Abstract
Cadmium (Cd), a known carcinogen and potent immunotoxicant in humans and animals, is dispersed throughout the environment as a result of pollution from a variety of sources. Tunisian radish (Raphanus sativus) extract (TRE) is a known anti-oxidant and free radical scavenger that has been shown to help alleviate immune system disorders, including some induced by environmental toxicants. The present study was undertaken to investigate potential protective effects of TRE against Cd-induced immunotoxicities (and general toxicities) in situ. Cadmium chloride (at 2.5 mg CdCl2/kg BW) and TRE (5, 10, or 15 mg/kg BW) were given (alone or in combination [actually, in sequence of Cd and then TRE]) to rats daily by oral gavage for 2 weeks. Results indicated that treatment with CdCl2 alone resulted in significant decreases in plasma levels of total protein, triglycerides, creatine kinase, creatinine, IgG and IgA, T-lymphocyte sub-types (CD4+, CD3+, CD56+, and CD8+), and in thymic and hepatic indices (relative weights). In contrast, CdCl2 treatment caused significant increases in serum LDH, AST, and ALT, in the formation/release of pro-inflammatory cytokines (IL-1 and TNFα), and in the relative weights of host spleen and kidneys. Rats treated with TRE alone had no discernable changes compared to the controls with regard to all test parameters. Combined treatment of CdCl2 and TRE—at any dose—resulted in a significant improvement of all test parameters compared to those seen with Cd alone. These results illustrated (and provided further support for a continuing belief in) the beneficial effects of TRE in reducing the harmful outcomes of commonly encountered toxicants (like Cd) on the immune system and on overall host health status.
Introduction
Contamination of food and feedstuff with heavy metals presents many problems for current and future human and animal health (FAO/WHO, Citation2003; Satarug et al., Citation2003). Cadmium (Cd) is widely distributed in the environment due to its various industrial uses and is known to bioaccumulate and cause harmful effects in different levels of the trophic chain (Stoeppler, Citation1991). As Cd is a known carcinogen (Banerjee & Flores-Rozas, Citation2005), teratogen (Hovland et al., Citation2000), nephrotoxin (Cai et al., Citation2001), hepatotoxin (Horiguchi et al., Citation2000), genotoxin, and immunotoxin, it is thus unsurprising that excessive acute/continuous low–mid-level exposures to Cd results in an increased incidence of diseases and deaths (Othumpangat et al., Citation2005). Various mechanisms have been suggested to explain Cd-induced toxicities; these include an indirect increase in the formation of reactive oxygen species that, in turn, give rise to increased lipid peroxidation in cell membranes, DNA damage, altered gene expression, and, in some cases, increased induction of apoptosis (Abbès et al., Citation2007; Stohs et al., Citation2001).
Toxic effects of Cd have also been demonstrated on the immune system (Abbès et al., Citation2007; Waalkes et al., Citation1999). Effects of Cd on immune system organs and on immune cells, as well as on specific and non-specific immune responses, indicate an impact that could lead to significant decreases in host resistance. However, experimental data has revealed that the immunotoxicity of Cd depends on form used, the route, dose, and duration of exposure, as well as host physiologic status (Koller, Citation1998; Payette et al., Citation1995). While many of the observations of effect result from acute exposures to Cd, immunotoxicity has also been reported after chronic Cd exposure (Blakley, Citation1985). For example, chronic exposure to Cd led to decreases in lymphocyte proliferation and IgE synthesis (Marth et al., Citation2001) and increases in IgM and IgG responses (Exon et al., Citation1996). Changes in release of critical cytokines (i.e. interleukin (IL)-1 and tumor necrosis factor [TNF]-α) (Marth et al., Citation2000) and phagocytosis by macrophages (Zelikoff et al., Citation1995) have also been documented. Cifone et al. (Citation1989) reported that exposure of rats to 200 or 400 ppm Cd caused both inhibitory and stimulatory effects on natural killer (NK) cell activity and cytotoxic activity. Moreover, Nasr et al. (2006) indicated that Cd treatment exerted differential effects on cytokine production.
There have been many studies that have illustrated a beneficial role for cruciferous plants in the human diet, as these contain a wide variety of anti-oxidants (Takaya et al., Citation2003) that provide host protection against cell/membrane damage (Gill et al., Citation2004). Several reports attributed the beneficial effect of a radish-based diet to a presence of one characteristic class of compounds, i.e. glucosinolates, which are hydrolyzed to isothiocyanates by gut microflora (Jeffery & Jarrell, Citation2001). Those compounds are thought to help prevent cancer and degenerative diseases by increasing cellular mechanisms to deactivate carcinogens and reactive oxygen species via a so-called “electrophile counterattack” (Prestera et al., Citation1993). Radish (Raphanus sativus), among the cruciferous vegetables with considerable anti-carcinogenic properties, is widely grown worldwide. For many years, it has been used for medicinal purpose (Nadkarni, Citation1976) as a laxative, a stimulant, a digestive aid, an appetizer, and in other stomach disorders (Kapoor, Citation1990). Over the years, other studies have found this material to be anti-microbial (Esaki & Onozaki, Citation1982), anti-mutagenic (Hashem & Saleh, Citation1999), and anti-carcinogenic (Hecht, Citation1999). More recently, Suh et al. (Citation2006) showed that radish extracts inhibited the abnormal growth of vascular smooth muscle cells—a prominent feature of vascular diseases like atherosclerosis and restenosis.
