Abstract
Aflatoxin B1 (AFB1) is a mycotoxin produced by Aspergillus spp. that can occur as a natural contaminant in foods and feeds of vegetable origin. Post-ingestion, AFB1 can be metabolized in the liver of mammals into hydroxylated aflatoxin M1 (AFM1) that is excreted with milk. Although several studies have been carried out to evaluate effects of AFB1 on the immune system, studies regarding AFM1 are moreover lacking. The aim of the current study was to investigate effects of AFB1 and AFM1 on immune function using a lymphoblastoid Jurkat T-cell line as an experimental model. Both AFB1 and AFM1 produced significant decreases in Jurkat cell proliferation, whereas only minor effects were noted on interleukin (IL)-2 and interferon (IFN)-γ cytokines mRNA expression in stimulated cells that had been pre-incubated with AFB1 and AFM1. Particularly, AFB1, but not AFM1, at the highest concentration (50 µM) induced a marked increase in IL-8 mRNA expression. The results of the current study suggested the existence of a concentration threshold for AFB1 and AFM1 needed to exert biological activity on cell viability and innate immunity.
Introduction
Aflatoxins are food contaminants of natural origin produced by secondary metabolism of some strains of molds belonging to Aspergillus genus such as A. flavus and A. parasiticus. Humans and animals are exposed to aflatoxins primarily through the ingestion of contaminated food, although inhalation could be also possible, particularly due to dusts of contaminated grains (Viegas et al., Citation2012). Aflatoxins are found in many foods and feeds, particularly in products of vegetable origin such as cereal grains (Wang and Liu, Citation2007); nevertheless, products of animal origin could be contaminated by some metabolites. Aflatoxins M1 (AFM1) and M2 (AFM2) are hydroxylated metabolites of aflatoxins B1 (AFB1) and B2 (AFB2), respectively, which are produced mainly in the liver of both humans and animals fed with contaminated products (Gallagher et al., Citation1994). AFM1 can be produced in mammary epithelial cells too (Caruso et al., Citation2009). AFM1 and AFM2 are secreted in the milk of several mammals including humans exposed through contaminated food; these toxic compounds link to caseins and are thermostable in dairy products (Prandini et al., Citation2009).
Likely owing to their established toxic effects in humans or pro-carcinogenic activity in susceptible laboratory animals, aflatoxins are the most intensively investigated mycotoxins. A number of epidemiological studies have linked the aflatoxin-contaminated food consumption with liver cancer (Hamid et al., Citation2013), hepatic failure resulting in death (Asim et al., Citation2011), and impaired child growth (Egal et al., Citation2005). Among the many aflatoxins, AFB1 is the predominant form exhibiting the highest toxicity and most potent hepatocarcinogenicity; for these reasons the International Agency for Research on Cancer classified AFB1 as a carcinogen (IARC, Citation1993).
Although several studies have been performed both in vitro and in vivo in order to evaluate the immunomodulatory effects of AFB1, literature data concerning the immunotoxicity of AFM1 are moreover lacking. AFB1 has been demonstrated to exert immune-toxic effects on cell-mediated immunity by reducing the number of circulating lymphocytes (Bondy and Pestka, Citation2000), or by alteration of NK cells activity and cytokine expression (Methenitou et al., Citation2001). AFB1 affects immune functions and also inhibited lymphocyte blastogenesis in several animal species (Meissonier et al., Citation2008; Ul-Hassan et al., Citation2012). Silvotti et al. (Citation1997) showed a reduction in the lymphoproliferative response, a reduction of macrophage activity linked to a reduced NO production and chemotaxis in suckling piglets by sow fed with feed naturally contaminated by AFB1. Moreover, AFB1 was also reported to alter macrophage function by inhibiting NO production in murine macrophages (Bianco et al., Citation2012b), phagocytosis in alveolar macrophages of pigs (Liu et al., Citation2002), and antigen presentation to lymphocytes (Meissonier et al., Citation2008). Cusumano et al. (Citation1995) showed that AFM1 altered the activity of Kupffer cells and inhibited phagocytosis in liver tissue, although this effect was 2–10 times lower than that induced by AFB1. Lastly, both AFB1 and AFM1 were shown to alter the ability of murine bone marrow cells to form myeloerythroid colonies (Roda et al., Citation2010).
