Abstract
Ferula species (Apiaceae) are considered important medicinal plants. The present in vitro study sought to investigate the immunomodulatory and anti-inflammatory properties of terpenoid coumarins isolated from Ferula szowitsiana on immune cells isolated from naïve mice and to elucidate possible underlying mechanisms of action. With splenocytes, effects of the agents on PHA-induced proliferation and interleukin (IL)-4 and interferon (IFN)-γ release were assessed. With peritoneal macrophages, anti-inflammatory potentials were evaluated in lipopolysaccharide (LPS)/IFNγ-stimulated cells via measures of changes in nitric oxide (NO) and PGE2 secretion. Expression of inducible nitric oxide synthase (iNOS) and cyclooxygenase-2 (COX-2) was also determined via Western blot analysis. The results indicated that umbelliprenin (UMB) and methyl galbanate (MG) reduced remarkably PHA-induced splenocyte proliferation and both preferentially induced TH2 IL-4 and suppressed TH1 IFNγ secretion. Each also significantly suppressed LPS-induced production of NO and PGE2 apparently and also led to reductions in inducible iNOS and COX-expression. To the authors’ knowledge, the present study is the first to report on anti-inflammatory and immunomodulatory effects of UMB and MG in vitro. The present results suggest that each could potentially be exploited as a natural immunosuppressant against inflammatory and autoimmune diseases once substantial further toxicological analyses have been done to confirm overall safety in vivo.
Introduction
The genus Ferula L. in the family Apiaceae consists of ∼170 species worldwide, and some species have been used in traditional medicine for the treatment of different ailments (Kurzyna-Mlynik et al., Citation2008). Ferula szowitsiana DC is a genus that has attracted attention as it is reputed to be a rich source of biologically active constituents, such as terpenoids and their derivatives (Kanani et al., Citation2011). However, while there have been many studies in vitro of effects of Ferula szowitsiana DC/its constituents on immune/non-immune cell types (Kohno et al., Citation2011; Soltani et al., Citation2010) and a few animal studies to examine effects on host health/various physiologic endpoints (see Dehghan et al., Citation2007; Hasani-Ranjbar et al., Citation2009), to date there have been no clinical studies performed (or at least reported in the literature).
Phytochemical analyses have revealed the presence of various bioactive compounds, including terpernoids, in different Ferula genus. Umbelliprenin (UMB) and methyl galbanate (MG) are terpenoid coumarins mostly found in F. szowitsiana (). MG was recently characterized as being able to impart immunomodulatory effects in vitro. Such effects would add to the panel of effects that have been associated with these agents (Kasaian et al., Citation2014; Shakeri et al., Citation2014) including imparting in vitro anti-inflammatory (Iranshahi et al., Citation2009; Kohno et al., Citation2011) and anti-oxidant (Murakami & Ohigashi, Citation2006) activities.
Within a host immune system, macrophages became activated and generate pro-inflammatory mediators to eliminate any pathogens that enter the body. Unfortunately, over-production of mediators may result in states of acute or chronic inflammation that contribute to pathological responses, including some autoimmune disorders (Karin et al., Citation2006; Martinez et al., Citation2008; Mosser et al., Citation2008). In activated macrophages, nitric oxide (NO) is synthesized by inducible nitric oxide synthase (iNOS) (MacMicking et al., Citation1997; Palmer et al., Citation1988), while cyclo-oxygenase-2 (COX-2) catalyzes the synthesis of prostaglandins (including PGE2) in response to inflammatory stimuli (Williams et al., Citation1999). Modulation of iNOS and COX-2 expression and their products has been reported to be a mechanism of action through which various natural and synthetic anti-inflammatory agents act (Gautam & Jachak, Citation2009; Shi et al., Citation2001). Due to increasing concerns about adverse side-effects from commonly-used synthetics to treat inflammation, there is increasing demand for the development of natural products to targeting these and other cells of the immune system (Kumar et al., Citation2013; McGettigan & Henry, Citation2011).
Accordingly, the impact of medicinal plants/their derivatives on aspects of immune function has been a focus of many researchers seeking to discover efficient compounds with anti-inflammatory and immunomodulatory potentials (Lopez-Varela et al., Citation2002; Zamani Taghizadeh Rabe et al., Citation2015). Even though some studies have already focused on some aspects of the immunomodulatory/anti-inflammatory effects of Ferula spp. and related terpenoid coumarins, further investigation is still needed to uncover underlying mechanisms of effects by these agents. Thus, the present study was conducted to investigate and compare potential anti-inflammatory activities of two terpenoid coumarins from Ferula szowitsiana, i.e. UMB and MG, with regard to effects on macrophage nitric oxide synthase (iNOS) and cyclooxygenase-2 (COX-2) pathways and on splenocyte proliferation and cytokine production.
Materials and methods
Preparation of UMB and MG from Ferula szowitsiana plant
Roots of F. szowitsiana were collected from the mountains of Golestan Province, Iran. The identity of the plant material was confirmed by the Department of Botany at Tehran University and a voucher specimen (No. M1001) deposited at the Department of Pharmacognosy and Biotechnology at Mashhad University of Medical Sciences.
To isolate the test materials, air-dried roots (250 g) were ground into powder and extracted by maceration at room temperature with acetone. After filtration, the extract was concentrated under vacuum to yield ≈20 g brownish residue. Part of the extract (15 g) was subjected to column chromatography on a silica gel (5 × 50 cm) using pet ether containing increasing amounts of acetone (i.e. pet-Me2CO 100:0, 95:5, 90:10, 85:15, 80:20, 75:25, 70:30, 60:40, 50:50 and, finally, 0:100). Each eluate fraction was resolved over a TLC silica gel (using pet ether as solvent) and those fractions giving similar spot patterns were combined.
Endotoxin content of the isolated compounds was determined using a Limulus Amebocyte Lysate (LAL) assay at the Samen Research Institute (Mashhad, Iran); all were reported to be < 0.01 IU/μg. Pure forms of UMB and MG were tested in parallel to confirm sample identity.
Isolation of splenocytes
Splenocytes were isolated from the spleens of healthy naïve Balb/c mice (purchased from Pasteur Institute, Tehran, solely for use in donating spleen/other immune cells). In brief, at necropsy, the spleen of a mouse was removed and cells isolated using a needle perfusion technique with RPMI 1640 medium (GlutaMAX, Gibco, Detroit, MI). The single cell suspension generated was then subjected to sterile Ficol-Hypaque (Sigma, St. Louis, MO) density centrifugation (700 × g, 20 min, 4 °C) to separate mononuclear cells (MNC) from erythrocytes. The MNC at the interface layer were collected, washed twice with ice-cold phosphate-buffered saline (PBS, pH 7.4) by centrifugation (400 × g, 10 min, 4 °C), and then suspended in RPMI 1640 supplemented with 10% heat inactivated fetal bovine serum (FBS), 100 μg/ml streptomycin and100 U/ml penicillin (all Gibco). The total number of cells present was counted and viability determined in a Neubauer chamber (Hamburg, Germany) using Trypan blue dye. Cell viability was routinely >90%.
