Abstract
The present work sought to investigate potential suppressive effects on mouse macrophages by in vitro treatment with clove (Syzygium aromaticum) ethanolic extracted essential oil (containing eugenol) or its water-soluble extract. Using doses (ranging from 0.001–1000 µg/ml) of each material freshly prepared in the laboratory, cell survival and production of nitric oxide (NO), tumor necrosis factor (TNF)-α, interleukin (IL)-6, and IL-12 by the treated cells (that in all cases also had received LPS stimulation) were measured. Results indicated that, except at doses ≥100 µg/ml, viability was unaffected in all groups. NO release by LPS-stimulated macrophages was generally significantly suppressed by either material; in contrast, low (i.e. 0.001–1 µg/ml) doses of either extract class appeared to enhance NO release by non-LPS (unstimulated)-treated macrophages. Among LPS-stimulated cells, TNFα release was also significantly affected by each extract; the ethanolic extract was suppressive at all doses tested, while the aqueous material was so up to 1 µg/ml and then became stimulatory. In contrast, nearly every dose of either extract appeared to stimulate IL-6 release from the LPS-treated cells. Effects on IL-12 production were overall inconsistent; in general, the ethanolic extract tended to be stimulatory of production by the LPS-treated cells. The data for the aqueous material showed no discernable pattern of effect. The results suggest that clove extracts do not have a distinct cytotoxic activity, but do impart potential anti- and pro-oxidant effects in cells, depending on their concentrations and on the activation state of the macrophages themselves at the time of exposure to the extracts. The impact of the extracts on macrophage cytokine release also displays a pattern of dose-relatedness.
Introduction
Herbal medicine is used to treat different diseases in many of the world cultures. Identification of the active components and mechanisms of action of traditional medicines on the immune system are highly desirable (Sun et al., Citation2006). The immune system divides to adaptive and innate systems and the adaptive immune system divides to cellular and humeral responses. Macrophages play significant roles in inflammation and host defense. Multiple molecular mechanisms mediate inflammatory processes, such as nitric oxide (NO), prostaglandins, leuko-trienes, reactive oxygen species, and some secreted cytokines (Moncada, Citation1999). Macrophage mediators mediates diverse physiological functions, such as non-specific host defense, anti-microbial defense, and anti-tumor activities, as well as pathophysiological functions, which include pathogeneses like septic shock and organ destruction in certain inflammatory and auto-immune diseases (Petros et al., 1991). Agents that can impact on formation/release of these mediators could, in turn, affect these different phenomena; these could include various stimuli, e.g. natural mediators, drugs, herbal medicines.
Eugenia caryophyllata L. Merr. & Perry (syn.: Syzygium aromaticum) (clove) plant belongs to the Myrtaceae families. It is grown naturally in Indonesia and cultivated in many parts of the world, including Iran (Apparecido et al., 2009). It is a well-known food flavor and also a popular remedy used for dental disorders, respiratory disorders, headaches, and sore throats in traditional medicines in Australia and several Asian countries (Domaracky et al., Citation2007). In addition to its anti-microbial, anti-fungal, and anti-viral properties, clove possesses anti-inflammatory, cytotoxic, and anesthetic properties (Chaieb et al., Citation2007). It has been reported that constituents of clove impart anti-oxidant activities and inhibit lipid peroxidation (Ko et al., Citation1995).
The major ingredients of clove have been shown to be eugenol (50–87%), eugenyl acetate, tanene, thymol, and β-cariophyllene (El-Ghorab & El-Massry, 2003). These components have been shown to modulate many of the aforementioned effects as well as some immune responses, although the mechanisms of effect remain unclear (Choi et al., Citation2007). It is clear that more than eugenol is extracted when clove is processed; there are other water-soluble ingredients that could also have important toxicologic functions.
While some biologic effects from exposure to clove have been reported (and noted above), apart from the above-noted anti-inflammatory effects, effects by clove or key constituents (like eugenol) on specific immune system components/mechanisms have only recently begun to be examined in detail (Bachiega et al., Citation2012; Grespan et al., Citation2012; Lee et al., Citation2007; Rodrigues et al., Citation2009; Yogalakshmi et al., Citation2010). As many of those studies have often yielded conflicting results, we undertook a series of studies that would hopefully provide some clarity regarding immunotoxicity/immunomodulatory effects of clove-associated materials.
