Abstract
The immunotoxic effects of the isoquinoline alkaloid berberine (BBR) were investigated in Balb/c mice. Here, BBR was administered daily by intraperitoneal injection at doses of 5 and 10 mg/kg for 14 days. Following the exposure, host spleen weight, cellularity and histopathology, as well as delayed-type hypersensitivity (DTH) responses, hemagglutination titers (HA), spleen cell subtype profiles, splenocyte cytokine production and lymphocyte proliferation were studied in all of the test groups of animals. The results showed that the high dose of BBR (10 mg/kg) could suppress both cellular and humoral immune functions in the treated hosts. BBR at 5 mg/kg only appeared to impact on DTH responses and lymphoproliferation. Based on the finding here, it would seem that BBR has effective immunosuppressive properties. Mechanistic studies are required to determine exactly how this material is acting to impart many of the immunotoxic effects demonstrated here. At the same time, further research should also be performed on BBR to further develop its potential use as an effective immunosuppressant or co-adjuvant for the treatment of diseases caused by an exaggerated or unwanted immune response.
Introduction
The body is protected against non-self antigens by the immune system. If the immune response is suppressed, the risks of infection and the formation of certain cancers are increased (Bendich, Citation1993). Over the past decades, natural compounds have become important candidates for investigation of the immunosuppression/stimulation of widely used natural compounds (Farsam et al., Citation2011; Noori et al., Citation2010; Rahnama et al., Citation2014). Since ancient times, people have sought cures for illness in nature. Application of natural medicines has intensified in recent time due to a low level of adverse effects and price, as well as a good efficacy against several human illnesses (Hasani-Ranjbar et al., Citation2009). Berberine (2,3-methylenedioxy-9,10-di-methoxyproto-berberine chloride) is an isoquinoline alkaloid with a wide range of pharmacologic and biochemical effects and is the major active constituent in plants like Berberis spp. and goldenseal (Kheir et al., Citation2010). In traditional medicine, berberine has been repeatedly used as an anti-biotic, -diarrhea, -hypertension, -arrhythmia and -cancer agent (see Letasiova et al., Citation2005).
Evidence has indicated that berberine also may impart some immunomodulatory effects (Ivanovska & Philipov, Citation1996; Ivanovska et al., Citation1999; Marinova et al., Citation2000; Xu et al., Citation2005). For example, in an investigation of anti-inflammatory effects of Berberis vulgaris root extract and its alkaloid fractions in mice, a total ethanol extract of the plant imparted the highest reducing effects on acute inflammation (i.e. in carrageenan- and zymosan-induced paw edema models). This extract was also most effective in alleviating signs of adjuvant-induced arthritis (a chronic inflammatory model). In addition, proto-berberine fractions and isoquinoline alkaloid berberine (BBR) were able to suppress delayed-type hypersensitivity (DTH) responses to sheep red blood cells in rodent hosts (Ivanovska & Philipov, Citation1996). In an autoimmune tubulo-interstitial nephritis model induced by injection of mice with bovine tubular basement membrane antigen, BBR was effective in reducing the intensity of pathologic injuries (Marinova et al., Citation2000). In a study by Ivanovska et al. (Citation1999), BBR suppressed in vitro proliferative responses to concanavalin A (ConA) and phytohemagglutinin-A (PHA) by mouse splenocytes. Surprisingly, in another part of that study, contrary to other findings, BBR enhanced murine DTH reactions against Candida albicans antigen.
Because the above-noted studies only investigated the effects of BBR on just a few aspects of the immune system, a comprehensive study seemed necessary, one that was based on the tiered-approach to immunotoxicity screening (Luster et al., Citation2003). Such studies are also needed in that, as noted above, some data were conflicting, thus restricting the potential wider use of BBR as a therapeutic immunomodulant. Thus, due to this overall lack of information about the immunotoxic effects of berberine, the present study was undertaken to evaluate such effects following a set of subacute exposures in mice.
Materials and methods
Animals
Balb/c mice (male, 19–21 g, 6–8-weeks-of-age) were purchased from the Pasteur Institute (Tehran, Iran) and housed in standard laboratory conditions (25 [±2]°C and 40–70% relative humidity) with a 12-h day/night lighting cycle throughout the experimental period. The mice were kept in spacious polypropylene cages and provided ad libitum access to standard rodent chow and filtered water. All mice were allowed to acclimate for 1 week prior to initiation of any experiment. The Ethnic Council of Mashhad University of Medical Sciences (Mashhad, Iran) approved all protocols used in this study.
Doses and treatment schedules
Dedicated sets of mice were used for each of the experimental protocols outlined below (). For each set of studies, mice were randomly divided into four groups (n = 24/group). Mice in the BBR experimental groups were injected intraperitoneally (IP) daily with a berberine solution (98%: Sigma, St. Louis, MO; prepared in 5% dimethyl sulfoxide [DMSO]/phosphate-buffered saline [PBS, pH 7.4] solution) in order to receive 5 or 10 mg BBR/kg/day. Mice in the positive control group received cyclophosphamide (Sigma) at 20 mg/kg/day. Mice in the vehicle control group received only DMSO/PBS injections. No injection volume ever exceeded 100 µl. Mice were dosed each day for 14 days. The doses of berberine used here were based on the study of Tsang et al. (Citation2013), demonstrating the anti-carcinogenic effects of doses of 5 or 10 mg berberine/kg in mice. The dose of cyclophosphamide was selected based on studies of Farsam et al. (Citation2011) and Rahnama et al. (Citation2014).
Determination of the hematological parameters
Blood was collected from the retro-orbital plexus of each mouse before they were euthanized by cervical dislocation. The blood (0.2 ml) was collected into sterile (K-EDTA) anti-coagulated tubes to permit total WBC (white blood cell) determinations. A blood smear was also prepared, stained with Giemsa dye and then examined under a light microscope for differential analyses (based on counts of at least 200 cells/slide/mouse).
