (Citation2010) developed a DTH model that used formalin-fixed C. albicans as the sensitizing agent, followed by challenge with chitosan, to produce a cell-mediated response with no detectable antibody component. Chitosan is a polysaccharide formed by deacylation of chitin, a purified extract from the C. albicans cell wall, produces minimal non-specific swelling following footpad injection, and does not initiate a humoral response. White et al. (Citation2012) validated the C. albicans DTH model in adult B6C3F1 mice for its ability to detect suppression (or lack thereof) of CMI following drug exposure, using four drugs (e.g., azathioprine, cyclophosphamide [CPS], cyclosprorin A [CsA] and dexamethasone [DEX]) with different mechanisms of action. The sensitivity of the in vivo C. albicans DTH model compared favorably to ex vivo CMI assays (Lebrec et al., Citation1994). In addition the C.albicans model was more sensitive than other DTH models that use KLH or SRBC as antigens, for detecting the immunosuppressive effects of benzo[a]pyrene exposure (Smith & White, Citation2010). Importantly, sensitization with C. albicans followed by challenge with chitosan did not result in detectable chitosan antibody production (Smith & White, Citation2010) and the cells observed to infiltrate the footpad following chitosan challenge are consistent with those observed for classical DTH responses (Hurtrel et al., Citation1984; Black, Citation1999).
The development of the C. albicans DTH model described by White and colleagues (Smith & White, Citation2010; White et al., Citation2012) was performed using adult B6C3F1 mice. However, particularly with respect to pharmaceutical assessments, the rat is more routinely used as the toxicology species. The purpose of the work described in this manuscript was to evaluate and optimize the C. albicans DTH model using juvenile rats, and to assess the assays ability to detect immunosuppression produced by known immunosuppressive compounds (CPS, CsA, and DEX), in order to provide a validated and optimized DTH model for use in DIT testing.
Materials and methods
Animals
Female Sprague--Dawley rat pups were obtained from Charles River Laboratories (Kingston, NY). Pups were shipped in litters of 10 along with lactating dams, and were received at least 5 days prior to study start. Pups were weaned at PND 21, randomized and group housed (n = 8–10), and provided normal rodent chow (Certified Rodent Diet 5002; PMI Feeds Inc., St. Louis, MO) and drinking water ad libitum. Rats were randomly assigned to respective treatment groups. All animal procedures were conducted in an Association for Assessment and Accreditation of Laboratory Care-accredited facility under an animal protocol approved by the Virginia Commonwealth University Institutional Animal Care and Use Committee.
Antigens
The Candida albicans DTH model used here was modified from the procedure established in the laboratory of Dr Steve Ullrich (Nghiem et al., Citation2002; Ramos et al., Citation2002) and further refined in the laboratory of Dr Kimber L. White Jr (Smith & White, Citation2010; White et al., Citation2012). Formalin-fixed C. albicans (ALerCHEK Inc., Portland, ME) was diluted in 0.9% saline and administered for sensitization at concentrations of 5 × 106–4 × 107 organisms/rat (0.2 ml/rat injected subcutaneously [sc] into the right flank). The C. albicans antigen chitosan (ALerCHEK Inc.) was administered sc at 50–200 µl/rat (at 1.5 mg/ml) into the right rear footpad for challenge.
Test articles
CsA (Novartis Pharmaceutical Corp., East Hanover, NJ) was administered orally at concentrations ranging from 1–30 mg/kg/day in corn oil vehicle. DEX (Sigma Aldrich, St. Louis, MO) was administered orally as a suspension in vehicle consisting of 0.5% methylcellulose with 0.2% Tween 80 at concentrations ranging from 0.003–0.300 mg/kg/day. CPS (Sigma) was prepared in phosphate-buffered saline (PBS, pH 7.4) vehicle and administered by intraperitoneal injection at doses ranging from 5–30 mg/kg/day.
Experimental design
The DTH response to C. albicans was evaluated essentially as described by Smith and White (Citation2010). In brief, formalin-fixed C. albicans was diluted in 0.9% NaCl to the appropriate concentration. Female juvenile rats were sensitized on PND 28 (unless otherwise stated) with formalin-fixed C. albicans (5 × 106–4 × 107 organisms/rat in a 200 µl volume) by sc injection in the right rear flank. On the day of challenge (PND 30-42) the right footpad was measured twice (pre-measurement) with a digital micrometer (Mitutoyo model No. 574-320, Tokyo, Japan) followed by sc injection of C. albicans antigen, chitosan (50–200 µl volume, at 1.5 mg/ml) into the right rear footpad. After 24 h, the right rear footpad thickness was again measured twice and footpad swelling for each animal was calculated (average post-challenge thickness – average pre-challenge thickness). The mean (± SEM) for each group was determined and data reported as (post-challenge thickness – pre-challenge thickness). Data are reported in terms of footpad swelling in units of mm × 100. Also included in each study was a group of juvenile female rats that were challenged in the right footpad but were neither exposed to test article nor sensitized with C. albicans. This “challenge only” (CO) group was used to determine background footpad swelling resulting from chitosan challenge. For studies that included dosing of rats with immunosuppressive drugs (CsA, CPS, DEX) in order to validate the C. albicans DTH model, dosing was initiated on PND 23 and continued until PND 37.