Splenocyte stimulation assay
The effect of UMB and MG on splenocyte proliferation was evaluated using an MTT colorimetric assay (Mosmann, Citation1983). The different doses of UMB and MG used in these experiments were selected on the basis of our preliminary study and a previously published report (Kohno et al., Citation2011). In brief, splenocytes (4 × 105 cells/well) were dispensed into 96-well plates, and then 0.5–15 μM of UMB or MG was added to dedicated wells (in triplicate). Non-treated controls received RPMI 1640 medium only. To all wells, PHA (Sigma) was then added to achieve a final concentration of 5 ng/ml. After incubation at 37 °C in a humid 5% CO2 atmosphere for 72 h, MTT solution (5 mg/ml) was added to each well and the plate incubated for a further 4 h. The plate was then centrifuged, supernatant from each well was discarded and the cells then lysed with dimethyl sulfoxide (DMSO) to dissolve formazan crystals that had been produced in viable cells. The optical density in each well was measured at 545 nm in a EL plate reader (Convergent Technologies, Marburg, Germany) and cell proliferation then calculated in terms of stimulation index (SI), wherein SI = (OD of PHA-treated cells/OD of non-treated) within each given regimen.
Assessment of splenocyte viability
In parallel with the splenocyte stimulation assay, sets of cells were exposed to the same concentrations of UMB and MG (0.5–15 μM), but without any stimulation by PHA, for 72 h. After this period, cells were checked for viability via trypan blue exclusion and the percentage cell viability was calculated.
Measures of cytokine production using ELISA
To evaluate potential effects on lymphocyte cytokine production, splenic MNC (106 cells/well) were seeded into 24-well plates and then treated with PHA (5 ng/ml) ± 0.5–15 μM of UMB or MG for 72 h at 37 °C. After this period, culture supernatants were collected and stored at −70 °C until measured for IFNγ and IL-4 levels using ELISA kits (R&D Systems, Minneapolis, MN) according to manufacturer protocols. Kit sensitivity was 15 pg IFNγ/ml and 4 pg IL-4/ml.
Effects of test agents on macrophage viability
The viability of peritoneal macrophages exposed to the test agents was assessed via MTT assay (Mosmann, Citation1983). Macrophages were isolated from peritoneal cavities of Balb/c mice by perfusion of the peritoneum with RPMI 1640 and aspiration of the liquid under sterile conditions. The resulting cell suspension was then centrifuged (10 min, 300 x g, 4 °C) and the cell pellet collected. The peritoneal macrophages were re-suspended in complete RPMI 1640 medium, seeded into multi-well plates at assay-driven numbers of cells/well, and incubated for 3 h at 37 °C to permit adherence. Thereafter, the naïve cultures were washed with medium prior to use in experiments.
To assess potential cytotoxicity of the test agents, the cells (2 × 104/well) were treated with increasing (5–30 μM) levels of UMB or MG. Cells exposed to RPMI only were used as the reference viability control for comparisons. After 24 h of incubation, MTT solution (5 mg/ml) was added to each well; 3 h later, the medium was removed and cells were lysed with DMSO to dissolve formazan crystals. The OD in each well was measured at 545 nm in the EL reader and percentage viability calculated as: Viability (%) = 100 × (ODtreated − ODcontrol)/ODcontrol
Induced release of nitric oxide and PGE2
Peritoneal macrophages were seeded in 24-well plates at 5 × 105 cells/well. After 3 h, the cells were treated for 24 h with 5–30 μM of UMB or MG in the presence or absence of 10 ng LPS/ml + 100 ng IFNγ/ml for 24 h (LPS [serotype 0111:B4 from Escherichia coli] and IFNγ, both from Sigma [St Louis, MO]). The combination of IFNγ and LPS was employed for the stimulation of mouse peritoneal macrophages, as previously described in Zamani Taghizadeh Rabe et al. (Citation2014). After this period, the culture medium was recovered; cells were used for analyses of iNOS and COX-2 expression (see below). The culture supernatants were then evaluated for nitrite (marker for NO) using Griess reagent (Green et al., Citation1982). The absorbance at 545 nm was then measured in the EL plate reader. Nitrite concentration (µM) in each sample was quantified by extrapolation from a sodium nitrite standard curve generated in parallel during each experiment. PGE2 levels in the medium (in pg/ml) were assessed using a commercial ELISA (R&D Systems, kit sensitivity = 16 pg PGE2/ml), following manufacturer instructions.
Western blot analysis
Treated and untreated peritoneal macrophages in the plates were lysed in freshly prepared lysis buffer (20 mM HEPES, [pH 7.9], 400 mM NaCl, 0.1% NP-40, 10% glycerol, 1 mM sodium vanadate, 1 mM NaF, 1 mM dithiothreitol (DTT), 1 mM phenylmethylsulfonyl fluoride (PMSF) and protease inhibitor cocktail) for 45 min on ice. The protein concentrations of each cytosolic extract were determined using a Bradford assay. Equal volumes of lysate (containing 30–50 μg protein/ml) were mixed with Laemmli buffer and then loaded into wells of 12.5% sodium dodecyl sulfate-polyacrylamide gel electrophoresis (SDS-PAGE) gels and resolved. Western blotting was performed using a Bio-Rad Mini transblotter by transferring proteins from the gel to a polyvinylidene difluoride membrane at 240 mA for 40 min at room temperature. The membrane was then blocked overnight at 4 °C with 5% skim milk in PBS. After extensive washing with PBS, the membrane was incubated for 2 h at room temperature with primary polyclonal antibodies (rabbit anti-mouse iNOS [1:200 dilution] and rabbit anti-mouse COX-2 [1:500 dilution]). After gently rinsing away any unbound antibodies, each membrane was then incubated with secondary antibody (goat anti-rabbit-horseradish peroxidase-conjugated antibody [1:10000 dilution]) for 1 h at room temperature. β-Actin protein was used as loading control. Subsequently, each blot was extensively washed with PBS and ultimately assessed for antibody binding using enhanced chemiluminescence (ECL) detection reagents (Pierce, Rockford, IL). Each blot was then analyzed using a Kodak Imaging Densitometer (Wayne, NJ).
Statistics
All data are reported as mean ± SEM of three independent determinations; all experiments were performed at least three times. Statistical analysis was performed using analysis of variance (ANOVA) tests and multiple comparisons were made using a Bonferroni’s test. A p value < 0.05 was considered significant.
Results
Effects on splenocyte viability
Assessment of cell viability revealed that none of the doses of UMB or MG employed in these studies (0.5–15 μM) remarkably altered the viability of splenocytes in comparison with that of untreated cells (data not shown).
Effect of terpenoid coumarins on splenocyte proliferation
The proliferation assay outcomes () clearly showed that PHA (5 ng/ml) enhanced naïve mouse splenocyte proliferation. Each test terpenoid suppressed PHA-induced proliferation. UMB only at >0.5 μM decreased the stimulation index in comparison to that with PHA only. However, MG decreased proliferation, even at the lowest test concentration used (0.5 μM).
