Abstract
The adjuvanticity of aluminum hydroxide and calcium phosphate on the antibody response in mice towards the venom of the snake Bothrops asper was studied. It was found that, in vitro, most of the venom proteins are similarly adsorbed by both mineral salts, with the exception of some basic phospholipases A2, which are better adsorbed by calcium phosphate. After injection, the adjuvants promoted a slow release of the venom, as judged by the lack of acute toxicity when lethal doses of venom were administered to mice. Leukocyte recruitment induced by the venom was enhanced when it was adsorbed on both mineral salts; however, venom adsorbed on calcium phosphate induced a higher antibody response towards all tested HPLC fractions of the venom. On the other hand, co-precipitation of venom with calcium phosphate was the best strategy for increasing: (1) the capacity of the salt to couple venom proteins in vitro; (2) the venom ability to induce leukocyte recruitment; (3) phagocytosis by macrophages; and (4) a host antibody response. These findings suggest that the chemical nature is not the only one determining factor of the adjuvant activity of mineral salts.
Introduction
Adjuvants are widely used to formulate vaccines (Petrovsky & Aguilar, Citation2004), but also to immunize animals for the manufacture of hyperimmune sera. An example is the immunization of horses with snake venoms to induce the production of antibodies capable to bind venom toxins and neutralize their toxic effects (León et al., Citation2011). After immunization, these antibodies are purified and used to formulate snake anti-venoms (Gutiérrez et al., Citation2011). Up to now, adjuvants based on water-in-oil emulsions, such as Freund’s adjuvants, promote the highest antibody response towards snake venoms (Freitas et al., Citation1991; Sunthornandh and Ratanabanangkoon, Citation1994). However, the severity of the local reactions induced limits their use to the first stages of the immunization protocols. Subsequent boosters are normally administered using other adjuvants, such as mineral salts (Chotwiwatthanakun et al., Citation2001; Harrison et al., Citation2006).
The actual mechanism by which mineral salts increase the immune response is still not well understood (HogenEsch, Citation2002). It has been assumed that they work through a sustained stimulus produced by the slow release of the venom proteins adsorbed on their surface. However, there is evidence suggesting that neither adsorption nor depot formation are necessary for the immunopotentiation induced by aluminum hydroxide (Romero et al., Citation2007; Noe et al., Citation2010).
In order to study the role of adsorption and depot formation in the adjuvant activity of mineral salts, the antibody response towards Bothrops asper snake venom induced by adsorbed and co-precipitated immunogens was investigated, evaluating: (a) the coupling of venom proteins on mineral salts in vitro and at interstitial conditions in vivo; (b) the leukocyte recruitment; (c) the phagocytic activity of macrophages; and, (d) the enhancement of the antibody responses towards B. asper snake venom. Aluminum hydroxide and calcium phosphate were used as adjuvants.
Materials and methods
Venom
Bothrops asper venom was collected from specimens captured in the Pacific versant of Costa Rica and maintained in captivity at the serpentarium of Instituto Clodomiro Picado. Venom was stabilized by lyophilization and stored at −20 °C. Solutions of venom were prepared immediately before use.
Mice
CD-1 mice (male, 6–8-weeks-old) were obtained from the bioterium of the Instituto Clodomiro Picado (San Jose, Costa Rica). All mice were housed in a facility maintained at 21 °C with a 64% relative humidity and a 12-hour light/dark cycle. All mice had ad libitum access to standard rodent chow and filtered water. All procedures involving the use of animals used in this study were approved by the Institutional Committee for the Care and Use of Laboratory Animals (CICUA) of Universidad de Costa Rica and meet the International Guiding Principles for Biomedical Research Involving Animals (CIOMS, Citation1985).
Preparation of aluminum-adsorbed immunogen
Aluminum hydroxide was obtained from Americhem Pharmaceutical (batch 1267504, Miami, FL). Venom proteins were adsorbed on the adjuvant surface by mixing 5 ml of a venom solution (0.25 mg/ml in 0.12 M NaCl, 0.04 M phosphate buffer, pH 7.2 [PBS]) with 5 mL of a 3 mg/ml suspension of the salt in PBS.