Several reports have indicated that the main therapeutic constituents of radish include 4-(methylthio)-3-butenyl isothiocyanate (MTBITC), allyl isothiocayanate, benzyl isothiocyanate and phenethyl isothiocyanate, flavonoides besides kaempherol glycosides, various types of peroxidases, and a plethora of anti-oxidants (Hashimoto et al., Citation2006; Katsuzaki et al., Citation2004; Suh et al., Citation2006; Wang et al., Citation2004). Lugasi et al. (Citation2001) reported that radish root extract imparted anti-oxidant activity in vitro; Sipos et al. (Citation2002) showed that use of the root extract helped to inhibit membrane changes caused by fat-rich diets, beneficially influenced the natural scavenging activity of colon mucosa, and could lead to increases in the ability of cell membranes to resist damage from lipid peroxidation. Takaya et al. (Citation2003) and Katsuzaki et al. (Citation2004) suggested a crude extract of radish contained anti-oxidant enzymes as well as the anti-oxidant l-tryptophan.
As many of the mechanisms implicated in Cd toxicity are related to increased cellular and genetic oxidative damage, it is understandable that these toxicities could be ameliorated/blocked by use of anti-oxidants (Yiin et al., Citation1999; Gupta Sen et al., Citation2004a,Citationb). Among the anti-oxidants evaluated to date have been ascorbic acid, flavonoids, isothiocyanates, and α-tocopherol (Fang et al., Citation2002; Yoshiro et al., Citation2003). We have already shown that a Tunisian radish extract (TRE) protected a host against liver and kidney damage and changes in host anti-oxidant enzymes status associated with food contaminant exposures (Ben Salah-Abbès et al., Citation2008a). Based on those findings, and the earlier studies about the utility of anti-oxidant therapy to mitigate Cd toxicity, the current work was undertaken to evaluate any protective role of TRE against immunotoxicities induced in rats by treatment with Cd (here, as soluble cadmium chloride).
Materials and methods
Plant material and preparation of extract
Tunisian radish plants (Raphanus sativus, Brassicaceae) were harvested from the Zeramdine region (Tunisia) in April 2013. These were botanically identified according to the flora of Tunisia (Cuénod, Citation1954), and a voucher specimen was placed in the college herbarium (RS123/1).
Freshly harvested roots and aerial parts of each radish (totaling ≈500 g) were crushed, and the juice extracted through a fine mesh. The extracts were then characterized by the method described previously in Wang et al. (Citation2004). The filtrate was then concentrated under vacuum and the final dry material weighed and then stored at −20 °C until needed for use in the experiments. An average yield from 500 g was ≈28 g extract. In a previous study, it was demonstrated that the radish extracts contained various quantities of isothiocyanates, flavonoids, and other agents that imparted anti-oxidant activity (Ben Salah-Abbès et al., Citation2008b). Those studies indicated that TRE had a high content of flavonoids (quercetin equivalents) and isothiocyanates that reached levels of, respectively, 344.0 [±3.8] and 39.0 [±4.2] µmol/100 mg TRE.
Chemicals
For the treatment regimens, a stock solution of CdCl2 (Sigma, Steinheim, Germany) was prepared in deionized water immediately before each day’s application.
Animals
Wistar male rats (8–9-weeks-old, 180–200 g) were obtained from SIPHAT (Tunis, Tunisia) and housed in groups of six/cage in specific pathogen-free facilities maintained at 25 °C and with a 12-h light/dark cycle. All rats had ad libitum access to water and commercial rodent chow. Animals were cared for under the Tunisian code of practice for the Care and Use of Animals for Scientific Purposes. The Faculty Ethics Committee (a part of the Faculty of Medicine at the University of Monastir) approved all experimental protocols used herein.
Experimental design
For these studies, rats were administered CdCl2 alone or “in combination” with TRE (actually, in sequence Cd and then TRE) daily by oral gavage for 2 weeks. The LD50 of CdCl2 when given orally to rodents was reported as 33–88 mg/kg BW (USAF, Citation1990); thus, the dose of Cd used here was well below one that would induce significant overt toxicities to the hosts. Only the single dose of 2.5 mg CdCl2/kg was used in these studies; apart from the LD50 factor, this dose was selected based on earlier findings from our laboratories (see Abbès et al., Citation2007). Body weights of rats in each group were measured every other day to allow for adjustment of dose preparation; all rats never received a gavage volume greater than 200 µl in the course of the exposures.
To perform the exposures, rats were randomly divided into six groups (n = 10/group) in order to receive by oral gavage: Group 1: control = only distilled water; Group 2: Cd = 2.5 mg CdCl2/kg BW; Group 3: TRE 1 = 5 mg TRE/kg; Group 4: Cd + TRE [2] = 2.5 mg CdCl2/kg and 5 mg TRE/kg; Group 5: Cd + TRE [3] = 2.5 mg CdCl2/kg and 10 mg TRE/kg; and Group 6: Cd + TRE [4] = 2.5 mg CdCl2/kg and 15 mg TRE/kg. We had noted in pilot studies potential protective effects using a dose of 5 mg TRE/kg; as such, we chose to examine TRE doses of 10 and 15 mg/kg to both ascertain if there were dose-related effects from the TRE and to discern if there were any inadvertent toxicities that could be induced by the TRE itself.