Measurement of spleen weight and cellularity
Twenty-four hours after the final dosing (i.e. on Day 15), mice (n = 6/group) were euthanized by cervical dislocation and the spleen of each was aseptically removed and weighed. Thereafter, single cell suspensions were prepared in RPMI 1640 medium using standard protocols (Riahi et al., Citation2010). Specifically, each spleen was placed into a small petri dish including 10 ml RPMI 1640 media supplemented with 10% fetal bovine serum (FBS), 100 U penicillin/ml, 100 µg streptomycin/ml and 2 mM L-glutamine (all Gibco, Paisley, UK). Each spleen was teased between two frosted slides; the released splenocytes were separated using a cell strainer and then were centrifuged at 1200 rpm at 4 °C for 10 min. The supernatant was discarded and the pellet was re-suspended in 3 ml of RBC lysing buffer containing 0.83% [w/v] NH4Cl in 100 mM Tris buffer [pH 7.4] and kept at room temperature for 3 min. The cells were then washed three times with medium and finally suspended in 1 ml of medium containing 10% FBS; these cells were then used in assays for lymphocyte proliferation, inducible cytokine formation and for lymphocyte subtype evaluations outlined below. Cell viability was assessed via trypan blue exclusion; viability was routinely > 90%. Measures of total cell counts were obtained using a Neubauer chamber (Gibco).
Histologic/cytopathologic analysis of the femur, thymus and spleen
Twenty-four hours after the final dosing (i.e. on Day 15), separate dedicated subsets of mice (n = 6/group) were euthanized by cervical dislocation and the femur, thymus and spleen of each mouse were collected. The spleen and thymus were fixed in 10% formalin solution. After mounting, 5-µm thick sections (three different sections per each tissue) of each tissue were stained using Hematoxylin & Eosin (H&E) dye solution. From the femurs that were collected, bone marrow smears (three smears/mouse) were prepared and stained using H&E solution. Histologic and cytologic changes in each examined set of samples was then assessed in a blinded manner via light microscopy and scored based on the degree of changes. Observations were scored as: negative = no changes observed; mild = minimal observable changes; positive (+) = readily detectable changes; and positive (++) = extensive changes.
Hemagglutination (HA) titer assay
Four days before ending the treatment period (i.e. on Day 10), additional dedicated subsets of mice in each regimen (n = 6/group) were immunized by IP injection of 5 × 108 SRBC (in saline; Pouyanazma, Mashad, Iran). Injection of BBR was continued until Day 14. At the termination of the experiment (i.e. Day 15), after preparing sera from collected blood samples, aliquots (50 µl) of 2-fold dilutions of the sera (in PBS) were combined with 50 µl of a 2% [v/v] SRBC suspension in a glass tubes. The tubes were then incubated at 37 °C for 2 h and checked for hemagglutination (button formation). The highest dilution giving hemagglutination was taken as the antibody titer.
Delayed-type hypersensitivity (DTH) response
Delayed-type hypersensitivity response (DTH) was determined for treatment and control groups using the method of Fararjeh et al. (Citation2008). On Day 9 of treatment, final dedicated subsets of mice in each regimen (n = 6/group) were immunized IP (100 µl) with 109 sheep red blood cells (SRBC). Five days later (i.e. Day 14 of dosing regimen), these mice were challenged with a booster dose of 108 (50 µl) SRBC in the left hind footpad. The right hind footpad was injected with the same volume of PBS to serve as trauma control for non-specific swelling. Footpad volumes were measured 24, 48 and 72 h after the SRBC challenge using a vernier caliper (Mitutoyo, Tokyo, Japan). Mean percentage increase in footpad thickness was calculated as 100 × (Left footpad [challenged with SRBC] volume − Right footpad volume)/Right footpad volume).
Lymphocyte proliferation test
An MTT [3-(4,5-diamethyl-2-thiazolyl)2,5-diphenyl-2H-tetrazolium] colorimetric assay was used to evaluate the cell proliferation. In brief, aliquots (100 µl) of the splenocytes isolated above (starting sample was pre-diluted in medium to 4 × 106 cells/ml) were dispensed into 96-well micro-plates. Thereafter, phytohemagglutinin-A (PHA [Sigma], at a final concentration of 5 µg/ml/well), lipopolysaccharide (LPS [Escherichia coli Type 0111:B4, Sigma], at a final level of 1 μg/ml/well) or complete media was added to triplicate designated wells. The cultures were then incubated for 72 h at 37 °C (under 5% CO2) and then cell proliferation was assessed using an MTT assay. For this, 15 µl of a 5-mg/ml solution of MTT was added to each well and the plates incubated at 37 °C for a further 4 h. The blue formazan precipitate that formed within each cell was then dissolved by addition of 100 µl DMSO and the optical density in each well was measured at 570 nm in a Stat-Fax Elisa Reader (Stat-Fax, Palm City, FL). Based on absorbance values, a stimulation index (SI) for each sample was calculated as absorbance for stimulated cells/absorbance for unstimulated cells – for each given group that received either LPS or PHA.
Measurement of cytokine production
In a separate set of cultures, aliquots of the isolated splenocytes (2 × 106 cells/well) were placed into each well of 24-well plates and then PHA (5 mg/ml; final concentration) was added to stimulate the cells. The cells were incubated for 72 h at 37 °C before well supernatants were collected and frozen at −70 °C until analyzed. Commercial ELISA assay kits (R&D Systems, Minneapolis, MN) were used to measure levels of interleukin (IL)-4 and interferon (IFN)-γ, according to manufacturer instructions. Level of sensitivity of the kits was 2 pg IL-4/ml and 1 pg IFNγ/ml.
