Abstract
An indigenous herbal extract IM-133N containing extracts of Prosopis glandulosa Torr and Symplocos racemosa Roxb were evaluated for potential immunomodulatory effects using RAW264.7 and THP-1 cells. The incubation of the cells for 24 h with IM-133N over a dose range 0–125 µg/ml did not cause cytotoxicity that exceeded 10%. The results indicated that non-cytotoxic doses of IM-133N effectively up-regulated iNOS, TNFα, IL-6, IL-10, IL-8 and IFNγ gene expression in both the RAW264.7 and THP-1 cells. The results also indicated IM-133N elicited dose-related increases in nitric oxide (NO) and tumor necrosis factor (TNF)-α production by RAW264.7 or THP-1 cells. These results demonstrated that IM-133N could stimulate NO and induced pro-inflammatory cytokine expression by monocytes/macrophages. As clinical studies have shown IM-133N to be an effective immunomodulator without any adverse effects, the results of the present study provide further support for the potential use of this agent as an immunostimulant or as an immunotherapy adjuvant.
Introduction
Modulation of the immune response to prevent diseases has long been a topic of interest (Fransisco et al., Citation2012). Macrophages and monocytes play significant roles in host defense mechanisms, as each, when activated, induce production of several cytokines that perform critical functions in a variety of immune responses (Park et al., Citation2013). Nitric oxide (NO) is synthesized by inducible nitric oxide synthase (iNOS) and mediates diverse functions, including vasodilation, neurotransmission and inhibition of platelet aggregation, immunoresponses and inhibition of extracellular matrix production. NO has been identified as a major effector molecule involved in the tumor cell destruction by activated macrophages (Lorsbach et al., Citation1993). Tumor necrosis factor (TNF)-α produced by monocytes/macrophages has also been recognized as an important host defense molecule to kill tumor cells (Gorelik et al., Citation1996; Lasek et al., Citation1997).
Immunomodulation that can be caused by environmental or chemotherapeutic factors is a process that alters the immune system of an organism by interfering with its functions. This process results in immunostimulation – an enhancement of immune reaction – or immunosuppression that results in reduced resistance against infections and stress (Benjamini et al., Citation2000). Use of adjuvants to treat immune-related disorders is a long-standing practice (Gautam et al., Citation2009). Currently, it is estimated that ≈50% of synthetic medicines are derived from phytochemicals. In this context, herbal adjuvants have been seen as promising and safe alternatives to some commonly-employed medications (Sakure et al., Citation2008). Herbal immunomodulators have been used to activate host defense systems that can then be used to support the health of the host during conventional chemotherapy when the immune system is impaired. Many of these herbal adjuvants can be administered along with regular therapeutics to elicit faster stronger immune responses. Herbal preparations have been shown to exert strong immunomodulatory properties including causing increases in cytokine expression, enhanced activation of CD4 and CD8 T-cells (Cho et al., Citation2002; Choi et al., Citation2001; Kim et al., Citation1990) and NK cell activity (Ha, Citation2003).
IM-133N, a herbal preparation containing extracts of Symplocos recemosa Roxb. (Symplocaceae) and Prosopis glandulosa Torr. (Fabaceae), has been reported to impart immunomodulatory effects (Mitra et al., Citation2009; Srivastava et al., 2004). Previous studies on IM-133N established its immunostimulatory properties. IM-133N has been shown to cause increases in the levels of antibody titer, phagocytic index and significant protection against invading pathogens (Mitra et al., Citation1999, Citation2009). Those studies also showed that IM-133N fed to Balb/c mice caused an increase in peritoneal macrophage NO production and phagocytic activity against Candida albicans. Another study showed the clinical efficacy of IM-133 and its role as an immunomodulant in both a mice model and human subjects; no harmful effects were observed by consumption of IM-133N by healthy subjects over a 6-week study period (Srivastava et al., 2004). That study also reported that IM-133N treatment resulted in normalized EAC rosette formation activity and LMI (leukocyte migration inhibition) in hosts with various types of carcinomas; this indicated IM-133N induced immunomodulation in both cellular and humoral immune responses, respectively. Furthermore, there was no decrease in mean plasma concentrations of IgM and IgG, which remained normal (Srivastava et al., 2004).
In the present study, the potential immunostimulatory role of IM-133N in a mouse macrophage cell line (RAW264.7) and human monocyte leukemia line (THP-1) was investigated. The in vitro studies reported here were undertaken to more clearly elucidate the modulatory role of IM-133N on inflammatory cascades and its effect on expression of some key inflammatory mediators (such as NO, TNFα, etc.) that could be involved in induced changes in host humoral and/or cell-mediated immunity.
Materials and methods
Chemicals
Bradford reagent, dexamethasone, RPMI 1640 medium, fetal bovine serum (FBS), 3-(4,5-dimethylthiazol-2-yl)-2,5-diphenyl tetrazolium bromide (MTT), actinomycin D, dexamethasone, custom prepared oligonucelotides, N-1-napthylethylenediamine dihydrochloride, sulfanilamide, sodium nitrite, lipopolysaccharide (LPS, Type 0111:B4 from Escherichia coli) and other reagents were purchased from Sigma (St. Louis, MO). Maloney murine leukemia virus (MMLV) reverse transcriptase, dNTP, and Taq DNA polymerase were purchased from MBI Fermentas (Glen Burnie, MD). Human tumor necrosis factor (TNF)-α ELISA kits were bought from Krishgen Biosystems (Mumbai, India); mouse TNFα ELISA kits were purchased from Invitrogen (Carlsbad, CA).
IM-133N preparation from starting plant materials
The IM-133N test preparation used in these studies contained the extracts of Prosopis glandulosa and Symplocos racemosa. Good agricultural and collection practices (GACP) were employed at the all the times during the procurement and processing of the plant materials. The bark of S. racemosa was collected from various places in Western Ghats in south India. Leaves from P. glandulosa (Syn. Prosopis juliflora) were collected from various places in Tamil Nadu in south India. Collected plants were examined and certified by Dr R. Kannan, a Pharmacognosist at The Himalaya Drug Company (Bangalore, India). Voucher specimens of each plant was archived in the company herbarium.
The extraction process was carried out by powdering both herbs (using a pulverizer) and combining the materials proportionately. The herbal blend was then extracted with water in hot condition (95–100 °C) three times. The extracts were each then filtered under vacuum; each filtrate was then combined, concentrated by evaporation under vacuum and dried using a spray dryer. Yields were routinely 130 mg/g of starting powder mixture (13%).