One day after the final dosing, all rats were weighed and then euthanized by decapitation while under ether anesthesia. At necropsy, samples of blood, as well as the spleen, thymus, liver and kidneys were collected. Each organ was blot-dried and weighed before undergoing further processing as outlined below.
Measure of tissue sample cadmium (Cd) content
Levels of Cd in each spleen, thymus, liver, and kidney sample were determined by atomic absorption spectrophotometry using a Perkin Elmer 306 system with a Perkin Elmer Intensitron lamp (Norwalk, CT). Representative fractions of each tissue sample collected at necropsy were lyophilized, weighed, and then digested in 2 ml concentrated HNO3 held within pressurized Teflon containers; samples were digested at 160 °C for 3 h. After cooling to room temperature, each sample was diluted with 10 ml deionized water (Congiu et al., Citation2000) and then subjected to analysis. Cd concentrations were expressed in mg Cd/g tissue (dry mass).
Activities of serum marker enzymes
Activities of serum aspartate transaminase (AST), alanine transaminase (ALT) and lactate dehydrogenase (LDH) were assayed spectophotometrically according to standard procedures using commercially available diagnostic kits (Sigma Diagnostics. Ltd., Oakville, ON, Canada).
Measurement of T-lymphocyte sub-types, immunoglobulins, and TNFα/IL-1 in blood
Mononuclear cells were isolated from blood samples as described by Boyum (Citation1968). T-Lymphocyte sub-sets were evaluated by indirect immunofluorescence using CD3+, CD4+, CD8+, and CD56+ antibodies (each R-phycoerythrin-conjugated; all Sigma, St. Louis, MO). Isotype controls (Sigma) were also utilized in parallel to assess any potential non-specific binding of the anti-marker antibodies. In brief, aliquots of 10,000 cells were incubated with 10 µg of each antibody (separately) for 1 h at 37 °C in the dark. Thereafter, the cells were washed with phosphate-buffered saline (PBS, pH 7.4) to remove unbound antibody and the final cell preparations then re-suspended in PBS for application to slides. At least 200 cells per sample were then analyzed using a fluorescence microscope (Nikon Eclipse E 400; Tokyo, Japan); six samples/rat were analyzed for each CD marker. All samples were analyzed in a blinded manner.
Serum total IgG and IgA levels were measured by kinetic nephelometry. This process measures light-scattering species in solution by means of the light intensity at an angle away from the incident light passing through the sample. In the presence of a protein antigen, an antibody reacts with the antigen, and a precipitation reaction begins; measurements are taken early in this precipitation reaction time sequence. A quantitative value is obtained by comparison with a standard curve generated in parallel. For the studies here, all samples were analyzed using a NEPHELOstar Plus laser system (BMG Labtech GmBH, Ortenberg, Germany).
Plasma TNFα and IL-1 were measured using commercial ELISA kits (OptEIATM Kits; BD Biosciences, Heidelberg, Germany), in accordance with manufacturer instructions. Optical density measures were taken using an automated plate reader (Spectra Image; Tecan, Morrisville, NC) at 450 and at 620 nm. All data were expressed in pg/ml. The levels of sensitivity of the kits for TNFα and IL-1 were, respectively, 4.0 and 3.5 pg/ml.
Statistical analysis
Data were analyzed using Stat View 512 + software (Abacus Concept, Inc., Piscataway, NJ). All data are reported as means ± SEM. Differences between controls and treated animal values were analyzed using a Student’s t-test. The level of significance was set at p < 0.05.
Results
Effects of treatments on body and lymphoid organ weights
The effects of TRE alone and in [sequential] combination with Cd on body and lymphoid organ weights are summarized in . The body weight gains were significantly decreased in rats that received Cd alone as compared with those in normal rats; there were no differences between these values for the TRE-only and control rats. Pilot studies with each of the higher TRE doses used here (alone) also indicated no impact on body weight (data not shown). Moreover, relative weights (i.e. organ indices) of the spleen, thymus, and liver were each markedly reduced in Cd-treated rats when compared with those for corresponding organs of the normal rats. For all end-points here, sequential co-treatment with TRE and Cd led to values approximating normal levels. To provide fuller information for the reader, both the absolute body weights over time and the final absolute organ weights can be found in Supplemental .
Table 1. Body weight gain and relative weights of organs of rats exposed daily to CdCl2, TRE, or a TRE + Cd co-treatment for 2 weeks.
Cd content in spleen, thymus, and liver
Organ Cd content after 2 weeks of treatment is seen in . There were clear increases in Cd levels in all three organs tissues in rats given CdCl2. The Cd levels in the kidney tissues were—as expected—higher (25.5 µg Cd/g tissue) compared to control values; Cd levels in the spleen and thymus were 11.2 and 17.2 µg Cd/g, respectively. In contrast, Cd levels in organs or rats given any of the TRE + Cd sequential co-treatments (or TRE alone) were below detection limits.