The work here examined in vitro effects on peritoneal macrophages arising from treatment with clove essential oils (containing mostly eugenol, obtained by ethanol extraction) or an aqueous extract (also containing eugenol) of the plant. Specifically, effects of each preparation on cell viability, nitric oxide (NO) production, and pro-inflammatory cytokine secretion (e.g., tumor necrosis factor [-]-α, interleukin [IL]-12, and IL-6) were examined in macrophages isolated from naïve mice that were then treated in vitro with lipopolysaccharide and the extracts—or received medium only (non-stimulated) and the extract (the latter scenario in order to assess background direct impact of the extracts on the cells).
Materials and methods
Animals
Balb/c mice (female, 8–10-weeks-old) were purchased from the Pasteur Institute of Iran (Tehran, Iran). All mice were kept singly in separate standard cages and maintained under in a pathogen-free facility maintained at 22 °C with a 12-h light:dark cycle. All mice had ad libitum access to standard rodent chow and filtered water. The Tarbiat Modares University Ethical Committee for Animal Care and Use approved all aspects of this study.
Preparation of plant materials
Flower buds were collected from clove plants cultivated in the Center of Medicinal Plants Research (Tehran, Iran), and confirmed by the Center of Agricultural Research (Tehran). Extraction of eugenol (in the ethanolic extract bearing clove essential oil) was done using the method of Myint et al. (1995a,b). In brief, the buds were dried in the sun and ground to fine powder with the aid of an electric blender. Thereafter, 25 g of the milled clove powder was soaked in 100 ml distilled water to prepare an aqueous extract, or in 100 ml ethanol to prepare an ethanol extract. Each solution was allowed to mix on a rotary system for 24 h before being filtered through Whatman No. 1 filter paper. Each filtrate extract was poured into a special lyophilizing flask that was in turn connected to a vacuum pump. The samples were then processed until dry. The isolates were each recovered, weighed, and representative stocks of 100 mg/ml prepared in 5 ml of, accordingly, water or 50% ethanol for use in the various assays herein. Yields for both materials were routinely 2–3 g (8–12% [w/w] starting material).
Analyses of extract contents
Previous reports indicated that clove oil contained eugenol (∼75%), β-caryophyllene (≈5%), eugenyl acetate (≈16%), and other components <1% (Chaieb et al., Citation2007); analyses of the aqueous extracts showed it contained 49% eugenol (Lee et al., Citation2009). The extracts in the current study were assessed using the same GC-MS methods as that of Lee et al. (Citation2009); the data showed eugenol made up 74% of material in the ethanolic extract and 43% in aqueous extract. Although we did not assess directly the levels of β-caryophyllene and eugenyl acetate, we assume these are in line with the values reported in both Chaieb et al. (Citation2007) and Lee et al. (Citation2009) and, thus, comprise the bulk of the remaining materials in each extract.
Macrophage culture and stimulation
Peritoneal macrophages were isolated from healthy naïve by peritoneal cavity lavage with 10 ml cold RPMI 1640 (Sigma, St. Louis, MO). The cells recovered were then centrifuged at 300 × g for 10 min and then adjusted to 1.5 × 106 cells/ml RPMI medium [now supplemented with 11 mM NaHCO3, 2 mM L-glutamine, 100 U penicillin/ml, 100 μg streptomycin/ml and 5% fetal bovine serum (all reagents from Gibco, Grand Island, NY)]. Aliquots containing 3 × 105 cell suspensions were plated (at 200 μl/well) into 96-well flat bottomed plates (Nunc, Kamstrup, Denmark) and incubated for 4 h in a humidified atmosphere with 5% CO2 at 37 °C to permit adherence. Non-adherent cells were removed by washing the wells three times with phosphate-buffered saline (PBS, pH 7.2). Adherent peritoneal macrophages then received complete RPMI medium; for stimulation, some wells received RPMI containing 10 μg/ml LPS (lipopolysac-charide, Type 0111:B4 from Escherichia coli [Sigma, St. Louis, MO]). To both populations of cells, different concentrations of clove extracts (final concentrations of 0.001–1000 μg/ml) were then immediately added and the plates were incubated for 48 h at 37 °C and under 5% CO2. After this period, the cells were examined for viability and for production (i.e. accumulated) of various cell products as outlined below.
