Abstract
Macrophage-1 antigen (Mac-1, αMβ2) is a leukocyte adhesion molecule that plays a significant role in leukocyte crawling and phagocytosis, and is homologous to its sister protein leukocyte function-associated antigen-1 (LFA-1, αLβ2). The authors have previously demonstrated that volatile anesthetics isoflurane and sevoflurane bound to and inhibited LFA-1. Here, the hypothesis tested was that isoflurane and sevoflurane would inhibit Mac-1. A binding assay of Mac-1 to its ligand inter-cellular adhesion molecule-1 (ICAM-1) using V-bottom plates was established. The effect of isoflurane and sevoflurane on Mac-1 was examined using this ICAM-1 binding assay and by probing exposure of activation-sensitive epitopes. The docking program Glide was used to predict anesthetic binding site(s) on Mac-1. The functional role of this predicted binding site was then assessed by introducing point mutations in this region. Lastly, the effect of anesthetic on activating mutants was evaluated. The results indicated that isoflurane inhibited binding of Mac-1 to ICAM-1, but sevoflurane did not. Isoflurane also attenuated the exposure of the activation-sensitive epitopes. The docking simulation predicted the isoflurane binding site to be at the cavity underneath the α7 helix of the ligand binding domain (the αM I domain). Point mutants at this predicted binding site contained both activating and deactivating mutants, suggesting its functional significance. The binding of activating mutants αM-Y267A β2-WT and αM-L312A β2-WT to ICAM-1 was not affected by isoflurane, but binding of another activating mutant αM-WT β2-L132A was inhibited supporting the binding of isoflurane to this cavity. The conclusion reached from these findings was that isoflurane inhibited Mac-1 binding to ICAM-1 by binding to the cavity underneath the α7 helix of the αM I domain, but sevoflurane did not. Thus, because these common clinical volatile anesthetics demonstrated different effects on Mac-1, this implied their effects on the immune system might differ.
Introduction
Volatile anesthetics isoflurane and sevoflurane are used clinically to provide general anesthesia for surgical procedures. The exact mechanism of anesthetic action by these drugs remains to be determined, but it is considered that they directly bind to a subset of ligand-gated ion channel proteins in the central nervous system and alter their functions (Campagna et al., Citation2003; Dilger, Citation2002). Interestingly these anesthetics are suggested to generate an array of pleiotropic effects, even outside the central nervous system, including the effects on the immune system (Eckenhoff, Citation2001). Because adequate immune function is critical to prevent perioperative complications such as infection, understanding the impact and mechanism of volatile anesthetic-induced immunomodulation is important.
Adhesion to the endothelium is an essential process for leukocytes to transmigrate through endothelial cells and accumulate at the sites of infection and inflammation. Volatile anesthetics isoflurane and sevoflurane at clinically relevant concentrations have been shown to reduce the ability of leukocytes to adhere to inflamed vascular endothelial cells, which is a requisite step for leukocyte recruitment (Kowalski et al., Citation1997; Mobert et al., Citation1999). As expected, isoflurane exposure attenuated the amount of accumulated neutrophils to the site of inflammation in the murine skin inflammation model (Carbo et al., Citation2012). The attenuation of neutrophil recruitment by anesthetics can be devastating in the setting of infection because they are the first defense innate immune cells to fight against infection.
Adhesion molecules leukocyte function-associated antigen-1 (LFA-1 aka αLβ2, CD11aCD18) and macrophage-1 antigen (Mac-1 aka αMβ2, CD11bCD18, complement receptor-3) are heterodimeric molecules consisting of α and β subunits and play a pivotal role in leukocyte arrest and intravascular crawling on the endothelium, both critical processes for leukocyte migration (Wagner & Frenette, Citation2008). In addition, Mac-1 facilitates bacterial phagocytosis. Both are expressed only on leukocytes and bind to the ligand called intercellular adhesion molecule-1 (ICAM-1) on the endothelium to facilitate these processes (Diamond et al., Citation1990). A functional defect in these molecules is significant as demonstrated in leukocyte adhesion deficiency characterized by severe infection, often leading to death (Anderson & Springer, Citation1987). Their major ligand-binding domain (called I domain) is located at the top of the extracellular portion of the α subunit (called the αL I domain for LFA-1, the αM I domain for Mac-1). Our laboratory previously demonstrated both isoflurane and sevoflurane bound to and inhibited LFA-1 (Yuki et al., Citation2008, Citation2010, Citation2012). While ICAM-1 binds to LFA-1 at the metal ion-dependent adhesion site (MIDAS) located at the top of the αL I domain (), isoflurane and sevoflurane bind to the hydrophobic cavity underneath the α7 helix of the αL I domain that acts as a functional regulator (). Unwinding of the C-terminal α7 helix presumably leads to conformational rearrangements of the MIDAS from a low- to a high-affinity configuration, only the latter of which can tightly bind to ICAM-1 (Sen et al., Citation2013). By binding to this hydrophobic pocket, volatile anesthetics stabilize the helix of this region and impede the conformational conversion of MIDAS to bind to the ligand.
Figure 1. Structural characteristics of I domain of the αL and αM subunits. (a) I domain of αL subunit (αL I domain) is shown. Structure is from protein data bank (PDB) 1ZOO. β-strands (yellow) are surrounded by α helices (red). Metal-ion dependent adhesion site (MIDAS) (= ICAM-1 binding site) is shown in cyan. (b) Surface of αL I domain is shown. Arrow represents the allosteric cavity underneath the α7 helix to which isoflurane and sevoflurane bind. (c) I domain of αM subunit (αM I domain) is shown (PDB 1M1U). (d) αL I domain (light green) and αM I domain (cyan) are superimposed. (e) Surface of αM I domain is shown. The arrow represents the allosteric cavity underneath α7 helix.
