Abstract
Despite the transient immunosuppressive properties of local radiotherapy (RT), this classical treatment modality of solid tumors is capable of inducing immunostimulatory forms of tumor-cell death. The resulting ‘immunotoxicity’ in the tumor, but not in healthy tissues, may finally lead to immune-mediated destruction of the tumor. However, little is known about the best irradiation scheme in this setting. This study examines the immunological effects of differently irradiated human colorectal tumor cells on human monocyte-derived dendritic cells (DC). Human SW480 tumor cells were irradiated with a norm-fractionation scheme (5 × 2 Gy), a hypo-fractionated protocol (3 × 5 Gy), and with a high single irradiation dose (radiosurgery; 1 × 15 Gy). Subsequently, human immature DC (iDC) were co-incubated with supernatants (SN) of these differently treated tumor cells. Afterwards, DC were analyzed regarding the expression of maturation markers, the release of cytokines, and the potential to stimulate CD4+ T-cells. The co-incubation of iDC with SN of tumor cells exposed to norm- or hypo-fractionated RT resulted in a significantly increased secretion of the immune activating cytokines IL-12p70, IL-8, IL-6, and TNFα, compared to iDC co-incubated with SN of tumor cells that received a high single irradiation dose or were not irradiated. In addition, DC-maturation markers CD80, CD83, and CD25 were also exclusively elevated after co-incubation with the SN of fractionated irradiated tumor cells. Furthermore, the SN of tumor cells that were irradiated with norm- or hypo-fractionated RT triggered iDC to stimulate CD4+ T-cells not only in an allogenic, but also in an antigen-specific manner like mature DC. Collectively, these results demonstrate that norm- and hypo-fractionated RT induces a fast human colorectal tumor-cell death with immunogenic potential that can trigger DC maturation and activation in vitro. Such findings may contribute to the improvement of irradiation protocols for the most beneficial induction of anti-tumor immunity.
Introduction
Radiotherapy (RT) is an inherent part of modern oncology. Since irradiation causes multiple DNA damage followed by a stop of proliferation and finally tumor cell death, RT is mainly applied for local tumor control. However, recently also the immunostimulatory effects of RT have gained more and more attention and give hints to promising combinations with anti-tumor immunotherapies (Formenti & Demaria, Citation2009; Postow et al., Citation2012; Rubner et al., Citation2012).
The immunostimulatory potential of RT can basically be classified as effects on the tumor-cell surface and on the tumor microenvironment. The former render the tumor cells more sensitive to cytotoxic T-cell responses due to an increased expression of immunogenic surface molecules like adhesion molecules, death receptors, stress-induced ligands, and classical stimulatory molecules like MHC-1 and B7.1 (CD 80). Concerning the tumor microenvironment, RT triggers a short-term release of many pro-inflammatory molecules, including chemokines, cytokines, and immune activating danger signals (Hatfield et al., Citation2005; Formenti & Demaria, Citation2013).
However, the immunogenic impact of RT is double-edged. In spite of its beneficial immunogenic effects during tumor treatment, it seems obvious that infiltrating immune cells can be directly affected or even killed by RT. Furthermore, irradiation raises the activated proportion of pro-oncogenic and immunosuppressive cytokine transforming growth factor (TGF)-β in the irradiation field (Barcellos-Hoff et al., Citation1994). Therefore, it is absolutely necessary for the successful combination of RT and anti-tumor immunotherapy to determine irradiation protocols that maximize immunostimulatory potentials and reduce immunosuppressive effects as much as possible. One crucial point in this connection is the definition of the most beneficial irradiation dose and fractionation scheme.
Technical advances made it possible to deliver higher irradiation doses to tumors without severely increasing damage to normal tissues. The resulting ‘immunotoxicity’ is restricted to the tumor microenvironment and may promote specific anti-tumor immune responses. Due to these innovations, new treatment approaches are under intensive clinical evaluation. This applies especially for hypo-fractionated RT, which consists of higher single doses by concurrently reduced treatment units (Ko et al., Citation2011). However, while the implicated decrease of total treatment units promise obvious benefits for patient convenience and could reduce healthcare costs, we still lack knowledge about the biological and especially the immunological effects of higher single irradiation doses compared to norm-fractionated RT with a single dose of ≈2 Gy.
It was shown in pre-clinical mouse model studies that high single irradiation doses led to better immunological tumor control than fractionated RT with lower single doses (Lee et al., Citation2009; Lugade et al., Citation2005). In contrast, Dewan et al. (Citation2009) indicated in a murine tumor cell model that an abscopal, out-of-irradiation-field tumor response was only trigged by the combination of fractionated RT with an antibody against the immune inhibitory molecule CTLA-4, whereas the combination with one high single irradiation dose (1 × 20 Gy) failed to induce such an effect. However, it is important to note that the single irradiation doses in this fractionation protocol (i.e. 3 × 8 Gy or 5 × 6 Gy) were much higher than normal clinical fractionation doses (2 Gy) and represented a hypo-fractionated irradiation scheme.
As DC are crucial for the initiation of an immunological anti-tumor immune response, we determined the effects of supernatants (SN) of differently irradiated colorectal tumor cells on DC maturation and activation. Immunostimulatory active DC present tumor antigens to T-cells and induce effective anti-tumor immune responses. However, the microenvironment of many tumors not only inhibits DC in their function, but also changes their phenotype to a more immunosuppressive one that even constrains anti-tumor immune responses and triggers tumor progression (Apetoh et al., Citation2011; Rutkowski et al., Citation2012). RT may be an effective approach to restore the immunostimulatory function of DC (Burnette et al., Citation2011; Gupta et al., Citation2012; Lee et al., Citation2009; Lugade et al., Citation2005). The few human pre-clinical studies revealed that the increase in immunostimulatory surface molecules (such as MHC-1 or ICAM-1) on tumor cells was radiation-dose dependent; however, only high single-irradiation doses were compared in these experiments (Garnett et al., Citation2004; Reits et al., Citation2006). Much less has been published about the immunostimulatory effects of fractionated RT. Tsai et al. (Citation2007) demonstrated that human tumor cells display different gene expression responses in vitro and in vivo after irradiation with single doses compared to fractionated RT. Unfortunately, studies that contrast the immunological effects of differently-fractionated RT with single high irradiation treatments in human tumor cell models are still lacking.