Statistical analysis
Results represent the mean ± SEM from studies that included 8–10 animals per group. Statistical analysis was performed using PRISM (Version 5.01) (GraphPad Software, Inc., La Jolla, CA). Pairwise comparisons between exposure groups and vehicle control groups for non-parametric data were made using the Wilcoxon Rank Test. In all evaluations, a p-value <0.05 indicated statistically significant differences.
Results
Determination of chitosan challenge level
Initial experiments set out to demonstrate that in non-sensitized CO animals (no immunization with C. albicans) the swelling induced by footpad challenge with C. albicans-derived chitosan was minimal, and produces no more swelling than that observed when the footpad is injected with an equivalent volume of saline. By optimizing the challenge level in this way, the footpad swelling induced by chitosan in C. albicans-sensitized animals would reflect a true DTH response. shows the results of CO studies for chitosan when injected into the footpad of juvenile (PND 38) rats. Challenge with chitosan at 75, 150 and 300 µg/rat resulted in swelling that was no different from that seen when the footpad was injected with an equivalent volume (50, 100 and 200 µl) of saline. From the results of this study, the challenge level of chitosan selected for future studies was 150 µg. This quantity of chitosan produced no increase in footpad swelling over that observed for saline alone, and also produced the lowest level of swelling for the levels of chitosan assessed in this study.
Figure 1. Footpad swelling in naïve juvenile rats following challenge with the C. albicans antigen chitosan. Pre-measurements of the right footpad thickness of naïve juvenile rats (PND 29) were obtained with a digital micrometer prior to challenge with either 75, 150, or 300 µg of chitosan or an equivalent volume of 0.9% saline. The thickness of the right footpad was again measured 24 h later, and the change in footpad thickness for each rat was calculated (post-challenge – pre-challenge thickness). The data are expressed as footpad swelling (mm × 100). Values represent the mean ± SEM from eight animals per group.
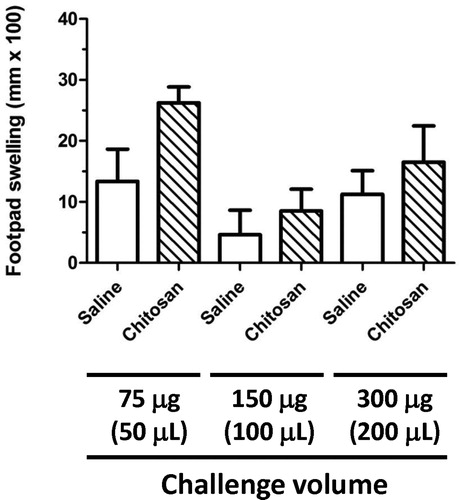
Sensitization timecourse
The results of timecourse studies conducted in order to identify the peak challenge interval (CI) in the juvenile rat C. albicans DTH model are shown in . Groups of animals were sensitized with 2 × 107 formalin-fixed C. albicans organisms on PND 26 and challenged by footpad injection of chitosan (150 µg/rat) either 6, 8, 10, 12 or 14 days post-sensitization (PND 32, 34, 36, 38, or 40, respectively). Twenty-four hours post-challenge, footpad swelling was statistically significantly increased over the CO control group at all timepoints examined. Initial studies () indicated that, although footpad swelling following challenge 8 days post-sensitization was greater, albeit slightly, than that observed 6 days post-challenge, it was not evident that the peak response was reached with a CI of 8 days. However, subsequent studies () indicated that there was little or no difference in the responses observed at either 8 or 10 days post-sensitization and that, indeed, a plateau in the response had been reached. From the results of these timecourse studies, the CI selected was 10 days (swelling 55 mm × 100). Footpad measurements taken 48-h post-challenge indicated that the response was diminished at this time point relative to 24 h post-challenge (data not shown). While both 24- and 48-h post-challenge measurements were taken in all subsequent studies, the greatest responses were noted at 24 h in all cases; therefore, 48-h measurements are not reported here.