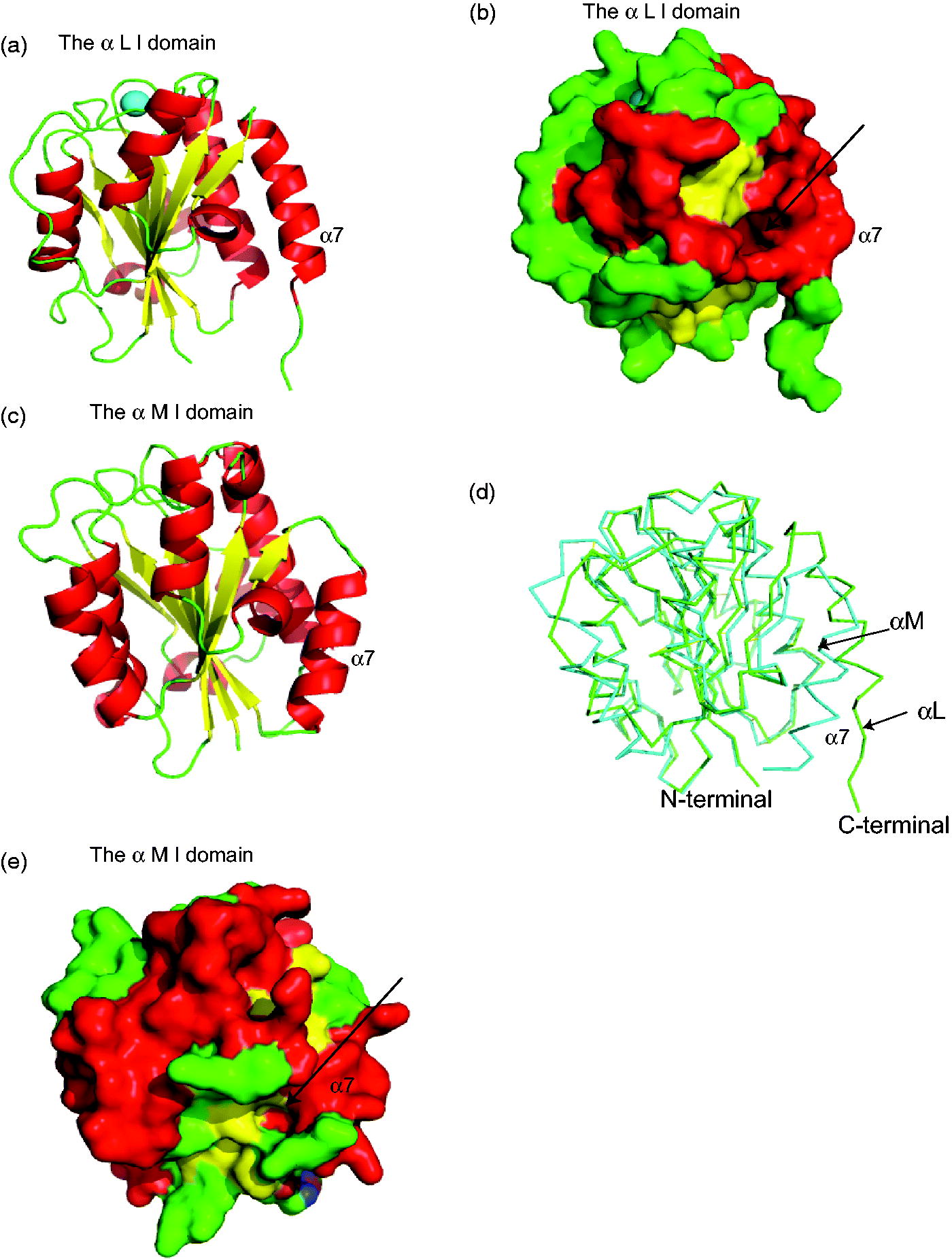
Mac-1 is highly homologous to LFA-1 including the αI domain ( and ). The αM I domain contains a cavity underneath the α7 helix like the αL I domain does (). Therefore, it was hypothesized that isoflurane and sevoflurane would bind this cavity and inhibit Mac-1, as was the case for LFA-1. To test this, an assay system was established to test Mac-1/ICAM-1 binding and examined the impact of volatile anesthetics on Mac-1 function. Further, their potential anesthetic binding sites were predicted using a docking stimulation called Glide and the function of corresponding site(s) was examined.
Materials and methods
Cells and transfection
Human embryonic kidney (HEK) cells (ATCC, Manassas, VA) were cultured in DMEM medium with HEPES modification and 10% fetal bovine serum (Atlanta Biological, Miami, FL) at 37 °C in an atmosphere containing 5% CO2. Integrin αL, αM and β2 plasmids, as well as ICAM-1 plasmid, were prepared are previously described (Jun et al., Citation2001; Shimaoka et al., Citation2002). Transfection with LFA-1 (combination of αL and β2 plasmids), Mac-1 (combination of αM and β2 plasmids) or ICAM-1 was performed using Fugene 6 (Promega; Madison, WI) following manufacturer protocols.
Antibodies
Mouse anti-human αL antibody TS1/22 and mouse anti-human β2 antibody CBR LFA1/7 and m24 were obtained from the Immune Disease Institute (Boston, MA). Mouse anti-human αM antibody ICRF44 and CBR M1/5, and mouse anti-human β2 antibody TS1/18 were purchased from Biolegend (San Diego, CA).
Flow cytometry-based ICAM-1 binding assay
Flow cytometric detection of ICAM-1 binding to LFA-1 or Mac-1 was assayed as described in Yuki et al. (Citation2010). HEK cells transiently transfected with LFA-1 or Mac-1 were harvested and washed once with HEPES-buffered saline (HBS) containing 10 mM EDTA and then 3-times with HBS. Cells were then incubated for 30 min with ICAM-1-fragment crystallizable α(Fcα) in HBS containing 1 mM MgCl2/CaCl2 (inactivating condition) or 1 mM MnCl2 (activating condition as Mn2+ is an activator for LFA-1 and Mac-1). The cells were then washed and incubated for 30 min with fluorescein isothiocyanate (FITC)-labeled anti-human IgA (Life Technologies, Grand Island, NY). After washing, bound ICAM-1 was detected by flow cytometry using an Accuri C6 system (BD Biosciences, San Jose, CA). A minimum of 10 000 events/sample was acquired.