Thus, we compared in this study—for the first time—norm-fractionated RT (5 × 2 Gy) with hypo-fractionated RT (3 × 5 Gy) and with a high single irradiation dose (1 × 15 Gy; which represents a radiosurgery treatment) with regard to their potential to render human colorectal tumor cells immunogenic. We focused on SW480 tumor cells that have been shown previously by our group to bear the capacity to activate DC when treated with a single irradiation dose and additional immune stimulation by hyperthermia (Schildkopf et al., Citation2011).
Materials and methods
Culture of colorectal tumor cells
The experiments were performed with the SW480 human colorectal adenocarcinoma cell line (CCL-228; LGC Standards GmbH, Wesel, Germany). Tumor cells were seeded in cell-culture flasks (Cellstar, Greiner BioOne, Nürtingen, Germany) and cultivated for 6 days at 37 °C with 5% CO2 and 90% humidity in Dulbecco’s Modified Eagle’s Medium (DMEM; PAN-Biotech GmbH, Aidenbach, Germany) containing 10% human AB Serum (Lonza Group AG, Basel, Switzerland) and 18 µg/ml gentamicin (GE Healthcare, Chalfont St. Giles, UK).
X-ray treatment of colorectal tumor cells
SW480 colorectal tumor cells were irradiated with three different irradiation schemes over the period of 5 days. One fraction was irradiated with a daily irradiation dose of 2 Gy (5 × 2 Gy) that mimics a norm-fractionated clinical standard irradiation protocol. Another fraction was irradiated according to a hypo-fractionated radiotherapy with a dose of 5 Gy administered every second day (3 × 5 Gy). A third fraction received one single high dose of 15 Gy on day 5 (that reflects a radiosurgery treatment). Non-irradiated tumor cells, also cultured for 5 days, were used as controls for the basal immunogenicity of colorectal tumor cells. All the following experiments with the irradiated colorectal tumor cells were performed 24 h after the last irradiation unit. Irradiation was performed with an X-ray generator (120 kV, 22.7 mA, variable time; GE Inspection Technologies, Hürth, Germany).
Analysis of the clonogenic potential
Clonogenic assays were conducted with a single-cell suspension of exponentially-growing SW480 colorectal tumor cells as previously described (Mantel et al., Citation2010). Briefly, counted cells were plated into petri dishes (Nunc Thermo Fisher, Waltham, MA) with growth-medium and irradiated 24 h after plating. The irradiation was performed with different irradiation protocols described above, with the exception that the high single irradiation dose of 15 Gy was already applied at day 1 and not on day 5 to compare colony growth after the different treatments. Two weeks later, cells were stained with methylene blue (Sigma, St. Louis, MO) for 30 min and all colonies that contained >50 cells were then counted using an automated colony analyzer.
Determination of tumor cell death
Irradiation-induced tumor cell death was examined by staining the tumor cells with Annexin A5 (AnxA5)-FITC and propidium iodide (PI). Tumor cell suspensions (2 × 105 cells/ml) were incubated with 0.5 µg/ml AnxA5-FITC (Geneart, Regensburg, Germany; FluoroTaq FITC conjugation Kit, Sigma) and 1 µg/ml PI (Sigma) in Ringer’s Solution (B. Braun, Melsungen, Germany) for 30 min at 4 °C. The samples were subsequently analyzed by flow cytometry in an EPICS XL MCL™ system (Beckman Coulter, Brea, CA) using associated Kaluza 1.2 Software®. Viable cells were generally negative for AnxA5 and PI, whereas apoptotic cells were stained by AnxA5, but not by PI. Necrotic cells bound both AnxA5 and PI.
Generation of human immature dendritic cells (iDC)
Monocyte-derived immature dendritic cells (iDC) were generated out of human peripheral blood derived mononuclear cells (PBMC) as previously described (Schaft et al., Citation2005). Briefly, PBMC were isolated from whole blood samples by density gradient centrifugation using Lymphoprep™ (Axis-Shield PoC AS, Oslo, Norway); all samples had been obtained from healthy human donors after informed consent. Afterwards, the PBMC were washed three times with phosphate-buffered saline (PBS, pH 7.4; Lonza) containing 1 mM EDTA and re-suspended in DC medium (RPMI 1640; Lonza) supplemented with 1% heat-inactivated human plasma (Transfusion Medicine, University Hospital Erlangen, Germany), 2 mM L-glutamine, and 20 µg/ml gentamycin. The PBMC were plated in tissue culture dishes (35 × 106 cells/dish; BD Biosciences, Franklin Lakes, NJ) and incubated for 1 h at 37 °C to permit adherence. Subsequently, the non-adherent fraction (NAF) was removed and 10 ml DC medium added to the remaining adherent cells. These cells were cultured for 6 days and fed three times with fresh DC medium (2 ml on day 1, 4 ml on days 3 and 5; up to a final 20 ml volume) containing 250 IU interleukin (IL)-4/ml and 800 IU GM-CSF/ml (both PeproTech, Rocky Hill, NJ) to generate iDC.
Maturation of human dendritic cells with SW480 colorectal tumor cells
As described above, SW480 colorectal tumor cells were differently irradiated and harvested 24 h after the last irradiation on day 6. Next, either 2.5 × 106 tumor cells (re-suspended in 9 ml of their supernatants [SN]) were co-incubated directly with simultaneously-cultured iDC (day 6 of DC culture) or only 9 ml of tumor cell SN was added to the iDC. Before adding the tumor cells in 9 ml of their SN or the SN alone, 5 ml DC medium was removed from every dish. The cells were then co-incubated for 48 h. Immature DC in additional dishes were matured for 48 h with a maturation cocktail bearing 200 U IL-1β (CellGenix Technologie Transfer GmbH, Freiburg, Germany), 1000 U IL-6 (Strathmann Biotec AG, Hamburg, Germany), 1 µg PGE2 (Prostine E2®, Pfizer, New York, NY), and 10 ng tumor necrosis factor (TNF)-α (Beromun, Boehringer Ingelheim, Ingelheim am Rhein, Germany) per ml of DC medium, to generate positive DC maturation controls (mature DC = mDC). Untreated DC dishes were used as negative immature DC controls (iDC).
