Abstract
Clostridium tetani causes a life-threatening infectious disease by production of tetanus neurotoxin (TeNT), a 150 kDa molecule composed of light (LC) and heavy chain (HC) polypeptides. The TeNT HC contains an N-terminal domain critical for LC translocation and a C-terminal toxin receptor-binding domain known as fragment C. Despite extensive investigations on epitope specificity of anti-TeNT antibodies, the immunodominant neutralizing epitopes of the toxin are poorly defined. This study describes the generation and characterization of four monoclonal antibodies (MAb) specific for TeNT. The characteristics of each MAb were explored in terms of isotype, specificity, affinity, and immuno-globulin heavy chain variable region (IGHV) gene usage using ELISA, Western blotting, and sequencing techniques. The toxin neutralizing activity of the MAbs was also investigated using the in vitro GT1b neutralizing assay. The data demonstrated that all MAbs bind to tetanus toxin and toxoid. Sub-fragments binding analysis showed that two MAbs react with fragment C, one with both fragment C and LC, and one with LC. Only the two fragment C-specific MAbs were able to neutralize the toxin. Sequencing of the expressed VH and VL genes revealed rearrangements of various VH and VL gene segments in all hybridoma clones. Clonality of the hybridomas was also confirmed by a competition assay that showed recognition of distinct epitopes by these MAbs. The results suggest the importance of TeNT fragment C in terms of immunogenicity and toxin neutralization activity.
Introduction
Tetanus neurotoxin (TeNT) and seven antigenically-distinct botulinum neurotoxins (BoNT/A-G) together comprise the family of clostridial neurotoxins (CNT). These toxins are exclusively responsible for the neuroparalytic syndromes of tetanus and botulism (Rawlings & Barrett, Citation1995). The basic mode of action is similar in all CNTs; they cleave a specific family of proteins integral to the exocytotic process (i.e. soluble NSF attachment protein receptor), thereby avoiding the synaptic SNARE complex formation that finally ends up in blocking vesicular mediator release to the extra-cellular environment (Chen et al., Citation2009; Galazka & Gasse, Citation1995).
Tetanus toxin is produced by a single clostridial species (Clostridium tetani) as a 150 kDa single polypeptide that is subsequently cleaved to an active holotoxin (Montecucco & Schiavo, Citation1994). This holotoxin is composed of a 50 kDa N-terminal light (LC) and a 100 kDa C-terminal heavy chain (HC) linked by a single disulfide bond (Schiavo et al., Citation1990, Citation1992). Intoxication of neurons with TeNT requires several steps: binding to its receptor on the pre-synaptic membrane of α-motor neurons, internalization, retrograde transport from the axon terminal to inhibitory interneurons and transynaptic transport prior to its intoxicating action (Montecucco & Schiavo, Citation1995; Schiavo et al., Citation1994). Different domains of TeNT take part in this procedure. The LC is a zinc metalloprotease and contains the catalytic domain of the neurotoxin. This zinc-endo-protease specifically cleaves the neuronal SNARE protein VAMP2 (vesicle associated membrane protein-2) and holds back the release of inhibitory neurotransmitter glycine and γ-aminobutyric acid that ultimately leads to spastic paralysis, tetanus (Chen et al., Citation2009; Galazka & Gasse, Citation1995). The TeNT HC is composed of two domains, each of approximately 50 kDa; the N-terminal fragment is critical for LC translocation and the C-terminal fragment (Fragment C) is the receptor binding domain (Lalli et al., Citation1999).
Although molecular mechanisms of TeNT toxicity are well characterized, the mechanism whereby TeNT binds to neurons is not fully understood. Gangliosides of the 1b series, most notably GT1b and GD1d, are clearly implicated as a component of TeNT receptor and have been shown to be essential for intoxication of neurons with tetanus neurotoxin (Halpern et al., Citation1990; Louch et al., Citation2002; Williamson et al., Citation1999). However, gangliosides are probably not the sole component of a TeNT receptor. Several lines of evidence suggest involvement of a dual receptor mechanism in the binding of the TeNT which includes gangliosides and a 15 kDa glycoprotein, which is present in both toxin-sensitive PC12 cells and spinal cord neurons (Herreros et al., Citation2000a, Citationb).
Treatment with formaldehyde detoxifies TeNT and converts it into a stable inactive form known as tetanus toxoid, the major constituent of tetanus vaccines. Formaldehyde reacts with ε-amino groups of lysine residues to provide an unstable product that can then react with a second amino group to form a stable methylene bridge (Di Tommaso et al., Citation1994). Formaldehyde-treated tetanus vaccine induces a very strong and long lasting humoral immune response in human. Vaccination with tetanus toxoid leads to antigen uptake by antigen-presenting cells (APC), antigen presentation via MHC Class Π molecules to CD4+ helper T-cells, secretion of cytokines by helper T-cells, and, finally, antibody production by B-cells (Demotz et al., Citation1989; Mayer et al., Citation2002).