Spleen cell subtyping
Splenocyte single-cell suspensions in RPMI 1640 (106 cells/ml) prepared above had their levels of CD19+, CD3+, CD4+ and CD8+ cell subtypes determined with the aid of a FACS Calibur flow cytometer (BD, San Jose, CA) in a central collaborative laboratory that performs these analyses for all investigators at the Bu-Ali Research Institute (Mashhad, Iran). For these analyses, cells were stained using a mouse T-lymphocyte subset antibody cocktail kit (with isotype control) containing PE-Cy7-hamster anti-mouse-CD3e, PE-rat anti-mouse-CD4, FITC rat anti-mouse-CD8 (BD Pharmingen, San Diego, CA) or with FITC [rat]-anti-mouse-CD19 (with isotype control, eBioscience, San Diego, CA), according to manufacturer protocols. A minimum of 10 000 events/sample was acquired for each sample. The absolute number of each cell type in each spleen was determined by multiplying the differential ratio of the subtypes by the total spleen cell contents (Riahi et al., Citation2010). Levels of T-lymphocyte subsets and CD19 cells in the splenocyte suspensions were separately determined.
Statistical analysis
All data (expressed as mean ± SE) were analysed using a Student’s t-test to determine significant differences among the various groups for a given endpoint. Data were analysed using INSTAT software (GraphPad, Inc., San Diego, CA). A p value < 0.05 was considered significant.
Results
Effects of berberine on hematologic parameters
None of the doses of BBR caused mortality in the present studies. BBR at a dose of 10 mg/kg/day was able to significantly decrease WBC (p < 0.01) as well as neutrophil (p < 0.05) and lymphocytes (p < 0.01) count. However, WBC and leukocyte differential counts did not change significantly at a dose of 5 mg/kg/day (). Cyclophosphamide (CYP) caused very significant (p < 0.001) reductions in WBC and lymphocyte levels and also affected the levels of monocytes in these hosts (p < 0.01). While the drug caused an increase in neutrophil levels, the effect was not statistically significant. Compared to the positive control, the effects induced by the BBR were different for the WBC, neutrophil and lymphocyte endpoints, with levels of significance being greater with the lower BBR dose.
Table 1. Effects of subacute exposure to berberine (BBR, IP daily, 14 days) on blood cellularity.
Effects on spleen weight, cellularity and histopathology
Of all the treatments, only BBR at 10 mg/kg/day was able to significantly (p < 0.05) decrease spleen weight relative to that in mice that received vehicle only (). Oddly, while both treatments with BBR at 10 mg/kg/day and CYP caused reductions in total cellularity in the organ, neither value reached statistical significance (BBR, p = 0.063; CYP, p = 0.096). Analyses of the various sub-populations in the spleen indicated that treatments with BBR at 10 mg/kg/day or CYP significantly reduced both the percentage (p < 0.05) and absolute levels (p < 0.01) of CD19+ cells. While neither regimen affected the percentage\absolute levels of CD3+ cells, within that population, each significantly (p < 0.05) impacted the percentage/absolute levels of CD8+ cells. Oddly, only the absolute numbers – but not the overall percentage – of CD4+ cells was significantly (p = 0.048) impacted by the 10 mg BBR/kg/day; while mice in the CYP regimen had lower values, the SE among the outcomes resulted in a p value > 0.05. Accordingly, the CD4:CD8 ratios in mice in those two groups were very significantly elevated (p < 0.01). Compared to control host values, treatment with 5 mg BBR/kg/day appeared to only significantly affect the CD4:CD8 ratio, with the impact mostly due to a non-significant (≈45%) reduction in CD8+ cells. In fact, in many cases, the effects of the 5 mg BBR/kg/day treatments were nominal as compared to control values; this lack of effects resulted, in the cases of spleen weight, the percentage of CD8+ cells and absolute numbers of CD4+, CD8+ and CD19+ cells, in values for the 5 mg BBR/kg/day hosts being closer to the controls and, thus, significantly (p < 0.05) different from those of their 10 mg BBR/kg/day or CYP counterparts.Footnote1
Table 2. Effects of subacute exposure to berberine (BBR, IP daily, 14 days) on mouse spleen cell subtypes.
Spleen samples from the treated hosts were also analyzed for the presence of white pulp atrophy (or hyperplasia), measures of red pulp:white pulp ratios and/or the existence of clumps, debris, necrosis and/or apoptosis in the white and red pulp. Splenic trabecular disorders were also investigated. The analyses revealed that BBR at 10 mg/kg/day induced mild splenic white pulp atrophy (). These changes were not noted in the 5 mg/kg/day regimen. Hosts treated with CYP only had a mild atrophy in their splenic white pulp (data not shown).
Figure 2. Pathologic changes in spleen after administration of 10 mg BBR/kg/day. (a) Vehicle-control group: White pulps with well-formed lymphoid follicles containing active germinal centers. (b) 10 mg BBR/kg/day group: Atrophic white pulps with relatively small lymphoid follicles. Each figure is a representative photomicrograph from a mouse in each group. Magnification = 100×.
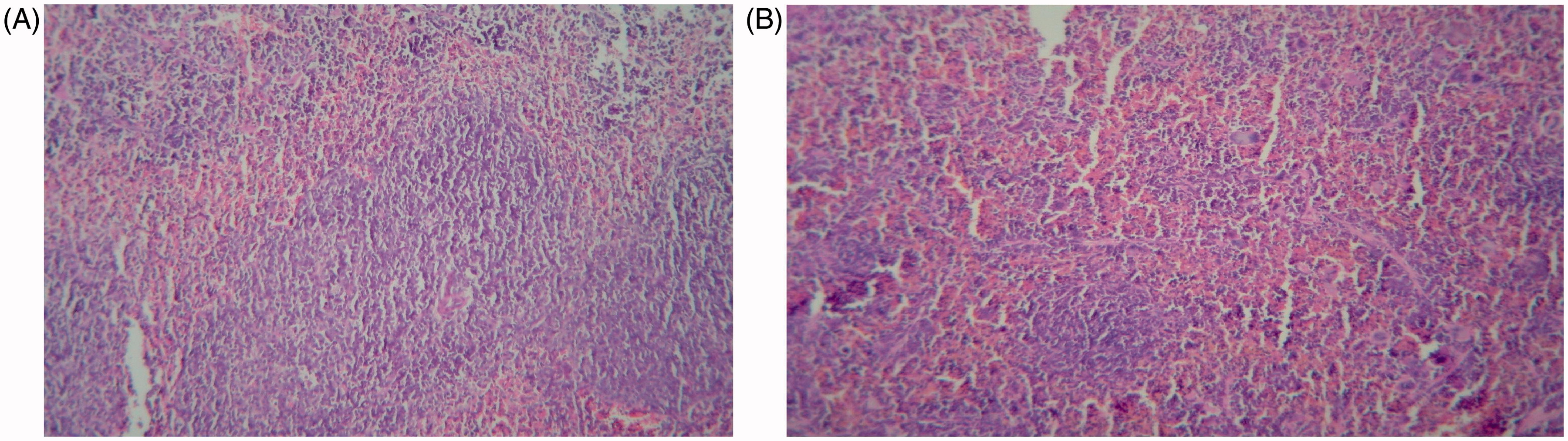
Effects on bone marrow cells histology/cytology
Cellularity, the existence and maturation of hematopoietic cell subtypes, as well as the erythroid:myeloid cell ratios, in each bone marrow specimen isolated was evaluated. Based on light microscopy, no significant pathologic differences were noted among the samples from any of the BBR-treated hosts (data not shown).