To confirm that any ability of IM-133N to affect the endpoints measured in this study could not be attributed to any LPS contamination, the IM-133N was tested for the presence of LPS using a Limulus ameobocyte lysate test. The level of LPS in IM-133N was found to be < 10 pg/ml (data not shown).
Mass spectrometer analysis
An API 2000 (Applied Biosystems/MDS SCIEX, Vaughan, ON, Canada) system containing a mass spectrometer coupled with an ESI (electron spray ionization) source and a chromatography system was used to analyze the IM-133N. Batch acquisition and data processing were controlled by Analyst 1.5 version software. Here, a direct infusion (Flow Injection Analysis) method was used wherein the liquid sample was continuously pumped into the electron spray mass spectrometer (ES-MS) via syringe pump. The MS was carried out with a 1 mg IM-133N/ml working sample prepared in purified water (J.T. Baker, Austin, TX). Intensity response was checked in both the positive and negative ionization modes. The good intense response was observed in the negative ionization mode; other parameters used were declustering potential (DP; −80 V), ion spray voltage (IS; −4500 V), nebulizing gas (GS1 and GS2; 30 and 40 psi), curtain gas (CUR; 25 psi), focusing potential (FP; −400 V), source temperature (TEM; 0 °C) and the entrance potential (EP; −10 V) were optimized with respect to the ionization intensity response. A representative mass spectra that was obtained is shown in . Compounds identified by the mass spectroscopy are provided in .
Figure 1. Mass spectra showing compounds of IM-133N extract. Spectrometric conditions are described in the Methods.
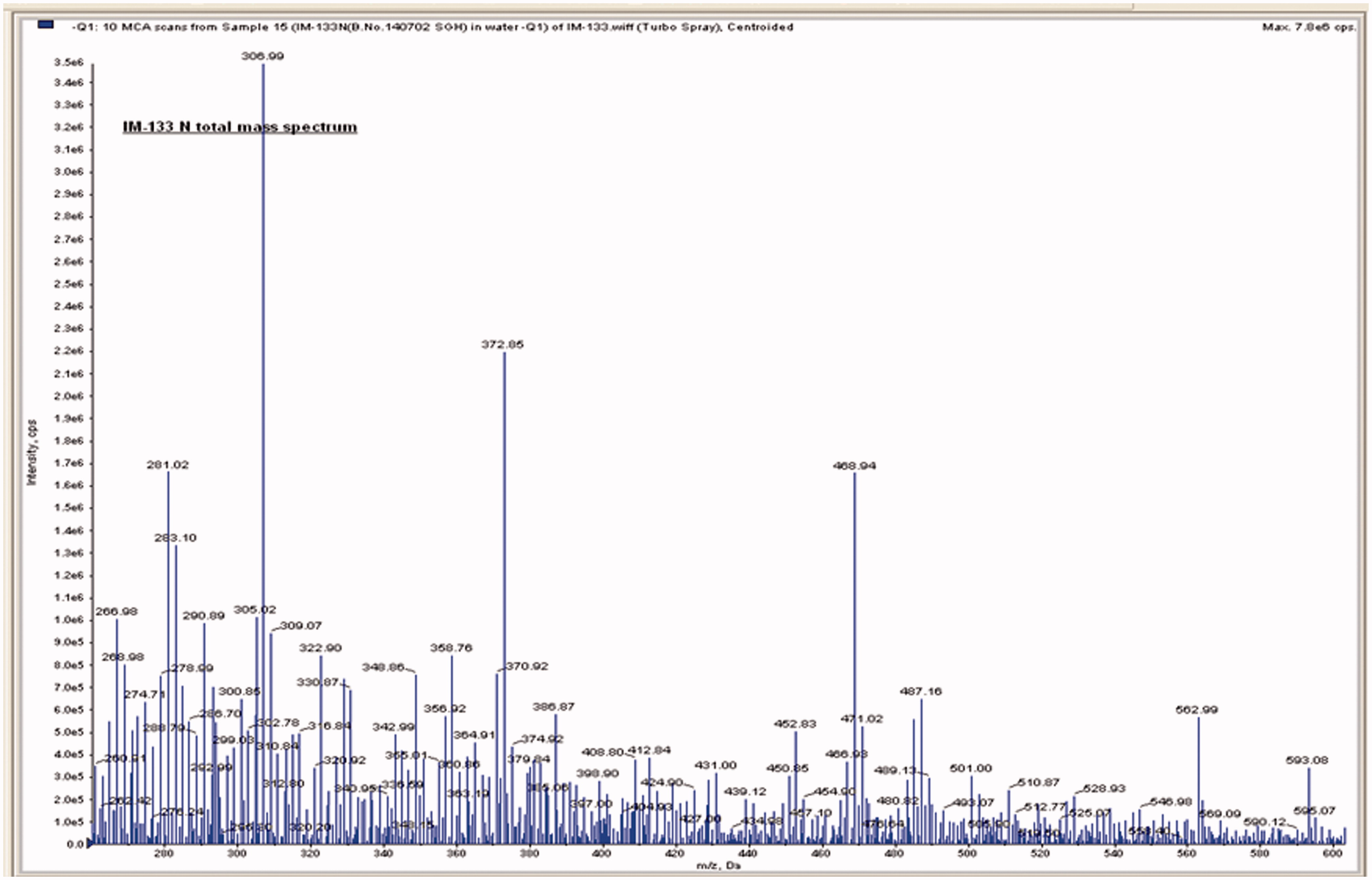
Table 1. List of compounds in IM-133N identified by mass spectrometry.
Cell culture
RAW264.7 cells (mouse macrophage) and THP-1 (human monocytes) were obtained from the National Centre for Cell Science (NCCS, Pune, India). All cells were cultured in 25 cm2 polystyrene flasks (Tarsons, Kolkata, India) under a 5% CO2 atmosphere at 37 °C. The RAW cells were maintained in DMEM high-glucose medium and THP-1 cells in RPMI 1640 media; both media contained 10% FBS, 1% antibiotic-antimycotic solution and 3.7 g sodium bicarbonate/L.