These antibodies that are directed against multiple epitopes of TeNT neutralize the toxin and are used for tetanus prophylaxis (Lang et al., Citation1993). Identification of the epitopes involved in toxin neutralization is important for understanding of the intoxication mechanisms and also for production of more effective neutralizing monoclonal antibody (MAb). In the present study, we report production and characterization of four TeNT-specific murine MAbs. Our results indicate that MAbs directed against the HC, but not the LC, of TeNT could have toxin-neutralizing activity in an in vitro assay. Given the fact that the L chain possesses the toxin activity, anti-fragment C antibodies are likely inducing their neutralization effect through a blocking of the toxin binding and subsequent entry into target cells.
Materials and methods
Toxin and toxoid production
The Harvard A47 strain of Clostridium tetani (Razi Vaccine and Serum Research Institute, Karaj, Iran) was used for toxin production in a modified Mueller and Miller medium (HiMedia Laboratories, Mumbai, India). Secreted toxin was purified using gel filtration chromatography and converted to toxoid by incubation of purified TeNT with 0.2–1% (v/v) formaldehyde for 42 days at a pH of 6.0–8.0 and a temperature of 4 °C. This toxoid was used to immunize mice and coat microtiter plates for detection of each MAb by ELISA.
Immunization, fusion, and culture conditions
Female BALB/c mice (6–8 weeks-of-age) were given an intraperitoneal injection of tetanus toxoid (40 µg) emulsified in complete Freund’s adjuvant (CFA) (Sigma, St Louis, MO). Each mouse received a total of three additional booster injections of 10 µg (at 3-week intervals) tetanus toxoid dissolved in incomplete Freund’s adjuvant (IFA; Sigma). The immunized mice were then screened for serum TeNT antibodies by an indirect ELISA. Hyper-immunized mice were then injected intravenously with 10 µg toxoid. After 3 days, splenocytes were harvested and fused with the mouse myeloma cell line SP2/0 (National Cell Bank of Iran, Tehran, Iran). Splenocytes were mixed at a 4:1 ratio with SP2/0 cells in the presence of polyethylene glycol (PEG) 1500 (Sigma). Fused cells were grown in hypoxanthine/aminopterin/thymidine (HAT) selective medium (Sigma) and cloned by limiting dilution assay. Supernatants of growing cells were then screened via an indirect ELISA, and all positive clones were further sub-cloned.
Screening of monoclonal antibodies by indirect ELISA
Microtiter polystyrene plates (Maxisorp, Nunc, Roskilde, Denmark) were coated with solutions of 10 µg/ml of tetanus toxoid, toxin, fragment C (Sigma), and light chain (List Biological Labs, Brea, CA)—each in phosphate-buffered saline (PBS, pH 7.2)—and incubated overnight at 4 °C. After four washes with PBS containing 0.05% Tween 20 (Sigma) (PBS-T) (each time taking 3 min), the plate was blocked using blocking buffer (PBS-T containing 3% non-fat skim milk) at 37 °C for 1.5 h. The supernatants of the hybridomas (100 µl/well) were then added at 37 °C for 1.5 h. Immunized mouse serum was used as positive control. Each well was then gently washed four times with PBS-T before addition of 100 µl of horseradish peroxidase (HRP)-conjugated sheep anti-mouse Ig (prepared in our laboratory) solution, and the plate incubated for a further 1.5 h at 37 °C. Following a washing to remove unbound detector antibody, each well received 100 µl of 3,3′,5,5′-tetramethylbenzidine (TMB) substrate solution (Sigma). After 5 min at room temperature (RT), the reaction was stopped by addition of 100 µl of stop solution (20% H2SO4) and the optical density (OD) of each sample was measured at 450 nm using a multiscan ELISA reader (Organon Teknika, Turnhout, Belgium). Samples yielding clear increases in OD (5-fold above background) were then selected for sub-cloning and further characterization.
Determination of isotype of monoclonal antibodies
The isotype of heavy and light chains of selected clones was determined using an IsoStrip mouse monoclonal antibody isotyping kit (Roche Diagnostics, Mannheim, Germany) according to manufacturer recommendations. The isotype of the positive clones was confirmed via ELISA, using sub-class-specific antibodies. Briefly, 100 µl of 0.5 µg/ml solutions of goat anti-mouse IgG1 or IgG2a (Sigma) in PBS were coated separately on wells of microtiter plates (Maxisorp, Nunc, Roskilde, Denmark) by overnight incubation at 4 °C. The plates were then washed (four times with PBS containing 0.05% Tween 20, each time taking 3 min) and blocked using blocking buffer for 1.5 h at 37 °C. After washing, supernatants of cultures of selected clones were added (100 µl) and the plates were incubated for 1.5 h at 37 °C. The washing step was repeated and 100 µl HRP-conjugated sheep anti-mouse Ig (prepared in our lab) solution was then added and the plate incubated for a further 1.5 h at 37 °C. Following a final washing step to remove unbound secondary antibody, the presence of the latter was revealed using TMB (as above) and the OD in each well was measured in a multi-scan ELISA reader at 450 nm.