Preparation of the calcium-adsorbed immunogen
A solution of 0.25 M calcium chloride/0.25 M EDTA/0.15 M potassium hydrogen phosphate underwent 8 h of electrolysis (144 mA/cm2) using platinum electrodes (initial pH of 7.21 and at 25 °C) (Montero et al., Citation2006). The precipitate was then washed eight times using distilled water. Adsorption of venom proteins on the nanoparticles surface was performed by mixing 5 ml of a suspension of precipitate in PBS (at 3 mg/ml) with 5 ml of venom (at 0.25 mg/ml) solution.
Preparation of calcium phosphate co-precipitated immunogen
Under slow stirring, 1.25 ml venom solution (1.0 mg/ml) was mixed with 5 ml of 0.125 M CaCl2. Afterwards, 5 ml of 0.125 M Na2HPO4 were added, the pH was adjusted to 7.0 with 0.156 M sodium citrate and the supernatant was separated by centrifugation. The pellet was suspended in 20 ml of 1.0% cellobiose and the mixture was incubated at 2–8 °C overnight (He et al., Citation2000).
Determination of capacity of mineral salts to couple venom proteins at in vitro conditions
Polystyrene plates (96-well, Corning Inc., Acton, MA) were coated with 100 µl of a solution containing 3 µg B. asper venom diluted in PBS, and left overnight at room temperature (RT). Then, plates were washed five times with water. In preparation for the assay, samples of venom coupled to mineral salts were centrifuged, and unbound proteins in the supernatants were separated by decantation and then serially diluted in PBS-2% casein. Thereafter, 50 µl of each dilution and 50 µl of a 1:1000 dilution of equine anti-venom (in PBS-2% casein; Instituto Clodomiro Picado) were added to each well and the plates were incubated at 25 °C for 1 h. Following another washing step, 100 µl anti-equine IgG conjugated with peroxidase (Sigma, St. Louis, MO; diluted 1:5000 in PBS-2% casein) was added to each well. Following an incubation at 25 °C for 1 h, the plates were washed and then each well received a 100 µl aliquot of o-phenylenediamine (2 mg OPD/ml; Sigma; in 6 mM H2O2/0.10 M sodium citrate buffer [pH 5.0]). The absorbance in each well was then assessed at 492 nm in a Dynatech MR5000 microplate reader (Denkendorf, Germany). The concentration of unbound venom was calculated using a calibration curve constructed by plotting the absorbance obtained for standard solutions as a function of venom concentration. The amount of venom coupled to adjuvants was calculated as the percentage of total venom used in preparation of the immunogen, corresponding to the difference between total and unbound venom. Results were expressed as a mean of triplicates (±SD).
Reverse-phase HPLC analysis of venom proteins coupled to adjuvants
In order to analyze which proteins bound to the adjuvants, samples of immunogens were centrifuged. After the unbound proteins in the supernatants were discarded by decantation, the pellets were dissolved in 300 µl 1.25 M EDTA. The proteins released from the immunogens were analyzed by reverse-phase HPLC, using an Agilent Technologies 1100 series HPLC system (Santa Clara, CA) equipped with a chromatographic data management system (ChemStation Data Analysis and Reporting, Agilent) and a Lichrosphere RP 100 C18 column (250 × 4 mm, 5 µm particle size). Fractions were eluted at 1 ml/min with a linear gradient of 0.1% trifluoroacetic acid (TFA) in water (Solution A) and acetonitrile containing 0.1% TFA (Solution B) (5% B for 10 min, followed by 5–15% B over 20 min, 15–45% B over 120 min, and 45–70% B over 20 min). Detection was set at 215 nm. Venom dissolved in PBS was used as control and fractions were identified as described previously (Alape-Girón et al., Citation2008).