Effects on thymus histology
Thymic cortex thickness, relative size of the medulla, the ratio of cortex to medulla and capsular changes were evaluated. The thymic tissues were also examined for the presence of necrosis, apoptosis and any abnormal infiltration of cells. Light microscopic examinations of the thymus samples did not reveal any significant effects from any of the BBR treatments (data not shown).
Effects on humoral immunity
Measures of the antibody titers () illustrated that treatment with 10 mg BBR/kg/day led to reductions in levels of SRBC-specific antibodies in treated hosts (p < 0.001, relative to control host values). There was no significant reduction in antibody titer observed in the group given the lower dose of BBR. In general, levels of anti-SRBC antibody in mice treated with 5 mg BBR/kg/day, 10 mg BBR/kg/day or CYP were reduced to, respectively, 33.3, 81.9 and 50.0% of vehicle control host antibody levels. These reductions seen with the 10 mg BBR/kg/day regimen were even significantly (p < 0.01) lower than those achieved with the CYP.
Figure 3. Effects of subacute berberine (BBR, 14 days) exposure on mouse antibody response. Levels of anti-SRBC produced in serum harvested from mice 24 h after the final treatment (on Day 15) in indicated regimens. Values shown are mean ± SE (n = 6/group). Value significantly different from vehicle control mice (**p < 0.01 or ***p < 0.001); ##Significantly different versus positive control (CYP) (p < 0.01); or ‡‡significantly different as a function of BBR dose (p < 0.01).
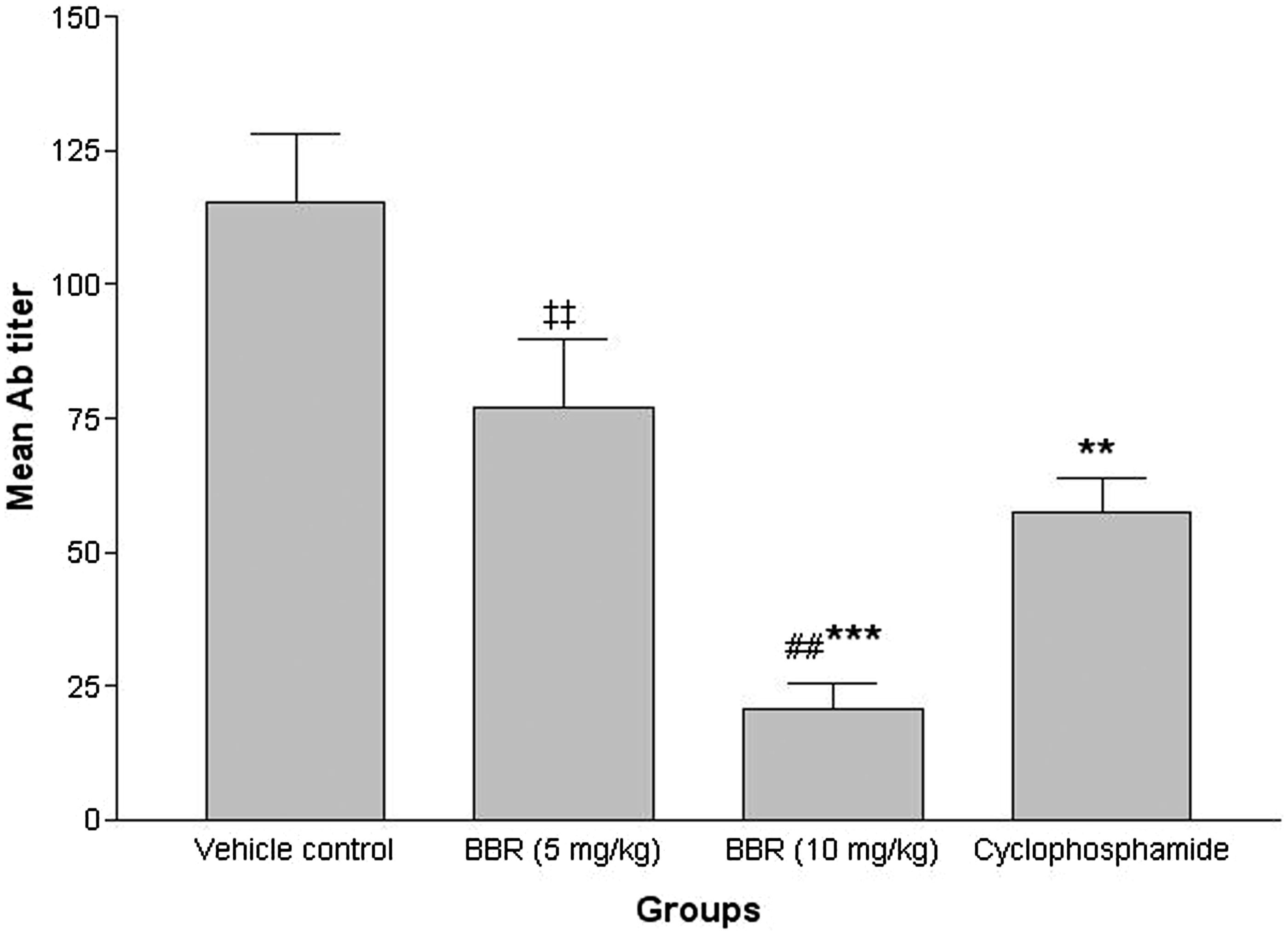
Effects on delayed-type hypersensitivity (DTH) response
As shown in , both doses of BBR contributed to a significant decrease in DTH responses at 48 and 72 h after the footpad challenge (p < 0.05). Only the mice in the 10 mg BBR/kg/day group showed a significant decrease in 24-h DTH response relative to measures for the vehicle controls [or even versus CYP]. In fact, at all three timepoints post-footpad challenge, the 10 mg BBR/kg/day regimen imparted the greatest effect of the three test treatments. While the mean percentage increase in footpad thickness after 24, 48, and 72 h in mice treated with 5 mg BBR/kg/day were decreased to, respectively, 30.0, 49.1 and 37.6% from control values, levels in mice treated with 10 mg BBR/kg/day were reduced in these same periods to 46.6, 60.0 and 65.2% of control values. In