Western blotting
Non-reducing SDS-polyacrylamide gel electrophoresis (SDS-PAGE) of tetanus toxoid was carried out in a 10% polyacrylamide gel. Thereafter, the electrophoretic transfer of proteins onto PVDF membrane (Roche Diagnostics) was accomplished using a Bio-Rad system (Life Science Research, Palo Alto, CA). After blocking the membrane with blocking buffer (PBS-T + 5% non-fat skim milk) overnight at 4 °C and then washing three times with PBS-T, primary antibodies (see above) in blocking buffer were added at 10 µg/ml and the membrane incubated with gentle rocking at RT for 1.5 h. The membrane was then gently washed before being incubated at RT for 1.5 h in an HRP-conjugated sheep anti-mouse Ig solution (prepared in our lab). After washing to remove unbound secondary antibody, each blot was developed using a chemiluminescence ECL detection kit (GE Healthcare Life Sciences, Uppsala, Sweden). The membrane was then visually analyzed to determine the presence of each ‘positive’ blot.
Affinity constant determination
The affinity constant (Ka) of each MAb was determined using an ELISA as described previously (Kazemi et al., Citation2011). Briefly, solutions containing 2000, 1000, 500, 250, 125 and 60 ng tetanus toxoid/ml were coated onto wells of a micotiter ELISA plate as outlined above. Serial concentrations of each MAb (from 30–2000 ng/ml) in blocking buffer were then added to each coated well and the plate incubated at 37 °C for 1.5 h. After a gentle washing, all wells received 100 µl HRP-conjugated sheep anti-mouse Ig and the plate was incubated for a further 1.5 h at 37 °C. Following a final washing step to remove unbound secondary antibody, the presence of the latter was revealed using TMB (as above) and the OD in each well was measured in a multiscan ELISA reader at 450 nm. Sigmoidal curves generated in plots of the OD values vs the logarithm of antibody concentrations were then drawn. The antibody concentration resulting in 50% of the maximum absorbance value ([Ab]t) at a particular antigen-coating concentration was selected for the affinity calculation using Ka = 1/2(2[Ab′]t − [Ab]t). [Ab′]t, where [Ab]t represents the antibody concentration resulting in 50% of the maximum absorbance value at two consecutive concentrations of coated antigen and where [Ag] = 2 [Ag′]. The final Ka value was accepted as the mean of such calculations for at least three antigen concentrations.
Assessment of epitope specificity of monoclonal antibodies by competition ELISA
ELISA plate wells were coated overnight with 100 µl of a solution of 10 µg tetanus toxoid/ml and then blocked with 3% skim milk-PBS/T 0.05%. Unconjugated MAb at final concentrations of 40, 20, 10, 5, 2.5, 1.25 or 0.612 µg/ml were added—together with 1 µg/ml of HRP-conjugated MAb (prepared in our lab)—and the plates incubated at 37 °C for 1.5 h. After four gentle washes with PBS-T, TMB substrate was added to each well and subsequently the OD (450 nm) in each well was measured. The percentage of inhibition for each MAb was calculated as: Percentage of inhibition = [(ODNI − ODWI)/ODNI] × 100, where ODNI is the OD in the absence of competitor MAb and ODWI is the OD in the presence of competitor MAb.
Cloning and sequencing of immunoglobulin heavy and light chains variable region genes
Total RNA from TeNT-specific clones was extracted using TRIZOL (Invitrogen, Grand Island, NY) according to the manufacturer instructions. First-strand cDNA was synthesized from 1 µg total RNA as described previously (Memarian et al., Citation2009). Two microliters of each cDNA was used to amplify mouse Ig VH and VL genes using specific degenerated primers:
5′-CAGGTSMARCTGCAGSAGTCWGG-3′as sense primer for VH;
5′-AGGGGCCAGTGGATAGA CAGATGG-3′ as antisense primer for VH;
5′-GAHRTTSWGNTSACYCAGWCTCCA-3′ as sense primer for Vκ; and
5′-TGGTGGGAAGATGGATACAG-3′ as antisense primer for Vκ.