V-bottom plate-based Mac-1 binding assay to ICAM-1
V-bottom plates were coated with 2 µg ICAM-1/ml (R&D systems, Minneapolis, MN) using standard protocols. HEK cells transfected with Mac-1 were stained with 2′,7′-bis-(2-carboxyethyl)-5- (and 6-)-carboxyfluorescein acetoxymethyl ester (BCECF-AM; Life Technologies) and incubated in HBS containing 10 mM EDTA, 1 mM MgCl2/CaCl2 or 1 mM MnCl2 with or without isoflurane or sevoflurane at indicated concentrations at 37 °C for 30 min. Following incubation, the plates were centrifuged at 200 x g for 5 min. Cells that did not bind to ICAM-1 were accumulated at the center of the well. Fluorescence was read at excitation = 485 nm and emission = 538 nm to assess HEK cell binding to ICAM-1. ICAM-1 binding percentage was defined as [(fluorescence intensity of EDTA sample) − (fluorescence intensity of samples)]/[fluorescence intensity of EDTA sample] × 100 (%). In some experiments, 5 µg/ml of LFA-1 or Mac-1 protein (a generous gift from Dr Timothy Springer [Boston Children’s Hospital]) was coated on the 96 well V-bottom plates and HEK cells transiently transfected with ICAM-1 were used.
Rigid docking simulation
Because the αM I domain is the major ligand binding domain (Diamond et al., Citation1993), it was postulated here that binding site(s) of volatile anesthetics localized within this domain. The structure of the αM I domain was obtained from the Protein Data Bank (PDB) ID number 1M1U (http://www.rcsb.org/pdb). The program Glide (Schrodinger, Cambridge, MA) was used to perform rigid docking of isoflurane with the αM I domain (Friesner et al., Citation2004). Isoflurane binding position was sought with the grid size of 20 × 20 × 20 cubic angstrom, which is substantially larger than the size of isoflurane (144-cubic angstrom; Jenkins et al., Citation2001). No positional constraint was applied. Glide has a scoring system that ranks docked pairs based on predicted affinity. The docked pair with the most negative score was selected, as it is considered to have the highest affinity.
Alanine scanning mutagenesis
Site-directed mutagenesis was performed using Quikchange XL kit (Agilent Technologies, Lexington, MA) and DNA sequences were confirmed. Mutants were tested for surface expression and ICAM-1 binding.
Statistical analysis
Data were analyzed as indicated in the figure legends. Statistical significance was defined at p values < 0.05. All statistical calculations were done using PRISM 5 software (GraphPad Software, La Jolla, CA).
Results
ICAM-1 binding is greater to LFA-1 than to Mac-1
A flow cytometry-based LFA-1:ICAM-1 binding assay previously reported (Yuki et al., Citation2008, Citation2012) was used in which dimeric soluble ICAM-1 can bind to one or two LFA-1 on the cell surface (). Binding of Mac-1 to soluble ICAM-1 was not significant using this method (). When a leukocyte binds to the endothelium, multiple LFA-1 or Mac-1 on a single leukocyte can interact with multiple ICAM-1 on an endothelial cell. To simulate LFA-1 (or Mac-1) binding to ICAM-1 between cells, the binding of coated LFA-1 or Mac-1 protein to cells over-expressing ICAM-1 was evaluated (). In this plate-based assay, both LFA-1 and Mac-1 bound to ICAM-1 (). ICAM-1 binding was more robust onto LFA-1 than onto Mac-1, compatible with results of the flow-based assay.
Figure 2. Flow cytometry- and plate-based ICAM-1 binding assays. (A) Schemes of flow cytometry- and plate-based ICAM-1 binding assays. In plate-based assay using ICAM-1 over-expressing HEK cells and coated LFA-1 (or Mac-1) protein is illustrated. Binding assay using LFA-1 (or Mac-1) over-expressing HEK cells and coated ICAM-1 protein are not shown. (B, left) Representative result of flow cytometry-based ICAM-1 binding to LFA-1 (or Mac-1) over-expressing HEK cells. Mock indicates HEK cells transiently transfected with empty vector. Mg/Ca = 1 mM MgCl2/CaCl2, Mn = 1 mM MnCl2. Three independent experiments were performed. (B, right) Representative result of V-bottom plate-based ICAM-1 binding assays using ICAM-1 over-expressing HEK cells and coated LFA-1 (or Mac-1) protein. 5 µg/ml of LFA-1 (or Mac-1) protein was coated on wells. Data shown are mean ± SD of triplicates. Three independent experiments were performed.
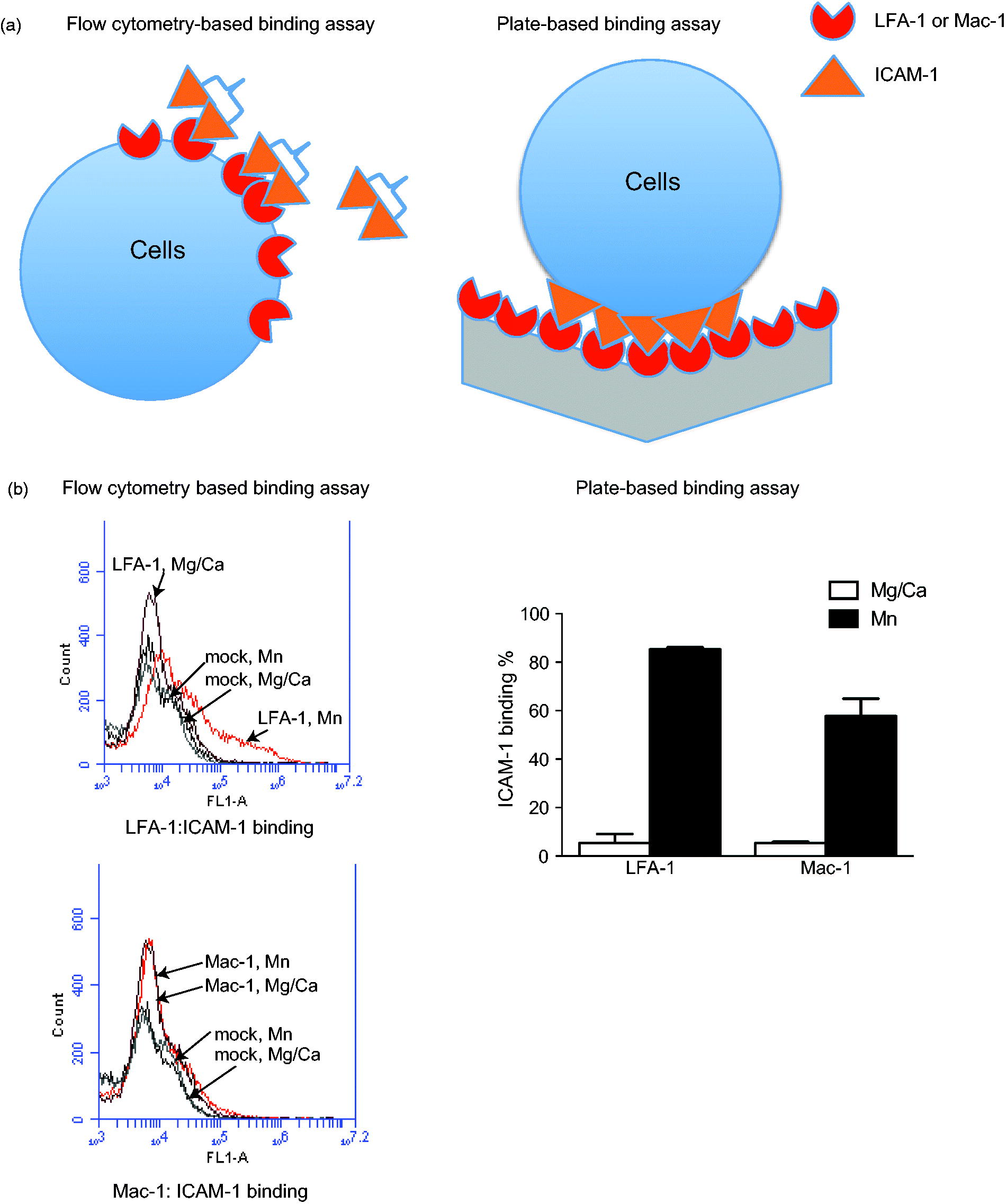
Effects of anesthetics on LFA-1 and Mac-1 binding to ICAM-1