Determination of capacity of mineral salts to keep venom proteins coupled at interstitial conditions in vivo
Groups of naïve mice (n = 10/group) were injected intraperitoneally (IP) with 0.5 ml of a preparation containing 5-LD50 of the venom coupled to adjuvants. Mortality was recorded over the subsequent 96 h. The proportion of surviving mice was related to the ability of mineral salts to keep the venom proteins coupled at interstitial conditions, thus reducing the acute toxicity resulting from the action of these toxins.
Evaluation of leukocyte recruitment
Dedicated groups of naïve mice (n = 4/group) were injected IP with 200 µl of a given immunogen preparation containing 50 µg of venom. Control groups were injected with venom dissolved in PBS or with PBS alone. After 24, 48, 72, and 96 h, groups of mice were euthanized by CO2 inhalation. At necropsy, 3 ml of 0.15 M NaCl was injected IP and the abdomen was gently massaged. The peritoneal fluid containing infiltrated leukocytes was collected by aspiration (Costa Rosa et al., Citation1994). The lavage was then centrifuged and the cell pellet re-suspended in 1 ml PBS; total cell counts were then determined in a Neubauer chamber.
Evaluation of phagocytic activity
A group of naïve mice (n = 4) were injected IP with 1.0 ml of 3% thioglycollate solution. After 96 h, the mice were euthanized by CO2 inhalation. At necropsy, 3 ml of 0.15 M NaCl was injected IP and the abdomen was gently massaged. Peritoneal fluid, containing elicited macrophages, was collected by aspiration, the lavage was centrifuged, and the cell pellet re-suspended in 1 ml PBS. Thereafter, 2.0 × 105 cells were placed on glass slides and allowed to adhere for 30 min at 37 °C in a 5% CO2 atmosphere. After washing with PBS, the slides containing a cell monolayer were placed in polystyrene plates (6-well, Corning) and coated with RPMI 1640 medium supplemented with 40 µg gentamicin sulfate/ml and 2 mM l-glutamine. Each dish/slide then received 60 µl of a given immunogen or PBS (control) and the cells were then incubated for 60 min at 37 °C. The slides were then washed and incubated for 40 min at 37 °C with zymosan particles (from yeast cell walls; Sigma) that had been suspended in PBS (at 3 mg/ml) and then sonicated for 15 min prior to use. The ratio of zymosan particles to macrophages was 1:10. Following the incubation, all unbound particles were removed by gentle washing with PBS. The slides were then washed, the macrophages were fixed with 250 µl 4% p-formaldehyde, and the extent of phagocytosis quantified by contrast phase microscopic observation. At least 200 macrophages were counted in each determination; cells containing ≥3 internalized particles were considered positive for phagocytosis. Results were presented as a percentage of cells positive for phagocytosis.
Immunization of animals
Dedicated groups of naïve mice (n = 10/group) were subcutaneously injected with 200 µl of adsorbed or a given co-precipitated immunogen containing 50 µg venom. An additional group injected with venom dissolved in PBS was used as control. Injections were repeated every 15 d over a period of 45 d (i.e. three immunizations). After the immunization program, mice were bled from the tail-vein using a heparinized microcapillary tube. After centrifugation, plasma in the sample was collected and stored at −20 °C for later analyses.
Evaluation of the antibody response towards several venom fractions
Wells of polystyrene ELISA plates (96-well, Corning) were coated overnight at RT with 100 µl of a solution containing 3 µg of B. asper venom, or HPLC fractions obtained by reverse phase HPLC (as described above) diluted in PBS. The plates were then gently washed with water before each well received 100 µl of a 1:500 dilution (in PBS-2% casein) of a sample of a pool of plasma prepared from all immunized mice. Samples were tested in triplicate. After incubation at 25 °C for 1 h, the plates were gently washed with water. Thereafter, 100 µl anti-murine IgG conjugated with peroxidase (diluted 1:5000 in PBS-2% casein; Sigma) was added to each well and the plates incubated for a further 1 h at 25 °C. The plates were then washed and each well then received a 100 µl aliquot of 2 mg OPD/ml (in 6 mM H2O2/0.10 M sodium citrate buffer [pH 5.0]). The absorbance value in each well was then recorded at 492 nm in the Dynatech microplate reader.