PCR reactions were performed in 25 µl volumes using 1 Unit Taq DNA polymerase (Fermentas Life Sciences, St. Leon-Rot, Germany), 2.5 µl of 10 × PCR buffer, 2 µl of 25 mM MgCl2, 1.0 µl of dNTPs (10 mM) (Roche Applied Science, Indianapolis, IN) and 6 and 1 pmol of sense and anti-sense primers, respectively. PCR was performed in 40 cycles, initiated by 3 min at 94 °C followed by 90 °C for 20 s, 60 °C for 30 s, 72 °C for 30 s and 10 min at 72 °C for the final extension. PCR products were finally visualized by electrophoresis over 1% agarose gels containing ethidium bromide. Clonal PCR products were extracted using the GF-1 Nucleic Acid Extraction Kit (Vivantis, Selangor Darul Ehsan, Malaysia). Gel-purified PCR products were then cloned into a pGEM-T easy vector system Ι (Promega, Madison, WI) according to the manufacturer instructions. Sequencing of selected clones was performed from both directions using a BigDye Terminator Cycle Sequencing Reaction Kit (Applied Biosystems, Foster City, CA), and T7 and SP6 primers.
Tetanus toxin binding inhibition to ganglioside GT1b by monoclonal antibodies
The ability of each MAb to inhibit binding of TeNT to GT1b ganglioside was assessed by a modification of a procedure by Fitzsimmons et al. (Citation2000). Briefly, an appropriate concentration of TeNT required for saturation of ganglioside GT1b binding (20 µg/ml) was mixed with an equal volume of serial concentrations of anti-TeNT MAbs (beginning from 10 µg/ml) and the mixture then incubated for 2 h at RT. Microtiter ELISA plates were coated with GT1b (Sigma; 10 µg/ml in methanol, 100 µl/well), and the plates were left at RT overnight to allow for evaporation of the methanol vehicle. The plates were then blocked with 1% BSA (bovine serum albumin)/PBS solution for 2 h at RT. After four washes with PBS-T, 100 µl of pre-incubated TeNT/antibody mixture was added to each well and the plate was incubated for 2 h at RT. The plates were then washed four times with washing solution before addition of 100 µl of HRP-conjugated human anti-TeNT polyclonal Ab (produced in our lab) solution to each well, and the plate was then incubated for 2 h at RT. After a final washing, TMB substrate was added and the OD at 450 nm in each well assessed in the multi-scan plate-reader.
Results
Production of anti-TeNT MAbs
Fusion of splenocytes from hyper-immunized mouse with SP2/0 myeloma cells resulted in establishment of growing hybridoma cells. All growing hybridomas were initially screened based on reactivity to tetanus toxoid and toxin by an indirect ELISA. Finally, four stable hybridomas producing antibodies reacting with both tetanus toxoid and toxin were selected. All selected hybridomas were sub-cloned and stable clones producing TeNT-specific MAb were finally selected. The isotype of each MAb was found to be either IgG2a (three clones) or IgG2b (one clone) (). The affinity constant (Ka) of each MAb varied between 7.7 × 107 to 1.78 × 109 M−1.
Table 1. Determination of isotype and affinity constant of selected monoclonal antibodies.
Reactivity of test MAb to tetanus toxoid, toxin, fragment C, and light chain
To further characterize each anti-TeNT MAb, Western blotting and ELISA were done using tetanus toxoid, toxin, and various sub-fragments. Each MAb had a positive reactivity to tetanus toxoid when assessed by Western blotting (). Additionally, two MAbs (1F3E3 and 1F2C2) showed positive reactivity to fragment C, one MAb (1F3B3) reacted only with light chain, and one MAb (1F4E11) bound to both fragment C and light chain (as determined by ELISA) ().
Figure 1. Western blot reactivity profile of selected anti-tetanus toxin monoclonal anti-bodies. Tetanus toxoid was separated over a 10% polyacrylamide gel, electroblotted to PVDF membranes, and then probed with anti-TeNT MAb. SM, protein size marker.
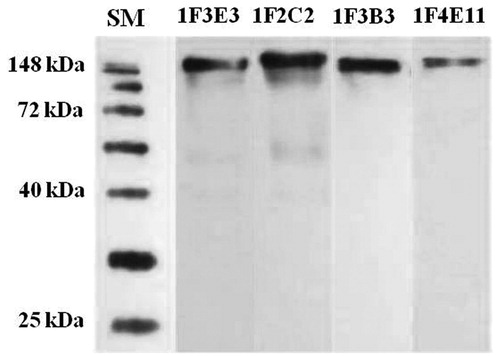
Table 2. Reactivity of specific monoclonal antibodies to tetanus toxoid, toxin, fragment C, and light chain.
Cross-inhibition of binding of monoclonal antibodies to tetanus toxoid
To investigate whether each MAb recognizes the same or different epitopes of tetanus toxoid, an antibody binding competition ELISA assay was performed. Each MAb was used as a detector (enzyme-conjugated) and competitor (unconjugated) in a multi-stage ELISA assay. Increasing concentrations of the competitor MAb (from 0.612–40 µg/ml) were used to inhibit binding of the detector MAb to tetanus toxoid. The results demonstrated that, apart from MAb 1F2C2 which partially inhibited (50% inhibition) the detector MAb 1F3E3 at the highest concentration (40 µg/ml) (), no cross-inhibition was observed for any other MAb (). No HRP-conjugated 1F3B3 MAb was available to assess the inhibitory effect of the other MAb on binding of this detector MAb to tetanus toxin.