Analyses of DC maturation by flow cytometry
After 48 h of co-incubation, DC (incubated with tumor cells plus SN or SN alone) were harvested, washed, and then adjusted to 105 cells/100 µl cold FACS solution containing PBS, 0.1% sodium azide (Merck, Darmstadt, Germany), and 1% FBS (PAA Laboratories GmbH, Cölbe, Germany). Staining was conducted with fluorochrome-conjugated antibodies and their appropriate isotype controls for 30 min at 4 °C. The following antibodies were used: CD25-PE, CD40-PE, CD70-PE, CD80-PE, CD83-PE, CD86-PE, IgG1-PE, HLA-DR-FITC, IgG2a-FITC (all BD Biosciences), and IgG3-PE (eBioscience, San Diego, CA). Stained cells were analyzed by 2-color flow cytometry with a FACScan cell analyzer (BD Biosciences). Besides using the forward and side-scatter properties, DC were distinguished from tumor cells by gating on HLA-DR-FITC+ cells as SW480 tumor cells do not express this marker. Results were evaluated with Cell Quest software (BD Biosciences). A minimum of 10 000 events per sample was acquired.
Cytometric bead arrays (CBA)
DC cytokine profiles were measured using a Human Inflammatory Cytokine Kit CBA (BD Biosciences) after co-incubation (48 h) with tumor cells or their SN. Cytokines analyzed were: IL-1β, IL-6, IL-8, IL-10, IL-12p70, and TNFα. A Human T-helper (TH)1/TH2 Cytokine Kit II (BD Biosciences) was used to assess CD4+ T-cell secretion of IL-2, IL-4, IL-6, TNFα, and interferon (IFN)-γ 24 h after stimulation with autologous MAGE-A3-peptide loaded DC. Every CBA was conducted according to manufacturer protocols. All analyses were performed with 2-color flow cytometry in a FACScan cell analyzer using Cell Quest software. Detailed characterization of the CBA principles has been published earlier (Morgan et al., Citation2004).
Generation of human CD4+ T-cells
As already described above for DC, CD4+ T-cells were similarly separated out of whole blood samples by density gradient centrifugation using Lymphoprep™ and subsequent washing steps. Thereafter, CD4+ T-cells were isolated from the PBMC using anti-CD4 MACS beads (Miltenyi, Bergisch Gladbach, Germany) according to manufacturer protocols. The CD4+ cells were then cultivated overnight in MLPC medium with 20 IU IL-7/ml (PeproTech), as noted in Schaft et al. (Citation2006), and used for the antigen-specific CD4+ T-cell-stimulation assays. CD4+ T-cells to be used for mixed-lymphocyte reactions (MLR) were isolated with a MACS isolation kit (CD4+ T-Cell Isolation Kit II, human; Miltenyi) and cultivated in MLPC medium without IL-7 to avoid T-cell-stimulation signals.
Mixed-lymphocyte reactions
CD4+ T-cells were isolated with an untouched MACS isolation kit, washed, re-suspended in PBS, and labeled with 1 µg/ml CFSE (Sigma) for 10 min at room temperature. Staining was stopped by washing with MLPC medium. Subsequently, 5 × 105 CD4+ T-cells were co-incubated with 2.5 × 103 allogeneic DC (ratio 20:1) that had been co-incubated previously with SN of the differently irradiated tumor cells. CD4+ T-cells/DC were plated in 96-well round bottom plates with 225 µl MLPC medium without IL-7 for 4 or 5 days. Subsequently, the cells were harvested and T-cell proliferation was determined by assessing decreases in CFSE fluorescence intensity via flow cytometry (FACSCanto II) using FlowJo software (Tree Star, Ashland, OR), as described in Ekong et al. (Citation2009). Furthermore, we stained the T-cells with CD3-V450, CD4-PerCP Cy5.5, and CD25-PE (all BD Biosciences) antibodies to distinguish CD4+ T-cells from DC and to determine the amount of activated CD4+ T-cells.
Loading of DC with MAGE-A3-peptides
DC peptide loading was performed as in Birkholz et al. (Citation2010). In brief, DC were harvested, adjusted to 106 cells/ml DC medium, and incubated with 10 µg/ml of tumor peptide MAGE-A3 (melanoma-associated antigen 3) (Bachem, Bubendorf, Switzerland) at 37 °C for 3 h. Afterwards, the DC were washed and used for the antigen specific CD4+ T-cell-stimulation assay.
Transfection of CD4+ T-cells with a MAGE-A3/HLA-DP4-specific TCR
CD4+ T-cell electroporation was conducted as described in Schaft et al. (Citation2006). RNA encoding MAGE-A3/HLA-DP4-specific T-cell receptor (TCR) was in vitro transcribed, as shown in Birkholz et al. (Citation2010). Briefly, CD4+ T-cells were harvested and washed with RPMI 1640 and OptiMEM (Life Technologies, Carlsbad, CA) before adjusting to a maximal concentration of 12 × 106 cells/100 µl OptiMEM. The CD4+ T-cells were then mixed with RNA (final concentration per TCR chain = 15 µg/100 µl) in 4-mm cuvettes (Peqlab, Erlangen, Germany). The cells were then pulsed in a Genepulser Xcell (Bio-Rad, Munich, Germany; settings: square wave pulse, 500 V, 5 ms). After electroporation, the cells were directly transferred into MLPC medium containing 20 IU IL-7/ml. Mock-electroporated CD4+ cells (without RNA) were used as negative controls.
Antigen-specific CD4+ T-cell stimulation by autologous DC
Immature DC were co-incubated with SN of the differently irradiated tumor cells and loaded with MAGE-A3-peptide, as explained above. Subsequently, they were co-incubated for 24 h with autologous CD4+ T-cells that were transfected with the MAGE-A3/HLA-DP4-specific T-cell receptor (see above). Cells were inserted equally (0.5 × 105 CD4+ T-cells with 0.5 × 105 DC) into 96-well round bottom plates with 100 µl MLPC-medium and 20 IU IL-7/ml. Antigen-specific CD4+ T-cell stimulation was indirectly determined through the release of cytokines by stimulated CD4+ T-cells, and measured with a TH1/TH2 CBA.
Statistical analysis
Results were expressed as mean (±SD) and compared by using a non-parametric Mann-Whitney U-test. A p value <0.05 was considered statistically significant (*); values <0.01 (**) or <0.001 (***) were considered highly significant.
Results
Irradiation strongly inhibits the colony formation of colorectal tumor cells
Initially, we analyzed the influence of the different irradiation protocols on the colony formation capability of SW480 tumor cells. While a single irradiation with 15 Gy resulted in complete loss of colony formation, norm-fractionated (5 × 2 Gy) and hypo-fractionated irradiation (3 × 5 Gy) reduced the colony formation of the tumor cells in vitro by more than two orders of magnitude, but not completely as displayed with a logarithmic scale in .