Figure 2. Effect of terpenoid coumarins on PHA-stimulated splenocyte proliferation. Proliferation of naïve cells exposed to 0.5–15 μM of UMB or MG was measured using MTT method. Results are shown as mean SI values ± SD. Values significantly different versus “0” terpenoid control: *p < 0.05, **p < 0.01 and ***p < 0.001. Values for a fixed dose of UMB and MG significantly differed (p < 0.05) at all doses ≥2.5 µM.
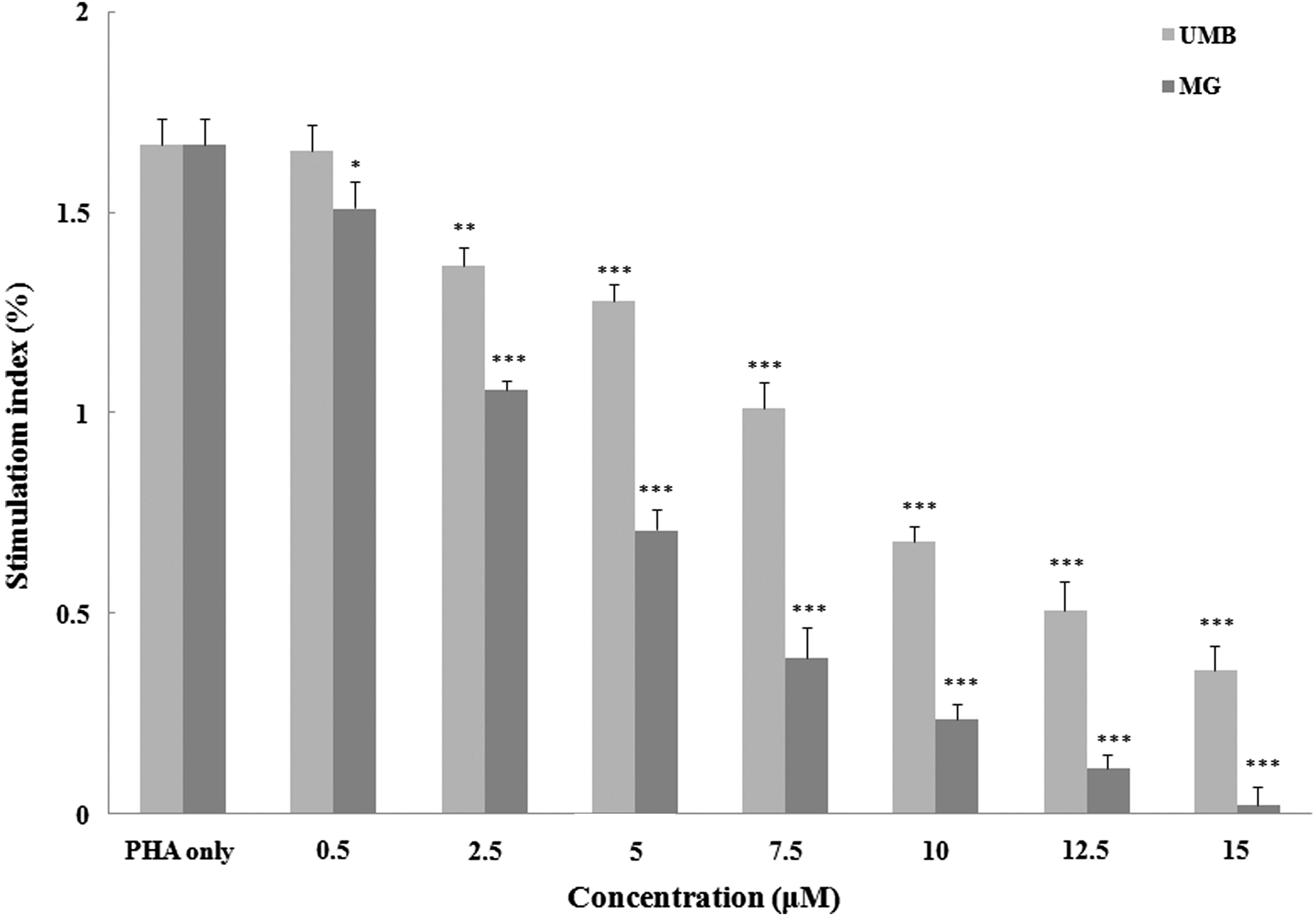
Effects on splenocyte cytokine production
To evaluate effects of the test agents on inducible splenocyte cytokine formation, the concentration of pro- (IFNγ) and anti-inflammatory (IL-4) was measured (). The levels of IFNγ and IL-4 produced by splenocytes after PHA stimulation were increased to 2730 [± 36] and 151 [± 5] pg/ml, respectively, compared with basal levels in cells cultured in medium only of 19.0 [± 0.8] pg IFNγ/ml and 4.2 [± 0.5] pg IL-4/ml.
Figure 3. Effect of terpenoid coumarins on cytokine production by murine splenocytes. (a) IFNγ and (b) IL-4. Naïve mouse splenocytes were treated with PHA (5 ng/ml) ± 0.5–15 μM of UMB or MG for 72 h at 37 °C before culture supernatants were collected for analysis. Results shown are mean ± SD (pg/ml). Values significantly different vs “0” terpenoid control: *p < 0.05, **p < 0.01 and ***p < 0.001. Values for a fixed dose of UMB and MG significantly differed (p < 0.05) at all doses ≥5 µM.