Figure 2. Sensitization timecourse studies for C. albicans in the juvenile rat delayed-type hypersensitivity model. Juvenile rats (PND 28) were sensitized with 2 × 107 C. albicans organisms/rat (200 µl volume) by subcutaneous injection in the right rear flank. On either Day 6, 8, 10, 12 or 14 (PND 34, 36, 38, 40 or 42) pre-measurements of the right rear footpad thickness were obtained with a digital micrometer, and rats subsequently challenged in the right footpad with either 150 µg (100 µl) of chitosan or an equivalent volume of 0.9% saline. The thickness of the right footpad was measured 24 h later and the change in footpad thickness was calculated (post-challenge – pre-challenge thickness). Background footpad swelling was determined in groups of juvenile rats that were challenged but not sensitized (challenge only). Data expressed as footpad swelling (mm × 100). Values represent the mean ± SEM from 10 animals per group. Panels (a) and (b) represent two independent experiments.
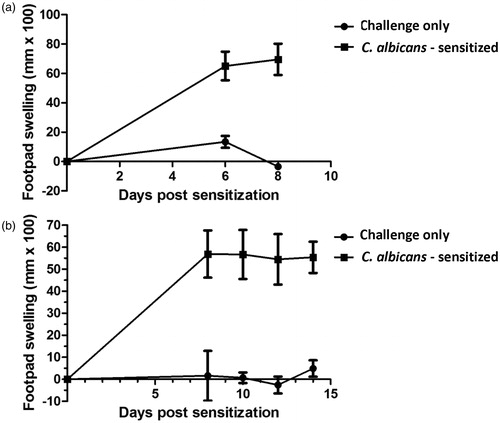
Sensitization dose–response
In order to optimize the sensitization dose for formalin-fixed C. albicans, a dose response study was performed whereby juvenile rats were sensitized by sc injection of either 5 × 106, 1 × 107, 2 × 107 or 4 × 107 formalin-fixed C. albicans on PND 28. Animals were subsequently challenged in the right rear footpad with 150 µg chitosan/rat on PND 38. As shown in , a dose-responsive increase in footpad swelling was observed up to a peak sensitization concentration of 2 × 107 organisms/rat (177 [±24.65] mm × 100), with no further increase observed when 4 × 107 organisms/rat were used (152.2 [±14.73] mm × 100).
Figure 3. Effect of C. albicans sensitization dose on the footpad swelling response of juvenile rats in the delayed-type hypersensitivity model. Juvenile rats were sensitized on PND 28 with 5 × 106 – 4 × 107 C. albicans organisms per rat (200 µl volume) by sc injection into the right rear flank 10 days prior to challenge. On the day of challenge (PND 38) pre-measurements of the right rear footpad thickness were obtained with a digital micrometer, and rats subsequently challenged in the right footpad by subcutaneous injection of 150 µg (100 µl) of chitosan. The right footpad thickness was measured 24 h later, and the change in footpad thickness calculated (post-challenge – pre-challenge thickness). Background footpad swelling was determined using a group of juvenile rats that were challenged but were not sensitized (challenge only). Data are expressed as footpad swelling (mm × 100) and values represent the mean ± SEM determined from 10 rats per group.
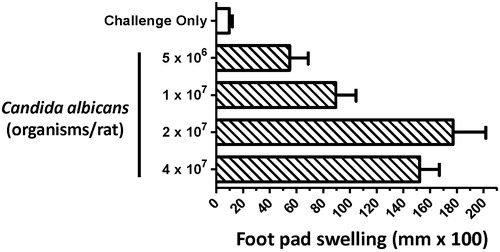
Effects of immune suppressive compounds on the C. albicans DTH footpad swelling model
Based on the previously described optimization studies, the juvenile rat C. albicans DTH model was validated using three known immunosuppressive drugs, CsA, CPS and DEX. In each study, dosing began on PND 23 and continued until PND 37. Sensitization with C. albicans (2 × 107 organisms/rat) occurred on PND 28, and animals were subsequently challenged with chitosan (150 µg/rat) on PND 38. Daily exposure of juvenile rats to each of these immunosuppressive agents for 14 days led to significant immunosuppressive effects in the C. albicans DTH model.
Juvenile rats were administered CsA (1, 3, 10, or 30 mg/kg/day), CPS (5, 10, 20 or 30 mg/kg/day), or DEX (0.003, 0.010, 0.030, 0.1, or 0.3 mg/kg/day) (, respectively). When compared to the vehicle control group, footpad swelling was significantly decreased in animals administered either 10 or 30 mg CSA/kg/day by 90 and 94%, respectively (). No decrease in footpad swelling was observed when CsA was administered at either 1 or 3 mg/kg/day. Administration of CPS significantly decreased footpad swelling at all doses examined (). Responses were decreased by 52, 54, 82 and 100% relative to vehicle control in animals administered 5, 10, 20 or 30 mg CPS/kg/day, respectively. No decrease in footpad swelling was observed in animals administered either 0.003 or 0.010 mg DEX/kg/day (). However, at doses of 0.1 and 0.3 mg/kg/day statistically significant decreases were observed, with decreases of 68 and 100% observed relative to vehicle control. While a 63% decrease relative to the vehicle control group was noted in the 0.030 mg/kg/day dose group, this did not reach the accepted level of statistical significance. For each of these compounds, suppression of footpad swelling was observed at doses that did not produce general toxic effects including effects on individual body weights.