To gain a better understanding of the potential immunotoxicity that might arise from exposure to AFM1, the current study was aimed to the evaluation of the effects of AFB1 and its metabolite AFM1 in a human lymphoblastoid Jurkat T-cell immune-competent model.
Materials and methods
Reagents
All tissue culture products, i.e. RPMI 1640 medium, L-glutamine, streptomycin, penicillin, fetal bovine serum (FBS), phosphate-buffered saline (PBS, pH 7.4), and non-essential amino acids were purchased from Cambrex Bioproducts Europe (Verviers, Belgium). Aflatoxins, lipopolysaccharide (LPS), reagents for LDH assays, phorbol myristate acetate (PMA), ionomycin, and antibiotics were purchased from Sigma (St. Louis, MO). Caspase-3 substrate, acetyl Asp-Glu-Val-Asp 7-amido-4-methylcoumarin (Ac-DEVD-AMC), and 7-amino-4-methylcoumarin (AMC) were purchased from Calbiochem (San Diego, CA). TRIZOL and all reagents used for reverse transcription were purchased from Invitrogen Ltd. (Paisley, UK), whereas reagents for real-time PCR were purchased from BioRad (Hercules, CA).
Cell cultures
Human lymphoblastoid Jurkat T-cells (ATCC, Manassas, VA) were grown at 37 °C in a humidified atmosphere consisting of 5% CO2/95% air in RPMI 1640 medium supplemented with 10% heat-inactivated FBS, 2 mM L-glutamine, 100 U penicillin/ml, 100 µg streptomycin/ml, and 1% (w/v) non-essential amino acids. Cells were maintained in exponential growth phase by passages at 2–3 days intervals.
Cell treatments
In each protocol outlined below, various numbers (protocol-specific) of Jurkat cells were exposed to varying concentrations of AFB1 or AFM1 (3–50 µM) for 24, 48, or 72 h, unless specified otherwise. We used this dose range here (and the other assays below) with the understanding that humans and animals could be exposed to levels of AFB1 or AFM1 as low as a few nM. However, our unpublished results using Jurkat cells and murine J774A. 1 macrophages incubated with such low levels of these agents (including the European maximum acceptable level for AFB1 and AFM1, ≈ 0.3 nM) did not show any effects on cell viability, apoptosis, or immune mediator formation. Thus, the concentrations here were chosen to reflect critical situations where there might arise an acute toxicity due to exposure, as might occur as a result of accidental exposures or due to use/sales of contaminated animal by-products (meats, dairy, etc.), as has been known to occur in areas of the world where there is less regulatory oversight (Lye et al., Citation1995; Probst et al., Citation2007; Bruchim et al., Citation2012). These doses were also chosen on the basis of data in the literature (Moon et al., Citation1999; Han et al., Citation2001; Caruso et al., Citation2009; Bianco et al., Citation2012b).
Cell proliferation assay
Jurkat cells (2 × 104/well) were cultured in flat-bottom 96-well plates and exposed to AFB1 or AFM1 (3–50 µM) for 24, 48 or 72 h. Eighteen hours prior to harvesting, cells were pulsed with 1 µCi/well [3H]-thymidine (Amersham Biosciences, Piscataway, NJ). Cultures were then harvested onto filters using a semi-automatic cell harvester (Filtermate, Packard, Danvers, MA). [3H]-Thymidine incorporation was assessed using a Top-count NXTTM microplate liquid scintillator (Packard). Results were expressed as cpm (counts per minute).
Caspase assay
Caspase-3 activity was measured by determining release of 7-amino-4-methylcoumarin (AMC) due to enzymatic hydrolysis of acetyl Asp-Glu-Val-Asp 7-amido-4-methylcoumarin (Ac-DEVD-AMC). Cells (6 × 105/well) were incubated with AFB1 or AFM1 (3–50 µM) for 24, 48, or 72 h in 96-well plates. At the end of each period, the cells were removed from culture plates and placed into tubes and then centrifuged. Subsequently, they were washed with PBS and re-suspended in 100 µl lysis buffer (50 mM HEPES [pH 7.4], 5 mM CHAPS, 5 mM DTT) for 20 min at 4 °C. Lysates were centrifuged (10,000 x g, 5 min, 4 °C) and aliquots of the supernatant incubated with reaction buffer (lysis buffer supplemented with 2 mM EDTA and 50 µM Ac-DEVD-AMC) for 60 min at 37 °C. Fluorescence data (360EX/460EM) were recorded in a Benchmark microplate reader (BioRad) and used to calculate the concentration of released AMC by means of a calibration curve generated in parallel with pure standard. Caspase-3 activity was ultimately expressed as nmol AMC/mg protein/h. Cells treated with 10 µM actinomycin D were used as a positive control.