Cell viability
RAW264.7 and THP-1 cells were plated in 96-multiwell culture plates and incubated at 37 °C. To assess the cytotoxicty of IM-133N on the cell lines, the culture medium was discarded after 24 h and fresh medium containing IM-133N at various concentrations (15–1000 μg/ml) was added. An MTT assay was performed after 24 h to assess cytotoxicity (Mosmann, Citation1983). The resultant formazan dye was extracted with DMSO and spectrophotometric readings taken at 540 nm using a Synergy HT plate reader (BioTek, Winooski, VT). From the values obtained, the percentage toxicity (relative to survival of control cells) was calculated. Each assay was performed three times (in triplicate/dose). Only non-toxic concentrations of the drug were used in all subsequent experiments.
Nitrite assay
RAW264.7 cells were cultured at 5 × 105 cells/ml in 24-well plates at 37 °C. The cells were treated with different concentrations of IM-133N (25–100 μg/ml) and incubated for 24 h at 37 °C. Cells that received LPS (1 μg/ml) only or medium only (control) were also evaluated in parallel. The conditioned media was collected and assayed for nitrite content using a Griess reaction, as described in Varma et al. (Citation2011). In brief, equal volumes (50 μl) of 0.1% N-1-naphthylethylenediamine dihydrochloride (in water), 1% sulphanilamide (in 5% phosphoric acid) and cell culture media were mixed in wells of flat-bottom 96-well plates and incubated for 15 min. The colored end-products were then measured at 540 nm in the plate reader. Different concentrations of sodium nitrite in culture media were used as standards; the concentration of nitrite in the samples was then extrapolated from the sodium nitrite standard curve. Each assay was performed three times (in triplicate/dose). Note, THP-1 cells did not produce detectable levels of NO by the Griess reaction and, hence, were not included in this study.
Immunoassay for TNFα
In brief, THP-1 and RAW264.7 cells were grown to confluence in 40-mm dishes at 37 °C. The cells were then treated with IM-133 N at different concentrations (25–100 μg/ml) for 4 h. Cells that received LPS (1 μg/ml) only or medium only (control) were also evaluated in parallel. The plate contents were then collected and centrifuged; the cell-free conditioned medium was assessed for TNFα using commercial ELISA kits, according to manufacturer instructions. The sensitivity of each kit was 3 pg TNFα/ml. Each assay was performed three times (in triplicate/dose).
RNA isolation and RT-PCR
For the RNA extraction, RAW264.7 and THP-1 cells were treated with IM-133 N (25–100 μg/ml) and incubated at 37 °C for 4 h to allow for TNFα gene amplification. For iNOS and the other cytokines investigated, the cells were incubated with IM-133N for 24 h. Cells that received LPS (1 μg/ml) only or medium only (control) were also evaluated in parallel. In each case, total RNA was extracted from the cells using FastPrep-R Mini RNA isolation kit as per manufacturer instruction (Krishgen Biosystems) and the samples were stored at −70 °C. cDNA synthesis as well as semi-quantitative RT-PCR for GAPDH, iNOS and other cytokines was performed as described previously (Mitra et al., Citation2008; Varma et al., Citation2011). PCR was performed in a final reaction volume of 25 μl following addition of specific primer pairs and Taq DNA polymerase using protocols described in Varma et al. (Citation2011). Primers used for the amplification are listed in the . PCR products were then resolved over 1.5% agarose gels and visualized by ethidium bromide staining. Expression of all genes of interest was normalized against GADPH expression. Quantification of PCR products was performed by densitometric analysis using Image Quant software (Microsoft, Santa Rosa, CA). Each assay was performed three times (in triplicate/non-cytotoxic dose).
Table 2. Primers used for amplifications.
Statistics
All results were expressed as mean ± SD. Statistical significance of the nitrite, TNFα and RT-PCR outcomes was determined using one-way analysis of variance (ANOVA) included in a Prism4 software package (Graph Pad, San Diego, CA). Results were considered significantly different from one another at p values < 0.05.
Results
IM-133N effects on NO and TNFα formation by cultured cells
IM-133N was evaluated for its cytotoxicity in RAW264.7 and THP-1 cells via an MTT assay; incubation of the cells for 24 h with IM-133N over a dose range 0–125 µg/ml did not cause cytotoxicity that exceeded 10% (). Among the tested concentrations below 125 µg/ml, doses of 25–100 µg/ml IM-133 N were both non-cytotoxic and effectively induced significant NO production by the RAW264.7 cells (). Basal NO formation by untreated RAW264.7 cells was < 2.5 μM. NO production after the 24-h exposures to 25, 50 or 100 μg/ml treatments reflected a dose-related trend for increases in NO levels of, respectively, 13.01 [± 5.03], 27.42 [± 3.29] and 51.12 [± 4.97] μM NO. LPS, a potent macrophage activator, induced synthesis of 67.91 [± 6.56] μM NO.
Figure 2. Cytotoxicity of IM-133N on (a) RAW264.7 and (b) THP-1 cells. Cells were incubated for 24 h with differing concentrations of IM-133N and cell viability was then determined using an MTT assay. Data are expressed as mean (±SE) cytotoxicity (i.e. percentage reduction in viable cell number vs control) from three assays using n = 3 samples/IM-133N concentration.