Figure 4. Effect of immunosuppressive drugs on juvenile rat footpad swelling using the C. albicans delayed-type hypersensitivity model. Juvenile rats were administered either vehicle, or (a) CsA, (b) CPS, or (c) DEX at the indicated doses. Dosing began on PND 23, 5 days prior to sensitization, and ended the day prior to challenge (PND 37). Rats were sensitized with 2 × 107 C. albicans organisms/rat by sc injection into the right rear flank on PND 28. On PND 38, pre-challenge footpad thickness measurements were obtained using a digital micrometer, and rats subsequently challenged by subcutaneous injection of 150 µg (100 µl) chitosan into the right footpad. Footpad thickness was again measured 24 h post-challenge and the change in footpad thickness calculated (post-challenge – pre-challenge thickness). Background swelling was determined in groups of juvenile rats that were challenged but not sensitized (challenge only). Data expressed as footpad swelling (mm × 100). Values represent the mean ± SEM from 10 animals per group. *Group mean values statistically different (p < 0.05) from control.
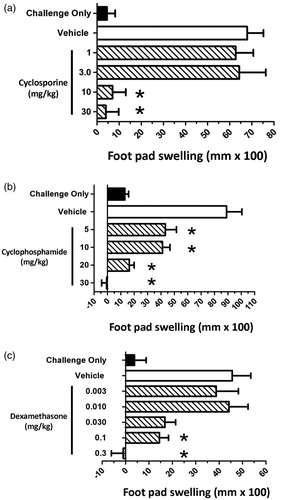
The specific immune cell types involved in the development of the footpad swelling response to C. albicans have been evaluated by histology (Smith & White, Citation2010) and immunohistochemistry (data not shown) and is a T-lymphocyte driven process with no observable B-lymphocyte involvement. While the three known immunosuppressive compounds do have different mechanisms of action, all would be anticipated to diminish the DTH response due to their effects on the cell types involved in the response. While CSA predominantly alters T-cell cytokine responses, CPS and DEX are generally less selective with respect to the cell types affected. A negative control compound, benzo[e]pyrene, was evaluated in the initial validation of the C. albicans DTH model in adult mice (White et al., Citation2012) and was indeed found not to alter the observed DTH response. Given the likelihood that this compound would also be negative in juvenile animals, this negative control was not performed as part of this study in an effort to reduce the number of animals used with respect to the “3Rs” (Replacement, Reduction, and Refinement). Additional compounds that specifically target other immune cell subsets, for example B-lymphocytes, that have been demonstrated not to play no role in DTH responses (Herrmann et al., Citation1988) were not evaluated as part of this study.
Discussion
A complete assessment of the ability of xenobiotics to produce DIT requires a battery of assays which can be used on a case-by-case basis, according to the specific cause for concern and mechanism of action. While some functional immunotoxicity assays have been developed and validated for use in rodents, few are currently accepted as sensitive and predictive in developing animals (Collinge et al., Citation2012). For the most robust assessment of DIT, immunopathology can be combined with an assessment of humoral immunity (e.g. TDAR) and an evaluation of cellular immunity such as DTH, and provides the broadest assessment of immunocompetence (Burns-Naas et al., Citation2008; Dietert & Burns-Naas, Citation2008; Dietert & Holsapple, Citation2007; Holsapple et al., Citation2004). With respect to the development of methods specifically for DIT testing, participants in a recent ILSI-HESI-ITC (International Life Sciences Institute-Health and Environmental Sciences Institute-Immunotoxicology Technical Committee) sponsored workshop identified the development of a robust CMI assay that could be applied to DIT assessments as a high priority (Collinge et al., Citation2012).
The notion that CMI assays are important in the overall assessment of DIT is consistent with recent reviews (Burns-Naas et al., Citation2008; Dietert & Holsapple, Citation2007; Holsapple et al., Citation2005, Citation2007) that stress the need to broaden the scope of DIT beyond the TDAR, and it is recommended that a CMI assay be included in DIT protocols (Dietert & Holsapple, Citation2007; Holsapple, Citation2002; Holsapple et al., Citation2005; Luster et al., Citation2003). The importance of this is highlighted by the demonstration that some developmental immunotoxicants fail to affect the TDAR response but can alter cellular immunity (Bunn et al., Citation2001a, b; Gehrs & Smialowicz, Citation1999; Karrow et al., Citation2004; Miller et al., Citation1998). In addition, it is possible that certain cellular immune assays may be performed earlier in postnatal development than the TDAR. For example, in vitro CTL responses using lymph node cells from 5-day-old mice show responses comparable to those of adult animals (Fadel & Sarzotti, Citation2000; Piguet et al., Citation1981), while it is difficult to produce a reproducible TDAR response in rodents prior to PND 21. While both a DTH response and the TDAR require functional T-lymphocytes, DTH responses may be detectable earlier in immune system development in rodents because fully functional B-lymphocytes, required for the TDAR, are observed later than for T-lymphocytes.