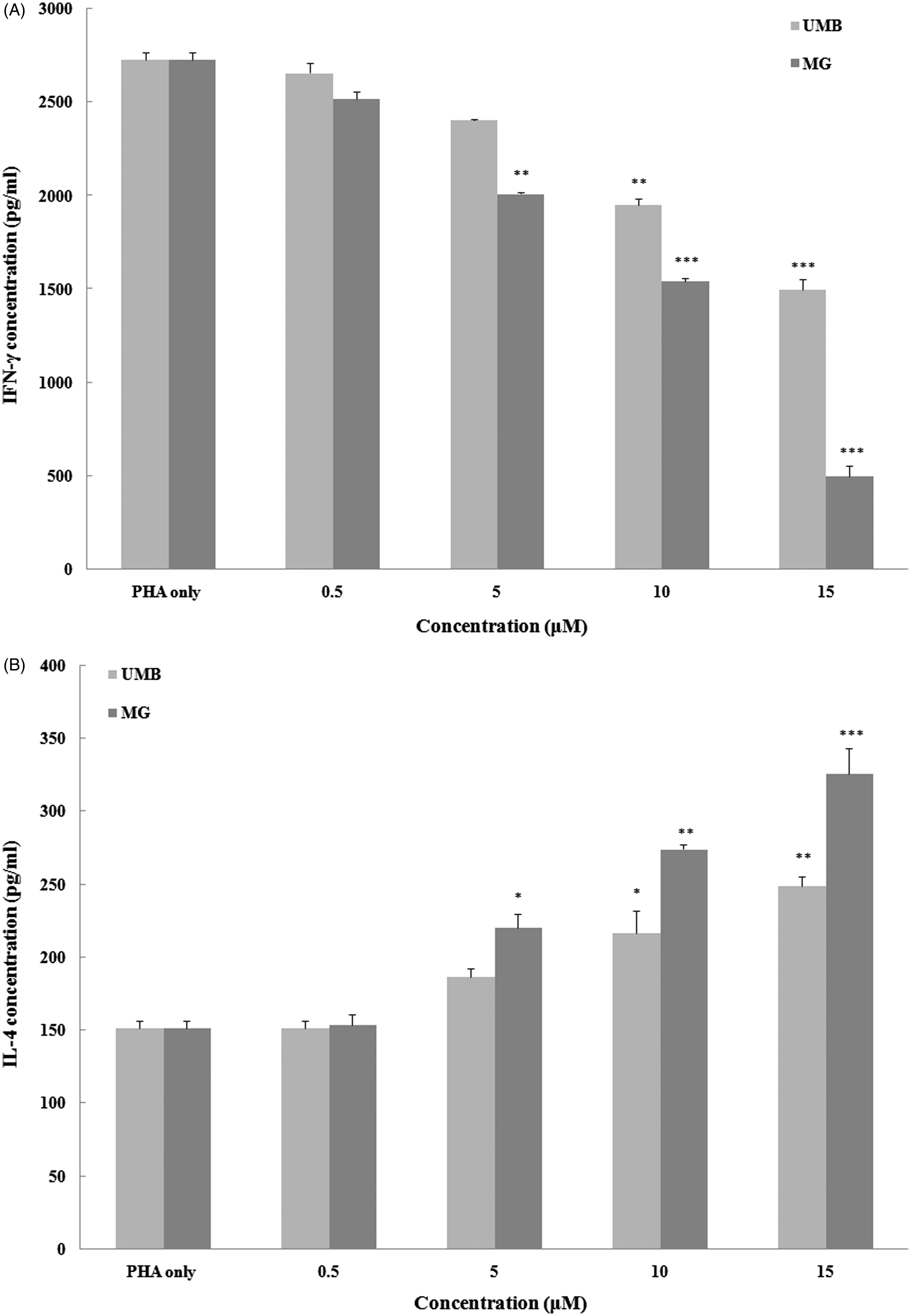
High concentrations (i.e. >10 μM) of UMB and MG were able to significantly abrogate inducible IFNγ production. At 5 µM, only MG had a significant inhibitory impact; neither agent at 0.5 µM impacted on the induced production. In contrast, each agent was able to significantly augment IL-4 production by the cells at ≥10 μM. Again, at 5 µM, only MG had this effect and neither agent altered production at 0.5 µM.
Effects on peritoneal macrophage viability
Peritoneal macrophage viability in the presence of 5–40 μM UMB or MG after 24 h was assessed (). None of the test doses of either agent imparted significant cytotoxicity. As such, concentrations from 5–30 μM of each compound were assumed to be ‘non-toxic’ and used for further studies of potential anti-inflammatory effects here.
Figure 4. Effect of terpenoid coumarins on peritoneal macrophage viability. Naïve macrophages were cultured with either UMB or MG (5–40 μM) for 24 h before viability was assessed using MTT. Results are shown as mean ± SD (% viability). Values significantly different versus “0” terpenoid control: Values for a fixed dose of UMB and MG only significantly differed (p < 0.05) at the two highest doses tested.
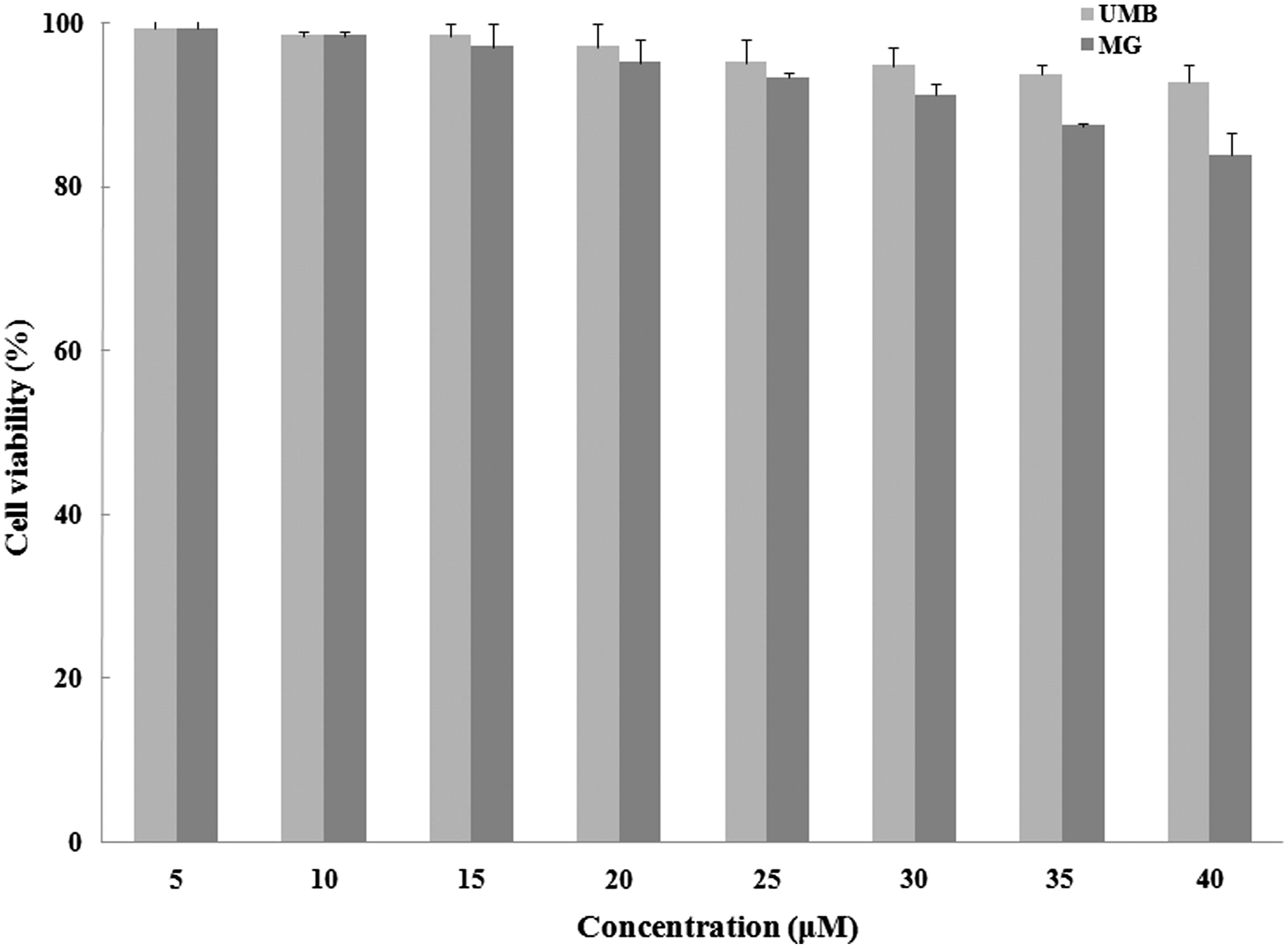
Effects on macrophage LPS-induced NO and PGE2 production
To evaluate any effect of UMB and MG (5–30 μM) on NO production by stimulated peritoneal macrophages, nitrite (index of NO) in culture medium was measured. In the absence of LPS + IFNγ (), basal NO production was 1.6 (± 0.2) µM. Stimulation with LPS + IFNγ increased NO production by the activated macrophages to 27.6 (± 0.1) µM. All concentrations of UMB and MG (except for 5 μM UMB) considerably inhibited NO production by the stimulated macrophages. MG seemed to be more potent in suppressing the inducible NO release.