Cytotoxicity assay
Lactate dehydrogenase (LDH) release was measured as an in vitro marker for cellular toxicity (Tipton et al., Citation2003). Cells (2 × 105/well) were incubated in 48-well plates with AFB1 or AFM1 (3–50 µM) for 24, 48, or 72 h. At the end of each period, the cells were removed from culture plates and placed into tubes. After incubation for 20 min at 4 °C, the cell suspensions were centrifuged (4000 x g, 5 min, 4 °C) and the supernatants recovered. The cell pellets were then lysed in 100 µl of 0.2 M Tris/HCl [pH 8.0] containing 1% (w/v) Triton X-100 for 30 min at 4 °C. Lysates and supernatant aliquots (100 µl) were then separately incubated for 30 min at 37 °C with 100 µl reaction buffer (0.7 mM p-iodonitrotetrazolium violet, 50 mM L-lactic acid, 0.3 mM phenazine methosulfate (PMS), 0.4 mM NAD, 0.2 M Tris/HCl [pH 8.0]). Absorbance was read at 490 nm in a Sunrise spectrometer (Tecan Group Ltd, Männedorf, Switzerland) and results expressed as a percentage of total LDH release from control cultures treated with 1% (w/v) Triton X-100 and calculated as: [(experimental value – blank value)/(total lysis – blank value) × 100].
Real-time PCR analysis of cytokine transcript levels
The mRNA expression of select cytokines in the Jurkat cell line was assessed by real-time PCR. Briefly, cells (2 × 106/well) were cultured in a 24-well plate and incubated for 24 h with different concentrations of AFB1 and AFM1 (3–50 µM), in the presence/absence of 1 µg PMA/L and 0.5 µM ionomycin. At the end of the incubation period, total RNA was isolated from cells using TRIZOL reagent according to the manufacturer protocols. cDNA was prepared from 1 µg total RNA by reverse transcribing with MMLV Reverse Transcriptase (Invitrogen) and oligo-(dT)12-18 at 42 °C for 60 min. Real-time quantitative PCR was then performed and analyzed using an iCycler iQTM Real-Time Detection System (BioRad). PCR reactions were carried out in a 96-well plate with 25 µl volume/well and each sample analyzed in triplicate. The reaction mixture contained 1X iQTM SYBR Green Supermix (BioRad), 0.2 μM each primer, and different aliquots of template DNA. The thermo-amplification program consisted of an initial denaturation (5 min at 94 °C), followed by 40 cycles of 30 s denaturation (94 °C), 30 s annealing at 56.4 °C (L-32 and IFNγ) or 58.4 °C (IL-2 and IL-8), and 40 s elongation at 72 °C. Negative controls were performed by omitting RNA from the cDNA synthesis and specific PCR amplification. The oligonucleotides used for amplification and size of the PCR products are described in . The housekeeping gene L-32 was used as an endogenous control. Relative gene expression levels were calculated using the 2−ΔΔCt method (Livak and Schmittgen, Citation2001), and presented as fold-change in gene expression, after normalization to the reference gene and relative to the control group (untreated cells).
Table 1. Primer sequences.
Statistical analysis
Results were expressed as mean ± SD of at least three independent experiments. Differences among the various treatment groups were determined by one-way analysis of variance (ANOVA). Multiple comparisons of treatment means were made using a Tukey test. The criterion for significance was *p < 0:05, **p < 0:01, ***p < 0.001.
Results
Effect of AFB1 and AFM1 on Jurkat T-cell proliferation
To investigate the ability of AFB1 and AFM1 to affect cell proliferation, [3H]-thymidine incorporation was used as a DNA synthesis indicator. Exposure of Jurkat cells to increasing concentrations of AFB1 and AFM1 (3–50 µM) for 24 h caused a significant concentration-dependent inhibitory effect on cell proliferation (); decreases became significant and dose-dependent starting from 15 µM for both mycotoxins. No effect was reported by incubation of cells with solvent alone. The time-dependent proliferation assays (48 and 72 h) did not reveal any significant differences with respect to the 24 h outcomes (data not shown).