Statistical analyses
The statistical significance of any differences between mean values of the end-points assessed for each experimental group was determined by one-way ANOVA, followed by Tukey test. A p value < 0.05 was considered to be significant.
Results and discussion
Coupling of B. asper venom proteins on mineral salts in vitro
To determine the capacity of mineral salts to bind to different venom components, proteins adsorbed on the surface of precipitates were released by solubilization of salt particles with EDTA. The proteins were then separated by reverse-phase HPLC and identified by comparison with a chromatogram of a control venom sample ().
Figure 1. Chromatogram of (a) B. asper venom, (b) venom adsorbed on aluminum hydroxide, (c) venom adsorbed on calcium phosphate, or (d) venom co-precipitated with calcium phosphate. Separation was performed by reverse-phase HPLC using a Lichrosphere RP 100 C18 column (250 × 4 mm, 5 µm particle size). Fractions were eluted at 1 ml/min with a linear gradient (discontinuous line) of water and acetonitrile as outlined in the Materials and methods. Detection was set at 215 nm. Fractions were numbered according to Alape-Girón et al. (Citation2008).

Since aluminum hydroxide has an isoelectric point of 11 (Al-Shakhshir et al., Citation1995), it is positively charged at physiological pH. Therefore, it has a preference (but not a restriction) to adsorb acidic proteins (Rinella et al., Citation1996). On the other hand, calcium phosphate species have isoelectric points of 5.5–7.5 and, therefore, at physiological pH they are likely to have a preference (but not a restriction) to adsorb basic proteins (Jiang et al., Citation2004).
In contrast with these predictions based on electrostatic attractions, fraction 17–19 (which was mainly composed of the slightly-basic metalloproteinase BaP1), fraction 22–23 (which was mainly composed of acidic serine proteinase and L-amino acid oxidase), and fraction 26–30 (which was mainly composed of acidic PI and PIII metalloproteinases) (Angulo & Lomonte, Citation2009) were each similarly adsorbed by both mineral salts (). On the other hand, fraction 9–11 (which includes basic phospholipases A2 such as myotoxin II and IV) (Angulo & Lomonte, Citation2009) was adsorbed to aluminum hydroxide to a lower extent than by calcium phosphate (). This outcome was despite the lower capacity of calcium phosphate to adsorb venom proteins in vitro, when compared with aluminum hydroxide (p < 0.05; ).
Table 1. Capacity of mineral salts to couple venom proteins.
Co-precipitation with calcium phosphate was performed with the aim of increasing the amount of venom coupled to the adjuvant, by complementing the electrostatic adsorption with mechanical entrapment of proteins. As compared with adsorption alone, venom co-precipitation increases the in vitro coupling capacity of calcium phosphate (p < 0.05; ), without changing the proportions of venom fractions coupled to the mineral salt ().
Coupling of B. asper venom proteins on mineral salts at interstitial conditions in vivo
After intramuscular administration, displacement of adsorbed antigens from an adjuvant surface can be produced by salts and proteins present in the interstitial fluid (Rinella et al., Citation1995; Shi et al., 2002). Furthermore, dissolution of aluminum-containing adjuvants by α-hydroxy-carboxylic acids that are present in interstitial fluids accelerates the early release of antigens from the tissue depot. Therefore, it has been suggested that immunopotentiation induced by mineral salts depends neither on in vitro adsorption (HogenEsch, Citation2002; Iyer et al., Citation2003; Romero et al., Citation2007) nor on the in vivo antigen depot formation (Noe et al., Citation2010). However, since opposing evidence has been recently published (de Veer et al., Citation2010), the role of depot formation in adjuvanticity of mineral salts is still under discussion.