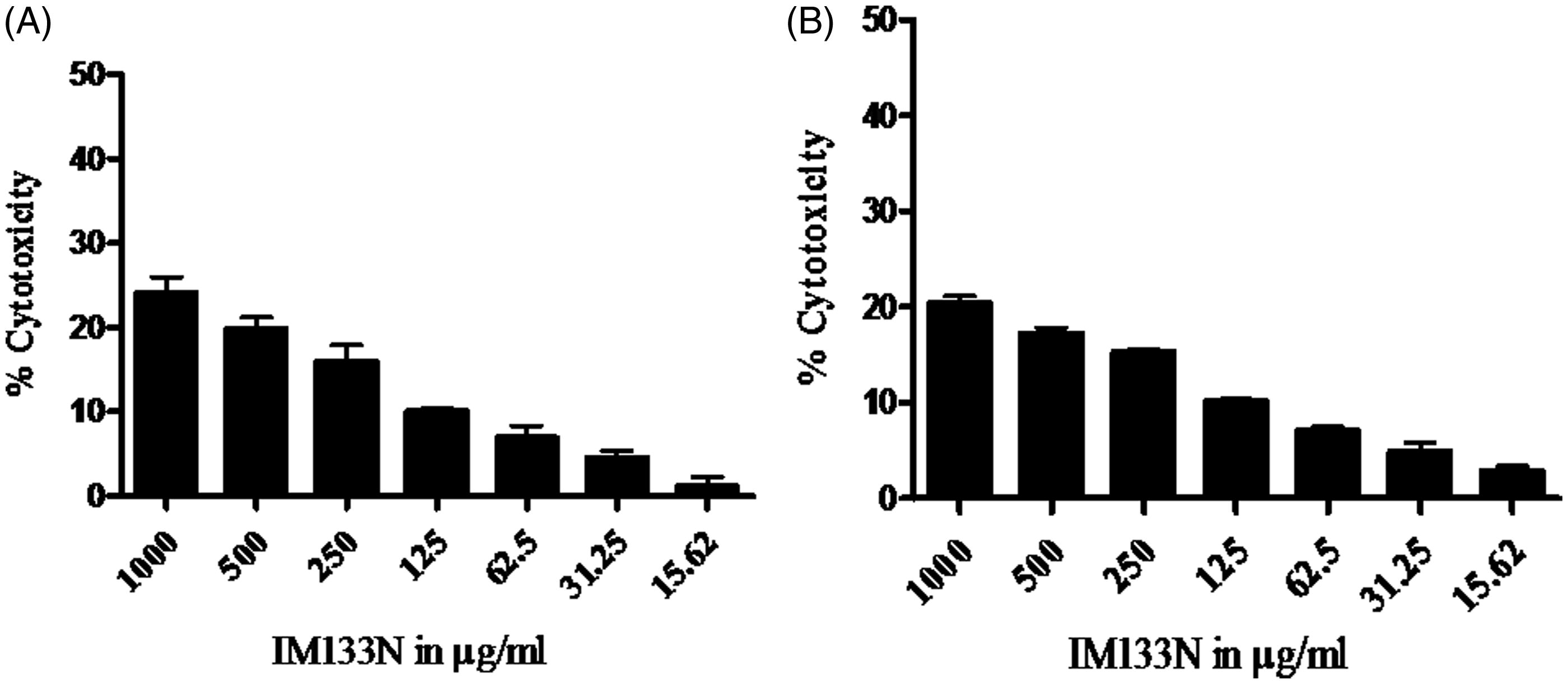
Table 3. Effect of IM133N on NO and TNFα secretion.
Inducible TNFα formation was also assessed in the treated cells (). Preliminary studies here showed that, when RAW264.7 and THP-1 cells were cultured in the presence of IM-133N, TNFα was rapidly secreted (i.e. within 4 h). Thus, unlike the other studies here, measures of TNFα release were performed after just 4 (not 24) h of incubation with IM-133N. The results showed that doses of 25–100 µg/ml IM-133N were non-cytotoxic and effectively induced significant TNFα production by the RAW264.7 and THP-1 cells in the 4-h timeframe. Basal TNFα formation by both types of untreated cells was ≤ 1 pg/ml. After the 25, 50 and 100 μg/ml treatments, RAW264.7 cell TNFα production was, respectively, 8.99 [± 0.42], 11.52 [± 0.82] and 20.58 [± 1.05] pg/ml; LPS induced synthesis of 25.09 [± 2.33] pg TNFα/ml. After the same IM-133N treatments, TNFα production by THP-1 cells was, respectively, 6.12 [± 0.76], 10.09 [± 0.91] and 19.31 [± 1.86] pg/ml; LPS induced synthesis of 23.77 [± 2.91] pg TNFα/ml.
To confirm that the ability of IM-133N to induce NO and TNFα could not be attributed to any LPS contamination, IM-133N was tested for a presence of LPS. As the level of LPS was << 10 pg/ml, this indicated that all of the induced production of NO and TNFα by IM-133N was not due to any exogenous LPS contaminants.
Effect of IM-133N on cytokine gene expression in THP-1 and RAW 264.7 cells
To determine if IM-133N regulated NO and TNFα secretion at the mRNA level, RT-PCR was carried out. TNFα gene expression was found to be elevated in IM-133N-treated (25, 50 or 100 µg/ml) RAW264.7 () and THP-1 () cells (relative to that in control cells) after 4 h of incubation. While the effects appeared to be more consistently dose-related only in the RAW264.7 cells, induction by the two lower doses tested only nominally enhanced gene expression compared to that of control cells. In comparison, while there was no apparent dose-associated effect among the THP-1 cells, each dose of IM-133N still appeared to have induced a notable increase in TNFα expression. As expected, LPS (1 μg/ml) also increased TNFα mRNA levels in both lines.
Figure 3. Densitometric analysis of gene transcripts obtained from RAW264.7 cells. Cells were incubated with the IM-133N for 4 h (for TNFα gene amplification) or for 24 h (for iNOS and the other cytokines investigated) before being harvested. Relative levels of each gene’s expression were normalized to that for GAPDH. Values shown depict arbitrary units. Values are mean ± SD of three individual experiments using n = 3 samples/IM-133N concentration. *Value significantly different from control or # from LPS control (p < 0.05).