mice that received CYP, the reductions (versus control) took longer to reach a significant effect, i.e. only by 72 h after challenge were levels significantly reduced relative to controls (35.1%, p < 0.05). From all the data presented, it is clear that the maximal impact of the 10 mg BBR/kg/day regimen was noted at 72 h, with the reduction in response being significant against all three other treatment group values.
Figure 4. Effects of subacute berberine (BBR, 14 days) exposure on mice DTH response. The percentage increase in paw thickness of mice 24, 48 and 72 h after footpad SRBC challenge in indicated regimens. Values shown are mean ± SE (n = 6/group). Value significantly different from vehicle control mice at *p < 0.05 or **p < 0.01; #significantly different versus positive control (CYP) (p < 0.05); or ‡significantly different as a function of BBR dose (p < 0.05).
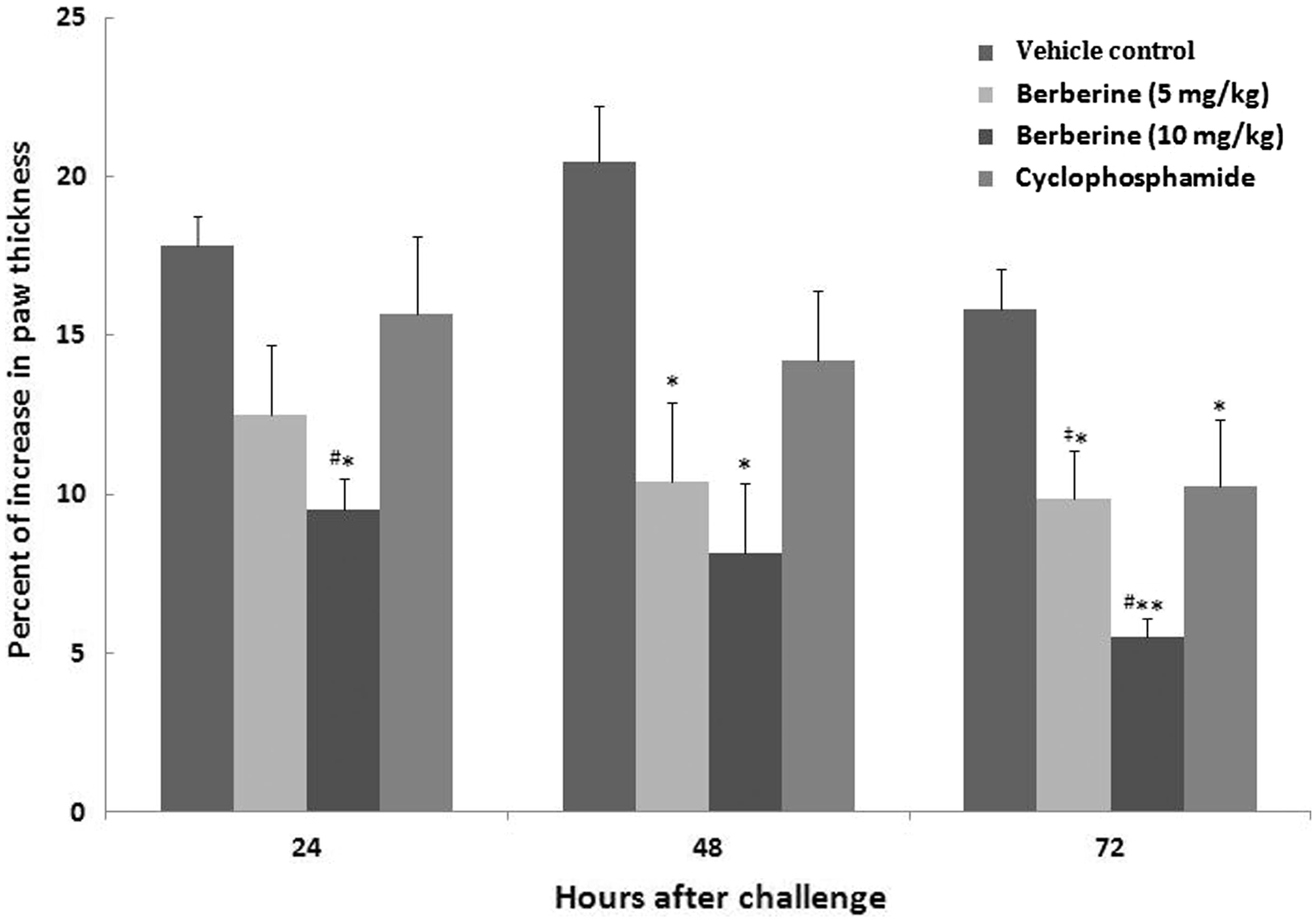
Effects on splenocyte proliferation responses to PHA or LPS
The results clearly demonstrated effects on the inducible proliferative responses of lymphocytes from mice treated with BBR in comparison with values seen with cells from the control hosts (). For cultures not treated with PHA or LPS, spontaneous proliferation was not significantly different as an outcome of host treatments (all had absorbance values of 0.20–0.23). Interestingly, effects on inducible proliferative responses appeared to be stimulant-related. For example, the SI (due to PHA stimulation) values relative to the SI values of vehicle control mouse cells were 20.8, 28.4 and 27.4% lower for cells from mice treated with, respectively, 5 mg BBR/kg/day, 10 mg BBR/kg/day or CYP. In contrast, SI (due to LPS stimulation) values for cells from mice treated with these three regimens were, respectively, reduced to 7.6, 31.3 and 27.1% of vehicle control host (LPS-stimulated) SI levels. In this case, the effect of 5 mg BBR/kg/day resulted in outcomes that were so close to the control values that these too were significantly different from those of the cells from the 10 mg BBR/kg/day or CYP hosts. Again, in contrast, in no instances with PHA were the effects of the various BBR regimens significantly different from those induced by CYP. Clearly, with the PHA, both BBR regimens resulted in significant reductions in proliferative responses; for LPS, the effect was significant only for the 10 mg BBR/kg/day regimen.