The DTH assay has been used in a number of DIT studies (see Collinge et al., Citation2010, and references therein) and has been part of the National Toxicology Program's Tier 2 test panel for cellular immune responses (Luster et al., Citation1988) and can be performed with a variety of antigens. However, the use of adjuvants is concerning (Holsapple, Citation2002), as is the potential of some antigens to produce antigen-specific antibodies. Such antibodies may either decrease DTH responses by neutralizing antigen, or may contribute to the measured CMI response, as part of a Type III hypersensitivity response (Collinge et al., Citation2012). Therefore, distinguishing the humoral from the cellular immune response is critical. It has been suggested that when a protocol is truly optimized for a maximal CMI response to an antigen such as KLH, no measureable antibody response is observed, and vice-versa (Bretcher, Citation1994; Burleson et al., Citation2009). In these cases separate groups of animals would be needed when using an immunogen to evaluate both CMI and humoral responses (Collinge et al., Citation2012). However, use of an antigen that produces no antibody response would be the ideal to accurately determine a CMI response.
The recent establishment of a rodent DTH model that uses formalin-fixed C. albicans as the sensitizing antigen, followed by challenge with chitosan (Smith & White, Citation2010), provides a model in which confounding antigen-specific antibodies are not produced. We have confirmed the lack of an antibody response to chitosan in rats, and have been unable to detect significant chitosan antibody in serum even after multiple injections of the antigen (data not shown). This is consistent with previous reports that the antigenicity of chitosan is weak (Felse & Panda, Citation1999) and that generation of antibodies to chitosan is difficult (El-Gueddari et al., Citation2002). It has been demonstrated that several injections over an extended period of time, with the addition of adjuvants, is necessary to generate significant antibody titers (Spindler-Barth & Buss, Citation1997). However, chitosan is capable of activating macrophages and promoting T-helper (TH)-1 cytokine production with concomitant down-regulation of TH2 cytokines (Chen et al., Citation2008; Lee, Citation2009; Lee et al., Citation2008; Muzzarelli, Citation2010; Shibata et al., Citation1997, Citation2001), consistent with a robust DTH response (i.e. TH1) and absence of a noticeable antibody response. Given this lack of a humoral response, the C. albicans DTH model was further optimized and validated for use in DIT assessments in rat.
For the studies described within this report assessments of DTH were conducted post-weaning and throughout the juvenile stage of young rats. For the purposes of this study, rats were sensitized with C. albicans on PND 26–28 to allow for sufficient dosing post-weaning to ensure that drug was on-board prior to the sensitization phase. However, we have been able to demonstrate that a significant DTH response can be measured in rats sensitized with C. albicans on PND 11 and challenged with chitosan on PND 21, the results of which will be described in a subsequent publication.
In adult mice, the peak challenge interval (CI) between sensitization and challenge was determined to be 7–8 days (Smith & White, Citation2010) and in rats sensitized on PND 26 peak responses to challenge were observed after 8–10 days. Whether the shift in peak CI between adult mice and juvenile rats represents a species difference or reflects a delayed ability of juvenile rats to develop memory T-lymphocytes to a specific antigen is not known. The relatively short, 21-day gestation period in rats, compared to humans, largely accounts for their comparatively immature immune system at birth (Collinge et al., Citation2010). At birth, in rodents, relatively low B- and T-lymphocyte numbers are observed in the peripheral blood (Ladics et al., Citation2000) and have characteristics of naïve lymphocytes. At weaning the 21-day old rat has comparable numbers of B-lymphocytes to the adult but there are relatively fewer numbers of T-lymphocytes. However, peripheral blood lymphocyte numbers do not necessarily reflect function and the robustness of a specific immune response. Further studies would be needed to determine if the longer CI required for an optimal footpad swelling response in juvenile rats is due to reduced numbers of T-lymphocytes, decreased ability of those T-lymphocytes to form memory cells, or is due to differences in other cell types or responses. The in vivo DTH model is a holistic assay that uses footpad swelling as an end-stage measure of the response and as such requires the complex cooperation of multiple cell types and processes.