Previous studies reported that both isoflurane and sevoflurane reduced binding of LFA-1 to ICAM-1 in a flow cytometry-based assay (Yuki et al., Citation2008, Citation2010). Both anesthetics attenuated binding of ICAM-1 to LFA-1 in the plate-based binding assay using coated LFA-1 protein and ICAM-1-expressing cells ( and ). Binding of ICAM-1-expressing cells to coated Mac-1 protein was also assessed; Mac-1 binding was impaired by isoflurane, but not sevoflurane ( and ). A binding assay was also performed using HEK cells over-expressing Mac-1 and coated ICAM-1 protein. In tests of binding of Mac-1-over-expressing cells to coated ICAM-1 protein, Mac-1 binding was inhibited by isoflurane, but not by sevoflurane ( and ), an outcome compatible with the results of binding of ICAM-1-expressing cells to coated Mac-1 protein
Figure 3. Effect of isoflurane and sevoflurane on ICAM-1 binding to LFA-1 or Mac-1. Impact of (a) isoflurane and (b) sevoflurane on ICAM-1 binding to LFA-1 or Mac-1 with Mn2+ stimulation was examined using ICAM-1 over-expressing HEK cells and coated LFA-1 (or Mac-1) protein [5 µg/ml LFA-1 (or Mac-1) coated on V-bottom wells]. Statistical significance between groups with and without anesthetics was assessed using two-tailed student t-test. Impact of (c) isoflurane and (d) sevoflurane on binding of ICAM-1 to Mac-1 with Mn2+ stimulation was examined by using Mac-1 over-expressing HEK cells and coated ICAM-1 protein. 2 µg ICAM-1/ml was coated on wells. For (c), significance was tested using 1-way analysis of variance with Bonferroni post-hoc analysis. For (d), significance was tested using 2-tailed Student’s t-test. (e) Effect of isoflurane and sevoflurane on epitope exposure of activation-sensitive antibody CBRM1/5. MFI = mean fluorescence intensity. Significance was tested using 2-tailed Student’s t-test. *p < 0.05; n.s. = not significant. Data shown are mean ± SD of triplicates. In (a–e), three independent experiments were performed.
![Figure 3. Effect of isoflurane and sevoflurane on ICAM-1 binding to LFA-1 or Mac-1. Impact of (a) isoflurane and (b) sevoflurane on ICAM-1 binding to LFA-1 or Mac-1 with Mn2+ stimulation was examined using ICAM-1 over-expressing HEK cells and coated LFA-1 (or Mac-1) protein [5 µg/ml LFA-1 (or Mac-1) coated on V-bottom wells]. Statistical significance between groups with and without anesthetics was assessed using two-tailed student t-test. Impact of (c) isoflurane and (d) sevoflurane on binding of ICAM-1 to Mac-1 with Mn2+ stimulation was examined by using Mac-1 over-expressing HEK cells and coated ICAM-1 protein. 2 µg ICAM-1/ml was coated on wells. For (c), significance was tested using 1-way analysis of variance with Bonferroni post-hoc analysis. For (d), significance was tested using 2-tailed Student’s t-test. (e) Effect of isoflurane and sevoflurane on epitope exposure of activation-sensitive antibody CBRM1/5. MFI = mean fluorescence intensity. Significance was tested using 2-tailed Student’s t-test. *p < 0.05; n.s. = not significant. Data shown are mean ± SD of triplicates. In (a–e), three independent experiments were performed.](/cms/asset/d3c71153-0238-4797-89d0-b376c330dfb7/iimt_a_1019596_f0003_b.jpg)
Effects of isoflurane and sevoflurane on Mac-1 activation
CBRM1/5 is an antibody with epitopes near the MIDAS and interacts with the αM I domain in an active conformation (Oxvig et al., Citation1999). The present study examined expression of CBRM1/5 epitopes with or without isoflurane or sevoflurane in the presence of Mn2+. The results indicated that isoflurane, not sevoflurane attenuated CBRM1/5 epitope expression, an outcome in line with results of the above-mentioned binding assays ().
Isoflurane docking to αM I domain cavity
LFA-1 and Mac-1 are quite homologous (Springer et al., Citation1985), with the amino acid homology between the αL I and αM I domains being ≈ 34 %. As expected, the structures of the αL I and αM I domain are well-superimposed (). ICAM-1 binds to LFA-1 at the MIDAS of the αL I domain (Shimaoka et al., Citation2003). The exact ICAM-1 binding site on Mac-1 has not been completely determined, but the αM I domain is the major recognition site if not the solo binding site (Diamond et al., Citation1993). It was previously reported that both isoflurane and sevoflurane inhibited LFA-1 by binding to the allosteric cavity of the αL I domain (lovastatin site). As Mac-1 has a similar cavity in the αM I domain called ‘SILEN (socket for isoleucine)’ (Xiong et al., Citation2000), it was predicted that isoflurane would bind to the cavity. Docking simulation indicated isoflurane bound to the pocket (), with the trifluoromethyl head of isoflurane interacting with αM-Asp-294 and αM-Gln-311. The difluoromethyl fluorine showed hydrophobic interaction with αM-Lys-315. Interestingly, sevoflurane did not show docking in this condition, which is in line with our binding data.