Figure 1. Effects of AFB1 and AFM1 on Jurkat cell proliferation. Jurkat T-cells were incubated in the presence of increasing concentrations (3–50 μM) of AFB1 (black bar) or AFM1 (grey bar) for 24 h. Eighteen hours prior to harvesting, cells were pulsed with 1 µCi/well [3H]-thymidine. DNA synthesis was then measured by [3H]-thymidine incorporation and expressed as counts per minute (cpm). Control (ctr): cells incubated with medium containing ethanol (0.1%) only. Each value represents the mean ± SD of three independent experiments. *p < 0.05; **p < 0.01; ***p < 0.001 versus ctr.
![Figure 1. Effects of AFB1 and AFM1 on Jurkat cell proliferation. Jurkat T-cells were incubated in the presence of increasing concentrations (3–50 μM) of AFB1 (black bar) or AFM1 (grey bar) for 24 h. Eighteen hours prior to harvesting, cells were pulsed with 1 µCi/well [3H]-thymidine. DNA synthesis was then measured by [3H]-thymidine incorporation and expressed as counts per minute (cpm). Control (ctr): cells incubated with medium containing ethanol (0.1%) only. Each value represents the mean ± SD of three independent experiments. *p < 0.05; **p < 0.01; ***p < 0.001 versus ctr.](/cms/asset/a99cf1f8-a9e7-45f3-b9c7-79d7c192e326/iimt_a_848250_f0001_b.jpg)
Effect of AFB1 and AFM1 on caspase-3 activity and LDH release
Caspase-3 and LDH released into the culture medium were determined to evaluate the involvement of cell apoptosis and necrosis, respectively, in the observed declines in proliferation induced by AFB1 and AFM1. Exposure of Jurkat cells to both aflatoxins was unable to modify caspase-3 activity (). Results of the LDH analyses showed a weak increase at any of the tested concentrations, although the changes were not significant versus control values ().
Figure 2. Effects of AFB1 and AFM1 on Jurkat cell caspase-3 activity and LDH release. (a) Each end-point was analyzed following exposure to AFB1 (black bar) or AFM1 (grey bar) for 24 h. Control (ctr) cells were incubated with medium containing ethanol (0.1%) only. In (a), cells treated with 10 μM actinomycin D (ActD) served as positive control. Values were ultimately expressed as nmole AMC/mg protein in cell lysate. In (b), cells treated with 1% NP-40 served as positive control. Amount of LDH released into medium was expressed as a percentage of LDH released from positive control. All values represent mean ± SD of three independent experiments. ***p < 0.001 versus ctr.
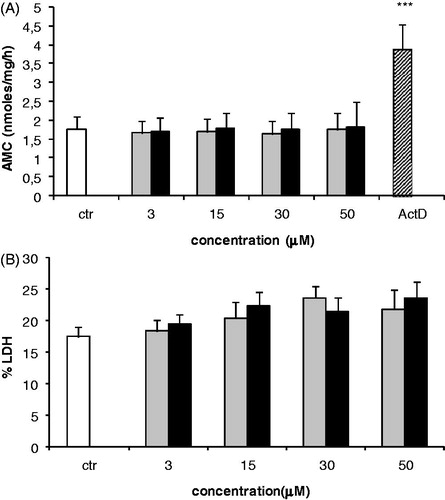
Effects of AFB1 and AFM1 on the Jurkat T-cell cytokine mRNA expression
Modulation on cytokine expression due to the test aflatoxins was evaluated in the Jurkat cells using real-time PCR. As previously reported, cytokine mRNA expression in Jurkat cells was detected only following stimulation with PMA/ionomycin (). In such cells, pre-incubation with AFB1 and AFM1 (3–50 µM) did not significantly change mRNA levels of the TH1-like cytokines IFNγ () and IL-2 () in comparison to values measured with positive control cells. In contrast, IL-8 mRNA was significantly induced, but only at the highest concentration of AFB1 (50 µM) tested and not by AFM1 (). Incubation of the mycotoxins with un-stimulated cells (control) did not elicit any transcript over the entire concentration range tested (data not shown).