Figure 5. Effect of terpenoid coumarins on peritoneal macrophage nitric oxide production. Naïve macrophages were stimulated with LPS (10 ng/ml) and IFNγ (100 ng/ml) ± UMB or MG (5–40 μM) for 24 h at 37 °C before culture supernatants were collected for analysis. Results are shown as mean ± SD (µM). Values significantly different vs “0” terpenoid control: *p < 0.05, **p < 0.01 and ***p < 0.001. Values for a fixed dose of UMB and MG significantly differed (p < 0.05) at all doses tested.
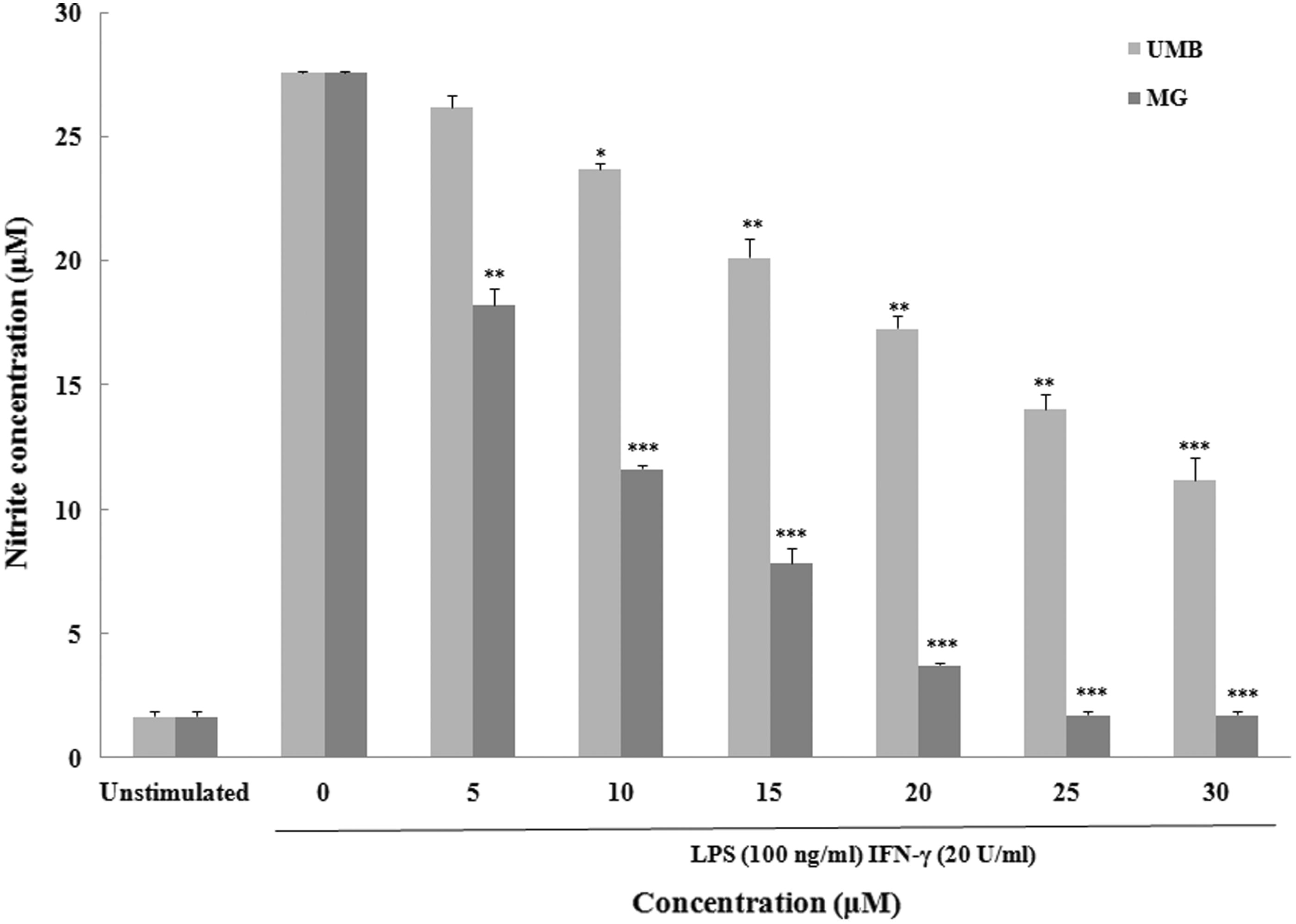
With respect to PGE2, stimulation with LPS + IFNγ caused appreciable increases in PGE2 (6.52 [± 0.10] pg/ml) from unstimulated levels (0.33 [± 0.02] pg/ml) (). Macrophages exposed to UMB exhibited a substantial increase in PGE2 release above the LPS + IFNγ-only levels, but only at >5 μM. In contrast, strong inhibition of PGE2 production was observed with all evaluated levels of MG.
Figure 6. Effect of terpenoid coumarins on peritoneal macrophage PGE2 production Naïve macrophages were stimulated with LPS (10 ng/ml) and IFNγ (100 ng/ml) ± UMB or MG (5–40 μM) for 24 h at 37 °C before culture supernatants were collected for analysis. Results are shown as mean ± SD (µM). Values significantly different vs “0” terpenoid control: *p < 0.05, **p < 0.01 and ***p < 0.001. Values for a fixed dose of UMB and MG significantly differed (p < 0.05) at all doses tested.