Effects of treatments on serum biochemical parameters
Serum biochemical analyses revealed that treatment with CdCl2 significantly increased blood levels of LDH, ALT, AST, and urea, and significantly decreased serum levels of creatinine kinase, creatinine, triglycerides, and total protein (). Animals treated with TRE alone had values comparable to control rats for all the serum parameters tested. Sequential co-treatment with Cd and TRE (at all test doses) led to a restoration of serum levels of LDH, ALT, and AST, and a significant amelioration of the Cd-related effect on serum levels of CK, triglycerides, and total protein.
Table 2. Effects of daily treatment with TRE alone or in combination with CdCl2 for 2 weeks on serum biochemical parameters.
Effects of treatments on T-lymphocyte phenotypes
The phenotyping of blood T-cells revealed that treatment with CdCl2 induced significant decreases in CD3+, CD4+, CD8+, and CD56+ cell counts (). Use of non-specific isotype antibodies allowed us to confirm there was no non-specific binding of the individual anti-CD marker antibodies by the cells from the individual hosts. Treatment with TRE alone did not affect any T-cell sub-types levels. Sequential co-treatment with Cd + TRE (at all test doses) resulted in a significant improvement in total T-lymphocyte (and sub-type) levels toward control values, although they were still higher (albeit not significantly so) than control values.
Figure 2. Effect of TRE and Cd alone or in combination on percentages of T-lymphocytes in blood of rats treated with CdCl2 alone or plus TRE with different doses for 14 days. (a) CD8+, CD56+. (b) CD3+, CD4+. Values shown are mean (±SEM). *Value significantly different (p < 0.01) compared to control and all co-treatment rats.
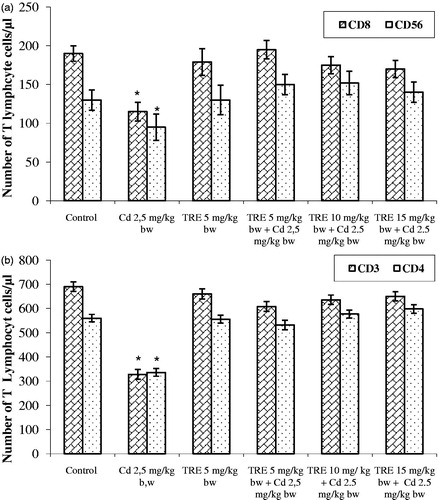
Effects of treatments of immunoglobulin profiles
Treatment with Cd alone caused a significant decrease in both serum IgA and IgG levels (). Treatment with TRE alone did not impact either isotype; use of TRE in sequential co-treatment with Cd led to normalization of the isotype profiles.
Effect of treatments on select pro-inflammatory cytokine (TNFα and IL-1) levels
Treatment with CdCl2 resulted in increases in the levels of circulating TNFα and IL-1 in the rats (changing from 20–25 pg/ml to ≈30–35 pg/ml; ). Rats treated with TRE alone, or that underwent sequential co-treatment with Cd + TRE, had values for each cytokine approximating/not-significantly different from those of the control rats. Sequential co-treatment with Cd + TRE resulted in a significant decrease in these elevated levels by 37 and 45%, respectively, from the TNFα and IL-1 values seen with the Cd-only counterparts.
Discussion
Cadmium (Cd) is an important toxicant due to its ever-increasing presence in the environment as a result of industrial and agricultural practices (Satarug et al., Citation2003). Tunisian radish extract (TRE) has been shown so far to protect against immunotoxicity and oxidative stress induced by various chemicals (Ben Salah-Abbès et al., Citation2008a, Citation2009a,b). This study was undertaken in order to investigate the effect of TRE on Cd toxicity to anti-oxidant enzymes and the immune system of rats.
The results here showed that Cd treatment (daily for 2 weeks, at 2.5 mg CdCl2/kg by gavage) led to significant accumulation of this metal in the spleen, thymus, and liver of treated rats. Lei et al. (Citation2005) reported that exposure of rats to Cd in drinking water increased the level of this metal in their tissues. It is well documented that Cd mainly accumulates in the liver and kidney as these organs contain a large amount of metallothionein, a binding protein with high affinity for Cd (Klaassen et al., Citation1999). The current work demonstrated that CdCl2 treatment resulted in augmentation of Cd content in the thymus, an outcome consistent with the findings of Dong et al. (Citation2001). Importantly, our study showed that Cd content in the organs of the rats sequentially co-exposed with Cd + TRE (at 5, 10, or 15 mg/kg) remained unchanged compared to control rats.
The data here also illustrated that the Cd exposure altered hepatic and renal cell functions in the treated rats. This was evidenced as increases in blood levels of LDH, ALAT, ASAT, and creatinine among the Cd-treated rats, an outcome similar to that reported by Kara et al. (Citation2005) with rats exposed to high Cd levels. Rana et al. (Citation1996) noted that Cd caused alterations in liver transaminases of treated rats. The increase in measured activities of AST and ALT in plasma is mainly due to leakage of these enzymes from liver cells into the blood (Navarro et al., Citation1993); this provides a clear indication of the hepatotoxicity of Cd. The present results also showed that plasma total protein levels were decreased due to the CdCl2. Concurrent with the increases in plasma urea and creatinine levels, these outcomes were illustrative of renal toxicities induced by Cd. The increase in kidney weights seen here could be due to hypertrophy secondary to other nephrotoxicities induced by the CdCl2. In contrast to the liver and kidney measures, there were no serum markers that could be used to reflect CdCl2 splenic toxicity. On its own, the observed increases in spleen weight in Cd-only-treated rats might have been due to excessive deposition of damaged erythrocytes/inflammatory cells in these rats’ spleens (Waalkes et al., Citation1999).