Assessment of cell viability
Macrophage viability was evaluated using an MTT [3-(4,5-dimethylthiazol-2-yl)-2,5-di-phenyltetrazolium bromide] reduction assay indicative of cellular metabolic activity (Sladowski et al., Citation1993). At the end of the 48-h incubation, MTT (20 µl, at 5 mg/ml in PBS) was added to each well and the plate incubated for 4 h at 37 °C. After incubation, culture supernatants were gently removed and 100 μl acidic isopropanol (0.04 M HCl in isopropanol) was added in order to dissolve the formazan crystals generated within the viable cells. Absorbance in each well was then assessed at 540 nm using a Multiskan plate reader (Thermo Scientific Vantaa, Finland). All results were expressed as Survival Index (SI) calculated as the OD540 of test cultures/OD540 of control wells that contained cells treated with medium only.
Measurement of nitric oxide (NO) levels
Nitric oxide production by the cells was measured using the method of Stuehr & Nathan (Citation1989). At the end of the 48-h incubation, nitrite levels in the well supernatants were measured as an indicator of NO production, using the Griess reaction [1% sulfanilamide/0.1% N-(1-naph-thyl) ethylenediamine dihydrochloride/2.5% H3PO4] (Merck, Darmstadt, Germany). In brief, 50 μl test solution (culture supernatant) was combined with 50 μl Griess reagent in a 96-well flat-bottomed plate (in triplicate). After 15 min at room temperature, the absorbance in each well was measured in the Multiskan reader (at 540 nm). The nitrite concentration in each sample was then extrapolated from a standard curve generated in parallel using stock solutions of sodium nitrite (Scuro et al., Citation2004).
Cytokine measurements
Cytokines levels (TNFα, IL-6, and IL-12) in the culture supernatants were determined using commercially available ELISA kit (eBiosciences, Frankfurt, Germany), according to manufacturer instructions. All samples were measured at least in duplicate. The limits of sensitivity of the kits were 3.7 pg TNFα/ml, 6.5 pg IL-6/ml, and 10 pg IL-12/ml. Among the myriad of macrophage-produced cytokines possible to assess, these three were selected for analyses so as to examine the impact of the treatments on the macrophage ability to affect a broad range of critical immune response functions, i.e., taken together the three cytokines here play critical roles in acute phase reactions, inflammation, immune response initiation, T-cell differentiation, and/or maintenance of the T-helper (TH)-1/TH2 response balance.
Statistical analysis
All results were expressed as a mean ± SD. Statistical analysis was performed using a one-way analysis of variance (ANOVA) and an LSD test. p Values <0.05 were considered indicative of a significant difference.
Results
Cell viability
Cytotoxicity of clove extracts on macrophages was determined by MTT assay. MTT reduction capability was the same in all groups, except higher doses of extracts (); 1000 µg/ml of each extract yielded roughly equivalent levels of survival, irrespective of the co-presence of LPS, i.e. 55% and 65% for the aqueous and ethanolic extracts, respectively. In contrast, 100 µg/ml ethanolic extract caused a slightly greater reduction in viability compared to the higher dose and, at this level, the aqueous material imparted no significant effect on cell survival (again in each case, irrespective of a co-presence of LPS).
Figure 1. MTT reduction by peritoneal macrophages treated with various levels of clove extract for 48 h. C(A) = Clove (essential oil containing eugenol; ethanolic extract); C(Aq) = Clove (aqueous extract). Values shown are mean ± SD (triplicate samples per dose). *Value significantly different from corresponding control (i.e. with or without LPS) at p < 0.05.