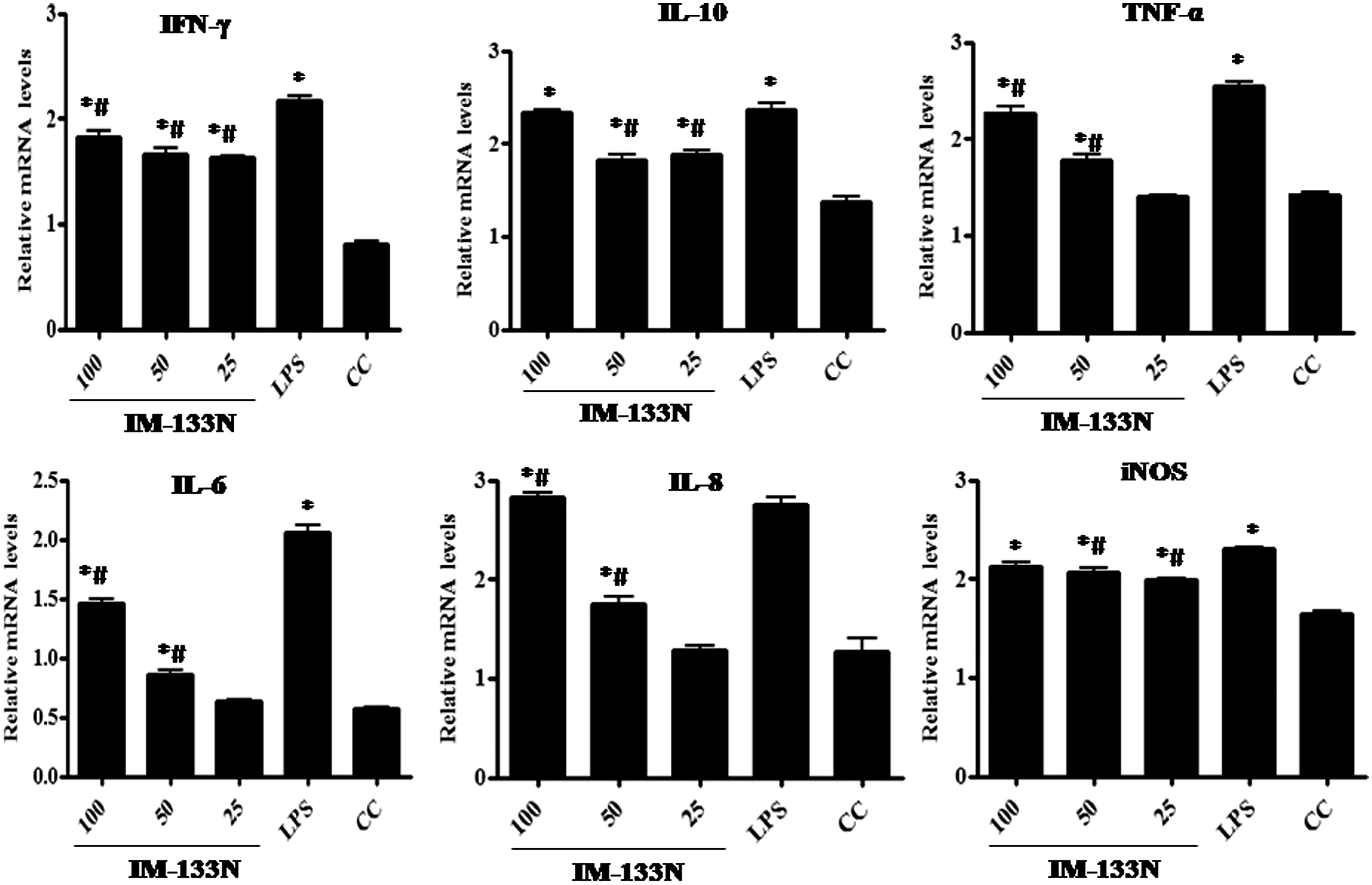
Figure 4. Densitometric analysis of gene transcripts obtained from THP-1 cells. Cells were incubated with the IM-133N for 4 h (for TNFα gene amplification) or for 24 h (for the other cytokines investigated) before being harvested. Relative levels of each gene’s expression were normalized to that for GAPDH. Values shown depict arbitrary units. Values are mean ± SD of three individual experiments using n = 3 samples/IM-133N concentration. * Value significantly different from control or # from LPS control (p < 0.05).
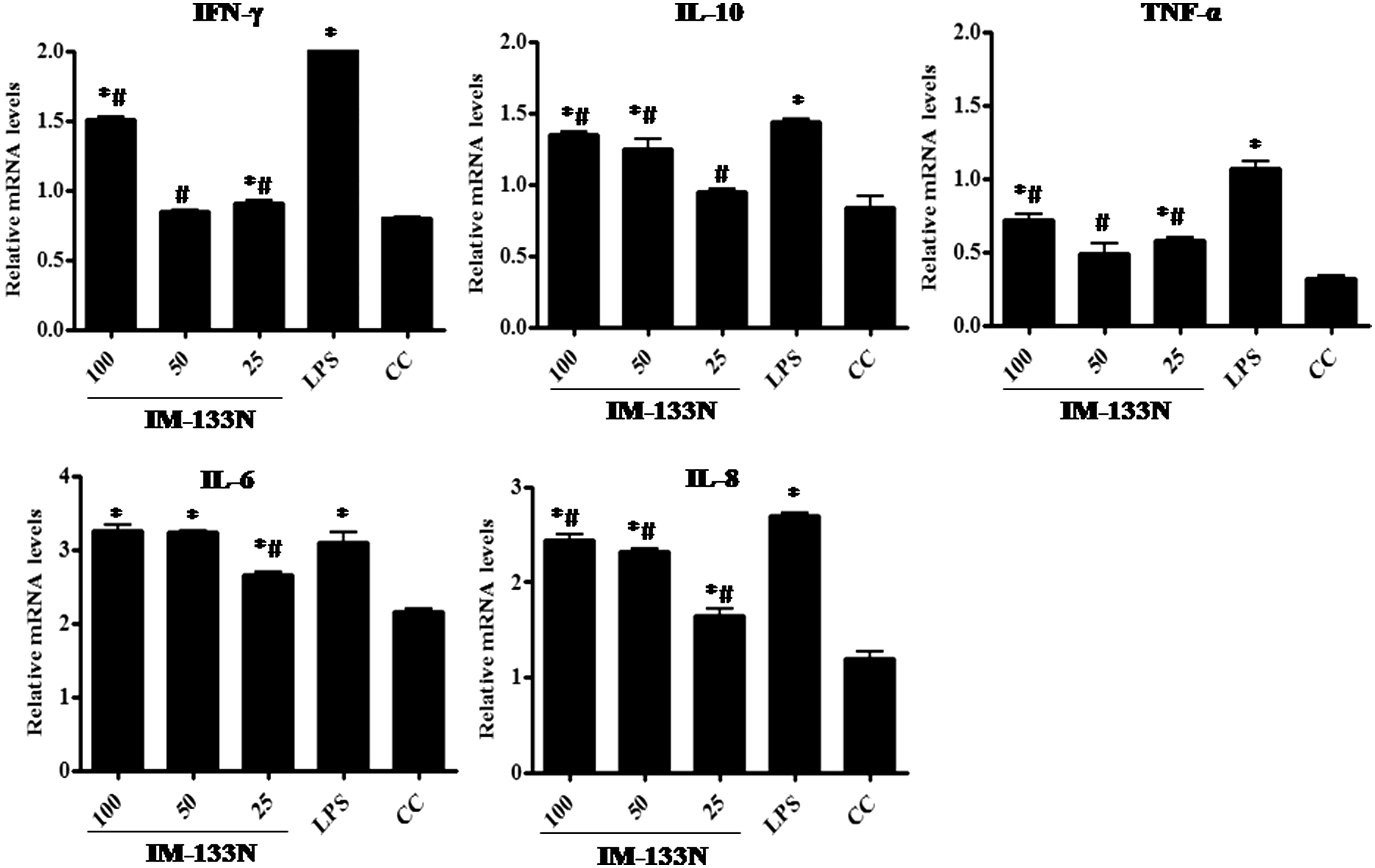
Consistent with the results from the NO assay, iNOS mRNA levels in the RAW264.7 cells were markedly increased by IM-133N treatment after 24 h of incubation (). However, although there seemed a case for a dose-related pattern of effect (i.e. all three doses of IM-133N used induced increases in iNOS expression), the pattern was not like that seen in the cell measures of NO itself. It is possible that this dichotomy might be explained by the fact that, by assessing iNOS expression and NO levels associated with the cells, the present study failed to account for likely accumulation of NO in the medium over the incubation period, while the RT-PCR reflected a single timepoint snapshot of gene expression. In either case, it is clear that the IM-133N treatment caused augmented formation of the NO by the RAW264.7 cells.