Figure 1. Colony formation and cell death of colorectal tumor cells after treatment with different irradiation protocols. (a) Change in colony formation of SW480 tumor cells after single irradiation with 1 × 15 Gy, norm- (5 × 2 Gy), or hypo-fractionated (3 × 5 Gy) irradiation. Colony formation of non-irradiated tumor cells (w/o) was set to 100% and used as reference. Data shown are mean (±SD) of three independent experiments and displayed in logarithmic scale. (b) Forms of SW480 tumor cell death after exposure to the different irradiation protocols described in (a). Tumor cells were stained with AnxA5-FITC/PI 24 h after the final irradiation unit and then analyzed by flow cytometry. Percentages of viable (AnxA5−/PI−), necrotic (AnxA5+/PI+), and apoptotic (AnxA5+, PI−) tumor cells are demonstrated. Values are means (±SD) of eight independent experiments. Gy, Gray; w/o, non-irradiated tumor-cell controls. *p < 0.05; ***p < 0.001.
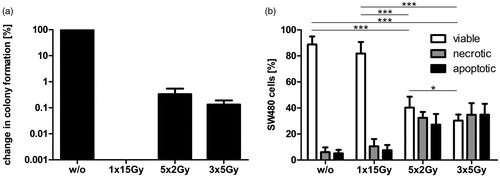
Only norm- and hypo-fractionated RT result in fast cell death induction of colorectal tumor cells
Despite the tumor-cell proliferation determined by the clonogenic assay (), we analyzed the tumor-cell-death induction at an early timepoint (24 h) after the last irradiation. Norm- and hypo-fractionated RT led to a significant decrease of viable cells compared to non-irradiated tumor cells (w/o) and to SW480 cells that were only irradiated once with a high dose (15 Gy; ). Conversely, the amounts of apoptotic and necrotic tumor cells were considerably extended by the fractionated irradiation protocols. Moreover, tumor cells showed a significant higher cell-death proportion, if they were treated with hypo-fractionated RT instead of norm-fractionated RT. From these results, we conclude that fractionated RT has a differential effect on colony formation and cell death of colorectal tumor cells compared to single irradiation.
DC maturation is significantly improved after co-incubation with supernatants of tumor cells treated with norm- or hypo-fractionated RT
To evaluate if the different irradiation schemes affect the SW480 tumor cell capability to mature DC, iDC were co-incubated for 48 h with supernatants (SN) of tumor cells treated with the different irradiation protocols. Thereafter, DC surface expression of classic maturation markers was assessed by flow cytometry (). As positive control for DC maturation, iDC were treated with a maturation cytokine cocktail (see Materials and Methods section) for 48 h (mDC). The SN of non-irradiated tumor cells (w/o) already slightly enhanced the expression of the DC maturation markers CD25 (), CD80 (), and CD83 (). These inherent immuno-stimulatory effects of SW480 tumor cells were not affected if the tumor cells were irradiated with one single high irradiation dose (1 × 15 Gy). In contrast, co-incubation of iDC with SN of tumor cells treated with norm- or hypo-fractionated RT led to a significant additional increase in the expression of these maturation markers compared to the SN of non-irradiated tumor cells and to tumor cells that received only one high irradiation dose (). However, there was no detectable difference between the norm- and the hypo-fractionated RT. Similar DC maturation results were obtained if iDC were co-incubated directly with differently irradiated tumor cells and their SN (data not shown). Surface expression of the maturation markers CD86, CD70, or CD40 was not influenced by the SN of/or by the SW480 tumor cells (data not shown). Taken together, our data indicate that the SN of colorectal tumor cells irradiated in a norm- or hypo-fractionated manner have a significantly higher maturation effect on iDC in contrast to the SN of tumor cells that obtained only a high single irradiation treatment or were left untreated.
Figure 2. Maturation of DC after incubation with supernatants (SN) of differently irradiated tumor cells. Immature DC (iDC) were co-incubated with the SN of non- or differently irradiated tumor cells for 48 h. Surface expression of DC maturation markers (a) CD25, (b) CD80, and (c) CD83 was then analyzed by flow cytometry. Untreated iDC were used as negative controls. DC matured with a classical maturation cocktail served as positive controls (mDC). Values displayed are mean (±SD) of six independent experiments. DC, dendritic cells; SN, supernatants; Gy, Gray; w/o, non-irradiated tumor cell controls; MFI, mean fluorescence intensity. *p < 0.05; **p < 0.01.
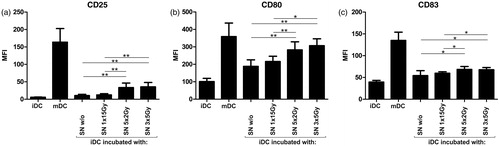
SN of tumor cells irradiated with norm- or hypo-fractionated RT trigger the release of pro-inflammatory cytokines by DC
To characterize the cytokine secretion profile of DC after contact with SN of non- and differently irradiated tumor cells, iDC were initially co-incubated with SN for 48 h. As controls, untreated iDC and cytokine cocktail-matured DC were used. Subsequently, DC cytokine secretion was analyzed with a Cytometric Bead Array (CBA; ). Similar to the elevated surface expression of distinct maturation markers, co-incubation of iDC with the SN of SW480 cells treated with norm- or hypo-fractionated RT resulted in the strongest cytokine secretion effects. Such SN notably increased release of the pro-inflammatory cytokines IL-12p70 (), IL-8 (), IL-6 (), and TNFα () compared to the SN of high single irradiated or untreated tumor cells. Secretion of the pro-inflammatory cytokine IL-1β () and the more immunosuppressive IL-10 () was slightly – but not significantly – enhanced by the SN of tumor cells irradiated with fractionated RT. Regarding disparities between the two fractionated irradiation protocols, the hypo-fractionated one tended to stimulate DC cytokine secretion more effectively than the norm-fractionated protocol, but the differences were insignificant. The relatively high amounts of IL-6, TNFα, and IL-1β associated with the mDC controls were probably caused by a presence of these cytokines in the maturation cocktail. Similar results were obtained in experiments performed by co-incubating irradiated SW480 tumor cells directly with iDC (in addition to their SN; data not shown). Moreover, we conducted CBAs with sole tumor cells to confirm that the measured cytokines were specifically produced by DC and not by the irradiated tumor cells, but did not detect notable amounts of cytokines (data not shown). Collectively, the SN of tumor cells irradiated with norm- or hypo-fractionated RT, but not of cells receiving a single irradiation dose, trigger the release of pro-inflammatory cytokines by DC.