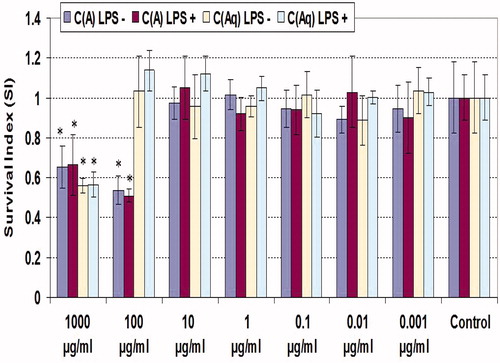
Nitric oxide formation
NO release by macrophages was determined using Griess reagent ( and ). The results overall show that NO release by LPS-stimulated macrophages was generally significantly (p < 0.05) suppressed by either material. NO release by the non-LPS- and LPS-treated cells averaged, respectively, 6.5 and 14.5 µM. In the case of the ethanolic extracts, with LPS-treated cells, this value never exceeded 10 µM; the effects from the aqueous forms were less austere and several doses yielded outcomes not significantly different from the extract-free LPS-treated counterpart. In contrast to what was seen with the LPS-treated cells, low (i.e. 0.001–1 µg/ml) doses of either extract class appeared to enhance NO release by the naïve (non-LPS-co-treated) macrophages With the ethanolic extract, there was no clear dose-related pattern of this effect, and the maximum amplification was ∼2-fold (at 0.1 µg/ml). With the aqueous extract, there was an inverse dose-related pattern of effect, and the maximum amplification was ∼5-fold at the lowest dose tested, i.e. 0.001 µg/ml. Interestingly, with both classes of extract, the dose of 100 µg/ml was an inflection dose, as the next highest dose tested (1000 µg/ml) caused a reversal/rebounding of NO formation by the cells; this was even more so the case with cells that either did or did not receive the LPS co-treatment.
Figure 2. NO production by unstimulated and LPS-stimulated peritoneal macrophages treated with various concentrations of clove ethanolic extract for 48 h. C(A) = Clove (essential oil containing eugenol; ethanolic extract). Values shown are mean ± SD (triplicate samples per dose). *Value significantly different from corresponding control (i.e. with or without LPS) at p < 0.05.
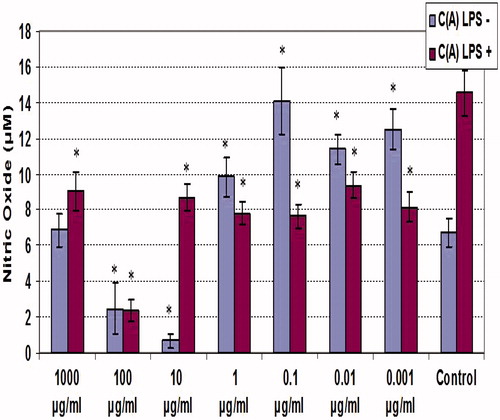
Figure 3. NO production by unstimulated and LPS-stimulated peritoneal macrophages treated with various concentrations of clove aqueous extract for 48 h. C(Aq) = Clove (aqueous extract). Values shown are mean ± SD (triplicate samples per dose). *Value significantly different from corresponding control (i.e. with or without LPS) at p < 0.05.
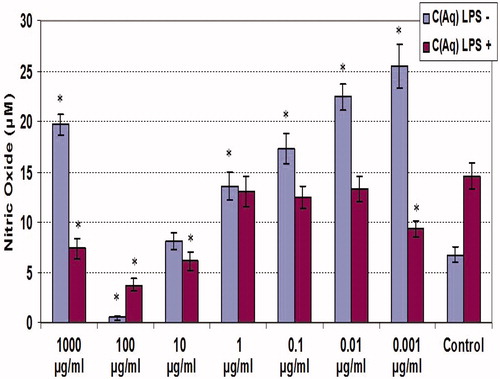
Inducible cytokine formation
Cytokine measures of the culture supernatants of LPS co-treated macrophages revealed differential effects from the two types of extracts. Among the LPS-stimulated cells, TNFα release was significantly affected by each extract (); the ethanolic extract was suppressive at all doses tested, while the aqueous material was inhibitory up to 1 µg/ml and then became stimulatory. The lowest dose of the ethanolic extract (0.001 µg/ml) caused a nearly 8-fold drop in TNFα production, while the dose of 1000 µg/ml caused near complete abrogation. For the aqueous extract, there was a near-complete blocking of TNFα release by the cells when doses used ranged from 0.001–1 µg/ml. Oddly, TNFα formation jumped to ∼4.5-fold above background when the dose crossed over from 1 µg/ml to 10 µg/ml. This enhancement waned as the dose used increased, but was still ∼3-fold over background at 1000 µg/ml.