Presumed isoflurane binding site on Mac-1 is functionally important
In the docking simulation, this study identified the amino acids within 4 angstrom from isoflurane and mutated them to alanine. Surface expression and activation status of all mutants were then examined ( and ) and the degree of activation probed with CBRM1/5 antibody. αM-Y267A β2-WT and αM-L312A β2-WT mutants were constitutively active as they demonstrated significant activation-sensitive epitope exposure, even in an inactivating condition (Mg2+/Ca2+), while the αM-K315A β2-WT mutant remained inactive even in an activating condition (Mn2+), demonstrated as limited activating epitope exposure. Binding of these mutants to ICAM-1 was also examined using a plate-based assay (). This result was compatible with the one of activation-sensitive epitope exposure (). These results reveal the presumed isoflurane-binding site was extremely important in Mac-1 activation and binding.
Figure 5. Functional role of predicted isoflurane binding site in Mac-1. (a) Surface expression of alanine scanning mutants at the predicted isoflurane binding site (the αM I domain) was probed by ICRF44 antibody. β2-WT was paired with all αM-mutants. MFI = mean fluorescence intensity. (b) Activation level of mutants probed by CBRM1/5 antibody. CBRM1/5 expression was normalized by expression of activating-insensitive antibody ICRF44 as follows: Normalized CBRM1/5 expression = [(MFI probed by CBRM1/5 antibody in WT or mutants) – (MFI probed by CBRM1/5 antibody in mock)]/[(MFI probed by ICRF44 antibody in WT or mutants) − (MFI probed by ICRF44 antibody in mock)]. Thereafter, relative value to normalized CBRM1/5 expression in WT, 1 mM Mg2+/Ca2+ was calculated. One-way analysis of variance with Bonferroni post-hoc analysis was performed to compare data within the 1 mM Mg2+/Ca2+ or 1 mM Mn2+ group. *p < 0.05 versus WT Mg2+/Ca2+ and **p < 0.05 versus WT Mn2+. (c) Binding of mutants to ICAM-1 using plate-based assay with 2 µg ICAM-1/ml-coated wells. *p < 0.05 versus WT Mg2+/Ca2+ and **p < 0.05 versus WT Mn2+. Data shown are mean ± SD of triplicates. In (a–c), representative data from three independent experiments are shown.
![Figure 5. Functional role of predicted isoflurane binding site in Mac-1. (a) Surface expression of alanine scanning mutants at the predicted isoflurane binding site (the αM I domain) was probed by ICRF44 antibody. β2-WT was paired with all αM-mutants. MFI = mean fluorescence intensity. (b) Activation level of mutants probed by CBRM1/5 antibody. CBRM1/5 expression was normalized by expression of activating-insensitive antibody ICRF44 as follows: Normalized CBRM1/5 expression = [(MFI probed by CBRM1/5 antibody in WT or mutants) – (MFI probed by CBRM1/5 antibody in mock)]/[(MFI probed by ICRF44 antibody in WT or mutants) − (MFI probed by ICRF44 antibody in mock)]. Thereafter, relative value to normalized CBRM1/5 expression in WT, 1 mM Mg2+/Ca2+ was calculated. One-way analysis of variance with Bonferroni post-hoc analysis was performed to compare data within the 1 mM Mg2+/Ca2+ or 1 mM Mn2+ group. *p < 0.05 versus WT Mg2+/Ca2+ and **p < 0.05 versus WT Mn2+. (c) Binding of mutants to ICAM-1 using plate-based assay with 2 µg ICAM-1/ml-coated wells. *p < 0.05 versus WT Mg2+/Ca2+ and **p < 0.05 versus WT Mn2+. Data shown are mean ± SD of triplicates. In (a–c), representative data from three independent experiments are shown.](/cms/asset/c228b83b-41b8-40ce-81dd-bc20608a1ab8/iimt_a_1019596_f0005_b.jpg)
Effect of isoflurane on Mac-1-activating mutants
The effect of isoflurane on activating mutants αM-Y267A β2-WT and αM-L312A β2-WT, both of which have alternation at the allosteric cavity of the αM I domain, was evaluated. Any attenuation of ICAM-1 binding by isoflurane might indicate the presence of any other potential isoflurane binding site outside the allosteric pocket in the αM I domain. Isoflurane did not attenuate binding of αM-Y267A β2-WT and αM-L312A β2-WT to ICAM-1 (). The study then searched for a Mac-1-activating mutant without modification of the allosteric cavity in the αM I domain. Previously, it was demonstrated that αL-WT β2-L132A and αX-WT β2-L132A mutants were constitutively active (Sen et al., Citation2013; Yuki et al., Citation2012). β2-Lys-132 is located at the cavity in the top domain of the β2 subunit called the β2 I domain. Here, tests were done to ascertain if αM-WT β2-L132A behaved similarly. As seen in , this mutant was constitutively active, as demonstrated by activation-sensitive epitope exposure in the βI domain probed by m24 (Sen et al., Citation2013) and ICAM-1 binding even under Mg2+/Ca2+ conditions. β2-Lys-135 is located at the same pocket where β2-Lys-132 is located.