Immunotoxicity was previously seen in animal models that underwent exposure to Cd (Dan et al., Citation2000; Descotes, Citation1992). We observed here that Cd treatments induced significant reductions in T-lymphocyte sub-types in rats, results in keeping with the differential effects on T-lymphocyte sub-sets induced by Cd (and other immunotoxins) seen by Holladay et al. (Citation1991) and Lai et al. (Citation1998). According to Kishimoto et al. (1995), in response to some toxicants, thymocytes begin to up-regulate a number of cell surface markers and concurrently undergo apoptosis. Thymocyte apoptosis has been shown in Cd-treated animals, leading to changes in patterns of thymocyte development and immune cell phenotypes in the body (Dong et al., Citation2001). In the study here, Cd exposures also led to significant reductions in CD4+, CD8+, CD3+, and CD56+ cells in treated rats. Based on the literature, we presume these outcomes reflected an induction of apoptosis among the thymocytes; however, further studies are needed to fully confirm this viewpoint.
The present study also noted that immunoglobulin profiles for circulating IgA and IgG were significantly decreased due to Cd treatments. These outcomes may suggest that Cd also induced damage to host B-lymphocytes (as had been suggested earlier by Dan et al., Citation2000; Schulte et al., Citation1994); however, the changes in Ig could also have arisen from the above-noted altered availability of mature T-lymphocytes, cells critical to propagation of any development/maturation of B-lymphocytes to attain the plasma cell stage. Other studies have attributed immunotoxic properties of Cd to interactions with T- or B-lymphocyte receptors, or an intracellular accumulation of the metal in these cells that, in turn, perturbs signal transduction and causes cell apoptosis or necrosis (Beyersmann & Hechtenberg, Citation1997; Satoh et al., 2003). Other suggested possible mechanisms for Cd-mediated impairment of lymphocyte functions have been modifications of Ca2+-dependent processes (Satoh et al., 2003) or of cell cycle regulation (Zhou et al., Citation2004) or of glutathione content (Nishimura et al., Citation2005). Regardless of the precise mechanism, the changes in Ig levels in the Cd-treated rats illustrate that this metal can perturb not only immune cell (i.e. lymphocyte) numbers but also functionality.
The current studies also showed that exposure to CdCl2 resulted in changes in the release of pro-inflammatory cytokines TNFα and IL-1 in situ. These cytokines are key mediators in the initiating of systemic inflammatory response and are important in the pathogenesis of septic shock (Liaudet et al., Citation2001). Dong et al. (Citation2001) indicated that Cd treatment induced increases in secretion of such cytokines (and other chemokines) by monocytes and Kupffer cells. Because pro-inflammatory cytokines activate inflammatory cells to release large amounts of reactive oxygen species, it is not surprising that Cd exposure is associated with severe oxidant damage (Boujelben et al., Citation2006), even though the metal itself is not redox-active per se.
With ameliorative effects of TRE from Tunisian R. sativus radish the focus of these studies, it was important to ascertain if the preparations inadvertently led to toxicities of their own in the rats. The results here showed that TRE alone did not impact negatively on the test biochemical and immune parameters in rats; previously, Ben Salah-Abbès et al. (Citation2008b) showed that TRE at 15 mg/kg did not impart any toxic effects in mice. With safety of the TRE itself a “non-problem”, the study here then examined whether administration of TRE could alleviate/block the negative effects of Cd on the various study parameters.
The beneficial influences of TRE noted here led us to conclude that one potential by-product from its consumption could be key to the observed findings, i.e. MTBITC. MTBITC is an efficient anti-oxidant and scavenger of oxygen free radicals; its anti-oxidant activity is greater than that seen with ascorbic acid or vitamin E (Suzuki et al., Citation2006). Compositional analyses of the TRE (Ben Salah-Abbès et al., Citation2008b) has revealed that there are significant quantities of several important phenolic agents, such as gallic acid, ferulic acid, isoferulic acid, sinapic acid, methyl ferulate, methylsinapate, and 4-(methylthio)-3-butenyl isothiocyanate (MTBITC), as well as the parent glucosinolate of MTBITC. Considering that gut microflora efficiently convert the glucosinolate to MTBITC (Shapiro et al., Citation2001), it would seem that consumption of radishes (or other cruciferous foods) could allow for significant levels of MTBITC to become present in the consuming host. As an anti-oxidant, this would afford the host increased protection against the oxidative stress/immunotoxicities potentially induced by an agent like Cd or any number of environmental toxicants that impart damage to exposed hosts via formation or reactive oxygen species and similar reactive agents.