Figure 3. Effects of AFB1 and AFM1 on the Jurkat T cell cytokine expression. mRNA levels of select cytokines in Jurkat cells following 24-h incubation with PMA/ionomycin concurrent with increasing concentrations (3–50 μM) of AFB1 (black bar) or AFM1 (grey bar). Control (ctr) was unstimulated cells; the positive control (ctr+) was cells incubated with PMA/ionomycin. Unstimulated cells were incubated with medium containing ethanol (0.1%) only. mRNA levels of (a) IFNγ, (b) IL-2, (c) IL-8, and L-32 were subsequently assessed by real-time PCR analysis. Results represent mean ± SD of two independent experiments. ***p < 0.001 versus ctr+.
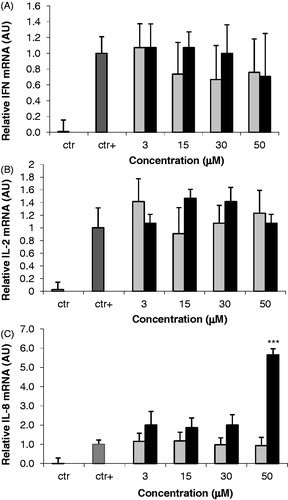
Discussion
Mycotoxins are known to affect cellular functions (Marzocco et al., Citation2009; Bianco et al., Citation2012a). In the current study, effects of AFB1 and its metabolite AFM1 on immune function were analyzed by means of an in vitro assessment of their effects on the human lymphoblastoid Jurkat T-cell line. Jurkat cells have long been used as a human T-cell model, and have been particularly useful for assessing IL-2 T-cell activation, cytokine production in response to different contaminants (Colombo et al., Citation2004; Ndebele et al., Citation2004; Oostingh et al., Citation2009), or for characterization of cell surface markers (Zhao et al., Citation2013). In our previous studies (Luongo et al., Citation2006; Severino et al., Citation2006), Jurkat cells were utilized to evaluate effects of different Fusarium toxins, showing their usefulness as an in vitro model in that findings were in line with other literature data (Meky et al., Citation2001; Pestka et al., Citation2005; Rocha et al., Citation2005).
In the current study, we showed both AFB1 and AFM1 inhibited Jurkat cell proliferation in a dose-related manner; the effect was similar following 24, 48 or 72 h of exposure. Similarly, Bodine et al. (Citation1984) showed that bovine lymphocyte proliferative capability was inhibited by each aflatoxin. Further, lymphocytes from rabbits or pigs exposed to high doses of AFB1 displayed significantly decreased proliferative responses (Harvey et al., Citation1995; Dimitri and Gabal, Citation1996). An in vivo study by Ul-Hassan et al. (Citation2012) noted severe immunosuppressive effects in progeny chicks as a result of exposure of parent hens to an AFB1-contaminated diet; in particular, lymphoid tissues, immunoglobulin production, lymphoblastogenic responses, and macrophage function were affected. Such modulation was confirmed here; indeed, while AFB1 at the lowest examined concentration (3 µM) did not exert any significant effects, a significant dose-related inhibitory effect was noted starting at 15 µM. These types of results confirmed there was a concentration threshold for AFB1 to exert toxicologic activity.
Immunosuppressive effects of AFB1 were also reported in other cell types such as J774A.1 murine macrophages (Bianco et al., Citation2012b) and murine peritoneal macrophages (Moon et al., Citation1999). On the other hand, our results did not show any enhancement of either caspase-3 activity or LDH release into the culture medium, although a weak increase in LDH levels was noted. From this, we assume that the anti-proliferative effect seen here was not due to caspase-3 induction or a significant alteration in LDH homeostasis. Although no data to our knowledge are available about effects of AFB1 and AFM1 on cellular necrosis, our results are in accordance with other works showing that both AFB1 and AFM1 did not induce apoptosis in murine macrophages exposed for 24, 48, or 72 h (Bianco et al., Citation2012b).