At interstitial conditions in vivo, a fast release of venom proteins coupled to mineral salts could be indirectly evidenced by observing signs of acute toxicity, i.e. death of the injected mice, when a supra-lethal dose of venom was injected. Therefore, groups of mice were injected with 5-LD50s of venom coupled to the adjuvant. According to previous results, 2-LD50s of the B. asper venom are enough to induce lethality in all mice injected by the intraperitoneal route (Solano et al., Citation2010); thus, in our experiment, a supralethal dose of venom was used. However, all mice injected with mixtures of 5-LD50s venom and mineral salts survived, without showing signs of envenomation. These results strongly suggest that both calcium phosphate and aluminum hydroxide were able to retard release of coupled venom proteins under in vivo conditions.
Leukocyte recruitment and phagocytosis
Inflammation induced by the venom of B. asper is characterized by edema and cellular infiltrate (Teixeiria et al., Citation2009). Our observations show that when venom is adsorbed on mineral salts, the number of recruited cells was significantly increased as compared when there was an injection of venom alone (). Differences observed between immunogens prepared by adsorption of venom on aluminum hydroxide and calcium phosphate were not significant. However, co-precipitation with calcium phosphate induced the maximum recruitment of leukocytes in the peritoneal cavity (p < 0.05).
Figure 2. Recruitment of leukocytes to the peritoneal cavity of mice injected with venom: dissolved in PBS (▾); adsorbed on aluminum hydroxide (•); adsorbed on calcium phosphate (▪); or co-precipitated with calcium phosphate (▴) (see Materials and methods for experimental details). Results are expressed as mean of quadruplicates (±SD). *Value significantly different from venom/PBS value (p < 0.05).
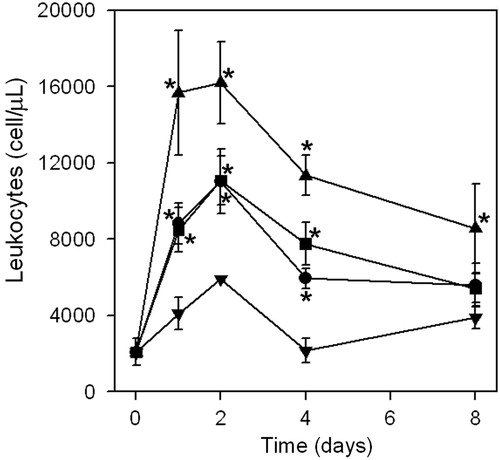
The internalization of antigens by phagocytosis is enhanced when antigens are adsorbed on aluminum-containing adjuvants (de Veer et al., Citation2010). According to our results, phagocytic activity of macrophages stimulated by venom dissolved in PBS was significantly increased by coupling of venom to mineral salts (), indicating that adjuvant activity of mineral salts influences not only leukocyte recruitment, but also activation of macrophages, which are key cells for antigen presentation.
Figure 3. Effects of adjuvants coupled to B. asper venom proteins on phagocytic activity of macrophages. Phagocytosis of zymosan particles was determined by phase-contrast microscopy (see Materials and methods for experimental details). Macrophages (harvested 96-h after intra-peritoneal injection of thioglycollate) were challenged with immunogen for 60 min before addition of zymosan. Bars correspond to experiments in which the venom was (a) dissolved in PBS, (b) co-precipitated with calcium phosphate, (c) adsorbed on calcium phosphate or (d) adsorbed on aluminum hydroxide. Values represent mean (±SE) from four mice. *Value significantly different from venom/PBS value (p < 0.05).