In conclusion, the possible mechanism of protection offered by TRE against Cd-induced toxicities here was potentially due to an ability to inhibit oxidative processes initiated by reactive oxygen species that were induced secondary to effects of the Cd on immune/local inflammatory cells. Further investigations are needed to better understand the mechanisms by which TRE exerted efficient prevention of immunotoxicity induced by Cd.
Declaration of interest
The authors report no conflicts of interest. The authors alone are responsible for the content and writing of the paper.
Supplemental Table 1
Download MS Word (124.3 KB)Acknowledgements
The Tunisian Ministry of Scientific Research and Technology (Laboratoire d’Immunologie et Microbiologie Environementale et Cancérologie: IMEC) supported this research. We also wish to thank the work team at the Laboratory of Histology Cytology and Genetics at the University of Monastir, for their help and their technical assistance.
References
- Abbès, S., Ben Salah-Abbès, J., Nahdi, K., et al. (2007). Inactivation of cadmium-induced immune-toxicological alterations in rats by Tunisian montmorillonite clay. Int. Immunopharmacol. 7:750–760
- Banerjee, S., and Flores-Rozas, H. (2005). Cadmium inhibits mismatch repair by blocking the ATPase activity of the MSH2-MSH6 complex. Nucl. Acids Res. 33:1410–1419
- Ben Salah-Abbès, J., Abbès, S., Abdel-Wahhab, M. A., and Oueslati, R. (2009a). Raphanus sativus extract protects against zearalenone-induced reproductive toxicity, oxidative stress and mutagenic alterations in male Balb/c mice. Toxicon 53:525–533
- Ben Salah-Abbès, J., Abbès, S., Haous, Z., et al. (2008b). Zearalenone induces immunotoxicity in mice: Possible protective effects of radish extract (Raphanus sativus). J. Pharm. Pharmacol. 60:761–770
- Ben Salah-Abbès, J., Abbès, S., Haous, Z., and Oueslati, R. (2009b). Raphanus sativus extract prevents and ameliorates zearalenone-induced peroxidative hepatic damage in Balb/c mice. J. Pharm. Pharmacol. 61:1545–1554
- Ben Salah-Abbès, J., Abbès, S., Ouanes, Z., et al. (2008a). Investigation of Tunisian radish extract (Raphanus sativus) as anti-oxidants and radical scavenging properties against Zeralenone toxicity in Balb/c mice. J. Appl. Toxicol. 28:6–14
- Beyersmann, D., and Hechtenberg, S. (1997). Cadmium, gene regulation, and cellular signaling in mammalian cells. Toxicol. Appl. Pharmacol. 144:247–261
- Blakley, B. R. (1985). The effect of CdCl2 on immune response in mice. Can. J. Comp. Med. 49:104–108
- Boujelben, M., Ghorbel, F., Vincent, C., et al. (2006). Lipid peroxidation and HSP72/73 expression in rat following cadmium chloride administration: Interactions of magnesium supplementation. Exp. Toxicol. Pathol. 57:437–443
- Boyum, A. (1968). Isolation of mononuclear cells and granulocytes from human blood. Isolation of mononuclear cells by one centrifugation, and of granulocytes by combining centrifugation and sedimentation of Ig. Scand. J. Clin. Lab. Invest. 21:98–100
- Cai, Y., Aoshima, K., Katoh, T., et al. (2001). Renal tubular dysfunction in male inhabitants of a cadmium-polluted area in Toyama, Japan - an eleven-year follow-up study. J. Epidemiol. 11:180–189
- Cifone, M. G., Alesse, E., di Eugenio, R., et al. (1989). In vivo Cd treatment alters natural killer activity and large granular lymphocyte number in the rat. Immunopharmacology 18:149–156
- Congiu, L., Chicca, M., Pilastro, A., et al. (2000). Effects of chronic dietary cadmium on hepatic glutathione levels and glutathione peroxidase activity in starlings (Sturnus vulgaris). Arch. Environ. Contam. Toxicol. 38:357–361
- Cuénod, A. (1954). Flore de la Tunisie: cryptogames vasculaires, gymnospermes et monocotylé-dones (Efarabi, Ed.). Tunis: Office de l’expérimentation de la vulgarisation agricoles de Tunisie, pp. 126
- Dan, G., Lall, S. B., and Rao, D. N. (2000). Humoral and cell-mediated immune response to cadmium in mice. Drug Chem. Toxicol. 23:349–360
- Descotes, J. (1992). Immunotoxicology of cadmium. IARC Sci. Publ. 118:385–390
- Dong, S. Y., Shen, H. M., and Ong, C. N. (2001). Cadmium-induced apoptosis and phenotypic changes in mouse thymocytes. Mol. Cell. Biochem. 222:11–20
- Esaki, H., and Onozaki, H. (1982). Antimicrobial action of pungent principles in radish root. Eiyo To Shokuryo. 35:207–211
- Exon, J. H., South, E. H., and Hendrix, K. (1996). Effects of metals on the humoral immune response. In: Toxicology of Metals (Chang, L. W., Ed.). Boca Raton, FL: CRC Lewis, pp. 798–801