We also evaluated effects of AFB1 and AFM1 on cytokine expression by Jurkat cells. These in particular focused on IFNγ (a pro-inflammatory cytokine), IL-2 (a lymphocyte growth factor), and IL-8 (involved in the early phase of the response to recruit neutrophils). The results in the present study clearly indicated an increased expression of IL-8 mRNA, but only at the highest concentration of AFB1. In contrast, gene expression for IFNγ and IL-2, cytokines that play major roles in adaptive responses in a host were not modified. These results are in agreement with a previous study showing that AFB1 was not able to modulate EL-4 thymoma cell (as T-cell model) IL-2 production (Marin et al., Citation1996). IL-8—a chemokine involved in innate immunity—has multiple functions including initiator of acute inflammatory responses, chemotactin for neutrophils (PMN), and inducer of basophil histamine and leukotriene release (Linevsky et al., Citation1997). As professional phagocytes, PMN constitute a prime part of the innate defense response against an extensive number of potential pathogens in the environment (Muller et al., Citation2009; Kumar and Sharma, Citation2010). Extensive damage can result from over-active IL-8-induced recruitment of PMN to specific tissues, thereby triggering activation of inflammatory responses (Braca et al., 2011). Indeed, recently it was shown that repeated AFB1 exposure promoted inflammatory responses by inducing changes in the regulation of cytokine expression in the treated rats (Quian et al., Citation2013). When taken together in the context of the data reported here, all of these studies provide novel insights into potential mechanisms by which AFB1- and potentially AFM1-exposure affects immunoreactivity and immunocompetence in an exposed host.
Conclusions
The results of the current study highlighted that aflatoxins could potentially impart adverse effects on innate immunity. In particular, this study showed that both AFB1 and AFM1 (a product of AFB1 detoxification) induced anti-proliferative effects in Jurkat T-cells. The results were confirmatory of our hypotheses that AFM1 was also likely to be an immunomodulant and that, while a minimal concentration threshold was needed for AFB1 to impair the immune response, i.e. by activating innate mechanisms (IL-8 expression), it was not likely to be sufficient to elicit changes in adaptive immunity. We plan in future studies to focus our attention on effects of mixtures of the mycotoxins since humans and animals are not likely to be exposed to either alone during ingestion of contaminated foods and feeds and, further, both forms are likely to co-exist in an exposed host once hepatic processes are activated to process the AFB1 itself. Lastly, we are keenly aware that our future studies should also examine the effects of these (and other critical mycotoxins) on the proliferative/cytokine formation activities of primary human peripheral blood mononuclear cells (PBMC) or isolated peripheral blood T-cells. Such studies would provide more clinically relevant and translatable immunotoxicity data. Ultimately, with that information and that of the current studies, more detailed biochemical and molecular mechanisms of the immunotoxic effects of these key mycotoxins will be able to be defined.
Declaration of interest
The authors declare that they have no direct financial relation with the commercial identities mentioned in the manuscript and there is not any conflict of interest.
References
- Asim, M., Sarma, M. P., Thayumanavan, L., and Kar, P. 2011. Role of aflatoxin B1 as a risk for primary liver cancer in north Indian population. Clin. Biochem. 44:1235–1240
- Bianco, G., Fontanella, B., Severino, L., et al. 2012a. Nivalenol and deoxynivalenol affect rat intestinal epithelial cells: A concentration-related study. PLOS One 7 e52051:1–14
- Bianco, G., Russo, R., Marzocco, S., et al. 2012b. Modulation of macrophage activity by aflatoxins B1 and B2 and their metabolites aflatoxins M1 and M2. Toxicon 59:644–650