Figure 3. Cytokine secretion by DC after incubation with SN of differently irradiated tumor cells. SN of non- or differently irradiated tumor cells were added to immature DC cultures (iDC) for 48 h. The DC cytokine secretion profiles were then determined by Cytometric Bead Array (a) IL-12p70, (b) IL-8, (c) IL-6, (d) TNFα, (e) IL-1β, and (f) IL-10. iDC were applied as negative controls. DC matured with a classical maturation cocktail served as positive controls (mDC). Mean values (±SD) of six independent experiments are illustrated. DC, dendritic cells; SN, supernatants; Gy, Gray; w/o, non-irradiated tumor cells. *p < 0.05; **p < 0.01.
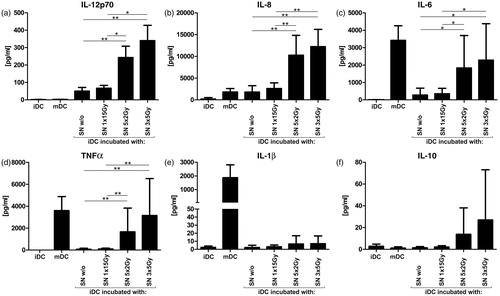
DC co-incubated with SN of irradiated or untreated tumor cells induce a strong allogenic CD4+ T-cell proliferation and increase the portion of activated CD4+ T-cells
A mixed-lymphocyte reaction (MLR) was used to determine if co-incubation with SN of differently irradiated tumor cells modified the ability of iDC to stimulate CD4+ T-cell proliferation (). iDC were matured for 48 h with SN of the differently irradiated tumor cells and co-incubated afterwards with CFSE-labeled allogeneic CD4+ T-cells for 4 or 5 days. Immature control DC induced only a weak T-cell proliferation. In contrast, iDC that had been incubated with the SN of tumor cells induced a strong allogeneic CD4+ T-cell proliferation and tended to stimulate CD4+ T-cells such as mature DC controls (). In addition, they increased the fraction of activated CD4+ T-cells on day 5, as illustrated by the enhanced surface expression of the late CD4+ T-cell activation marker CD25 (). In summary, DC co-incubated with SN of differently irradiated or untreated tumor cells not only elicited a markedly CD4+ T-cell proliferation, but also extended the number of activated CD4+ T-cells.
Figure 4. CD4+ T-cell proliferation and activation after co-incubation with allogeneic iDC matured with SN of differently irradiated tumor cells. CD4+ T-cells were stained with CFSE and co-incubated with allogeneic iDC that had been incubated with the SN of non- or differently irradiated tumor cells for 48 h. After (a) day 4 or (b) day 5, CD4+ T-cell proliferation was assessed by CFSE fluorescence decrease. (c) Additional staining with CD3-V450, CD4-PerCP Cy5.5, and CD25-PE antibodies ensured the separation between CD4+ T-cells and DC and identified the amount of activated CD4+ T-cells. CD4+ T-cells co-incubated with DC matured with a classical maturation cocktail (mDC) served as positive controls. Unstimulated CD4+ T-cells (without DC co-incubation) were used as baseline controls. Results shown are mean (±SD) of four independent experiments. DC, dendritic cells; SN, supernatants; Gy, Gray; w/o, non-irradiated tumor-cell controls. *p < 0.05.
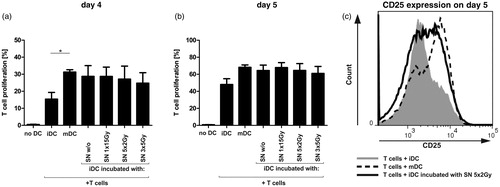
Immature DC treated with SN of tumor cells irradiated with norm- or hypo-fractionated RT stimulate CD4+ T-cells in an antigen-specific manner similar to mature DC
To further characterize how differently irradiated tumor cell conditions affect interactions between human DC and human CD4+ T-cells, an antigen-specific CD4+ T-cell stimulation assay was developed. After co-incubating HLA-DP4+ iDC with SN of the non- or differently irradiated tumor cells, DC were loaded with the tumor peptide MAGE-A3 (melanoma-associated antigen 3) and then co-incubated with autologous CD4+ T-cells for 24 h. As these CD4+ T-cells had been transfected previously with a MAGE-A3/HLA-DP4-specific TCR, they were able to recognize the MAGE-A3/HLA-DP4 complexes on DC surfaces. This specific interaction enabled us to perform an antigen-specific human CD4+ T-cells stimulation assay. The degree of CD4+ T-cell stimulation was finally determined by measuring specific release of IFNγ (), TNFα (), and IL-10 () using a CBA.
Figure 5. Antigen-specific CD4+ T-cell stimulation by peptide-loaded DC matured with the SN of differently irradiated tumor cells. Immature DC (iDC) were co-incubated with the SN of non- or differently irradiated SW480 tumor cells loaded with MAGE A3-peptide and co-incubated for 24 h with autologous CD4+ T-cells that were transfected with a T-cell receptor specific for MAGE-A3/HLA-DP4. Specific CD4+ T-cell stimulation was determined by amount of cytokines secreted: (a) IFNγ, (b) TNFα, and (c) IL-10. Reference values result from CD4+ T-cells co-incubated with iDC or matured DC (mDC) generated with a classic maturation cocktail. Mock-electroporated CD4+ T-cells were used as unspecific controls (T-cells without TCR). Cytokine secretion profiles of mDC without further CD4+ T-cell co-incubation are displayed for comparison. Data illustrated are means (±SD) of four independent experiments with T-cells and DC of four different healthy donors. DC, dendritic cells; SN, supernatants; Gy, Gray; w/o, untreated tumor-cell controls; TCR, specific T-cell receptors.
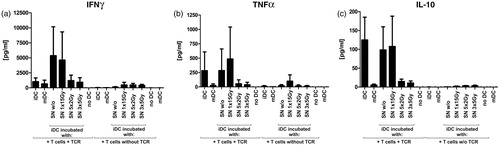
The iDC matured with SN of tumor cells that were irradiated with norm- or hypo-fractionated RT induced a specific, but only slightly enhanced cytokine secretion by CD4+ T-cells: compared to unspecific controls, they induced a 2–3-fold-increase for IFNγ, TNFα, and IL-10. Similar cytokine concentrations were obtained for CD4+ T-cells that were co-incubated with iDC that were matured with a classical maturation cocktail (mDC) ().