Figure 2. Representative cross-inhibition profiles of tetanus toxin-specific monoclonal antibody. Increasing concentrations of competitor MAb were used to inhibit binding of the enzyme-conjugated detector MAbs 1F3E3 (a), 1F2C2 (b) and 1F4E11 (c) to tetanus toxin. The results show the percentage inhibition of the binding of detector MAb to tetanus toxoid.
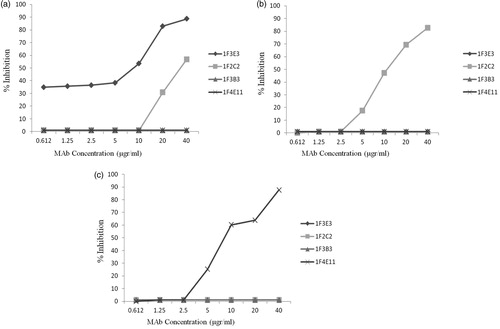
Immunoglobulin heavy and light chains variable region genes expressed by the hybridoma clones
Sequencing of the cDNA amplified from the TeNT-specific hybridoma clones revealed a rearrangement of distinct immunoglobulin heavy and light chain genes in the variable (VH and Vκ) and diversity (DH) segments within each hybridoma clone. However, the joining gene segments of heavy chain (JH) rearranged in three of the hybridomas (e.g. 1F3E3, 1F2C2, and 1F4E11) were derived from the same gene family (i.e. JH3*01) and two different Jκ segments were rearranged (IGKJ2*01 and IGKJ1*01), each in two hybridoma clones ().
Table 3. Immunoglobulin heavy and light chain variable region genes usage by the TeNT-specific hybridoma clones.
Inhibition of TeNT binding to ganglioside GT1b by monoclonal antibodies
The ability of each MAb to block TeNT binding to immobilized ganglioside GT1b was assessed by an indirect ELISA. As shown in , the 1F3E3 and 1F2C2 MAbs dose-dependently inhibited binding of TeNT to GT1b, whereas 1F3B3 and 1F4E11 did not block TeNT binding to its receptor.
Figure 3. Inhibition of tetanus toxin binding to ganglioside GT1b by monoclonal antibodies. An appropriate level of tetanus toxin in the presence or absence of increasing concentrations of MAb was allowed to bind to ganglioside GT1b. The percentage inhibition of TeNT biding was then calculated as described in the Materials and methods.
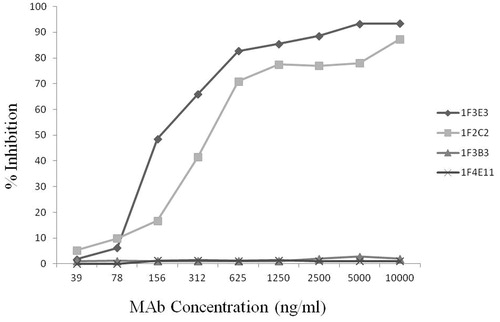
Discussion
Tetanus is an acute and a highly fatal infectious disease among all species of domestic animals. Humoral immunity is the main immune mechanism that provides total protection from tetanus. Active immunization is efficiently induced by tetanus toxoid and is crucial for the prevention of death caused by tetanus (Inić-Kanada et al., Citation2009). In spite of having a potent vaccine for tetanus, there have been too many cases involving deaths in developing countries, probably due to imperfect vaccination schedules. Additionally polyclonal Ig isolated from hyper-immunized donors has been efficiently used for passive immunotheraphy of tetanus. These preparations of polyclonal antibodies which are derived from human plasma have many inherent disadvantages including cost, the need to immunize donors, lot-to-lot heterogeneity, safety, and the adverse reactions associated with the administration of a relatively large amount of proteins (Chin et al., Citation2003). Monoclonal antibodies, however, offer an unlimited supply of a safe and homogeneous product with potential implication for therapy and analysis of the structure and function of tetanus toxin. There are several reports in the literature concerning production and characterization of human and mice monoclonal antibodies against tetanus toxin (Ahnert-Hilger et al., Citation1983; Boyd et al., Citation1984; Ichimori et al., Citation1985; Kenimer et al., Citation1983; Kozbor et al., Citation1982; Larrick et al., Citation1983; Matsuda et al., Citation1992; Sheppard et al., Citation1984; Volk et al., Citation1984; Ziegler-Heitbrock et al., Citation1986; Zurawski et al., Citation1978).