- Bodine, A. B., Fischer, S. F., and Gangiee, S. 1984. Effects of aflatoxin B1 and major metabolites on PHA-stimulated lymphoblastogenesis of bovine lymphocytes. J. Dairy Sci. 67:110–114
- Bondy, G. S., and Pestka, J. J. 2000. Immunomodulation by fungal toxins. J. Toxicol. Environ. Health 3:109–143
- Braca, A., Dal Piaz, F., Marzocco, S., et al. 2011. Triterpene derivatives as inhibitors of protein involved in the inflammatory process: molecules interfering with phospholipase A2, cycloxygenase, and lipoxygenase. Curr Drug Targets 12:302--321
- Bruchim, Y., Segev, G., Sela, U., et al. 2012. Accidental fatal aflatoxicosis due to contaminated commercial diet in 50 dogs. Res. Vet. Sci. 93:279–287
- Caruso, M., Mariotti, A., Zizzadoro, C., et al. 2009. A clonal cell line (BME-UV1) as a possible model to study bovine mammary epithelial metabolism: Metabolism and cytotoxicity of aflatoxin B1. Toxicon 53:400–408
- Colombo, M., Hamelin, C., Kouassi, E., et al. 2004. Differential effects of mercury, lead, and cadmium on IL-2 production by Jurkat T-cells. Clin. Immunol. 111:311–322
- Cusumano, V., Costa, G. B., Trifiletti, R., et al. 1995. Functional impairment of rat Kupffer cells induced by aflatoxin B1 and its metabolites. FEMS Immunol. Med. Microbiol. 10:151–155
- Dimitri, R. A., and Gabal, M. A. 1996. Immunosuppressant activity of aflatoxin ingestion in rabbits measured by response to Mycobacterium bovis antigen I. Cell-mediated immune response measured by skin test reaction. Vet. Hum. Toxicol. 38:333–336
- Egal, S., Hounsa, A., Gong, Y. Y., et al. 2005. Dietary exposure to aflatoxin from maize and groundnut in young children from Benin and Togo, West Africa. Int. J. Food Microbiol. 104:215–224
- Gallagher, E. P., Wienkers, L. C., Kunze, K. L., et al. 1994. Role of CYP1A2 and 3A4 in the bioactivation of aflatoxin B1 in human liver microsomes. Cancer Res. 54:101–108
- Hamid, A. S., Tesfamariam, I. G., Zhang, Y., and Zhang, Z. G. 2013. Aflatoxin B1-induced hepatocellular carcinoma in developing countries: Geographical distribution, mechanism of action and prevention. Oncol. Lett. 5:1087–1092
- Han, S. H., Jeon, Y. J., Yea, S. S., and Yang, K. H. 2001. Suppression of IL-2 gene expression by aflatoxin B1 is mediated through down-regulation of the NF-AT and AP-1 transcription factors. Toxicol. Lett. 108:1–10
- Harvey, R. B., Edrington, T. S., Kubena, L. F., et al. 1995. Influence of aflatoxin and fumonisin B1-containing culture material on growing barrows. Am. J. Vet. Res. 56:1668–1672
- IARC Working Group on the Evaluation of Carcinogenic Risks to Humans International Agency for Research on Cancer. 1993. Some naturally occurring substances: food items and constituents, heterocyclic aromatic amines and mycotoxins. IARC monographs on the evaluation of carcinogenic risks to humans: v. 56. Geneva: World Health Organization, International Agency for Research on Cancer
- Kumar, V., and Sharma, A. 2010. Neutrophils: Cinderella of innate immune system. Int. Immunopharmacol. 10:1325–1334
- Linevsky, J. K., Pothoulakis, C., Keates, S., et al. 1997. IL-8 release and neutrophil activation by Clostridium difficile toxin-exposed human monocytes. Am. J. Physiol. 273:G1333–G1340
- Liu, B. H., Yu, F. Y., Chan, M. H., and Yang, Y. L. 2002. The effects of mycotoxins, fumonisin B1, and aflatoxin B1 on primary swine alveolar macrophages. Toxicol. Appl. Pharmacol. 180:197–204
- Livak, K. J., and Schmittgen, T. D. 2001. Analysis of relative gene expression data using real-time quantitative PCR and the 2−ΔΔCt method. Methods 25:402–408
- Luongo, D., Severino, L., Bergamo, P., et al. 2006. Interactive effects of fumonisin B1 and α-zearalenol on proliferation and cytokine expression in Jurkat T-cells. Toxicol. In Vitro 20:1403–1410
- Lye, M. S., Ghazali, A. A., Mohan, J., et al. 1995. An outbreak of acute hepatic encephalopathy due to severe aflatoxicosis in Malaysia. Am. J. Trop. Med. Hyg. 53:68–72