Three compounds, CsA, CPS, and DEX, were evaluated in this study. CPS is an alkylating agent, DEX a synthetic glucocorticoid, and CsA alters T-lymphocyte cytokine production through inhibition of calcineurin. Each of the compounds produced a dose-related decrease in the footpad swelling response. Inhibition was achieved with doses of 30, 0.3, and 30 mg/kg/day of CPS, DEX, and CSA, respectively. While it could be concluded that juvenile rats were more sensitive to DEX than to either CSP and CsA based on the dose administered, and taking into account the differences in the molecular masses of each of these compounds, it should be noted that pharmacokinetic analysis was not performed on animal serum to determine the level of exposure. Therefore, comparisons should be made with caution. In addition, while CsA and DEX were administered orally, CPS was administered intraperitoneally, and the route of administration may have impacted exposure.
While the results of this juvenile rat study compared favorably with data reported by White et al. (Citation2012), it is clear that juvenile rats are significantly more sensitive to each of the immunosuppressive compounds than were adult mice. This is most clear from the results of DEX and CsA. For DEX, 0.1 mg/kg/day had no effect on the footpad swelling in adult mice, whereas a 63% reduction in footpad swelling was observed at 0.03 mg/kg/day in juvenile rats. Similarly, for CSA, while only a 33% reduction in the response was observed in adult mice administered 10 mg/kg/day, a 90% reduction in the response was observed in juvenile rats at the same dose. While species differences must be factored into these comparisons, the data are consistent with previous reports of increased sensitivity of the developing immune system with these compounds relative to the adult immune system, including differential effects on DTH responses (Dietert et al., Citation2003).
Further work is needed and is currently being conducted to determine the DTH response to C. albicans in younger rats, including pre-weaning. While the paradigm for DIT testing of environmental chemicals has historically been to perform DIT studies in rats by dosing pregnant dams throughout gestation and lactation, and in some cases pups both pre- and post-weaning, followed by assessing immune function at PND 42, the paradigm for pharmaceuticals is different. This is largely because unintentional exposure of offspring to chemicals may occur at any point pre- or post-natally. However, in the case of drug development, exposure of the developing immune system to drug likely only occurs due to inadvertent exposure in utero or via breast milk, or direct dosing of children to treat a childhood disease. In the latter instance it is essential in preclinical DIT testing to assess immune function at a time in development equivalent to that of the intended childhood population. Therefore, the ability to apply immune function tests such as DTH at any stage prior to or subsequent to weaning may be important. The results described in this report establish the use of the C. albicans DTH model for use in rat DIT studies.
Conclusions
We have successfully optimized a DTH model in juvenile rats using C. albicans as the sensitizing antigen. This assay was validated using three known immunosuppressive compounds, CPS, CsA, and DEX. The C. albicans DTH model is a sensitive in vivo assay of CMI and should be broadly applicable to DIT assessments of both environmental chemicals and pharmaceutical drugs, and fills a key gap previously identified in several DIT workshops.
Declaration of interest
Dr Kimber L. White, Jr. is the owner of a company, ImmunoTox®, Inc. that conducts immunotoxicological studies under Good Laboratory Practices (GLP).
Acknowledgements
The authors wish to extend special thanks to Julia Brantley, Joseph Hoehn, and Taneishia Edwards Taylor for excellent technical assistance.
References
- Black, C. A. 1999. Delayed type hypersensitivity: Current theories with an historic perspective. Dermatol. Online J. 5:7
- Bretcher, P. A. 1994. Requirements and basis for efficaceous vaccination by a low antigen dose regimen against intracellular pathogens uniquely susceptible to cell-mediated attack. In: Strategies in Vaccine Design (Ada, G. L.). Austin, TX: RG Landes, pp. 99–112
- Bunn, T. L., Dietert, R. R., and Ladics, G. S. 2001a. Developmental immunotoxicology assessments in the rat: Age, gender, and strain comparisons after exposure to lead. Toxicol. Meth. 11:41–58