To analyze effects of IM-133N on expression of other key pro-inflammatory cytokines, RT-PCR was carried out using oligo dT primers for first stand synthesis followed by mouse and human-specific gene primers for PCR amplification. The results shown in and reflect what might be a “dose-related” increase (between 25–50 µg/ml IM-133N doses) in the mRNA expression of IL-6 and IL-8 genes in both cell lines, as compared to expression levels in corresponding control cells. This same pattern was only evident for the IL-10 gene in the THP-1 cells. With each line, any “dose-related” effect on IFNγ gene expression only was evident as the dose increased from 50 µg/ml to 100 µg/ml IM-133N, although, again, the effect was more apparent in the THP-1 cells. LPS, as expected, up-regulated the gene expression of each test cytokine in both cell lines.
As was done for TNFα here, our ongoing studies are performing measures of the products of each of these assayed genes to better discern if the IM-133N also results in changes in the actual formation/release of these key immunomodulating proteins. Those results will be presented in a follow-on publication about the immunotoxic effects of IM-133N.
Discussion
In chronic and acute infections, cytokines mediate these immunological responses and also maintain immune homeostasis (Liu et al., Citation2002). Immune system cells are equipped to synthesize and release a variety of cytokines that signal other (both immune and non-immune) cells and trigger them to become either more (up-regulated) or less (down-regulated; modulated) active (Balkwill, Citation2000). Among these cytokines are interferons (IFN), interleukins (IL), colony-stimulating factors (CSF), tumor necrosis factor (TNF) and transforming growth factors (TGF) (Roitt et al., Citation1998). The distinctive pattern and effect of each cytokine depends on concentration-dependent binding to specific receptors on the surface of target cells and subsequent activation of cellular machinery, in some cases, at the level of the nucleus and genetic machinery.
Compounds/drugs that may act as adjuvants and alter the synthesis of various immune signaling molecules during immune responses are of immense importance in the designing of therapeutic strategies (Gautam et al., Citation2009). Immune-related diseases have long been treated with herbal medicines (Spelman et al., Citation2006; Tan & Vanitha, Citation2004). Herbal immunomodulators perhaps might be safe and help in modulating immune responses (You et al., Citation2001). In regard to phytotherapy, immunomodulators may be defined as botanical medicines that alter the activities of the immune system via the dynamic regulation of informational molecules, such as cytokines, hormones, neurotransmitters and other peptides. In the past 20 years, hundreds of traditional and herbal medicines with immunomodulating functions have been screened. Of these, most induced modulation of expression of multiple cytokines including IL-1, IL-6, TNFα and IFNγ (Spelman et al., Citation2006). For example, the methanol extract of Echinacea purpurea caused an increase in production of IL-6 and IL-10 by human and murine macrophages (Burger et al., Citation1997; Rininger et al., Citation2000). Similarly, a glycoprotein fraction from rice bran (Oryza sativa) strongly increased the production of TNFα, IL-6 and IL-10 by RAW264.7 cells (Park et al., Citation2013).
IM-133N contains the extracts of Symplocos recemosa and Prosopis glandulosa. The S. racemosa extract contains many alkaloids, e.g. loturine, colloturine, loturidine, symplocososide, symploveroside and salireposide (Bhusnar et al., Citation2014; Hanumant et al., Citation2014). Two phenolic glycosides, Symconoside A and Symconoside B, have also been isolated from this plant (Ahmad et al., Citation2005). It is well documented that phenolic glycosides have strong immunostimulant properties (Lakshmi et al., Citation2003). The extract of P. glandulosa contains gallic acid, quercetin, apigenin, coumaric acid, luteolin, catechin and epicatechin gallate (Andrade et al., Citation2013; Bisby, Citation1994). Quercetin and gallic acid are also known to immunomodulate responses by mouse macrophages (Liao & Lin, Citation2014; Yadav et al., Citation2012). Alkaloids like indolizidine and galactomannan have also been reported present in Prosopis spp. (Michael, Citation2008); these alkaloids and related compounds have immunostimulatory properties (Wijesekera, Citation1991). Therefore, in the present study, that IM-133N was able to impact on various immunological markers associated with human monocytes (THP-1) and mouse macrophage (RAW264.7) cells in vitro – including formation of TNFα and nitric oxide (NO) – was not unexpected.
Among the various markers analyzed here, NO has been proposed to function as a potent tool in the immune response and a key molecule produced by macrophages for elimination of pathogens (Park et al., Citation2013). The fact there was enhanced NO production by RAW cells here clearly showed that IM-133N could probably affect transcription of various signaling genes normally regulated by NO. For example, it was previously shown that NO synthase inhibition causes increases in pro-inflammatory cytokine protein/mRNA expression, in part because NO normally causes decreases in NF-κB available for binding to regulatory regions of those genes (Walley et al., Citation1999). These results also suggested that secretion of NO and TNFα was regulated by the same mechanism(s), or that TNFα (which is produced first) may have affected NO formation via some autocrine or paracrine mechanism.