Figure 4. TNFα production by LPS-stimulated peritoneal macrophages co-treated with various concentrations of clove ethanolic or aqueous extracts for 48 h. (a) Ethanolic extract. (b) Aqueous extract. Values shown are mean ± SD (triplicate samples per dose). *Value significantly different from corresponding control (i.e. with LPS) at p < 0.05.
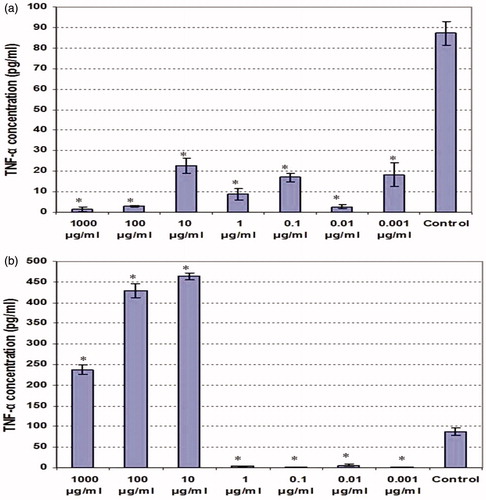
In contrast, nearly every dose of either extract appeared to stimulate IL-6 release from the LPS-treated cells (). Over the dose range of 0.001–100 µg/ml ethanolic extract, the degree of IL-6 formation by the stimulated cells was enhanced 1.83–1.96-fold over background. Only at 1000 µg/ml did the level of formation re-attain background levels. With respect to the aqueous extract, the effects on IL-6 release were moreover similar to those on TNFα, except for the fact that in this case many of the lower doses were stimulatory until the 10 µg/ml dose, at which production increased to almost 10-fold above background (and then again decreased thereafter as the dose rose to 1000 µg/ml).
Figure 5. IL-6 production by LPS-stimulated peritoneal macrophages co-treated with various concentrations of clove ethanolic or aqueous extracts for 48 h. (a) Ethanolic extract. (b) Aqueous extract. Values shown are mean ± SD (triplicate samples per dose). * Value significantly different from corresponding control (i.e. with LPS) at p < 0.05.
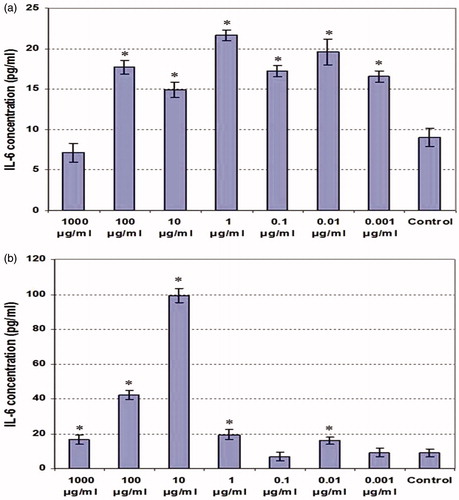
Effects on IL-12 production were overall inconsistent (); in general, the ethanolic extract tended to be stimulatory of production by the LPS-treated cells. The data for the aqueous material showed no discernable pattern of effect.
Figure 6. IL-12 production by LPS-stimulated peritoneal macrophages co-treated with various concentrations of clove ethanolic or aqueous extracts for 48 h. (a) Ethanolic extract. (b) Aqueous extract. Values shown are mean ± SD (triplicate samples per dose). *Value significantly different from corresponding control (i.e. with LPS) at p < 0.05.
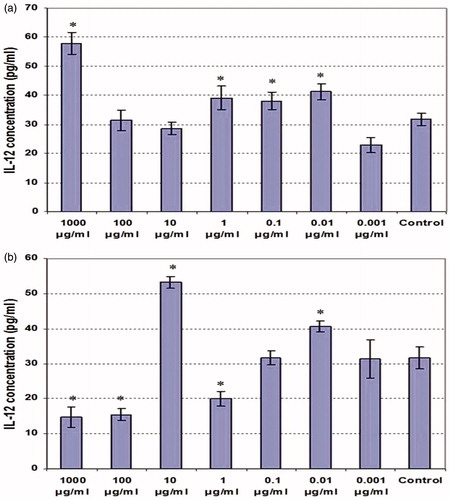
Discussion
This study evaluated effects of an ethanolic (rich in eugenol) and an aqueous extract of clove on macrophages. Evaluation of cytotoxicity showed that each extract only was cytotoxic at high concentrations (100–1000 μg/ml). In comparison to the macrophages here, a study using fibroblasts exposed to clove oil showed the product was cytotoxic at concentrations as low as 0.03% (v/v) and that eugenol was the main effector molecule in this phenomenon (Prashar et al., Citation2006).