Figure 6. Effect of isoflurane on activating mutants. (a) Binding of WT and activating mutants αM-Y267A β2-WT and αM-L312A β2-WT to ICAM-1 with or without 2% isoflurane in presence of Mn2+. A 2-tailed Student’s t-test was performed between groups with or without isoflurane in either Mac-1 WT, αM-Y267A β2-WT or αM-L312A β2-WT. *p < 0.05; n.s. = not significant. (b) Mac-1 WT, αM-WT β2-L132A and αM-WT β2-L135A were subject to ICAM-1 binding assay and probing activation-sensitive epitopes in β2 I domain using m24. Expression of m24 epitopes was normalized by CBR LFA1/7 expression as [(MFI of m24 of sample) – (MFI of m24 of mock)]/[(MFI of CBR LFA1/7 of sample) – (MFI of CBR LFA1/7 of mock)]. Statistical significance was tested using 1-way analysis of variance with Bonferroni post-hoc analysis to compare data within 1 mM Mg2+/Ca2+ or 1 mM Mn2+. *p < 0.05 vs WT Mg2+/Ca2+ and **p < 0.05 vs WT Mn2+. (c) Effect of isoflurane on ICAM-1 binding in Mac-1 WT and αM-WT β2-L132A. Statistical significance tested using 2-tailed Student’s t-test. *p < 0.05. (d) TS1/18 antibody epitope exposure in LFA-1 and Mac-1. TS1/18 expression normalized by CBR LFA1/7 expression was calculated as [(MFI of TS1/18 of sample) – (MFI of TS1/18 of mock)]/[(MFI of CBR LFA1/7 of sample) – (MFI of CBR LFA1/7 of mock)]. Statistical significance was analyzed using 1-way analysis of variance with a Bonferroni post-hoc analysis. *p < 0.05; n.s. = not significant. Data shown are mean ± SD of triplicates. In (a–d), representative data from three independent experiments are shown.
![Figure 6. Effect of isoflurane on activating mutants. (a) Binding of WT and activating mutants αM-Y267A β2-WT and αM-L312A β2-WT to ICAM-1 with or without 2% isoflurane in presence of Mn2+. A 2-tailed Student’s t-test was performed between groups with or without isoflurane in either Mac-1 WT, αM-Y267A β2-WT or αM-L312A β2-WT. *p < 0.05; n.s. = not significant. (b) Mac-1 WT, αM-WT β2-L132A and αM-WT β2-L135A were subject to ICAM-1 binding assay and probing activation-sensitive epitopes in β2 I domain using m24. Expression of m24 epitopes was normalized by CBR LFA1/7 expression as [(MFI of m24 of sample) – (MFI of m24 of mock)]/[(MFI of CBR LFA1/7 of sample) – (MFI of CBR LFA1/7 of mock)]. Statistical significance was tested using 1-way analysis of variance with Bonferroni post-hoc analysis to compare data within 1 mM Mg2+/Ca2+ or 1 mM Mn2+. *p < 0.05 vs WT Mg2+/Ca2+ and **p < 0.05 vs WT Mn2+. (c) Effect of isoflurane on ICAM-1 binding in Mac-1 WT and αM-WT β2-L132A. Statistical significance tested using 2-tailed Student’s t-test. *p < 0.05. (d) TS1/18 antibody epitope exposure in LFA-1 and Mac-1. TS1/18 expression normalized by CBR LFA1/7 expression was calculated as [(MFI of TS1/18 of sample) – (MFI of TS1/18 of mock)]/[(MFI of CBR LFA1/7 of sample) – (MFI of CBR LFA1/7 of mock)]. Statistical significance was analyzed using 1-way analysis of variance with a Bonferroni post-hoc analysis. *p < 0.05; n.s. = not significant. Data shown are mean ± SD of triplicates. In (a–d), representative data from three independent experiments are shown.](/cms/asset/03e7a0b2-4ae3-45c1-a346-b57a3ab9cffb/iimt_a_1019596_f0006_b.jpg)
Our previous data showed both αL-WT β2-L135A and αX-WT β2-L135A were deactivating mutants. Likewise, αM-WT β2-L135A was deactivating (). The βI domain is considered functionally important as it relays the activation signal to the α I domain and induces its conformational change; results here suggested that LFA-1, Mac-1, and αXβ2 use a common mechanistic approach to activate the α subunit from the β2 subunit. Isoflurane exposure reduced the degree of ICAM-1 binding to αM-WT β2-L132A, which does not have any mutation in the allosteric cavity of the αM I domain (). Taken together, it appears isoflurane would bind to this cavity (). These results are in contrast to our previous LFA-1 study. With LFA-1, binding of the activating mutant αL-I306A β2-WT (αL-Ile-306 is amino acid residue in allosteric cavity of α I domain) was attenuated by isoflurane; isoflurane bound to the β2 I domain in addition to the αL I domain (Yuki et al., Citation2012). The isoflurane binding pocket in the β2 I domain of LFA-1, where β2-Lys-132 and β2-Lys-135 are located, can be probed by TS1/18 antibody as its epitopes (β2-Arg-133 and β2-Gln-322 (Huang et al., Citation2000)) are present in this pocket (Yuki et al., Citation2008). Because isoflurane was unlikely to bind to the β2 subunit of Mac-1 based on binding studies, it was hypothesized the β2 I domain conformation might be not exactly the same between LFA-1 and Mac-1. We examined TS1/18 antibody epitope exposure in relative to the expression of another β2 antibody (CBR LFA1/7) that probes more C-terminal residues of the β2 subunit (residue 432–487) (Lu et al., Citation2001). The epitopes of TS1/18 were less exposed in Mac-1 than in LFA-1 as predicted (), which likely hindered isoflurane binding to the β2 I domain of Mac-1.
Discussion and conclusion
In the study reported here, it was shown that isoflurane inhibited binding of both LFA-1 and Mac-1 to ICAM-1, but that sevoflurane inhibited only LFA-1. Docking stimulation suggested to us that isoflurane bound to the allosteric cavity located underneath the α7 helix of the αM I domain. A mutagenesis experiment confirmed the functionality of this cavity and also supported the binding of isoflurane to this cavity. In all the binding assays, plate-based binding assays were used, as the functional role of soluble ICAM-1 is yet to be determined (Witkowska & Borawska, Citation2004) and the assay system using soluble ICAM-1 may not reflect the binding of Mac-1 to ICAM-1 in vivo.
Both sevoflurane and isoflurane are volatile anesthetics with an ether group that are widely used in clinical practice. However, their anesthetic potencies differ, as demonstrated in their minimum alveolar concentration (MAC) values (sevoflurane ≈ 1.8% versus isoflurane 1.2 % in adults), suggesting that the underlying mechanism of their anesthetic actions may not be exactly the same or there exists a difference in their affinity with the same molecules. For example, sevoflurane and isoflurane demonstrated different effects on 5-HT3AB receptors (Stevens et al., Citation2005). While sevoflurane inhibited 5-HT3AB, isoflurane minimally affected it. Volatile anesthetics presumably interact with multiple targets including targets outside the central nervous system (Eckenhoff, Citation2001). Therefore, it is natural to think that volatile anesthetics may not have the same functional profiles outside the central nervous system, possibly having different target molecules. Mac-1 is a good example to suggest that isoflurane and sevoflurane may have different immunological effects.