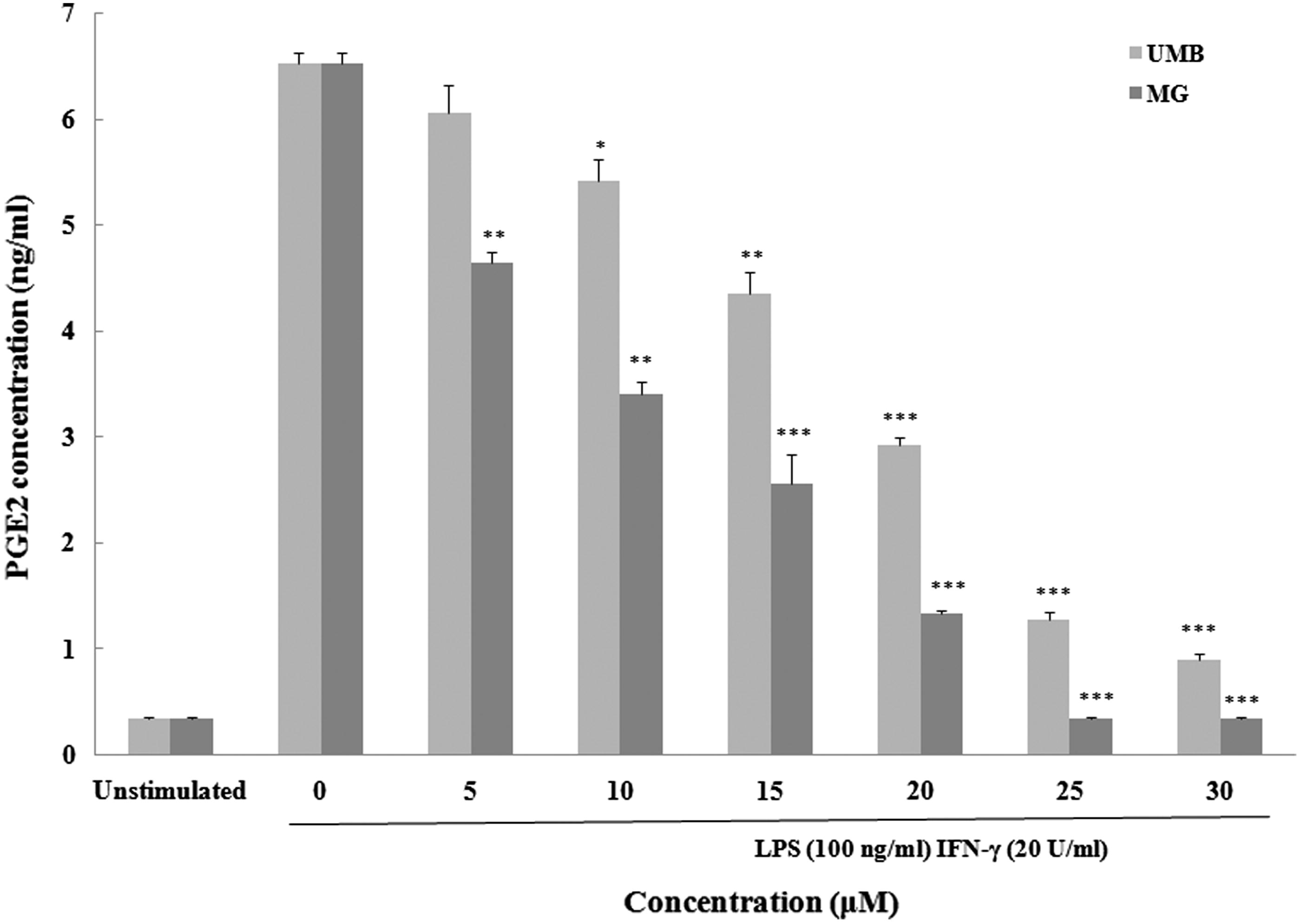
Effects on induced iNOS and COX-2 expression
To determine if UMB and MG could modulate iNOS and COX-2 induction in the macrophages, expression of each enzyme was evaluated by Western blot. Densitometric analyses revealed that UMB and MG (20 μM) were able to attenuate the LPS/IFNγ-induced iNOS and COX-2 expression (). These altered profiles were consistent with the above-noted effects of UMB and MG on NO and PGE2 production.
Figure 7. Effect of UMB and MG (10 and 25 μM) on LPS-/IFNγ-induced iNOS, COX-2 and β-actin expression in cultured macrophages. Lysates were prepared from macrophages incubated for 24 h with LPS (10 ng/ml) + IFNγ (100 ng/ml) alone or in combination with test terpenoid coumarins. Cell lysates were then isolated and subjected to Western blot analyses as outlined in Methods. Picture shown is a representative blot from each indicated regimen.
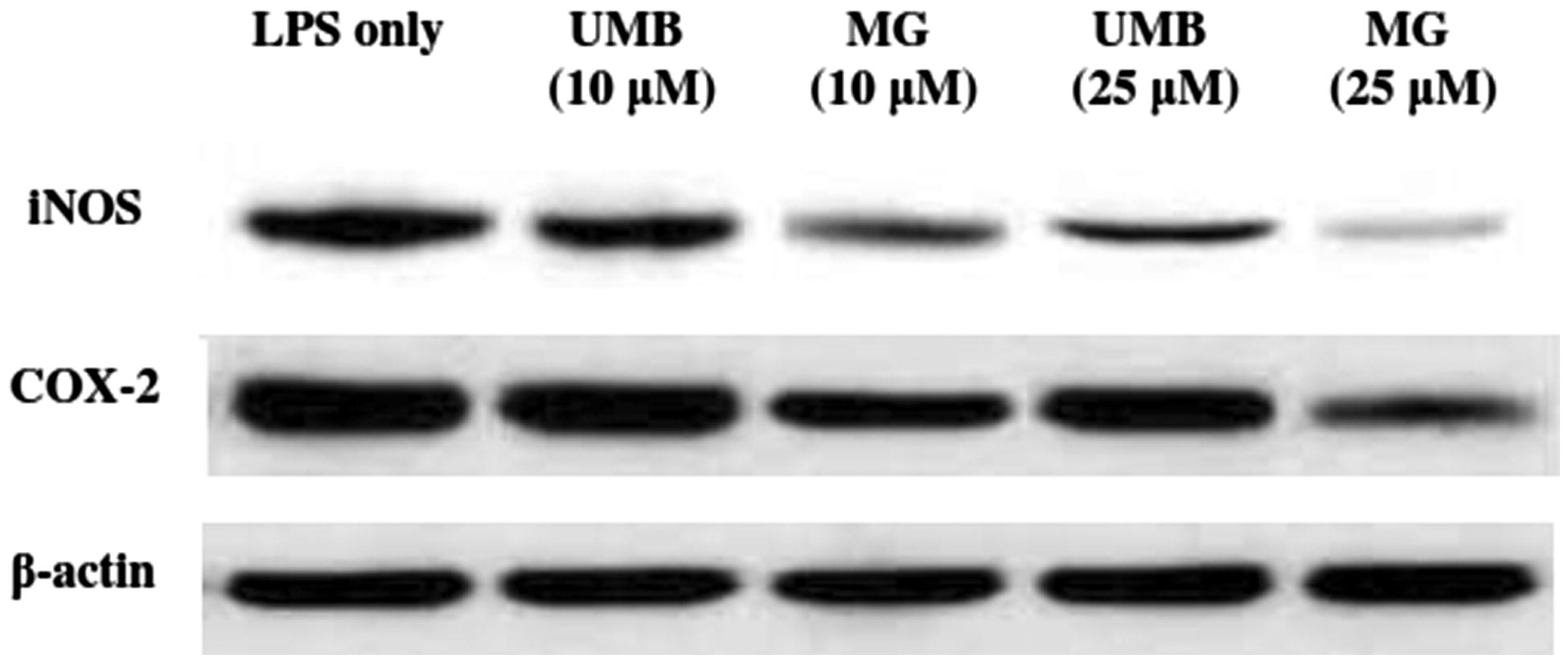
Discussion
Natural compounds from medicinal plants are outstanding candidates for preventive and therapeutical modalities with low toxic side-effects. Considering the relationship between the immune system and health, many researchers have increasingly focused their efforts on identifying traditional plant-derived products to modulate different aspects of the immune system (Craig, Citation1999; Kooy & Sullivan, Citation2013; Tapsell et al., Citation2006). Ferula (Apiaceae) has been employed in traditional medicine against several ailments and some studies have reported a vast range of biological activities with different Ferula species (Iranshahi et al., Citation2007, Citation2008; Kanani et al., Citation2011). Ferula szowitsiana contains several natural compounds, including terpenoid coumarins (Kanani et al., Citation2011; Kurzyna-Mlynik et al., Citation2008). In the present study, the immunomodulatory and anti-inflammatory properties of UMB and MG coumarins were evaluated to elucidate possible mechanisms of action.