Some previous studies have noted that phenolic components in clove may be responsible for the observed anti-oxidant activities of clove oil preparations. One study showed that dichloro-methane and ethanol extracts of clove, as well as flavonoids isolated from clove buds, acted as strong free radical scavengers (in comparison with commercial anti-oxidants like BHT) (Nassar et al., Citation2007). That particular article also suggested that the anti-oxidant activity of clove extract might be due to high concentrations of phenolic compounds like eugenol, eugenol acetate, and thymol (Nassar et al., Citation2007). A study on isoeugenol and its analogs (eugenol and allylbenzene) constituents showed that inhibition of NO production and iNOS expression occurred in a dose-dependent manner in LPS-stimulated RAW 264.7 murine macrophages that were also treated with the test materials (Choi et al., Citation2007). In light of these earlier findings, it was no surprise that the evaluation here of nitric oxide production by treated LPS-stimulated peritoneal macrophages revealed an inhibitory effect from both extracts upon the activated macrophages.
It was interesting to note that, in the absence of LPS, the peritoneal macrophages appeared to be stimulated by the materials in both extracts – but only at relatively low dose levels (i.e. < 1 µg/ml). In contrast, as component concentrations increased, not only was the effect lost, but the cells were actually inhibited (relative to control stimulated cells) with regard to NO formation and/or release. While not a study that measured NO formation as an endpoint, work by Atsumi et al. (Citation2005) noted that reactive oxygen species (ROS) production by macrophages was increased by treatment with low concentrations of eugenol, but decreased when high levels were used. Thus, the results here are in keeping with expected effects from materials that are relatively rich in eugenol and, accordingly, display concentration-related effects on test cells.
Unlike with the NO, extract effects on macrophage cytokine formation were inconsistent. In general, the results indicated the ethanolic extract containing the larger quantity of eugenol suppressed TNFα production, had little effect on IL-12 production (except at very highest dose tested, i.e. 1000 µg/ml), and was mostly stimulatory for IL-6 formation by the macrophages. In comparison, the aqueous extract material imparted the same pattern of effects, but the impact from doses >10 µg/ml were vastly greater than that by the ethanolic extract. In fact, at these doses, the aqueous material became stimulatory for TNFα formation—a complete switch from the overall pattern of inhibition noted with the lower doses. Effects on IL-12 production, again, were simply inconsistent to allow clear conclusions to be reached.
In the studies with the ethanol extract, the lack of any concurrent decrease in IL-12 formation while IL-6 production was stimulated was somewhat unexpected given that IL-6 is known to down-regulate IL-12 formation, especially by LPS-stimulated macrophages (La Flamme et al., Citation2000; Takenaka et al., Citation1997). On the other hand, since NO can auto-regulate (downward) LPS-induced formation of IL-6 (see Persoons et al., Citation1996), the trend toward decreases in NO release concurrent with increased IL-6 levels in the culture supernatants is in keeping with the literature reports on macrophage reactions to NO. Nevertheless, it is important to note for the reader that, because peritoneal macrophages are distinct from other types of macrophages in the body, it is not certain that the auto-regulating effects from NO/cytokines reported in the literature are as prevalent/potent in these particular cells.
In general, our findings here appear to contrast with those from an in vitro study by Bachiega et al. (Citation2012) that showed a presence of clove oil could inhibit IL-1β, IL-6, and IL-10 production by LPS-stimulated macrophages after a 24-h culture. In contrast, an in vivo study by Grespan et al. (Citation2012) indicated that eugenol treatment resulted in enhanced IL-10 cytokine production by cells at sites of inflammation in a murine model of collagen-induced arthritis. The results regarding effects on TNFα formation/release here were certainly more in line with several other studies assessing effects of clove/related products on macrophages. For example, eugenol administration caused significant reductions in TNFα, interferon (IFN)-γ, and transforming growth factor (TGF)-β levels in vivo in mice. In vivo, treatment with clove/eugenol reduced the ability of mice exposed to thioacetamide (and subsequently undergoing hepatic damage) to produce/release TNFα into their systemic circulation (Yogalakshmi et al., Citation2010). Based on in vitro and in vivo studies of the effects of clove on pro-inflammatory cytokine production by macrophages, Rodrigues et al. (Citation2009) claimed eugenol was the causative agent for the particular changes in cytokine formation by cells cultured for 24 h. Those results, like those in the present study, built on evidence from Lee et al. (Citation2007) showing that eugenol suppressed production of LPS-induced TNFα formation by phagocytes treated in culture for 20 h.