Consistent with the results obtained for effects on cell expression of NO and TNFα, IM-133N also affected the expression of genes for other cytokines, including IL-6, -8 and -10 and IFNγ in the RAW264.7 and THP-1 cells. IL-6 is a pro-inflammatory cytokine that, along with TNFα and IL-1, is induced by LPS. Immunostimulant activity of Heracleum maximum was reported, where the aqueous root extracts stimulated IL-6 levels in mouse macrophages (Webster et al., Citation2006). IL-8 has an important role in activating neutrophils and to potentiate chemotaxis. IL-10, produced by a variety of cells (including T- and B-lymphocytes, monocytes), is a key immunomodulatory cytokine capable of mediating immunosuppressive and immunostimulating effects (Fujii et al., Citation2001). The immunomodulatory effects of Oenothein B isolated from Epilobium augustifolium and Uncaria tomentosa on the regulation of IL-6, IL-10 and IL-8 was shown by Schepetkin et al. (Citation2009) and by Fransisco et al. (Citation2012). IFNγ secretion is associated with protective responses against intracellular pathogens, including parasites, bacteria and viruses. IFNγ is also both an inducer and a product of NK cells; it is also released by many types of stimulated macrophages (Fenton et al., Citation1997; Schindler et al., Citation2001; reviewed in Schroder et al., Citation2004). Immunostimulating activities of Rauwolfia serpentina were demonstrated wherein aqueous root extracts of the plant increased IFNγ expression by human T-cells (Jin et al., Citation2002). Similarly, leaf ethanol extracts of Cissampelos sympodialis increased IFNγ expression in spleen cells (comprised of mixture of lymphocytes/macrophages, etc.) (Piuvezam et al., Citation1999). That the IM-133N was able to modulate expression of genes for these cytokines in both macrophage–monocyte cell lines would, thus, be in keeping with effects of other herbal materials.
Biological response modifiers are widely known to potentiate therapeutic efficacy or alleviate toxicity of other agents (You et al., Citation2001). Because of the pivotal role of NO and TNFα in many immune responses (i.e. antimicrobial, tumoricidal), significant efforts have been undertaken to develop therapeutic agents that regulate production/secretion of these molecules (Poderoso et al., Citation1999). High levels of cytokines such as IFNγ, TNFα, IL-2 and IL-12 tend to favor the induction of cell-mediated immune responses, whereas high levels of cytokines like IL-4, -5, -6 and -10 tend to favor humoral immune response induction. The present study results are significant in that the induction of IFNγ, IL-6 and IL-10 could also potentially be regulated by IM-133N, i.e. secondary to effects on the various cytokines/NO assayed herein.
Conclusions
In general, the results of the present study raise the possibility that IM-133N may cause shifts in immune responses based on levels of cytokine production. Hence, judicious use of IM-133N at appropriate concentrations during treatment is recommended to bring about a positive immune response and not an untoward one – such as allergy and/or autoimmunity.
Acknowledgments
The authors are thankful to Dr Shyam Ramakrishnan, Chief Scientific Officer, The Himalaya Drug Company, for providing support and encouragement. The first author is thankful to Dr D. Palaniyamma and Dr Abheepsa Mishra, The Himalaya Drug Company for valuable suggestions and editing the manuscript.
Declaration of interest
The authors report no conflicts of interest. The authors alone are responsible for the content and writing of the paper.
References
- Ahmad, V. U., Zubair, M., Abbasi, M. A., et al. 2005. Phenolic glycosides from Symplocos racemosa. Z. Naturforsch. 60:1101–1104
- Andrade, M. G, Laredo, R. F., Guzman, N. E., et al. 2013. Mesquite leaves (Prosopis laevigata), a natural resource with anti-oxidant capacity and cardioprotection potential. Ind. Crops Prod. 44:336–342
- Balkwill, F., (Ed.). 2000. The Cytokine Network. Oxford: Oxford University Press
- Benjamini, E., Coico, R., and Sunshine, G., (Eds.). 2000. Immunology; A Short Course, 4th Edition. Toronto: Wiley-Liss
- Bhusnar, H. U., Nagore, D. H., and Nipanikar, S. U. 2014. Phytopharmacological profile of Symplocos racemosa. A review. Pharmacologia 5:76–83
- Bisby, F., (Ed.). 1994. Phytochemical Dictionary of Leguminosae. London: Chapman and Hall
- Burger, R. A., Torres, A. R., Warren, R. P., et al. 1997. Echinacea-induced cytokine production by human macrophages. Intl. J. Immunopharmacol. 19:371–379
- Cho, J. Y., Kim, A. R., Yoo, E. S., et al. 2002. Ginsenosides from Panax ginseng differentially regulate lymphocyte proliferation. Planta Med. 6:497–500
- Choi, C. Y., Kim, J. Y., Kim, Y. S., et al. 2001. Aqueous extract isolated from Platycodon grandiflorum elicits release of nitric oxide and TNFα from murine macrophages. Intl. Immunopharmacol. 1:1141–1151
- Fenton, M. J., Vermeulen, M. W., Kim, S., et al. 1997. Induction of IFNγ production in human alveolar macrophages by Mycobacterium tuberculosis. Infect. Immun. 65:5149–5156
- Fransisco, V., Liberal, J., Ferreira, J., et al. 2012. Immunostimulant activity of Uncaria tomentosa and its tannins. Planta Med. 78:PD9
- Fujii, S., Shimizu, K., Shimizu, T., and Lotze, M. T. 2001. IL-10 promotes maintenance of anti-tumor CD8+ T-cell effector function in situ. Blood 98:2143–2151
- Gautam, M., Saha, S., Bani, S., et al. 2009. Immunomodulatory activity of Asparagus racemosus on systemic TH1/TH2 immunity: Implications for immuno-adjuvant potential. J. Ethnopharmcol. 121:241–247
- Gorelik, L., Bar-Dagan, Y., and Mokyr, M. B. 1996. Insight into mechanism(s) through which TNF promotes generation of T-cell-mediated anti-tumor cytotoxicity by tumor-bearer splenic cells. J. Immunol. 156:4298–4308