- Bunn, T. L., Parsons, P. J., Kao, E., and Dietert, R. R. 2001b. Exposure to lead during critical windows of embryonic development: Differential immunotoxic outcome based on stage of exposure and gender. Toxicol. Sci. 64:57–66
- Burleson, G. R., Burleson, F. G., and Dietert, R. R. 2009. The cytotoxic T-lymphocyte (CTL) assay for evaluating cell-mediated immune function. In: Immunotoxicity Testing Series: Methods in Molecular Biology (Dietert, R. R.). Totowa, NJ: Humana press, Inc., pp. 199–205
- Burns-Naas, L. A., Hastings, K. L., Ladics, G. S., et al. 2008. What's so special about the developing immune system? Int. J. Toxicol. 27:223–254
- Chen, C. L., Wang, Y. M., Liu, C. F., et al. 2008. The effect of water-soluble chitosan on macrophage activation and the attenuation of mite allergen-induced airway inflammation. Biomaterials 29:2173–2182
- Collinge, M., Burns-Naas, L. A., Chellman, G. J., et al. 2012. Developmental immunotoxicity (DIT) testing of pharmaceuticals: Current practices, state of the science, knowledge gaps, and recommendations. J. Immunotoxicol. 9:210--230
- Collinge, M., Burns-Naas, L. A., and Kawabata, T. T. 2010. Developmental immunotoxicology. In: Reproductive Toxicology. 6th ed. (Kapp, R. W., and Tyl, R. W.). New York: Informa Healthcare, pp. 134–156
- Dietert, R. R., and Burns-Naas, L. A. 2008. Developmental immunotoxicity in rodents. In: Immunotoxicology strategies for pharmaceutical safety assessment (Herzyk, D. J., and Bussiere, J. L.). Hoboken, NJ: John Wiley and Sons, Inc., pp. 273–297
- Dietert, R. R., and Holsapple, M. P. 2007. Methodologies for developmental immunotoxicity (DIT) testing. Methods 41:123–131
- Dietert, R. R., Lee, J. E., Olsen, J., et al. 2003. Developmental immunotoxicity of dexamethasone: Comparison of fetal versus adult exposures. Toxicology 194:163–176
- El-Gueddari, N. E., Rauchhaus, U., Moerschbacher, B. M., et al. 2002. Developmentally regulated conversion of surface exposed chitin to chitosan in cell walls of plant pathogenic fungi. New Phytol. 156:103–112
- Exon, J. H., Bussiere, J. L., and Mather, G. G. 1990. Immunotoxicity testing in the rat: An improved multiple assay model. Int. J. Immunopharmacol. 12:699–701
- Fadel, S., and Sarzotti, M. 2000. Cellular immune responses in neonates. Int. Rev. Immunol. 19:173–193
- Felse, P. A., and Panda, T. 1999. Studies on applications of chitin and its derivatives. Bioprocess Eng. 20:505–512
- Gehrs, B. C., and Smialowicz, R. J. 1999. Persistent suppression of delayed-type hypersensitivity in adult F344 rats after perinatal exposure to 2,3,7,8-tetrachlorodibenzo-p-dioxin. Toxicology 134:79–88
- Henningsen, G. M., Koller, L. D., Exon, J. H., et al. 1984. A sensitive delayed-type hypersensitivity model in the rat for assessing in vivo cell-mediated immunity. J. Immunol. Meth. 70:153–165
- Herrmann, P., Schreier, M. H., Bazin, H., et al. 1988. Delayed-type hypersensitivity (DTH) in anti-IgM-treated B-cell-depleted mice: Analysis of induction and effector phase. Immunobiology 177:382–389
- Holsapple, M. P. 2002. Developmental immunotoxicology and risk assessment: A workshop summary. Hum. Exp. Toxicol. 21:473–478
- Holsapple, M. P., Burns-Naas, L. A., Hastings, K. L., et al. 2005. A proposed testing framework for developmental immunotoxicology (DIT). Toxicol. Sci. 83:18–24
- Holsapple, M. P., Paustenbach, D. J., Charnley, G., et al. 2004. Symposium summary: Children's health risk – what's so special about the developing immune system? Toxicol. Appl. Pharmacol. 199:61–70
- Holsapple, M. P., van der Laan, J. W., and van Loveren, H. 2007. Development of a framework for developmental immunotoxicity (DIT) testing. In: Immunotoxicology and Immunopharmacology (Luebke, R. W., House, R., and Kimber, I.). Boca Raton, FL: CRC Press, pp. 347–361
- Hurtrel, B., Hurtrel, M., and Lagrange, P. H. 1984. Time course and histological differences between sheep red blood cells and tuberculin DTH reactions in mice. Ann. Immunol. (Paris) 135C:219–230
- Jerne, N. K., and Nordin, A. A. 1963. Plaque formation in agar by single antibody-producing cells. Science 140:405
- Karrow, N. A., Guo, T. L., Delclos, K. B., et al. 2004. Nonylphenol alters the activity of splenic NK cells and the numbers of leukocyte subpopulations in Sprague-Dawley rats: A two-generation feeding study. Toxicology 196:237–245