To assess the immunomodulant activity of UMB and MG, effects on lymphocytes (splenocytes) and peritoneal macrophages isolated from naïve Balb/c mice were evaluated. Markers of immune function assessed included lymphocyte proliferative responses and cytokine production and the production/release of NO and PGE2 by stimulated macrophages. The present results indicated UMB and MG were capable of suppressing the function of macrophages as evidenced by remarkable decreases in inducible NO production and expression of iNOS, without considerable changes in cell viability. Both UMB and MG were also able to significantly suppress inducible PGE2 release and COX-2 expression. This suggested to us that UMB and MG appeared to act as suppressants of iNOS and COX-2.
Generally, regulation of cell-mediated immune responses is through modulation of the T-helper 1 (TH1)/TH2 balance, cell proliferation and production of related cytokines. Through these mechanisms, various natural compounds could control, prevent or ameliorate different immune reactions (Heikamp & Powell, Citation2012; Saenz et al., Citation2010). The present results showed that PHA stimulation resulted in induction of IFNγ and IL-4 by splenocytes. Excessive production of pro-inflammatory cytokines (like IFNγ) is associated with deleterious inflammatory responses that can lead to tissue damage. In this study, UMB and particularly MG exhibited considerable inhibitory effects on PHA-stimulated proliferation of splenocytes, even at a relatively low concentration. In addition, UMB and MG suppressed considerably the secretion of TH1 cytokine IFNγ and notably stimulated the secretion of TH2 cytokine IL-4. The results with splenocyte cultures here illustrate that UMB and MG might play a critical role in regulating the immune response mechanism by biasing cell-mediated reactions toward TH2. Thus, UMB and MG could be considered potential therapeutics in treatments of autoimmune disorders.
Lipopolysaccharide-activated macrophages produce a large amount of NO and PGE2 via iNOS and COX-2 expression (Zamani Taghizadeh Rabe et al., Citation2014a). In the present experiment, UMB and MG demonstrated an anti-inflammatory capacity against mouse peritoneal macrophages stimulated with LPS through inhibition of iNOS and COX-2 expression, resulting in reduced NO and PGE2 production. The transcription factor NF-κB regulates iNOS and COX-2 inflammatory genes; as such, suppression of iNOS and COX-2 expression via an inhibition of NF-κB activity could prevent/treat human inflammatory diseases by causing reductions in inflammatory mediator release (Li & Verma, Citation2002). The profound inhibition of both iNOS and COX-2 pathways in inflammatory macrophages was noted as the key mechanism by which UMB and MG attenuates inflammatory responses in vitro (di Rosa et al., Citation1996; Pinheiro & Calixto, Citation2002). Furthermore, in a pattern similar to the immunomodulatory results noted here, MG exhibited a stronger anti-inflammatory effect at lower concentrations compared to UMB. This inhibitory effect has important implications for the development of new anti-inflammatory agents and strategies to restrict the inflammatory conditions (Pinheiro & Calixto, Citation2002; Stefanovic-Racic et al., Citation1994).
While both UMB and MG were purified from Ferula szowitsiana – and each differ only in the sidechain ether group linked to the coumarin moiety – MG clearly showed a more potent inhibitory effect on lymphocyte responses (e.g. lymphocyte proliferation, IFNγ production) and also macrophage inflammatory activities (e.g. production of inflammatory mediators [NO and PGE2] and expression of their corresponding generating enzymes [iNOS and COX-2]). Thus, MG should be considered the more potent immunomodulant of the two. Still, one should be careful with regard to the potential use of either of these compounds for immunomodulatory therapies. For example, due to the ability of each to cause increases in release of IL-4, they could help to shift a host (especially pre-disposed individuals) toward TH2-type responses and, potentially then, allergic reactions. Further study of this potential adverse trend needs to be done in animal models.
Overall, the data from the peritoneal macrophage studies are in agreement with previous reports that noted UMB and MG exerted anti-inflammatory effects in RAW264.7 mouse macrophages through an inhibition of NO/PGE2 production and iNOS/COX-2 expression (Iranshahi et al., Citation2008). The present experiment also showed that UMB and MG exerted a suppressive effect on macrophages in a manner similar to that seen in in vitro studies with auraptene (another terpenoid coumarin in Ferula szowitsiana, with chemical structure similar to UMB and MG). It is to be noted that auraptene exhibited anti-inflammatory effects on LPS-and/or IFNγ-induced RAW cells as well as on LPS-induced mouse macrophages (Murakami et al., Citation2000, Citation2003, Citation2005).
In conclusion, the present study provides a basis for the use of UMB and MG as adjuvants to immunomodulatory therapies. It is clear that F. szowitsiana presents a rich source of constituents that could be useful in future studies dealing with the development of novel natural compounds of medical value. However, further studies of the potential (immuno)toxicity of this substance is warranted prior to moving forward with any use in clinical settings.
Declaration of interest
The authors report no conflicts of interest. The authors alone are responsible for the content and writing of the paper.
Acknowledgements
This research was supported by the research council of Mashhad University of Medical Sciences (MUMS) vice president (grant number 89120), Mashhad University of Medical Sciences.