The present study did not demonstrate the direct interaction of isoflurane with Mac-1 using X-ray crystallography or nuclear magnetic resonance (NMR). While αM-Y267A β2-WT and αM-L312A β2-WT mutants have altered allosteric cavity in the αM I domain, this cavity is presumably intact in the αM-WT β2-L132A mutant. The result showing that isoflurane inhibited αM-WT β2-L312A, not αM-Y267A β2-WT and αM-L312A β2-WT, in conjunction with the docking simulation, strongly supports the proposition that isoflurane inhibits Mac-1 directly by binding to the cavity in the αM I domain. In the future, detailed analyses using X-ray crystallography will be important, particularly studies performing co-crystallization of full-length Mac-1 with isoflurane. Unfortunately, there is no reported X-ray crystallographic structure of full-length Mac-1 available, but co-crystallization with isoflurane will likely provide significantly valuable information because the α7 helix of αM I domain is considered to be extremely flexible in associated with the β2 subunit, as suggested in αXβ2, another sister protein of Mac-1, and the conformation of and around the allosteric cavity in the αM I domain can change significantly. An alternative approach will be to use dynamic NMR using the αM I domain to assess the interaction with isoflurane or sevoflurane, especially at around the α7 helix. The use of full-length Mac-1 in dynamic NMR is impractical due to the size of the molecule. Sevoflurane is larger in size than isoflurane. Detailed structural analysis including the size of the pocket using full-length Mac-1 in the future will allow us to understand the underlying mechanism of differential effects of volatile anesthetics on Mac-1 from a structural perspective.
Similar to volatile anesthetics, Mac-1 interacts with several ligands such as ICAM-1, iC3b, fibrinogen and Factor X. As a result, it is involved in a wide range of immunological events such as leukocyte intravascular crawling (Dunne et al., Citation2002), phagocytosis (Harris et al., Citation2000) and neutrophil apoptosis (Coxon et al., Citation1996; Mayadas & Cullere, Citation2005). In this study, binding of Mac-1 to ICAM-1 was evaluated because this interaction is critical for the recruitment of leukocytes (particularly neutrophils) in patients who undergo surgery. Although both LFA-1 and Mac-1 bind to ICAM-1, they reveal different binding kinetics; the binding affinity for LFA-1 is much stronger than that for Mac-1 (Li et al., Citation2013), which is in line with our binding results. It is generally noted that the binding of LFA-1 to ICAM-1 allows neutrophils to arrest on the endothelium, while the binding of Mac-1 to ICAM-1 will allow neutrophils to crawl on the endothelium (Phillipson et al., Citation2006). However, differences in neutrophil recruitment among different tissues have been recognized (Kolaczkowska & Kubes, Citation2013), and it is still yet to be determined how each tissue uses these adhesion molecules for neutrophil migration.
We recently found that, in a polymicrobial sepsis model, Mac-1 knockout mice had a significant reduction in neutrophil migration to their liver, but LFA-1 knockout mice did not show any reduction (unpublished data, paper in submission). This suggests to us that Mac-1 is a dominant factor in neutrophil migration to the liver in the model. Neutrophils are responsible for various, acute inflammatory liver injuries such as ischemic-reperfusion mediated liver injury, alcoholic hepatitis and acetaminophen liver toxicity (Ramaiah & Jaeschke, Citation2007). Ischemic reperfusion of liver can be observed during liver transplantation, liver surgery using Pringle maneuver and hemorrhagic shock. In those cases, isoflurane or sevoflurane may be given to patients for surgical anesthesia. Attenuation of neutrophil extravasation is important to alleviate the severity of liver injury. Based on our in vitro results, isoflurane anesthesia may be preferable over sevoflurane anesthesia in these clinical scenarios to attenuate the degree of inflammatory-related liver injury. In vivo examination is warranted in the future. In addition, whether or not there is any difference in bacterial phagocytosis as a result of exposure to the different anesthetics still needs to be determined.
Additional interesting findings in the present study were related to integrin biology. First, the β2 I domain structure of Mac-1 was not exactly the same with the one of LFA-1. Isoflurane binding pocket in the β2 I domain of LFA-1 was less exposed in Mac-1 when probed by TS 1/18 antibody. This may hinder access of isoflurane to the β2 I domain in Mac-1. Second, there exists a common activation mechanism from the β2 I domain to the α I domain among LFA-1, Mac-1 and αXβ2. When mutations at the cavity of the β2 I domain were studied, αL-WT β2-L132A, αM-WT β2-L132A and αX-WT β2-L132A were all activating, while αL-WT β2-L135A, αM-WT β2-L135A and αX-WT β2-L135A were all deactivating, mutants. The role of this cavity needs to be explored more in detail in the future. Information about the full-length LFA-1 and Mac-1 structures would be critical for that purpose.
In conclusion, the present study showed that two commonly used volatile anesthetics, isoflurane and sevoflurane, affected an important immunological molecule, i.e. Mac-1, differently. This suggested to us that widely used volatile anesthetics potentially have different immunotoxic effects.
Declaration of interest
The authors report no conflicts of interest. This work is supported by National Institute of Health (NIH) grant K08GM101345 (K.Y.) and CHMC Anesthesia Foundation (K.Y.).
Acknowledgements
The authors wish to thank Dr Roderic G. Eckenhoff (University of Pennsylvania) for insightful comments.