Figure 5. Effects of subacute berberine (BBR, 14 days) exposure on ex vivo lymphocyte proliferation. The SI values shown are based upon measures associated with splenocytes harvested from mice 24 h after final treatment (on Day 15) in each regimen. With each treatment group, SI values were calculated from absorbance values for PHA (or LPS)-stimulated cells/absorbance values for unstimulated cells from the same hosts. Values shown are mean ± SE (n = 6/group). Values significantly different from stimulated vehicle control counterpart at *p < 0.05, **p < 0.01 or ***p < 0.001. Value is significantly different versus positive control (CYP) (at #p < 0.05) or as a function of BBR dose (at ‡‡p < 0.01).
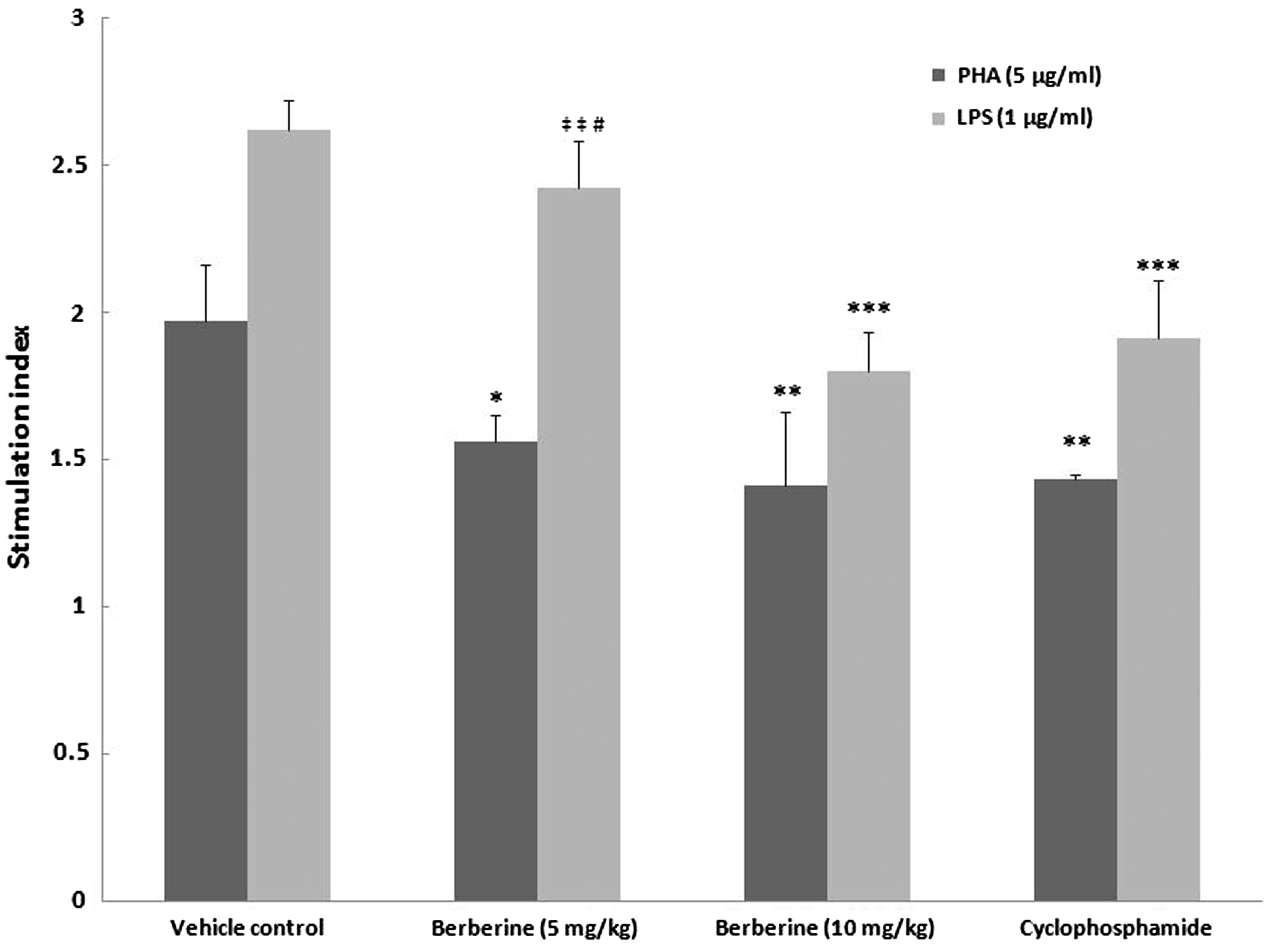
Effects on splenocyte stimulated cytokine production
Host treatment with berberine at 10 mg/kg/day significantly decreased the ex vivo IFNγ production of their splenocytes in response to PHA (p < 0.001) relative to that by control mice cells (). In contrast, IL-4 production by these same cells was markedly increased in comparison to that of control mouse cells (p < 0.01). Similar significant changes were also noted in cells recovered from hosts that were treated with CYP. On the other hand, cytokine production in response to PHA was not significant affected by the 5 mg BBR/kg/day treatment. Values associated with these cells were, accordingly, also very significantly (p < 0.01 or p < 0.001) different from those cells from the 10 mg BBR/kg/day- or CYP-treated hosts.