In contrast, iDC that were co-incubated with SN of tumor cells that were irradiated with a high single irradiation dose or were not irradiated, triggered – depending on the donor (n = 4) – a strongly varying cytokine release by the antigen-specific CD4+ T-cells. These stimulating effects corresponded to those induced by untreated iDC, especially with regard to TNFα and IL-10 (). Secretion of IL-4 was not influenced by the SN of SW480 colorectal tumor cells; release of IL-2 and IL-6 showed no antigen specificity (data not shown). In summary, the data suggest to us that the SN of tumor cells irradiated with norm- or hypo-fractionated RT may push iDC in the direction of mDC, which is indicated by their stimulatory capacity of antigen-specific CD4+ T-cells.
Discussion
We here compared in a human pre-clinical in vitro study for the first time the immuno-stimulatory effects of norm-fractionated radiotherapy with a hypo-fractionated irradiation protocol, and irradiation with one high single dose. To exclude that the results were influenced by varying cultivation times, all human colorectal tumor cells (SW480) were cultivated simultaneously over 5 days and irradiated with the different irradiation protocols during this period.
All irradiation schemes induced an almost entire loss of tumor-cell-colony formation, which reflects a strong local tumor control: norm- and hypo-fractionated irradiation protocols decreased the colony formation of the tumor cells in vitro by more than two orders of magnitude, and the high single irradiation treatment resulted in a complete colony-formation stop (). Conversely, norm- and hypo-fractionated irradiation approaches increased the tumor-cell-death proportion greatly 24 h after the last irradiation treatment; irradiation with one high single dose induced only a slight percentage of dead tumor cells at this early timepoint after exposure to RT (). Based on our knowledge about ablative total body irradiation before stem cell transplantations (Wolden et al., Citation2013), one may expect that a single irradiation with 15 Gy must trigger a strong and fast cell death. However, in tumor cells such as SW480 colorectal ones, cell death occurs at later timepoints after irradiation, following the once induced proliferation stop of the tumor cells (Frey et al., Citation2012). We focused in our experiments consciously on this early time-point (24 h) after the last irradiation treatment, since a fast induction of an immunogenic tumor-cell phenotype is required for combining RT with distinct further immune therapies. The hypo-fractionated irradiation scheme provoked a slight, but significant higher amount of tumor-cell death compared to the norm-fractionated irradiation protocol. This may explain our result that SN of hypo-fractionated treatment conditions tended to be a stronger cytokine secretion stimulus for iDC than SN of norm-fractionated tumor cells (). Nevertheless, the co-incubation of iDC with SN of tumor cells irradiated with norm- or hypo-fractionated RT resulted in increased expression of DC maturation markers ().
It has been described that DC possess both tumor-inhibitory and tumor-stimulatory potentials. Their maturation is pivotal in this context. Only entirely matured DC (mDC) with immunostimulatory phenotypes are able to induce anti-tumor immune responses, whereas iDC can provoke tumor progression (Hargadon, Citation2013). Thus, we co-incubated iDC with SN of the differently irradiated colorectal tumor cells and analyzed afterwards their maturation state to determine irradiation protocols that stimulate DC maturation most effectively. The co-incubation with SN of colorectal tumor cells irradiated with norm- or hypo-fractionated RT enhanced the surface expression of the classical DC maturation markers CD80 and CD83 (Banchereau & Steinman, Citation1998) significantly compared to the SN of high single irradiated or untreated tumor cells (). The surface expression of CD25 showed even a 3-fold increase. Although the biological function of CD25 (IL-2Rα chain) on monocyte-derived DC is not completely understood, it was suggested as an informative maturation marker due to its relation to T-cell activation capacity (Velten et al., Citation2007). While some publications have related CD25 more with immunosuppressive functions (Driesen et al., Citation2008), Wuest et al. (Citation2011) revealed that the surface expression of CD25 on DC was important for CD4+ T-cell stimulation and may act by delivering IL-2 to CD4+ T-cells within the formed immunesynapse between DC and CD4+ T-cells.
Another important feature of mDC is their secretion of immunostimulatory cytokines. Accordingly to the elevated DC maturation markers, the SN of tumor cells irradiated with norm- or hypo-fractionated RT increased the release of the pro-inflammatory cytokines IL-12p70, IL-8, IL-6, and TNFα significantly compared to that from the SN of single irradiated and untreated tumor cells (). Release of immunostimulatory IL-1β and rather immunosuppresssive IL-10 cytokines was also raised by the SN from tumor cells treated with norm- or hypo-fractionated RT, but only slightly and without considerable differences to the other treatment conditions (). Positive control DC (mature DC) showed no up-regulation of IL-12p70, although its secretion is a crucial quality of mDC in vivo. As described elsewhere (Kalinski et al., Citation1997), this conflicting observation is caused by prostaglandin E2 (PGE2) that was used in the DC maturation cocktails here (see Materials and Methods section). The SN of tumor cells treated with hypo-fractionated RT tended to be more effective secretion stimuli for DC than SN of norm-fractionated tumor cells. This might be due to the slightly higher amount of dead tumor cells already 24 h after the last irradiation.
In a recently published systematic review, Lippitz (Citation2013) demonstrated that different tumor entities exhibit uniform patterns of cytokine reactions. Briefly, these common cytokine reactions terminate in a condition of immune stimulation and coincidental immunosuppression, which guides immune cells to the tumor side, but blocks their tumor destructive functions. For example, elevated serum levels of pro-inflammatory cytokines like IL-6, IL-8, TNFα, and IL-1β are regularly found in tumor patients, but failed to induce anti-tumor immune responses and even promoted tumor progression. Increased serum levels of IL-10 and the concomitant down-regulation of IL-12 are highly distinctive for tumor cytokine patterns. IL-10 is crucial to maintain an immunosuppressive condition by mainly suppressing DC to release IL-12p70, which is indispensable for the induction of sufficient anti-tumor TH1 responses. Our data indicate that DC release notably higher amounts of IL-12p70 after co-incubation with SN of tumor cells irradiated with norm- or hypo-fractionated RT. Interestingly, the secretion of IL-10 was only slightly enhanced and without considerable differences to the other treatment conditions. Based on this, we suggest that fractionated radiotherapy is especially suited to induce DC that generate effective anti-tumor cytokine profiles.