That our present results are in line with some earlier reports (re: TNFα), in contrast to some (re: IL-6), illustrate the problems inherent to assessing/defining clearly effects of clove and its related constituents on macrophages and, thus, on the overall immune response. We realize that accumulative cytokine production values were assessed at a single timepoint; as such, it is plausible that some differences may or may not have been captured, as not all of the cytokines assayed share actual ‘peak response’ timepoints.
At this point, attempts to assign mechanisms of action of the materials, in particular eugenol, are a risky endeavor until clear uniform patterns of effect are achieved in our laboratory or of other investigators. Thus, the results of the present study should be considered observations to be assimilated into the growing pool of data/studies on immunotoxicologic effects of clove and its essential oils.
Conclusions
This study demonstrated effects of an ethanolic (rich in eugenol) and aqueous extract of clove on macrophages. Cytotoxic effects of each extract were seen only at high (≥100 µg/ml) concentrations. Nitric oxide (NO) production by LPS-stimulated cells was suppressed by both extracts—an outcome likely associated with the anti-oxidant activity of clove and some of its individual constituents. Low doses of either extract appeared to enhance NO release by unstimulated macrophages. Effects of the clove materials on macrophage cytokine formation/release were mainly dose-related and seemingly bi-phasic (for IL-6 and TNFα), while effects on IL-12 were disparate and mostly unremarkable. The data here and from previous studies suggest a narrow immunomodulatory effect from clove components. Further studies using different doses, pure forms of eugenol, and several other target cell types may yield a clearer picture, as any potentially wider applicability of clove and/or associated extracts for medicinal purposes.
Declaration of interest
The authors report no conflicts of interest. The authors alone are responsible for the content and writing of the paper. This work was supported by a grant of Tarbiat Modares University.
References
- Apparecido, N. D., Simone, M. S., Gustavo, S., et al. 2009. Anti-inflammatory and anti-nociceptive activities of eugenol essential oil in experimental animal models. Rev. Bras. Farmacogn. 19:212–217
- Atsumi, T., Fujisawa, S., and Tonosaki, K. 2005. A comparative study of the anti-oxidant/pro-oxidant activities of eugenol and isoeugenol with various concentrations and oxidation conditions. Toxicol. In Vitro 19:1025–1033
- Bachiega, T. F., de Sousa, J. P., Bastos, J. K., and Sforcin, J. M. 2012. Clove and eugenol in non-cytotoxic concentrations exert immunomodulatory/anti-inflammatory action on cytokine production by murine macrophages. J. Pharm. Pharmacol. 64:610–616
- Chaieb, K., Hajlaoui, H., Zamantar, T., et al. 2007. The chemical composition and biological activity of clove essential oil, Eugenia caryophyllata (Syzugium aromaticum L Myrtaceae): A short review. Phytother. Res. 21:501–506
- Choi, C. Y., Park, K. R., Lee, J. H., et al. 2007. Isoeugenol suppression of inducible nitric oxide synthase expression is mediated by down-regulation of NF-κB, ERK1/2, and p38 kinase. Eur. J. Pharmacol. 576:151–159
- Domaracky, M., Rehak, P., Juhas, S., and Koppel, J. 2007. Effects of selected plant essential oils on the growth and development of mouse pre-implantation embryos in vivo. Physiol. Res. 56:97–104
- El-Ghorab, A., and El-Massry, K. F. 2003. Free radical scavenging and anti-oxidant activity of volatile oils of local clove [Syzygium aromaticum L.] and Cinnamon [Cinnamomum zeylanicum] isolated by supercritical fluid extraction. J. Essen. Oil Bearing Plants 6:9–20