References
- Craig, W. J. 1999. Health-promoting properties of common herbs. Am. J. Clin. Nutr. 70:491–499
- Dehghan, G., Shafiee, A., Ghahremani, M.H., et al. 2007. Anti-oxidant potential of various extracts from Ferula szovitsiana in relation to their phenolic content. Pharm. Biol. 45:691–699
- di Rosa, M., Lalenti, A., Ianaro, A., and Sautebin, L. 1996. Interaction between nitric oxide and cyclo-oxygenase pathways. Prostag. Leukotr. Essen. Fatty Acids 54:229–238
- Gautam, R., and Jachak, S. M. 2009. Recent development in anti-inflammatory natural products. Med. Res. Rev. 29:767–820
- Green, L. C., Glowski, J., Skipper, P. L., et al. 1982. Analysis of nitrate, nitrite and [15N] in biological fluids. Anal. Biochem. 126:131–138
- Hasani-Ranjbar, S., Larijani, B., and Abdollahi, M. 2009. A systematic review of the potential herbal sources of future drugs effective in oxidant-related diseases. Inflam. Allergy Drug Targets 8:2–10
- Heikamp, E. B., and Powell, J. D. 2012. Sensing the immune micro-environment to coordinate T-cell metabolism, differentiation, and function. Seminars Immunol. 24:414–420
- Iranshahi, M., Arfa, P., Ramezani, M., et al. 2007. Sesquiterpene coumarins from Ferula szowitsiana and in vitro anti-leishmanial activity of 7-prenyloxycoumarins against promastigotes. Phytochemistry 68:554–561
- Iranshahi, M., Askari, M., Sahebkar, A., and Hadjipavlou-Litina, D. 2009. Evaluation of anti-oxidant, anti-inflammatory, and lipoxygenase inhibitory activities of prenylated coumarin umbelliprenin. Daru. J. Pharm. Sci. 17:99–102
- Iranshahi, M., Kalategi, F., Rezaee, R., et al. 2008. Cancer chemopreventive activity of terpenoid coumarins from Ferula species. Planta Med. 74:147–150
- Kanani, M. R., Rahiminejad, M. R., Mozaffarian, V., et al. 2011. Chemo-taxonomic significance of essential oils of 18 Ferula species (Apiaceae) from Iran. Chem. Biodivers. 8:503–517
- Karin, M., Lawrence, T., and Nizet, V. 2006. Innate immunity gone awry: Linking microbial infections to chronic inflammation and cancer. Cell 124:823–835
- Kasaian, J., Iranshahy, M., and Iranshahi, M. 2014. Synthesis, biosynthesis, and biological activities of galbanic acid: A review. Pharm. Biol. 52:524–531
- Kohno, S., Murata, T., Sugiura, A., et al. 2011. Methyl galbanate, a novel inhibitor of nitric oxide production in mouse macrophage RAW 264.7 cells. J. Nat. Med. 65:353–359
- Kooy, F., and Sullivan, S. E. 2013. The complexity of medicinal plants: Traditional Artemisia annua formulation, current status, and future perspectives. J. Ethnopharmacol. 150:1–13
- Kumar, N., Drabu, S., and Mondal, S. C. 2013. NSAIDs and selectively COX-2 inhibitors as potential chemoprotective agents against cancer. Arabian J. Chem. 6:1–23
- Kurzyna-Mlynik, R., Oskolski, A. A., Downie, S., et al. 2008. Phylogenetic position of the genus Ferula (Apiaceae) and its placement in tribe Scandiceae as inferred from nrDNA ITS sequence variation. Plant Syst. Evol. 274:47–66
- Li, Q., and Verma, I. M. 2002. NF-κB regulation in the immune system. Nat. Rev. Immunol. 2:725–734
- Lopez-Varela, S., Gonzalez-Gross, M., and Marcos, A. 2002. Functional foods and the immune system: A review. Eur. J. Nutr. 56:29–33
- MacMicking, J., Xie, Q. W., and Nathan, C. 1997. Nitric oxide and macrophage function. Annu. Rev. Immunol. 15:323–350
- Martinez, F. O., Sica, A., Mantovani, A., and Locati, M. 2008. Macrophage activation and polarization. Front. Biosci. 13:453–461
- McGettigan, P., and Henry, D. 2011. Cardiovascular risk with non-steroidal anti-inflammatory drugs: Systematic review of population-based controlled observational studies. Plos Med. 8:1–18
- Mosmann, T. 1983. Rapid colorometric assay for cellular growth and survival: Application to proliferation cytotoxicity assay. J. Immunol. Meth. 65:55–63
- Mosser, D. M., and Edwards, J. P. 2008. Exploring the full spectrum of macrophage activation. Nat. Rev. Immunol. 8:958–969
- Murakami, A., and Ohigashi, H. 2006. Cancer-preventive anti-oxidants that attenuate free radical generation by inflammatory cells. Biol. Chem. 387:387–392
- Murakami, A., Matsumoto, K., Koshimizu, K., and Ohigashi, H. 2003. Effects of selected food factors with chemopreventive properties on combined LPS- and IFNγ-induced IκB degradation in RAW264.7 macrophages. Cancer Lett. 195:17–25
- Murakami, A., Nakamura, Y., Tanaka, T., et al. 2000. Suppression by citrus auraptene of phorbol ester- and endotoxin-induced inflammatory responses: Role of attenuation of leukocyte activation. Carcinogenesis 21:1850–2000
- Murakami, A., Shigemori, T., and Ohigashi, H. 2005. Zingiberaceous and citrus constituents. J. Nutr. 135:2987–2992
- Palmer, R. M., Rees, D. D., Ashton, D. S., and Moncada, S. 1988. L-Arginine is physiological precursor for formation of nitric oxide in endothelium-dependent relaxation. Biochem. Biophys. Res. Commun. 153:1251–1256
- Pinheiro, R. M., and Calixto, J. B. 2002. Effect of the selective COX-2 inhibitors, celecoxib and rofecoxib, in rat acute models of inflammation. Inflamm. Res. 51:603–610
- Saenz, S. A., Noti, M., and Artis, D. 2010. Innate immune cell populations function as initiators and effectors in TH2 cytokine responses. Trends Immunol. 31:407–413
- Shakeri, A., Iranshahy, M., and Iranshahi, M. 2014. Biological properties and molecular targets of umbelliprenin: A mini-review. J. Asian Nat. Prod. Res. 16:884–889
- Shi, F. D., Flodstrom, M., Kim, S. H., et al. 2001. Control of autoimmune response by Type 2 nitric oxide synthase. J. Immunol. 167:3000–3006
- Soltani, F., Mosaffa, F., Iranshahi, M., et al. 2010. Auraptene from Ferula szowitsiana protects human peripheral lymphocytes against oxidative stress. Phytother. Res. 24:85–89
- Stefanovic-Racic, M., Meyers, K., Meschter, C., et al. 1994. N-Monomethyl arginine, an inhibitor of nitric oxide synthase, suppresses the development of adjuvant arthritis in rats. Arthritis Rheum. 37:1062–1069
- Tapsell, L. C., Hemphill, I., Cobiac, L., et al. 2006. Health benefits of herbs and spices: The past, the present, the future. Med. J. Australia 185:4–24
- Williams, C. S., Mann, M., and DuBois, R. N. 1999. The role of cyclo-oxygenases in inflammation, cancer and development. Oncogene 18:7908–7916
- Zamani Taghizadeh Rabe, S., Mousavi, S. H., Tabasi, N., et al. 2014. Rose Bengal suppresses gastric cancer cell proliferation via apoptosis and inhibits nitric oxide formation in macrophages. J. Immunotoxicol. 11:367–375
- Zamani Taghizadeh Rabe, S., Iranshahi, M., Rastin, M., et al. 2015. In vitro immunomodulatory properties of a sesquiterpene lactone-bearing fraction from Artemisia khorassanica. J. Immunotoxicol. 12:223–230