- Fang, Y. Z., Yang, S., and Wu, G. (2002). Free radicals, anti-oxidants and nutrition. Nutrition 18:872–877
- FAO/WHO. (2003). Summary and Conclusions of the 61st Meeting on Food Additives and Contaminants. Rome, 10-19 June (2003). Available online at: fttp://ftp.fao.org/es/esn/jecfa/jecfa61sc.pdf
- Gill, C. I., Haldar, S., Porter, S., et al. (2004). The effect of cruciferous and leguminous sprouts on genotoxicity, in vitro and in vivo. Cancer Epidemiol. Biomarkers Prev. 13:1199–1205
- Gupta Sen, R., Gupta Sen, E., Dhakal, B. K., et al. (2004b). Vitamin C and vitamin E protect rat testes from Cd-induced reactive oxygen species. Mol. Cells 17:132–137
- Gupta Sen, R., Kim, J., Gomes, C., et al. (2004a). Effect of ascorbic acid supplementation on testicular steroidogenesis and germ cell death in cadmium-treated male rats. Mol. Cell. Endocrinol. 221:57–65
- Hashem, F. A., and Saleh, M. M. (1999). Anti-microbial components of some Cruciferae plants. Phytother. Res. 13:329–332
- Hashimoto, T., Ueda, Y., Oi, N., et al. (2006). Effect of combined administration of quercetin, rutin, and extract of White Radish sprout rich in campherol glucosides on the metabolism in rats. Biosci. Adv. Biochem. Eng. Biotechnol. 70:279–281
- Hecht, S. S. (1999). Chemoprevention of cancer by isothiocyanates, modifiers of carcinogen metabolism. J. Nutr. 129:768S–774S
- Holladay, S. D., Lindstrom, P., Blaylock, B. L., et al. (1991). Perinatal thymocyte antigen expression and postnatal immune development altered by gestational exposure to tetrachlorodibenzo-p-dioxin (TCDD). Teratology 44:385–392
- Horiguchi, H., Harada, A., Oguma, E., et al. (2000). Cadmium-induced acute hepatic injury is exacerbated in human IL-8 transgenic mice. Toxicol. Appl. Pharmacol. 163:231–239
- Hovland, J., Cantor, R. M., Lee, G. S., et al. (2000). Identification of a murine locus conveying susceptibility to cadmium-induced forelimb malformations. Genomics 63:193–201
- Jeffery, E. H., and Jarrell, V. (2001). Cruciferous vegetables and cancer prevention. In: Handbook of Nutraceuticals and Functional Foods, (Wildman, R. E., Ed.). Boca Raton, FL: CRC Press, pp. 169–192
- Kapoor. L. D. (Ed.). (1990). Handbook of Ayurvedic Medicinal Plants. Boca Raton, FL: CRC Press
- Kara, H., Karatas, F., Canatan, H., and Servi, K. (2005). Effects of exogenous metallothionein on acute cadmium toxicity in rats. Biol Trace. Elem. Res. 104:223–232
- Katsuzaki, H., Miyahara, Y., Ota, M., et al. (2004). Chemistry and anti-oxidative activity of hot water extract of Japanese radish (daikon). Biofactors 21:211–214
- Kishimoto, H., Surh, C.D., and Sprent J. (1995). Upregulation of surface markers on dying thymocytes. J. Exp. Med. 181:649–655
- Klaassen, C. D., Liu, S., and Choudhuti, S. (1999). Metallothionein: An intracellular protein to protect against cadmium toxicity. Ann. Rev. Pharmacol. Toxicol. 39:267–294
- Koller, L. D. (1998). Cadmium. In: Immunotoxicology of Environmental and Occupational Metals (Zelikoff, J. T., and Thomas, P. T., Eds.). London: Taylor & Francis, pp. 41–61
- Lai, Z. W., Fiore, N. C., Gasiewicz, T. A., and Silverstone, A. E. (1998). 2,3,7,8-Tetrachloro-dibenzo-p-dioxin and diethylstilbestrol affect thymocytes at different stages of development in fetal thymus organ culture. Toxicol. Appl. Pharmacol. 149:167–177
- Lei, L. J., Jin, T. Y., and Zhou, Y. F. (2005). Effects of cadmium on levels of insulin in rats. Wei Sheng Yan Jiu. 34:394–396
- Liaudet, L., Mabley, J. G., Soriano, F. G., et al. (2001). Inosine reduces systemic inflammation and improves survival in septic shock induced by cecal ligation and puncture. Am. J. Respir. Crit. Care Med. 164:1213–1220
- Lugasi, A., Dwoeschak, E., Blazovics, A., and Kery, A. (2001). Antioxidant and free radical scavenging properties of squeezed juice from black radish (Raphanus sativus L. var niger) root. Phytother Res. 12:502–506
- Marth, E., Barth, S., and Jelavcan, S. (2000). Influence of Cd on the immune system description of stimulating reactions. Cent. Eur. Public Health 8:40–44
- Marth, E., Jelovcan, S., Kleinhappl, B., et al. (2001). The effect of heavy metal on the immune system at low concentrations. Int. J. Occup. Med. Environ. Health 14:375–386
- Nadkarni, K. M. (1976). Indian materia medica. Bombay: Popular Prakashan, pp. 35–49