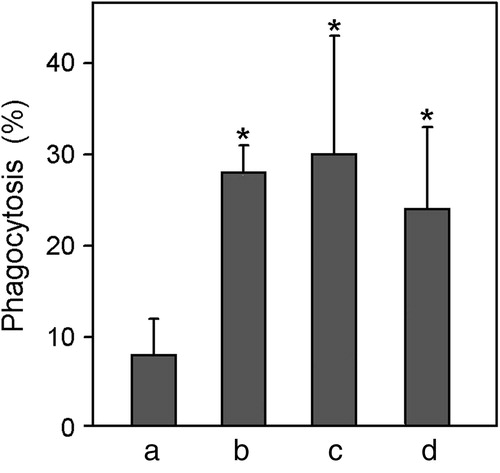
Enhancement of the antibody responses towards B. asper snake venom
When compared with the response induced by venom dissolved in PBS, both aluminum hydroxide and calcium phosphate significantly increase the antibody response towards all venom fractions (). Moreover, the highest enhancement of the antibody response towards fraction 9–11 is reached when calcium phosphate was used as adjuvant. This finding could be explained by the lower capacity of aluminum hydroxide to adsorb this fraction. However, since calcium phosphate induces a higher antibody response towards other fractions which were similarly adsorbed by both mineral salts, it could be that factors other than adsorption capacity determine the ability of mineral salts to increase the antibody response. On the other hand, co-precipitation of venom and calcium phosphate is the strategy that induces the highest antibody response towards all venom fractions (p < 0.05; ). This finding could be explained as a consequence of antigen coupling capacity ().
Figure 4. Antibody response towards fractions (a) 9–11, (b) 17–19, (c) 22–23, and (d) 26–30 of B. asper venom, induced by venom dissolved in PBS (▾), adsorbed on aluminum hydroxide (•), adsorbed on calcium phosphate (▪), or co-precipitated with calcium phosphate (▴). *Value significantly different from venom/PBS value (p < 0.05).

In conclusion, when the venom of the snake B. asper is used as antigen, immunogens prepared by adsorption on calcium phosphate have higher immunogenicity than those prepared by adsorption on aluminum hydroxide. In turn, when calcium phosphate is used as adjuvant, immunogens prepared by co-precipitation of venom and this salt have higher immunogenicity than those prepared by adsorption. The higher capacity of co-precipitated immunogen to induce leukocyte recruitment could be associated to adverse effects associated with inflammation at the injection site, especially if the immunogen is administered by the subcutaneous or intramuscular routes. All adjuvants tested in this work were capable of maintaining venom proteins adsorbed at interstitial conditions and forming antigen depots. These findings suggest that the adjuvant activity of mineral salt does not only depend on the chemical nature of the preparation.
Declaration of Interest
The authors report no conflicts of interest. The authors alone are responsible for the content and writing of the paper.
Acknowledgments
This study was supported by the International Foundation for Science (Project reference B/5043-1), the Vicerrectoría de Investigación, Universidad de Costa Rica (project 741-B2-501) and INCTTOX-CNPq/FAPESP (Proc. 08/57898-0), Brazil. Hidekel Olmedo was the recipient of a MSc student scholarship from the Deutscher Akademischer Austauschdienst (DAAD).
References
- Al-Shakhshir, R. H., Regnier, F. E., White, J. L., and Hem, S. L. 1995. Contribution of electrostatic and hydrophobic interactions to the adsorption of proteins by aluminum-containing adjuvants. Vaccine 13:41–44
- Alape-Girón, A., Sanz, L., Escolano, L., et al. 2008. Snake venomics of the lancead pitviper Bothrops asper: geographic, individual, and ontogenic variations. J. Proteome Res. 7:3556–3571
- Angulo, Y., and Lomonte, B. 2009. Biochemistry and toxicology of toxins purified from the venom of the snake Bothrops asper. Toxicon 54:949–957
- Chotwiwatthanakun, C., Pratanaphon, R., Akesowan, S., et al. 2001. Production of potent polyvalent anti-venom against three elapid venoms using a low dose, low volume, multi-site immunization protocol. Toxicon 39:1487–1494
- Costa Rosa, L. F., Safi, D. A., and Curi, R. 1994. Effect of thioglycollate and BCG stimuli on glucose and glutamine metabolism in rat macrophages. J. Leukocyte Biol. 56:10–14