As effective anti-tumor immune reactions require the induction of sufficient TH1 responses by DC, we analyzed the capability of iDC to stimulate CD4+ T-cells after co-incubation with SN of the differently irradiated tumor cells. All iDC that were previously co-incubated with SN of differently irradiated or untreated tumor cells induced a strong proliferation of allogeneic CD4+ T-cells that was similar to the proliferation rate induced by mature DC controls (). Moreover, these DC increased the fraction of activated CD4+ T-cells that greatly expressed CD25 (). We conclude from these results that the co-incubation with SN of differently irradiated or untreated tumor cells generates functional active dendritic cells which are able to trigger effective T-cell responses.
Since we could not detect disparities between the different irradiation schemes and the untreated controls, we developed an antigen-specific CD4+ T-cell stimulation assay. As target antigen, we selected the tumor peptide MAGE-A3, which is widely expressed on several human colorectal tumor tissues and tumor cell lines including SW480 cells (Kim et al., Citation2006). Accordingly, we confirmed MAGE-A3 mRNA expression in our SW480 cells by RT-PCR (data not shown), but the amount of MAGE-A3 protein was too low to trigger effective antigen-specific CD4+ T-cell responses if we use irradiated SW480 tumor cells as the sole antigen sources. Hence, to ensure sufficient amounts of antigen for CD4+ T-cell presentation by DC, the experimental setting was modified and DC were loaded with extrinsic MAGE-A3 peptide after co-incubation with the different tumor-cell SN. As shown by the specifically increased secretions of IFNγ, TNFα, and IL-10, all DC conditions induced specific CD4+ T-cell responses after co-incubation with SN of differently irradiated tumor cells (). The DC co-incubated with the SN of norm- or hypo-fractionated irradiated tumor cells triggered cytokine secretion specifically, but only slightly, and thereby acted similar to mature DC.
Conversely, DC treated with SN of single irradiated tumor cells or untreated tumor cells exhibited stimulation characteristics like iDC: they induced a strong release of IFNγ, TNFα, but also of the more immunosuppressive cytokine IL-10. In addition, they showed a large variability between different blood donors. Surprisingly, iDC stimulated CD4+ T-cells more effectively than matured DC. However, this could be attributed to their higher endocytotic activity (Mellman & Steinman, Citation2001), which may lead to an accelerated HLA-DP4+ antigen turnover that resulted in a better antigen presentation to CD4+ T-cells. Further, to avoid cytokine biases, all DC were washed prior to the co-incubation and were finally cultivated with the antigen-specific CD4+ T-cells in fresh medium. During this washing, all cytokines in the DC media were also removed. This may explain the fact that DC that were co-incubated with the SN of tumor cells treated with norm- or hypo-fractionated RT induced only slight IFNγ secretion by CD4+ T-cells, although the same DC conditions produced the highest levels of IL-12p70, which is a key IFNγ-inducing cytokine (Abbas et al., Citation1996). Taken together, DC under all co-incubation conditions induced CD4+ T-cell cytokine profiles that indicated to be consistent with the induction of TH1-like T-cell responses: each DC condition stimulated CD4+ T-cells to release the typical TH1 cytokines IFNγ and TNFα, whereas secretion of IL-4 (preferentially produced by TH2 cells; Abbas et al., Citation1996) was not enhanced (data not shown).
Conclusion
Collectively, our results reveal that norm- and hypo-fractionated radiotherapy induce a colorectal tumor cell death with immunogenic potential, since SN of the treated tumor cells trigger DC maturation and release of immune activating cytokines, such as IL12p70. In addition, our data demonstrate that iDC co-incubated with SN of differently irradiated or untreated tumor cells provoke a potent allogeneic CD4+ T-cell proliferation and increased the number of activated CD4+ T-cells simultaneously. In the antigen-specific assay, the CD4+ T-cell responses were most likely consistent with TH1 reactions in all iDC conditions that have been previously co-incubated with SN of differently irradiated or untreated tumor cells. However, only iDC that were co-incubated with SN of tumor cells irradiated with norm- or hypo-fractionated RT demonstrated a similar antigen-specific CD4+ T-cells stimulation profile like mature DC controls. Our data therefore suggest the highest ‘immunotoxic’ potential of norm-fractionated and particularly hypo-fractionated tumor-cell-irradiation protocols, which may contribute to the induction of specific anti-tumor immune responses. However, it is definitely too early for final conclusions. Further work has to be done that evaluates the immunologic effects of different irradiation protocols in other human tumor cell lines and tumor-bearing mice models. From that, we will further expand our knowledge about the complex interplay between the tumor cells, tumor microenvironment, surrounding tissue, and especially the immune system. Our findings may ultimately contribute to development of innovative irradiation strategies that could be combined with further immune stimulating therapies to reinforce the patient immune system and trigger effective anti-tumor immune responses.
Declaration of interest
The authors report no conflicts of interest. The authors alone are responsible for the content and writing of the paper.
This work corresponds to the medical doctoral thesis of first author Kulzer and fulfills the requirements for obtaining the degree “Dr med.”, and was supported by the German Research Foundation (DFG-Graduiertenkolleg 1660: Key signals of the adaptive immune response and GA 1507/1-1), the DFG-SFB643 (Project C1) and the Graduate School of the SFB 643 (GRK643), the German Federal Ministry of Education and Research (BMBF; m4 Cluster, 16EX1021R and GREWIS, 02NUK017G), and the European Commissions (DoReMi, European Atomic Energy Community’s Seventh Framework Programme (FP7/2007-2011) under grant agreement n°249689).