- Nasr, Y. A., Hemdan, F. E., and Ulrich, S., et al. (2006). The in vitro immune modulation by cadmium depends on the way of cell activation. Toxicology 222:37–45
- Navarro, C. M., Montilla, P. M., Martin, A., et al. (1993). Free radicals scavenger and anti-hepatotoxic activity of Rosmarinus. Plant Med. 59:312–314
- Nishimura, Y., Yamaguchi, J. Y., Kanada, A., et al. (2005). Increase in intracellular Cd2+ concentration of rat cerebellar granule neurons incubated with cadmium chloride: Cadmium cytotoxicity under external Ca-free condition. Toxicol. In Vitro 20:211–216
- Othumpangat, S., Kashon, M., and Joseph, P. (2005). Eukaryotic translation initiation factor 4E is a cellular target for toxicity and death due to exposure to cadmium chloride. J. Biol. Chem. 280:25162–25169
- Payette, Y., Lachapelle, M., Daniel, C., et al. (1995). Decreased IL-2 receptor and cell cycle changes in murine lymphocytes exposed in vitro to low doses of cadmium chloride. Int. J. Immunopharmacol. 17:235–246
- Prestera, T., Zhang, Y., Spencer, S. R., et al. (1993). Electrophile counterattack response: Protection against neoplasia and toxicity. Adv. Enzyme Regul. 33:281–296
- Rana, S. V., Rekha, S., and Seema, V. (1996). Protective effects of few antioxidants on liver function in rats treated with cadmium and mercury. Indian J. Exp. Biol. 34:177–179
- Satarug, S., Baker, J. R., Urbenjapol, S., et al. (2003). A global perspective on cadmium pollution and toxicity in non-occupationally exposed population. Toxicol. Lett. 137:65–83
- Satoh, M., Kaji, T., and Tohyama C. (2003). Low dose exposure to cadmium and its health effects. Part 3. Toxicity in laboratory animals and cultured cells. Nippon Eiseigaku Zasshi 57:615–623
- Schulte, S., Mengel, K., Gatke, U., and Friedberg, K. D. (1994). No influence of cadmium on the production of specific antibodies in mice. Toxicology 93:263–268
- Shapiro, T. A., Fahey, J. W., Wade, K. L., et al. (2001). Chemo-protective glucosinolates and isothiocyanates of broccoli sprouts metabolism and excretion in humans. Cancer Epidemiol. Biomarkers Prev. 10:501–508
- Sipos, P., Hagymasi, K., Lugasi, A., et al. (2002). Effects of black radish root (Raphanus sativus L. var niger) on the colon mucosa in rats fed a fat rich diet. Phytother. Res. 16:677–679
- Stoeppler, M. (1991). Cadmium. In: Metals and Their Compounds in the Environment, (Merian, E., Ed.). New York: VCH, pp. 803–851
- Stohs, S. J., Bagchi, D., Hassoun, E., and Bagchi, M. (2001). Oxidative mechanisms in toxicity of chromium and cadmium ions. J. Environ. Pathol. Toxicol. Oncol. 20:77–83
- Suh, S. J., Moon, S. K., and Kim, C. H. (2006). Raphanus sativus and its isothiocyanates inhibit vascular smooth muscle cells proliferation and induce G1 cell cycle. Int. Immunopharmacol. 68:854–861
- Suzuki, C., Ohnishi-Kameyama, M., Sasaki, K., et al. (2006). Behavior of glucosinolates in pickling cruciferous vegetables J. Agric. Food Chem. 54:9430–9436
- Takaya, Y., Kondo, Y., Furukawa, T., and Niwa, M. (2003). Anti-oxidant constituents of radish sprout (Kaiware-daikon), Raphanus sativus L. J. Agric. Food Chem. 51:8061–8066
- USAF (United States Air Force). (1990). Cadmium. In: Installation Restoration Program Toxicology Guide, Vol. 5. Wright Patterson AFB, OH: Harry G. Armstrong Aerospace Medical Research Laboratory
- Waalkes, M. P., Anver, M. R., and Diwan, B. A. (1999). Chronic toxic and carcinogenic effects of oral cadmium in the Noble (NBL/Cr) rat: Induction of neoplastic and proliferative lesions of adrenal, kidney, prostate, and testes. J. Toxicol. Environ. Health 58:199–214
- Wang, L., Burhenne, K., Kristensen, B. K., and Rasmussen, S. K. (2004). Purification and cloning of a Chinese red radish peroxidase that metabolize pelargonidin and forms a gene family in Brassicaceae. Gene 343:323–335
- Yiin, S. J., Chern, C. L., Sheu, J. Y., and Lin, T. H. (1999). Cadmium-induced lipid peroxidation in rat testes and protection by selenium. Biometals 12:353–357
- Yoshiro, S., Yasukazu, Y., Takashi, A., et al. (2003). Cell death caused by selenium deficiency and protective effect of anti-oxidants. J. Biol. Chem. 278:39428–39433
- Zelikoff, J. T., Bowser, D., Squibb, K. S., and Frenkel, K. (1995). Immunotoxicity of low level Cd exposure in fish: An alternative animal model for immunotoxicological studies. J. Toxicol. Environ. Health 45:235–248
- Zhou, T., Jia, X., Chapin, R. E., et al. (2004). Cadmium at a non-toxic dose alters gene expression in mouse testes. Toxicol. Lett. 154:191–200