References
- Anderson, D. C., and Springer, T. A. 1987. Leukocyte adhesion deficiency: An inherited defect in the Mac-1, LFA-1, and p150,95 glycoproteins. Annu. Rev. Med. 38:175–194
- Campagna, J. A., Miller, K. W., and Forman, S. A. 2003. Mechanisms of actions of inhaled anesthetics. New Engl. J. Med. 348:2110–2124
- Carbo, C., Yuki K., Demers, M., et al. 2012. Isoflurane inhibits neutrophil recruitment in the cutaneous Arthus reaction model. J. Anesth. 27:261–268
- Coxon, A., Rieu, P., Barkalow, F. J., et al. 1996. A novel role for the β2 integrin CD11b/CD18 in neutrophil apoptosis: A homeostatic mechanism in inflammation. Immunity 5:653–766
- Diamond, M. S., Garcia-Aguilar, J., Bickford, J. K., et al. 1993. The I domain is a major recognition site on the leukocyte integrin Mac-1 (CD11b/CD18) for four distinct adhesion ligands. J. Cell Biol. 120:1031–1043
- Diamond, M. S., Staunton, D. E., de Fougerolles, A. R., et al. 1990. ICAM-1 (CD54): A counter-receptor for Mac-1 (CD11b/CD18). J. Cell Biol. 111:3129–3139
- Dilger, J. P. 2002. The effects of general anaesthetics on ligand-gated ion channels. Br. J. Anaesth. 89:41–51
- Dunne, J. L., Ballantyne, C. M., Beaudet, A. L., and Ley, K. 2002. Control of leukocyte rolling velocity in TNFα-induced inflammation by LFA-1 and Mac-1. Blood 99:336–341
- Eckenhoff, R. G. 2001. Promiscuous ligands and attractive cavities: How do the inhaled anesthetics work? Mol. Interv. 1:258–268
- Friesner, R. A., Banks, J. L., Murphy, R. B., et al. 2004. Glide: A new approach for rapid, accurate docking and scoring. 1. Method and assessment of docking accuracy. J. Med. Chem. 47:1739–1749
- Harris, E. S., McIntyre, T. M., Prescott, S. M., and Zimmerman, G. A. 2000. The leukocyte integrins. J. Biol. Chem. 275:23409–23412
- Huang, C., Zang, Q., Takagi, J., and Springer, T. A. 2000. Structural and functional studies with antibodies to the integrin β2 subunit. A model for the I-like domain. J. Biol. Chem. 275:21514–21524
- Jenkins, A., Greenblatt, E. P., Faulkner, H. J., et al. 2001. Evidence for a common binding cavity for three general anesthetics within the GABAA receptor. J. Neurosci. 21:RC136
- Jun, C. D., Shimaoka, M., Carman, C. V., et al. 2001. Dimerization and the effectiveness of ICAM-1 in mediating LFA-1-dependent adhesion. Proc. Natl. Acad. Sci. USA 98:6830–6835
- Kolaczkowska, E., and Kubes, P. 2013. Neutrophil recruitment and function in health and inflammation. Nat. Rev. Immunol. 13:159–175
- Kowalski, C., Zahler, S., Becker, B. F., et al. 1997. Halothane, isoflurane, and sevoflurane reduce post-ischemic adhesion of neutrophils in the coronary system. Anesthesiology 86:188–195
- Li, N., Mao, D., Lu, S., et al. 2013. Distinct binding affinities of Mac-1 and LFA-1 in neutrophil activation. J. Immunol. 190:4371–4381
- Lu, C., Ferzly, M., Takagi, J., and Springer, T. A. 2001. Epitope mapping of antibodies to the C-terminal region of the integrin β2 subunit reveals regions that become exposed upon receptor activation. J. Immunol. 166:5629–4637
- Mayadas, T. N., and Cullere, X. 2005. Neutrophil β2 integrins: Moderators of life or death decisions. Trends Immunol. 26:388–395
- Mobert, J., Zahler, S., Becker, B. F., and Conzen, P. F. 1999. Inhibition of neutrophil activation by volatile anesthetics decreases adhesion to cultured human endothelial cells. Anesthesiology 90:1372–1381
- Oxvig, C., Lu, C., and Springer, T. A. 1999. Conformational changes in tertiary structure near the ligand binding site of an integrin I domain. Proc. Natl. Acad. Sci. USA 96:2215–2220
- Phillipson, M., Heit, B., Colarusso, P., et al. 2006. Intraluminal crawling of neutrophils to emigration sites: A molecularly distinct process from adhesion in the recruitment cascade. J. Exp. Med. 203:2569–2575
- Ramaiah, S. K., and Jaeschke, H. 2007. Role of neutrophils in the pathogenesis of acute inflammatory liver injury. Toxicol. Pathol. 35:757–766
- Sen, M., Yuki, K., and Springer, T. A. 2013. An internal ligand-bound, metastable state of a leukocyte integrin, αXβ2. J. Cell Biol. 203:629–642
- Shimaoka, M., Lu, C., Salas, A., et al. 2002. Stabilizing the integrin α M inserted domain in alternative conformations with a range of engineered disulfide bonds. Proc. Natl. Acad. Sci. USA 99:16737–16741
- Shimaoka, M., Xiao, T., Liu, J. H., et al. 2003. Structures of the αL I domain and its complex with ICAM-1 reveal a shape-shifting pathway for integrin regulation. Cell 112:99–111
- Springer, T. A., Teplow, D. B., and Dreyer, W. J. 1985. Sequence homology of the LFA-1 and Mac-1 leukocyte adhesion glycoproteins and unexpected relation to leukocyte interferon. Nature 314:540–542
- Stevens, R., Rusch, D., Solt, K., et al. 2005. Modulation of human 5-hydroxytryptamine type 3AB receptors by volatile anesthetics and n-alcohols. J. Pharmacol. Exp. Ther. 314:338–345
- Wagner, D. D., and Frenette, P. S. 2008. The vessel wall and its interactions. Blood 111:5271–5281
- Witkowska, A. M., and Borawska, M. H. 2004. Soluble intercellular adhesion molecule-1 (sICAM-1): An overview. Eur. Cytokine Network 15:91–98
- Xiong, J. P., Li, R., Essafi, M., et al. 2000. An isoleucine-based allosteric switch controls affinity and shape shifting in integrin CD11b A-domain. J. Biol. Chem. 275:38762–38767
- Yuki, K., Astrof, N. S., Bracken, C., et al. 2008. The volatile anesthetic isoflurane perturbs conformational activation of integrin LFA-1 by binding to the allosteric regulatory cavity. FASEB J. 22:4109–4116
- Yuki, K., Astrof, N. S., Bracken, C., et al. 2010. Sevoflurane binds and allosterically blocks integrin lymphocyte function-associated antigen-1. Anesthesiology 113:600–609
- Yuki, K., Bu, W., Xi, J., et al. 2012. Isoflurane binds and stabilizes a closed conformation of the leukocyte function-associated antigen-1. FASEB J. 26:4408–4417