- Kaufmann, S. H., and Schaible, U. E. 2005. 100th anniversary of Robert Koch's Nobel Prize for the discovery of the tubercle bacillus. Trends Microbiol. 13:469–475
- Ladics, G. S., Smith, C., and Bunn, T. L. 2000. Characterization of an approach to developmental immunotoxicology assessment in the rat using SRBS as an antigen. Toxicol. Meth. 10:283–311
- Lagrange, P. H., and Mackaness, G. B. 1975. A stable form of delayed-type hypersensitivity. J. Exp. Med. 141:82–96
- Lagrange, P. H., Michel, J. C., Hurtrel, B., et al. 1980. Delayed-type hypersenstivity to sheep red blood cells in selected lines of mice with high or low antibody responses. Ann. Immunol. (Paris) 131C:257–277
- Lebrec, H., Blot, C., Pequet, S., et al. 1994. Immunotoxicological investigation using pharmaceutical drugs: in vivo evaluation of immune effects. Fundam. Appl. Toxicol. 23:159–168
- Lee, C. G. 2009. Chitin, chitinases and chitinase-like proteins in allergic inflammation and tissue remodeling. Yonsei Med. J. 50:22–30
- Lee, C. G., Da Silva, C. A., Lee, J. Y., et al. 2008. Chitin regulation of immune responses: An old molecule with new roles. Curr. Opin. Immunol. 20:684–689
- Luster, M. I., Dean, J. H., and Germolec, D. R. 2003. Consensus workshop on methods to evaluate developmental immunotoxicity. Environ. Health Perspect. 111:579–583
- Luster, M. I., Munson, A. E., Thomas, P. T., et al. 1988. Development of a testing battery to assess chemical-induced immunotoxicity: National Toxicology Program's guidelines for immunotoxicity evaluation in mice. Fundam. Appl. Toxicol. 10:2–19
- Mackaness, G. B., Lagrange, P. H., Miller, T. E., et al. 1974. Feedback inhibition of specifically sensitized lymphocytes. J. Exp. Med. 139:543–559
- Miller, T. E., Golemboski, K. A., Ha, R. S., et al. 1998. Developmental exposure to lead causes persistent immunotoxicity in F344 rats. Toxicol. Sci. 42:129–135
- Morikawa, Y., Kuribayashi, K., Yoshikawa, F., et al. 1991. Role of antibodies in the regulation of delayed-type hypersensitivity. Immunology 74:146–152
- Muzzarelli, R. A. 2010. Chitins and chitosans as immunoadjuvants and non-allergenic drug carriers. Mar. Drugs 8:292–312
- Nghiem, D. X., Walterscheid, J. P., Kazimi, N., et al. 2002. Ultraviolet radiation-induced immunosuppression of delayed-type hypersensitivity in mice. Methods 28:25–33
- Piguet, P. F., Irle, C., Kollatte, E., et al. 1981. Post-thymic T-lymphocyte maturation during ontogenesis. J. Exp. Med. 154:581–593
- Plitnick, L. M., and Herzyk, D. J. 2010. The T-dependent antibody response to keyhole limpet hemocyanin in rodents. Methods Mol. Biol. 598:159–171
- Ramos, G., Nghiem, D. X., Walterscheid, J. P., et al. 2002. Dermal application of jet fuel suppresses secondary immune reactions. Toxicol. Appl. Pharmacol. 180:136–144
- Shibata, Y., Honda, I., Justice, J. P., et al. 2001. TH1adjuvant N-acetyl-D-glucosamine polymer up-regulates TH1 immunity but down-regulates TH2 immunity against a mycobacterial protein (MPB-59) in IL-10 knockout and wild-type mice. Infect. Immun. 69:6123–6130
- Shibata, Y., Metzger, W. J., and Myrvik, Q. N. 1997. Chitin particle-induced cell-mediated immunity is inhibited by soluble mannan: Mannose receptor-mediated phagocytosis initiates IL-12 production. J. Immunol. 159:2462–2467
- Smith, M. J., and White, K. L. Jr 2010. Establishment and comparison of delayed-type hypersensitivity models in the BCF mouse. J. Immunotoxicol. 7:308–317
- Spindler-Barth, M., and Buss, U. 1997. ELISA for determination of chitin and chitosan. In: Chitin Handbook (Muzzarelli, R. A., and Peter, M. G.). Grottammare, Italy: European Chitin Society
- Temple, L., Kawabata, T. T., Munson, A. E., et al. 1993. Comparison of ELISA and plaque-forming cell assays for measuring the humoral immune response to SRBC in rats and mice treated with benzo[a]pyrene or cyclophosphamide. Fundam. Appl. Toxicol. 21:412–419
- Vos, J. G. 1980. Immunotoxicity assessment: Screening and function studies. Arch. Toxicol. Suppl. 4:95–108
- White, K. L. Jr., McLoughlin, C. E., Auttachoat, W., et al. 2012. Validation of the Candida albicans delayed-type hypersensitivity (DTH) model in the female B6C3F1 mouse for use in immunotoxicological investigations. J. Immunotoxicol. 9:141–147
- White, K. L. Jr., Musgrove, D. L., and Brown, R. D. 2010. The sheep erythrocyte T-dependent antibody response (TDAR). Methods Mol. Biol. 598:173–184
- Whittingham, S., Feery, B., and Mackay, I. R. 1982. Use of tetanus toxoid for testing cell-mediated immunity. Aust. N.Z. J. Med. 12:511–514