In the present study, we reported development of several new MAbs with different specificities by immunizing mice with tetanus toxoid. Characteristics of each MAb were explored in terms of isotype, specificity, affinity, VH and VL gene usage, and in vitro toxin neutralization activity. The data revealed that each MAb was reactive to tetanus toxin and toxoid in ELISA and Western blotting systems. The specificity of each MAb was further investigated using sub-fragments of the light and heavy chain of TeNT. Two MAbs (1F3E3 and 1F2C2) reacted with fragment C, while the other two MAbs recognized either LC alone (1F3B3) or both LC and fragment C (1F4E11). It seems that 1F4E11 recognizes a conformational epitope to which both LC and fragment C contribute to its expression. The different levels of reactivity of 1F4E11 with fragment C and LC implies that fragment C might contribute more to the formation of this conformational epitope. These findings, together with results obtained for sequencing of Ig VH and VL gene segments of the hybridoma clones and those of the cross-inhibition studies; clearly indicate individuality of the hybridoma clones and derivation of these MAbs from distinct unrelated clones. Indeed, various VH, Vκ, and DH gene segments were utilized by the four hybridoma clones, although the JH genes rearranged in three of the hybridomas were derived from the JH3*01 family member (). The MAb with toxin-neutralizing activity (i.e. 1F3E3 and 1F2C2) expressed different combinations of VH (IGHV1-9*01 and IGHV3-6*02, respectively) and VL genes (IGKV19-39*01 and IGKV6-17*01, respectively) that have not already been reported. This data suggests that multiple VH and VL gene rearrangements may contribute to TeNT neutralization.
The results of cross-inhibition studies showed that only one MAb (1F2C2) was able to partially inhibit binding of another MAb (1F3E3) to tetanus toxin. This partial inhibition that was achieved at the highest concentration of the inhibitor MAb (i.e. 40 µg/ml) suggests that these two MAbs recognize two distinct, but spatially or linearly close or overlapping epitopes located within fragment C of TeNT. Interestingly, both MAbs bound only to fragment C, but not LC of the toxin. It is notable that binding of 1F2C2 MAb to its epitope can partially inhibit binding of 1F3E3 to its epitope, but not vice-versa; this suggests to us a special topographical location of these two epitopes.
As previously mentioned, several reports have shown that gangliosides of the 1b series are implicated as the most important part of the clostridial neurotoxin receptor (Chen et al., Citation2009; Halpern et al., Citation1990; Kozaki et al., Citation1998; Nowakowski et al., Citation2002; Singh et al., Citation2000; Williamson et al., Citation1999). Accordingly, the GT1b toxin neutralization assay was employed in this study to assess the ability of our generated MAbs to inhibit toxin binding to its receptors. This method has been widely utilized by others (Fitzsimmons et al., Citation2000; Gustafsson et al., Citation1993; Holmgren, et al., Citation1980; Kenimer et al., Citation1983; Petrušić et al., Citation2011) as a suitable method to study the neutralizing function of anti-TeNT antibodies.
Our results showed that only the anti-fragment C antibodies (1F3E3 and 1F2C2) dose-dependently inhibited toxin binding to receptor, whereas the anti-LC antibody (1F3B3) or anti-light and fragment C antibody (1F4E11) failed to neutralize the toxin. Interestingly, these two MAbs displayed the highest binding affinity to the toxin. The affinity of these MAbs, however, does not seem to affect their neutralization potency, because they inhibited toxin binding to GT1b even at a very low concentration (i.e. 30 ng/ml), whereas the other two MAbs failed to neutralize the toxin even at the highest concentration employed in this study (i.e. 10 μg/ml).
Since fragment C plays a crucial role in binding of TeNT to its receptor, antibodies against this fragment are expected to be neutralizing, a phenomenon also noted elsewhere (Arunachalam et al., Citation1992; Fitzsimmons et al., Citation2000; Gustafsson et al., Citation1993; Simpson et al., Citation1990; Sheppard et al., Citation1984). Contradictory results have also been reported (Ahnert-Hilger et al., Citation1983; Gigliotti & Insel, Citation1982; Kamei et al., Citation1990; Lang et al., Citation1993; Matsuda et al., Citation1992) showing involvement of other parts of the toxin in receptor binding. For example, the cited Lang et al. study reported that the majority of neutralizing antibodies present in commercial polyclonal anti-TeNT antibodies were found to bind to fragment A (LC + N-terminal of HC). They also revealed that the protective capacity of all three tested commercial polyclonal anti-TeNT antibodies was completely abolished by adsorption with purified fragment A, but not fragment C. These results suggest that binding of antibodies to various parts of toxin, not necessarily fragment C, might contribute to TeNT neutralization. Such attachments may also indirectly affect the toxin binding through conformational changes in the structure of the toxin.
Conclusion
In conclusion, the studies here demonstrated that the fragment C-specific MAbs were able to neutralize TeNT in vitro. Each MAb expresses rearrangement of novel combinations of VH-DH-JH and Vκ-Jκ gene segments that have not already been reported. The results suggest that these MAbs are potentially suitable candidates for TeNT neutralization and immuno-prophylaxis of tetanus. In vivo analysis of the neutralization capacity of these MAbs in a murine model will extend our understandings about their functional activity and clinical implications.