- Council of International Organizations of Medical Sciences (CIOMS). 1985. The International Guiding Principles for Biomedical Research Involving Animals. CIOMS [online]. Available at: http://www.cioms.ch/images/stories/CIOMS/guidelines/1985_texts_of_guidelines.htm [last accessed 23 Feb 2012]
- de Veer, M., Kemp, J., Chatelier, J., et al. 2010. The kinetics of soluble and particulate antigen trafficking in the afferent lymph, and its modulation by aluminum-based adjuvant. Vaccine 14:6597–6602
- Freitas, T. V., Fortes-Dias, C. L., Diniz, C. R., et al. 1991. Immunization of horses with Crotalus durissus terrificus (South American rattlesnake) venom. A comparison of four different procedures. Braz. J. Med. Biol. Res. 24:281–290
- Gutiérrez, J. M., León, G., Lomonte, B., and Angulo, Y. 2011. Anti-venoms for snakebite envenomings. Inflamm. Allergy Drug Targets 10:369–380
- Harrison, R. A., Hasson, S. S., Harmsen, M., et al. 2006. Neutralization of venom-induced haemorrhage by IgG from camels and llamas immunized with viper venom and also by endogenous, non-IgG components in camelid sera. Toxicon 47:364–368
- He, Q., Mitchell, A. R., Johnson, S. L., et al. 2000. Calcium phosphate nanoparticle adjuvant. Clin. Diag. Lab. Immunol. 7:899–903
- HogenEsch, H. 2002. Mechanisms of stimulation of the immune response by aluminum adjuvants. Vaccine 20:S34–39
- Iyer, S., HogenEsch, H., and Hem, S. L. 2003. Relationship between the degree of antigen adsorption to aluminum hydroxide adjuvant in interstitial fluid and antibody production. Vaccine 21:1219–1223
- Jiang, D., Premachandra, G. S., Johnston, C., and Hem, S. L. 2004. Structure and adsorption properties of commercial calcium phosphate adjuvant. Vaccine 23:693–698
- León, G., Sánchez, L., Hernández, A., et al. 2011. Immune response towards snake venoms. Inflamm. Allergy Drug Targets 10:381–398
- Montero, M. L., Sáenz, A., Rodríguez, J. G., et al. 2006. Electrochemical synthesis of nanosized hydroxyapatite. J. Mater. Sci. 41:2141–2144
- Noe, S. M., Green, M. A., HogenEsch, H., and Hem, S. L. 2010. Mechanism of immunopotentiation by aluminum-containing adjuvants elucidated by the relationship between antigen retention at the inoculation site and the immune response. Vaccine 28:3588–3594
- Petrovsky, N., and Aguilar, J. C., 2004. Vaccine adjuvants: current state and future trends. Immunol. Cell Biol. 82:488–496
- Rinella, J. V., White, J. L., and Hem, S. L. 1995. Effect of anions on model aluminum-adjuvant-containing vaccines. J. Colloid. Interf. Sci. 172:121–130
- Rinella, J. V., White, J. L., and Hem, S. L. 1996. Treatment of aluminum hydroxide adjuvant to optimize the adsorption of basic proteins. Vaccine 14:298–300
- Romero, I. Z., Shi, Y., HogenEsch, H., and Hem, S. L. 2007. Potentiation of the immune response to non-adsorbed antigens by aluminum-containing adjuvants. Vaccine 25:825–833
- Shi, Y., HogenEsch, H., and Hem, S. L. 2002. Change in the degree of adsorption of proteins by aluminum-containing adjuvants following exposure to interstitial fluid: freshly prepared and aged model vaccines. Vaccine 20:80–85
- Solano, G., Segura, A., Herrera, M., et al. 2010. Study of the design and analytical properties of the lethality neutralization assay used to estimate anti-venom potency against Bothrops asper snake venom. Biologicals 38:577–585
- Sunthornandh, P., and Ratanabanangkoon, K. 1994. A comparative study of three vehicles on antibody responses against elapid snake neurotoxin immunogens. Toxicon 32:561–571
- Teixeiria, C., Cury, Y., Moreira, V., et al. 2009. Inflammation induced by Bothrops asper venom. Toxicon 54:988–997