Table 3. Effects of subacute exposure to berberine (BBR, IP daily, 14 days) on ex vivo cytokine production by mouse spleen cells.
Discussion
Since it has been believed that BBR, as a traditional medicine or dietary supplement, has a very low toxicity (Kheir et al., Citation2010) and because it is very important to have a healthy immune system, this study sought to evaluate potential immunotoxic effects from BBR after a subacute exposure. In the study, potential immunotoxicity of BBR in mice was explored. In the present study, a dose of 10 mg BBR/kg/day caused significant suppression in the immune endpoints examined. Specifically, BBR at a dose of 10 mg/kg/day significantly decreased the weight and cellularity of the spleen. In addition, a significant decrease in PHA- and LPS-induced splenocyte proliferation response (SI values) from the BBR-treated mice suggested a toxic effect on the production/differentiation of T- and B-cells; these effects, in turn, seemed to manifest as suppressed DTH and HA response. BBR at 10 mg/kg/day also caused a significant decrease in blood total leukocytes, lymphocytes and neutrophils. On the other hand, a significant decrease in the absolute numbers and percentages of splenic CD19+ B-cells and in the absolute numbers of splenic CD4+ and CD8+ T-cells in the 10 mg BBR/kg mice suggested that there was a toxic effect on the production of lymphocytes in primary lymphatic organs and/or on the deposition of blood lymphocytes in spleen. In contrast, the data here showed that BBR at a level of 5 mg/kg/day caused toxic effects on some of the assessed endpoints, such as DTH responses (after 48 and 72 h) and lymphoproliferation assay, but not much else.
As noted earlier, some of the immunotoxic properties of BBR observed here might be due to a direct effect on the activation/differentiation of lymphocytes. T-cells organize the acquired immune response and are vital for defense and immunological memory. During the time naive T-cells are stimulated by foreign antigen, they endure an activation program in which they undergo rapid proliferation and make them ready to differentiate into effector subtypes (Sena Laura et al., Citation2013). Activation requires interactions between the T-cell receptor (TCR) and the CD28 co-stimulatory molecule. The TCR and CD28 then trigger signaling cascades that provoke activation of transcription factors to promote expression of proteins that induce T-cell activation, such as interleukin (IL)-2 (Smith-Garvin et al., Citation2009).
There are studies demonstrating that reactive oxygen species (ROS) can act as signaling intermediates in the aforementioned processes (Byun et al., Citation2008; Schieke et al., Citation2008) and that ROS are formed within 15 min of TCR cross-linking (Devadas et al., Citation2002). Another study reported small amounts of ROS were pivotal in inducing transcription of NF-κB and expression of genes for several critical cytokines and receptors required for T-cell proliferation. This key role for ROS in cell proliferation was borne out in studies showing that, if mice were exposed to anti-oxidants, subsequent ex vivo T-cell proliferation was diminished (Laniewski & Grayson, Citation2004; Piganelli et al., Citation2002). Therefore, because cell redox status plays an important role in T-cell functions (Los et al., Citation1995), the BBR at higher doses may have acted as a potent anti-oxidant (in role as a polyphenolic) to remove any ROS that could partake in the activation/proliferation of T-cells, ultimately resulting in overall immunosuppression. The anti-oxidant properties of BBR in situ were recently illustrated by Li et al. (Citation2014) wherein it was shown that BBR administration to diabetic mice led to changes in levels of key oxidative stress markers, such as malondialdehyde (MDA) and glutathione (GSH), and in activities of glutathione peroxidase (GSHPX) and superoxide dismutase (SOD). Such findings were in keeping with earlier studies of the potent anti-inflammatory effects of BBR (Choi et al., Citation2006; Jeong et al., Citation2009; Lou et al., Citation2011).
In addition, in the present study, a significant reduction (in comparison with values from cells from vehicle control mice) in IFNγ and an increase in IL-4 formation in cultures of splenocytes was observed with cells from mice treated with the higher dose of BBR, suggesting a shift in the host to a more T-helper (TH)-2 cell-based response. This purported shift in TH cell profiles here would be in agreement with earlier findings in in vitro studies of mouse splenocytes that were cultured in the presence of BBR; production of some TH1 cytokines like tumor necrosis factor (TNF)-α and IL-1β, as well as the expression of both cyclo-oxygenase-2 (COX-2) and inducible nitric oxide synthase (iNOS), were reduced in these treated cells (Lin & Lin, Citation2011). Reductions in iNOS expression are relatable to changes in the TH1:TH2 balance (i.e. TH1 cells induce iNOS, whereas TH2 cells induce arginase; Munder et al., Citation1999). Similarly, increases in TH2 cell levels (or associated products) are known to cause reductions in COX-2 expression by granulocytes (Endo et al., Citation1996, Citation1998). Nevertheless, while the expansion of IFNγ-producing TH1 CD4+ cells would enhance cell-mediated immunity, the expansion of IL-4-producing TH2 CD4+ T-cells would promote generation of regulatory T-cells and, thus, weaken immune system reactions (Farsam et al., Citation2011). This shift could be a major factor in the overall observed immunosuppressive effects of berberine; further study is underway to confirm this.