- Ha, C. L. 2003. The inhibitory effect of the Chinese herb Ganoderma lucidum mycelium on gut immunoglobulin A responses to cholera toxin in mice. Nutr. Res. 23:691–701
- Hanumant, U. B., Dheeraj, H. N., and Sanjay U. N. 2014. Phytopharmacological profile of Symplocos racemosa: A Review. Pharmacologia 5:76–83
- Jin, G. B., Hong, T., Inoue, S., et al. 2002. Augmentation of immune cell activity against tumor cells by Rauwolfia radix. J. Ethnopharmacol. 81:365–372
- Kim, J. Y., Germolec, D. R., and Luster, M. I. 1990. Panax ginseng as a potential immune-modulator: Studies in mice. Immunopharmacol. Immunotoxicol. 12:257–276
- Lakshmi, V., Pandey, K., Puri, A., et al. 2003. Immunostimulant principles from Curculigo orchioides. J. Ethnopharmacol. 89:181–184
- Lasek, W., Feleszko, W., Golab, J., et al. 1997. Anti-tumor effects of the combination immuno-therapy with IL-12 and TNFα in mice. Cancer Immunol. Immunother. 45:100–108
- Liao, Y. R., and Lin, J. Y. 2014. Quercetin, but not its metabolite quercetin-3-glucoronide, exerts prophylactic immunostimulatory activity and therapeutic anti-inflammatory effects on LPS-treated mouse peritoneal macrophages ex vivo. J. Agric. Food Chem. 62:2872–2880
- Liu, E., Moriyama, H., Abiru, N., et al. 2002. Anti-peptide auto-antibodies and fatal anaphylaxis in NOD mice in response to insulin self-peptides B:9-23 and B:13-23. J. Clin. Invest. 110:1021–1027
- Lorsbach, R. B., Murphy, W. J., Lowenstein, C. J., et al. 1993. Expression of the nitric oxide synthase gene in mouse macrophages activated for tumor cell killing. Molecular basis for the synergy between IFNγ and LPS. J. Biol. Chem. 268:1908–1913
- Michael, J. P. 2008. Indolizidine and quinolizidine alkaloids. Nat. Prod. Rep. 25:139–165
- Mitra, S. K., Gupta, M., Suryanarayana, T., and Sarma, D. N. 1999. Immunoprotective effect of IM-133. Intl. J. Immunopharmacol. 21:115–120
- Mitra, S. K., Saxena, E. and Dixit, M. N. 2009. Natural immunostimulant compositions, methods for obtaining the same and pharmaceutical formulations thereof. US Patent No. 7948131
- Mitra, S. K., Varma, R. S., Ashok, G., and Nandakumar, K. S. 2008. Liv.52 regulates TNFα and PPARγ expression in HepG2 cells. Mol. Cell. Biochem. 315:9–15
- Mosmann, T. 1983. Rapid colorimetric assay for cellular growth and survival: Application to proliferation and Cytotoxicity assays. J. Immunol. Methods 65:55–63
- Park, H. Y, Yu, A. R., Choi, I. W., et al. 2013. Immunostimulatory effects and characterization of a glycoprotein fraction from rice bran. Intl. Immuno-pharm. 17:191–197
- Piuvezam, M. R., Pecanha, L. M., Alexander, J., and Thomas, G. 1999. Cissampelos sympodialis Eichl. leaf extract increases the production of IL-10 by Concanavalin-A-treated BALB/c spleen cells. J. Ethnopharmacol. 67:93–101
- Poderoso, J. J., Carreras, M. C., Schopfer, F., et al. 1999. The reaction of nitric oxide with ubiquinol: Kinetic properties and biological significance. Free Radic. Biol. Med. 16:925–935
- Rininger, J. A., Kickner, S., Chigurupati, P., et al. 2000. Immuno-pharmacological activity of Echinacea preparations following stimulated digestion on murine macrophages and human peripheral blood mononuclear cells. J. Leukocyte Biol. 68:503–510
- Roitt, I., Brostoff, J., and Male, D., (Eds.). 1998. Immunology. 5th Edition. Philadelphia, PA: Mosby
- Sakure, S., Negi, V. D., Mitra, S. K., et al. 2008. Vaccine with herbal adjuvant - A better cocktail to combat infection. Vaccine 26:3387–3388
- Schepetkin, I. A., Kirpotina, L. N., Jakiw, L., et al. 2009. Immunomodulatory activity of Oenothein B isolated from Epilobium augustifolium. J. Immunol. 183:6754–6766
- Schindler, H., Lutz, M. B., Röllinghoff, M., and Bogdan, C. 2001. The production of IFNγ by IL-12/IL-18-activated macrophages requires STAT4 signaling and is inhibited by IL-4. J. Immunol. 166:3075–3082
- Schroder, K., Hertzog, P. J., Ravasi, T., and Hume, D. A. 2004. IFNγ: An overview of signals, mechanisms, and functions. J. Leukocyte Biol. 75:163–189
- Spelman, K., Burns, J. J., Nichols, D., et al. 2006. Modulation of cytokine expression by traditional medicines: A review of herbal immunomodulators. Alter. Med. Rev. 11:128–150
- Srivastava, A. N., Singh, U., and Kolhapure, S. A. 2004. Evaluation of clinical efficacy and safety of IM-133N as an immunomodulator in various carcinomas: A prospective clinical trial. Indian J. Clin. Pract. 15:25–37
- Tan, B. K., and Vanitha, J. 2004. Immunomodulatory and anti-microbial effects of some traditional Chinese medicinal herbs: A review. Curr. Med. Chem. 11:1423–1430
- Varma, R. S., Godavarthi, A., Vidyashankar, S., et al. 2011. Bresol inhibits phosphodiesterase-4 gene expression and modulates the levels of select mediators of inflammation in human monocytic cells. J. Immunotoxicol. 8:315–323
- Walley, K. R., McDonald, T. E., Higashimoto, Y., and Hayashi, S. 1999. Modulation of pro-inflammatory cytokines by nitric oxide in murine acute lung injury. Am. J. Respir. Crit. Care Med. 160:698–704
- Webster, D., Taschereau, P., Lee, T. D., and Jurgens, T. 2006. Immunostimulant properties of Heracleum maximum Bartr. J. Ethnopharmacology 106:360–363
- Wijesekera, R. O., (Ed.). 1991. The Medicinal Plant Industry. Boca Raton, FL: CRC Press
- Yadav, D. K., Khan, F., and Negi, A. S. 2012. Pharmacophore modeling, molecular docking, QSAR, and in silico ADMET studies of gallic acid derivatives for immunomodulatory activity. J. Mol. Model 18:2513–2525
- You, H. J., Choi, C. Y., Kim, J. Y., et al. 2001. Ursolic acid enhances nitric oxide and TNFα production via NF-κB activation in resting macrophages. FEBS Lett. 509:156–160