References
- Abbas, A. K., Murphy, K. M., and Sher, A. 1996. Functional diversity of helper T-lymphocytes. Nature 383:787–793
- Apetoh, L., Locher, C., Ghiringhelli, F., et al. 2011. Harnessing dendritic cells in cancer. Semin. Immunol. 23:42–49
- Banchereau, J., and Steinman, R. M. 1998. Dendritic cells and the control of immunity. Nature 392:245–252
- Barcellos-Hoff, M. H., Derynck, R., Tsang, L. S., and Weatherbee, J. A. 1994. Transforming growth factor-β activation in irradiated murine mammary gland. J. Clin. Invest. 93:892–899
- Birkholz, K., Schwenkert, M., Kellner, C., et al. 2010. Targeting of DEC-205 on human dendritic cells results in efficient MHC Class II-restricted antigen presentation. Blood 116:2277–2285
- Burnette, B. C., Liang, H., Lee, Y., et al. 2011. The efficacy of radiotherapy relies upon induction of Type I IFN-dependent innate and adaptive immunity. Cancer Res. 71:2488i–2496
- Dewan, M. Z., Galloway, A. E., Kawashima, N., et al. 2009. Fractionated but not single-dose radiotherapy induces an immune-mediated abscopal effect when combined with anti-CTLA-4 antibody. Clin. Cancer Res. 15:5379–5388
- Driesen, J., Popov, A., and Schultze, J. L. 2008. CD25 as an immune regulatory molecule expressed on myeloid dendritic cells. Immunobiology 213:849–858
- Ekong, U. D., Miller, S. D., and O’Gorman, M. R. 2009. In vitro assays of allo-sensitization. Pediatr. Transplant. 13:25–34
- Formenti, S. C., and Demaria, S. 2009. Systemic effects of local radiotherapy. Lancet Oncol. 10:718–726
- Formenti, S. C., and Demaria, S. 2013. Combining radiotherapy and cancer immunotherapy: A paradigm shift. J. Natl. Cancer Inst. 105:256–265
- Frey, B., Stache, C., Rubner, Y., et al. 2012. Combined treatment of human colorectal tumor cell lines with chemotherapeutic agents and ionizing irradiation can in vitro induce tumor cell death forms with immunogenic potential. J. Immunotoxicol. 9:301–313
- Garnett, C. T., Palena, C., Chakarborty, M., et al. 2004. Sublethal irradiation of human tumor cells modulates phenotype resulting in enhanced killing by cytotoxic T-lymphocytes. Cancer Res. 64:7985–7994
- Gupta, A., Probst, H. C., Vuong, V., et al. 2012. Radiotherapy promotes tumor-specific effector CD8+ T-cells via dendritic cell activation. J. Immunol. 189:558–566
- Hargadon, K. M. 2013. Tumor-altered dendritic cell function: Implications for anti-tumor immunity. Front. Immunol. 4:1–13
- Hatfield, P., Merrick, A., Harringtony, K., et al. 2005. Radiation-induced cell death and dendritic cells: Potential for cancer Immunotherapy? Clin. Oncol. 17:1–11
- Kalinski, P., Hilkens, C. M., Snijders, A., et al. 1997. IL-12-deficient dendritic cells, generated in the presence of prostaglandin E2, promote type 2 cytokine production in maturing human naive T-helper cells. J. Immunol. 159:28–35
- Kim, K. H., Coi, J. S., Kim, I. J., et al. 2006. Promoter hypo-methylation and reactivation of MAGE-A1 and MAGE-A3 genes in colorectal cancer cell lines and cancer tissues. World J. Gastroenterol. 12:5651–5657
- Ko, E. C., Forsythe, K., Buckstein, M., et al. 2011. Radiobiological rationale and clinical implications of hypo-fractionated radiation therapy. Cancer Radiother. 15:221–229
- Lee, Y., Auh, S. L., Wang, Y., et al. 2009. Therapeutic effects of ablative radiation on local tumor require CD8+ T-cells: Changing strategies for cancer treatment. Blood 114:589–595
- Lippitz, B. E. 2013. Cytokine patterns in patients with cancer: A systematic review. Lancet Oncol. 14:e218–e228
- Lugade, A. A., Moran, J. P., Gerber, S. A., et al. 2005. Local radiation therapy of B16 melanoma tumors increases the generation of tumor antigen-specific effector cells that traffic to the tumor. J. Immunol. 174:7516–7523
- Mantel, F., Frey, B., Haslinger, S., et al. 2010. Combination of ionizing irradiation and hyperthermia activates programmed apoptotic and necrotic cell death pathways in human colorectal carcinoma cells. Strahlenther. Onkol. 186:587–599
- Mellman, I., and Steinman, R. M. 2001. Dendritic cells: Specialized and regulated antigen- processing machines. Cell 106:255–258
- Morgan, E., Varro, R., Sepulveda, H., et al. 2004. Cytometric bead array: A multiplexed assay platform with applications in various areas of biology. Clin. Immunol. 110:252–266
- Postow, M. A., Callahn, M. K., Barker, C. A., et al. 2012. Immunologic correlates of the abscopal effect in a patient with melanoma. New Engl. J. Med. 366:925–931
- Reits, E. A., Hodge, J. W., Herberts, C. A., et al. 2006. Radiation modulates the peptide repertoire, enhances MHC Class I expression, and induces successful anti-tumor immunotherapy. J. Exp. Med. 203:1259–1271
- Rubner, Y., Wunderlich, R., Rühle, P. F., et al. 2012. How does ionizing irradiation contribute to the induction of anti-tumor immunity? Front. Oncol. 2:1–11
- Rutkowski, M. R., Stephen, T. L., and Conejo-Garcia, J. R. 2012. Anti-tumor immunity: Myeloid leukocytes control the immune landscape. Cell. Immunol. 278:21–26
- Schaft, N., Dörrie, J., Müller, I., et al. 2006. A new way to generate cytolytic tumor-specific T-cells: Electroporation of RNA coding for a T-cell receptor into T-lymphocytes. Cancer Immunol. Immunother. 55:1132–1141
- Schaft, N., Dörrie, J., Thumann, P., et al. 2005. Generation of an optimized polyvalent monocyte-derived dendritic cell vaccine by transfecting defined RNAs after rather than before maturation. J. Immunol. 174:3087–3097
- Schildkopf, P., Frey, B., Ott, O. J., et al. 2011. Radiation combined with hyperthermia induces HSP70-dependent maturation of dendritic cells and release of pro-inflammatory cytokines by dendritic cells and macrophages. Radiother. Oncol. 101:109–115
- Tsai, M. H., Cook, J. A., Chandramouli, G. V. R, et al. 2007. Gene expression profiling of breast, prostate, and glioma cells following single vs. fractionated doses of radiation. Cancer Res. 67:3845–3852
- Velten, F. W., Rambow, F., Metharom, P., and Goerdt, S. 2007. Enhanced T-cell activation and T-cell-dependent IL-2 production by CD83+, CD25high, CD43high human monocyte-derived dendritic cells. Mol. Immunol. 44:1544–1550
- Wolden, S. L., Rabinovitch, R. A., Bittner, N. H., et al. 2013. American College of Radiology (ACR) and American Society for Radiation Oncology (ASTRO) Practice Guideline for the Performance of Total Body Irradiation (TBI). Am. J. Clin. Oncol. 36:97–101
- Wuest, S. C., Edwan, J. H., Martin, J. F., et al. 2011. A role for O:-2 trans-presentation in dendritic cell-mediated T-cell activation in humans, as revealed by daclizumab therapy. Nat. Med. 17:604–609