Declaration of interest
The authors report no conflicts of interest. The authors alone are responsible for the content and writing of the paper.
Acknowledgments
This study was supported in part by a grant from the Avicenna Research Institute. The authors thank Mrs Neda Mohajer for her technical assistance.
References
- Ahnert-Hilger, G., Bizzini, B., Goretzki, K., et al. 1983. Monoclonal antibodies against tetanus toxin and toxoid. Med. Microbiol. Immunol. 172:123–135
- Arunachalam, B., Ghosh, S., Talwar, G., and Raghupathy, R. 1992. A single human monoclonal antibody that confers total protection from tetanus. Hybridoma 11:165–179
- Boyd, J., Hastings, I., Farzad, Z., et al. 1984. Experiences in the production of human monoclonal antibodies to tetanus toxoid. Dev. Bol. Standard. 57:93–98
- Chen, C., Fu, Z., Kim, J. P., et al. 2009. Gangliosides as high affinity receptors for tetanus neurotoxin. J. Biol. Chem. 284:26569–26577
- Chin, J., Sohn, Y., Lee, S. H., et al. 2003. Production of neutralizing human monoclonal antibody directed to tetanus toxin in CHO cell. Biologicals 31:45–53
- Demotz, S., Matricardi, P. M., Irle, C., et al. 1989. Processing of tetanus toxin by human antigen-presenting cells. Evidence for donor and epitope-specific processing pathways. J. Immunol. 143:3881–3886
- Di Tommaso, A., de Magistris, M. T., Bugnoli, M., et al. 1994. Formaldehyde treatment of proteins can constrain presentation to T-cells by limiting antigen processing. Infect. Immun. 62:1830–1834
- Fitzsimmons, S. P., Clark, K. C., Wilkerson, R., and Shapiro, M. A. 2000. Inhibition of tetanus toxin fragment C binding to ganglioside GT1b by monoclonal antibodies recognizing different epitopes. Vaccine 19:114–121
- Galazka, A., and Gasse, F. 1995. The present status of tetanus and tetanus vaccination. Curr. Topics Microbiol. Immunol. 195:31–53
- Gigliotti, F., and Insel, R. 1982. Protective human hybridoma antibody to tetanus toxin. J. Clin. Invest. 70:1306–1309
- Gustafsson, B., Whitmore, E., and Tiru, M. 1993. Neutralization of tetanus toxin by human monoclonal antibodies directed against tetanus toxin fragment C. Hybridoma 12:699–708
- Halpern, J. L., Habig, W., Neale, E., and Stibitz, S. 1990. Cloning and expression of functional fragment C of tetanus toxin. Infect. Immun. 58:1004–1009
- Herreros, J., Lalli, G., Montecucco, C., and Schiavo, G. 2000a. Tetanus toxin fragment C binds to a protein present in neuronal cell lines and motoneurons. J. Neurochem. 74:1941–1950
- Herreros, J., Lalli, G., and Schiavo, G. 2000b. C-terminal half of tetanus toxin fragment C is sufficient for neuronal binding and interaction with a putative protein receptor. Biochem. J. 347:199–204
- Holmgren, J., Elwing, H., Fredman, P., and Svennerholm, L. 1980. Polystyrene‐adsorbed gangliosides for investigation of the structure of the tetanus toxin receptor. Eur. J. Biochem. 106:371–379
- Ichimori, Y., Sasano, K., Itoh, H., et al. 1985. Establishment of hybridomas secreting human monoclonal antibodies against tetanus toxin and hepatitis B virus surface antigen. Bioch. Biophys. Res. Commun. 129:26–33
- Inić-Kanada, A. B., Stojanović, M. M., Živković, I. P., et al. 2009. The monoclonal antibody 26 raised against tetanus toxoid also recognizes tetanus toxin and β2-glycoprotein I-its binding properties in vitro and potential applications. J. Serb. Chem. Soc. 74:245–257
- Kamei, M., Hashizume, S., Sugimoto, N., et al. 1990. Establishment of stable mouse/human-human hybrid cell lines producing large amounts of anti-tetanus human monoclonal antibodies with high neutralizing activity. Eur. J. Epidemiol. 6:386–397
- Kazemi, T., Tahmasebi, F., Bayat A. A., et al. 2011. Characterization of novel murine mono-clonal antibodies directed against extracellular domain of human HER2 tyrosine kinase receptor. Hybridoma 30:347–353
- Kenimer, J. G., Habig, W. H., and Hardegree, M. C. 1983. Monoclonal antibodies as probes of tetanus toxin structure and function. Infect. Immun. 42:942–948
- Kozaki, S., Kamata, Y., Watarai, S., et al. 1998. Ganglioside GT1b as a complementary receptor component for Clostridium botulinum neurotoxins. Microb. Pathogen. 25:91–99