- Grespan, R., Paludo, M., Lemos, H. P., et al. 2012. Anti-arthritic effect of eugenol on collagen-induced arthritis. Experimental model. Biol. Pharm. Bul. 35:1818–1820
- Ko, F. N., Liao, C. H., Kuo, Y. H., and Lin, Y. L. 1995. Anti-oxidant properties of demethyl di-isoeugenol. Biochim. Biophys. Acta. 1258:145–152
- La Flamme, A., MacDonald, A. S., and Pearce, E. J. 2000. Role of IL-6 in directing the initial immune response to schistosome eggs. J. Immunol. 164:2419–2426
- Lee, S., Najiah M., Wendy W., and Nadirah M. 2009. Chemical composition and anti-microbial activity of the essential oil of Syzygium aromaticum flower bud (Clove) against fish systemic bacteria isolated from aquaculture sites. Front. Agric. China 3:332–336
- Lee, Y. Y., Hung, S. L., Pai, S. F., et al. 2007. Eugenol suppressed the expression of lipopolysaccharide-induced pro-inflammatory mediators in human macrophages. J. Endod. 33:698–702
- Moncada, S. 1999. Nitric oxide: Discovery and impact on clinical medicine. J. Royal Soc. Med. 92:164–169
- Myint, S., Wan Daud, W. R., and Mohamad, A. B. 1995a. Determination of optimal conditions for extraction of alcohol-soluble eugenol containing material from cloves. Pertanika. J. Sci. Technol. 3:96–106
- Myint, S., Wan Daud, W. R., Mohamad, A. B., and Kadhum, A. A. 1995b. Separation and identification of eugenol in ethanol extract of cloves by reversed-phase high-performance liquid chromatography. J. Am. Oil. Chem. Soc. 72:1231–1233
- Nassar, M. I., Gaara, A. H., El-Ghorab, A. H., et al. 2007. Chemical constituents of clove (Syzygium aromaticum) and their anti-oxidant activity. Rev. Latinoamer. Quim. 35:47–57
- Persoons, J. H., Schornagel, K., Tilders, F. F., et al. 1996. Alveolar macrophages auto-regulate IL-1 and IL-6 production by endogenous nitric oxide. Am. J. Respir. Cell Mol. Biol. 14:272–278
- Petros, A., Bennett, D., and Vallance, P. 1991. Effect of nitric oxide synthase inhibitors on hypotension in patients with septic shock. Lancet 338:1557–1558
- Prashar, A., Locke, I. C., and Evans, C. S. 2006. Cytotoxicity of clove (Syzygium aromaticum) oil and its major components to human skin cells. Cell Prolif. 39:241–248
- Rodrigues, T. G., Fernandes, A., Sousa, J. P., et al. 2009. In vitro and in vivo effects of clove on pro-inflammatory cytokine production by macrophages. Nat. Prod. Res. 23:319–326
- Scuro, L. S., Simioni, P. U., Gabriel, D. L., et al. 2004. Suppression of nitric oxide production in mouse macrophages by soybean flavonoids accumulated in response to nitroprusside and fungal elicitation. BMC Biochem. 5:5
- Sladowski, D., Steer, S. J., Clothier, R. H., and Balls, M. 1993. An improved MTT assay. J. Immunol. Meth. 157:203–207
- Stuehr, D. J., and Nathan, C. F. 1989. Nitric oxide. A macrophage product responsible for cytostasis and respiratory inhibition in tumor target cells. J. Exp. Med. 169:1543–1555
- Sun, J. L., Hu, Y. L., Wang, D. Y., et al. 2006. Immunologic enhancement of compound Chinese herbal medicinal ingredients and their efficacy comparison with compound Chinese herbal medicines. Vaccine 24:2343–2348
- Takenaka, H., Maruo, S., Yamamoto, N., et al. 1997. Regulation of T-cell-dependent and -independent IL-12 production by the three TH2-type cytokines IL-10, IL-6, and IL-4. J. Leukocyte Biol. 61:80–87
- Yogalakshmi, B., Viswanathan, P., and Anuradha, C. V. 2010. Investigation of anti-oxidant, anti-inflammatory, and DNA-protective properties of eugenol in thioacetamide-induced liver injury in rats. Toxicology 268:204–212