- Marin, M. L., Murtha, J., Dong, W., and Pestka, J. J. 1996. Effects of mycotoxins on cytokine production and proliferation in EL-4 thymoma cells. J. Toxicol. Environ. Health 48:379–396
- Marzocco, S., Russo, R., Bianco, G., et al. 2009. Pro-apoptotic effects of nivalenol and deoxynivalenol trichothecenes in J774A.1 murine macrophages. Toxicol. Lett. 89:21–26
- Meissonier, M., Pinton, P., Laffitte, J., et al. 2008. Immunotoxicity of aflatoxin B1: Impairment of the cell-mediated response to vaccine antigen and modulation of cytokine expression. Toxicol. Appl. Pharmacol. 231:142–149
- Meky, F. A., Hardie, L. J., Evans, S. W., and Wild, C. P. 2001. Deoxynivalenol-induced immunomodulation of human lymphocyte proliferation and cytokine production. Food Chem. Toxicol. 39:827–836
- Methenitou, G., Maravelias, C., Athanaselis, S., et al. 2001. Immuno-modulative effects of aflatoxins and selenium on human natural killer cells. Vet. Hum. Toxicol. 43:232–234
- Moon, E. Y., Rhee, D. K., and Pyo, S. 1999. In vitro suppressive effect of aflatoxin B1 on murine peritoneal macrophage functions. Toxicology 133:171–179
- Muller, I., Munder, M., Kropf, P., and Hansch, G.M. 2009. Polymorphonuclear neutrophils and T lymphocytes: Strange bedfellows or brothers in arms? Trends Immunol. 30:522–530
- Ndebele, K., Tchounwou, P. B., and McMurray, R. W. 2004. Coumestrol, bisphenol-A, DDT, and TCDD modulation of IL-2 expression in activated CD4+ Jurkat T-cells. Int. J. Environ. Res. Public. Health. 1:3–11
- Oostingh, G. J., Wichmann, G., Schmittner, M., et al. 2009. The cytotoxic effects of the chlorpyrifos and diazinon differ from their immunomodulating effects. J. Immunotoxicol. 6:136–145
- Pestka, J. J., Uzarski, R. L., and Islam, Z. 2005. Induction of apoptosis and cytokine production in Jurkat human T-cells by deoxynivalenol: Role of mitogen-activated protein kinases and comparison to other ketotrichothecenes. Toxicology 206:207–219
- Prandini, A., Tansini, G., Sigolo, S., et al. 2009. On the occurrence of aflatoxin M1 in milk and dairy products. Food Chem. Toxicol. 47:984–991
- Probst, C., Njapau, H., and Cotty, P. J. 2007. Outbreak of an acute aflatoxicosis in Kenya in 2004: Identification of the causal agent. Appl. Environ. Microbiol. 73:2762–2764
- Quian, G., Tang, L., Guo, X., et al. 2013. Aflatoxin B1 modulates expression of phenotypic markers and cytokines by splenic lymphocytes of male F344 rats. J. Appl. Toxicol. DOI 10.1002/jat.2866
- Rocha, O., Ansari, K., and Doohan. F. M. 2005. Effects of trichothecene mycotoxins on eukaryotic cells: A review. Food Addit. Contam. 22:369–378
- Roda, E., Coccini, T., Acerbi, D., et al. 2010. Comparative in vitro and in vivo myelotoxicity of aflatoxins B1 and M1 on hemopoietic progenitors (BFU-E, CFU-E and CFU-GM): Species-related susceptibility. Toxicol. In Vitro 24:217–223
- Severino, L., Luongo, D., Bergamo, P., et al. 2006. Mycotoxins nivalenol and deoxynivalenol differently modulate cytokine mRNA expression in Jurkat T-cells. Cytokine 36:75–82
- Silvotti, L., Patterino, C., Bonomi, A., and Cabassi, E. 1997. Immunotoxicological effects on piglets of feeding sows diets containing aflatoxins. Vet. Res. 141:469–472
- Tipton, D. A., Lyle, B., Babich, H., and Dabbous, M. K. 2003. In vitro cytotoxic and anti-inflammatory effects of myrrh oil on human gingival fibroblasts and epithelial cells. Toxicol. In Vitro 17:301–310
- Ul-Hassan, Z., Khan, M. Z., Khan, A., and Javed, I. 2012. Immunological status of the progeny of breeder hens kept on ochratoxin A (OTA)- and aflatoxin B1 (AFB1)-contaminated feeds. J. Immunotoxicol. 9:381–391
- Viegas, S., Veiga, L., Malta-Vacas, J., et al. 2012. Occupational exposure to aflatoxin (AFB) in poultry production. J. Toxicol. Environ. Health 75:1330–1340
- Wang, J., and Liu, X. M. 2007. Contamination of aflatoxins in different kinds of foods in China. Biomed. Environ. Sci. 20:483–487
- Zhao, M. F., Qu, X. J., Qu, J. L., et al. 2013. The role of e3 ubiquitin ligase cbl proteins in IL-2-induced Jurkat T-cell activation. BioMed Res. Int. 2013:430861