- Kozbor, D., Roder, J. C., Chang, T. H., et al. 1982. Human anti-tetanus toxoid monoclonal antibody secreted by EBV-transformed human B-cells fused with murine myeloma. Hybridoma 1:323–328
- Lalli, G., Herreros, J., Osborne, S. L., et al. 1999. Functional characterisation of tetanus and botulinum neurotoxins binding domains. J. Cell Sci. 112:2715–2724
- Lang, A., Cryz, S., Schurch, U., et al. 1993. Immunotherapy with human monoclonal antibodies. Fragment A specificity of polyclonal and monoclonal antibodies is crucial for full protection against tetanus toxin. J. Immunol. 151:466–472
- Larrick, J. W., Truitt, K. E., Raubitschek, A. A., et al. 1983. Characterization of human hybridomas secreting antibody to tetanus toxoid. Proc. Natl. Acad. Sci. USA 80:6376–6380
- Louch, H. A., Buczko, E. S., Mary, A., et al. 2002. Identification of a binding site for ganglioside on the receptor binding domain of tetanus toxin. Biochemistry 41:13644–13652
- Matsuda, M., Kamei, M., Sugimoto, N., et al. 1992. Characteristics of toxin-neutralization by anti-tetanus human monoclonal antibodies directed against the three functional domains [A],[B], and [C] of the tetanus toxin molecule and a reliable method for evaluating the protective effects of monoclonal antibodies. Eur. J. Epidemiol. 8:1–8
- Mayer, S., Laumer, M., Mackensen, A., et al. 2002. Analysis of the immune response against tetanus toxoid: Enumeration of specific T helper cells by the Elispot assay. Immunobiology 205:282–289
- Memarian, A., Hojjat-Farsangi, M., Asgarian-Omran, H., et al. 2009. Variation in WNT genes expression in different subtypes of chronic lymphocytic leukemia. Leukemia Lymphoma 50:2061–2070
- Montecucco, C., and Schiavo, G. 1994. Mechanism of action of tetanus and botulinum neurotoxins. Mol. Microbiol. 13:1–8
- Montecucco, C., and Schiavo, G. 1995. Structure and function of tetanus and botulinum neurotoxins. Quart. Rev. Biophys. 28:423–472
- Nowakowski, A., Wang, C., Powers, D., et al. 2002. Potent neutralization of botulinum neurotoxin by recombinant oligoclonal antibody. Proc. Natl. Acad. Sci. USA 99:11346–11350
- Petrušić, V., Živković, I., Stojanović, M., et al. 2011. Production, characterization and applications of a tetanus toxin specific monoclonal antibody T-62. Acta Histochem. 114:480–486
- Rawlings, N. D., and Barrett, A. J. 1995. Evolutionary families of metallopeptidases. Meth. Enzymol. 248:183–228
- Schiavo, G., Papini, E., Genna, G., and Montecucco, C. 1990. An intact interchain disulfide bond is required for the neurotoxicity of tetanus toxin. Infect. Immun. 58:4136–4141
- Schiavo, G., Rossetto, O., and Montecucco, C. 1994. Clostridial neurotoxins as tools to investigate molecular events of neurotransmitter release. Semin. Cell Biol. 5:221–229
- Schiavo, G. G., Benfenati, F., Poulain, B., et al. 1992. Tetanus and botulinum-B neurotoxins block neurotransmitter release by proteolytic cleavage of synaptobrevin. Nature 359:832–835
- Sheppard, A. J., Cussell, D., and Hughes, M. 1984. Production and characterization of monoclonal antibodies to tetanus toxin. Infect. Immun. 43:710–714
- Simpson, L. L., Lake, P., and Kozaki, S. 1990. Isolation and characterization of a novel human monoclonal antibody that neutralizes tetanus toxin. J. Pharmacol. Exp. Ther. 254:98–103
- Singh, A. K., Harrison, S. H., and Schoeniger, J. S. 2000. Gangliosides as receptors for biological toxins: Development of sensitive fluoroimmunoassays using ganglioside-bearing liposomes. Anal. Chem. 72:6019–6024
- Volk, W., Bizzini, B., Snyder, R., et al. 1984. Neutralization of tetanus toxin by distinct monoclonal antibodies binding to multiple epitopes on the toxin molecule. Infect. Immun. 45:604–609
- Williamson, L. C., Bateman, K. E., Clifford, J., and Neale, E. A. 1999. Neuronal sensitivity to tetanus toxin requires gangliosides. J. Biol. Chem. 274:25173–25180
- Ziegler-Heitbrock, H. W., Reiter, C., Trenkmann, J., et al. 1986. Protection of mice against tetanus toxin by combination of two human monoclonal antibodies recognizing distinct epitopes on the toxin molecule. Hybridoma 5:21–31
- Zurawski, V. R. Jr., Haber, E., and Black, P. H. 1978. Production of antibody to tetanus toxoid by continuous human lymphoblastoid cell lines. Science 199:1439–1441