Lastly, it is known that ROS are essential mediators in antigen presentation by phagocytic cells (Maemura et al., Citation2005; Riahi et al., Citation2011). Antigen-presenting cells (APC) produce ROS during processing/presentation of antigens and so inhibition of ROS generation by anti-oxidants (or a simple reduction in levels of the products themselves) could result in depressed antigen-specific APC-dependent T-cell responses (Matsue et al., Citation2003). In an in vitro study by Sarna et al. (Citation2010), BBR could decrease ROS formation by macrophages, in part, by reducing expression of NADPH oxidase, a main source of ROS production in phagocytic cells. In keeping with the observed comments on effects of berberine on ROS levels, we surmise that the observed suppression of the DTH and HA responses in the BBR-treated hosts here might have been due to a failure of presentation and processing of SRBC antigens by antigen-presenting cells, secondary to reductions in ROS availability.
In conclusion, the present study showed that BBR at a high dose (i.e. 10 mg/kg/day for 14 days) imparted an inhibitory effect on the mouse immune system. At a lower dose (i.e. 5 mg/kg/day), BBR only seemed to affect DTH responses and mitogen-induced splenocyte/lymphocyte proliferation. Further studies, including far more doses, are required to better assess the immunotoxic effects of this natural product. In addition, mechanistic studies are needed to clarify precisely how this material is acting to impart many of the immunotoxic effects demonstrated here. Further studies should be performed to measure levels of ROS formed by mouse spleen T- or B-lymphocytes after berberine administration and changes in the activation of pathways in which ROS act as a signaling mediator in these cells. Further, since a failure in antigen processing/presentation by phagocytic cells could be a major factor in the current and earlier-observed immunosuppressive effects, it is also recommended that ROS formation by antigen-exposed phagocytic cells after berberine treatment also be evaluated in these future studies.
Declaration of interest
The authors report no conflicts of interest. The authors alone are responsible for the content and writing of this paper.
Acknowledgements
The authors are thankful to the Vice Chancellor of Research, Mashhad University of Medical Sciences for their financial support.
Notes
1The flow analysis was performed in a certified central collaborative laboratory that performs a large amount of similar analyses for all the investigators at the Bu-Ali Research Institute. This laboratory collected and analyzed all the dot-plots generated from the current study's samples provided and then forwarded to us all the data as numerical outputs for statistical analyses and presentation here (Table 2). Unfortunately, the original representative dot-plots of the spleen cell analyses are not currently available for presentation (due to issues of memory capacity of the instrument used).
References
- Bendich, A. 1993. Physiological role of anti-oxidants in the immune system. J. Dairy Sci. 76:2789–2794
- Byun, H. O., Kim, H. Y., Lim, J. J., et al. 2008. Mitochondrial dysfunction by complex II inhibition delays overall cell cycle progression via reactive oxygen species production. J. Cell Biochem. 104:1747–1759
- Choi, B. H., Ahn, I. S., Kim, Y. H., et al. 2006. Berberine reduces the expression of adipogenic enzymes and inflammatory molecules of 3T3-L1 adipocyte. Exp. Mol. Med. 38:599–605
- Devadas, S., Zaritskaya, L., Rhee, S. G., et al. 2002. Discrete generation of superoxide and hydrogen peroxide by T-cell receptor stimulation: Selective regulation of mitogen-activated protein kinase activation and fas ligand expression. J. Exp. Med. 195:59–70
- Endo, T., Ogushi, F., and Sone, S. 1996. LPS-dependent COX-2 induction in human monocytes is down-regulated by IL-13, but not by IFNγ. J. Immunol. 156:2240–2246
- Endo, T., Ogushi, F., Kawano, T., and Sone, S. 1998. Comparison of the regulations by TH2-type cytokines of the arachidonic acid metabolic pathways in human alveolar macrophages and monocytes. Am. J. Respir. Cell Mol. Biol. 19:300–307
- Fararjeh, M., Mohammad, M. K., Bustanji, Y., et al. 2008. Evaluation of immunosuppression induced by metronidazole in Balb/c mice and human peripheral blood lymphocytes. Intl. Immunopharmacol. 8:341–350
- Farsam, V., Hassan, Z. M., Zavaran-Hosseini, A., et al. 2011. Antitumor and immunomodulatory properties of artemether and its ability to reduce CD4+ CD25+ FoxP3+ Treg cells in vivo. Int. Immunopharmacol. 11:1802–1808
- Hasani-Ranjbar, S., Larijani, B., and Abdollahi, M. 2009. A systematic review of the potential herbal sources of future drugs effective in oxidant-related diseases. Inflamm. Allergy Drug Targets 8:2–10
- Ivanovska, N., and Philipov, S. 1996. Study on the anti-inflammatory action of Berberis vulgaris root extract, alkaloid fractions, and pure alkaloids. Int. J. Immunopharmacol. 18:553–561
- Ivanovska, N., Philipov, S., and Hristova, M. 1999. Influence of berberine on T-cell-mediated immunity. Immunopharmacol. Immunotoxicol. 21:771–786
- Jeong, H.W., Hsu, K. C., Lee, J. W., et al. 2009. Berberine suppresses pro-inflammatory responses through AMPK activation in macrophages. Am. J. Physiol. 296:E955–964
- Kheir, M. M., Wang, Y., Hua, L., et al. 2010. Acute toxicity of berberine and its correlation with the blood concentration in mice. Food Chem. Toxicol. 48:1105–1110
- Laniewski, N. G., and Grayson, J. M. 2004. Anti-oxidant treatment reduces expansion and contraction of antigen-specific CD8+ T-cells during primary but not secondary viral infection. J. Virol. 78:11246–11257
- Letasiova, S., Jantova, S., Muckova, M., and Theiszova, M. 2005. Anti-proliferative activity of berberine in vitro and in vivo. Biomed. Pap. Med. Fac. Univ. Palacky Olomouc Czech Repub. 149:461–463
- Li, Z., Geng, Y. N., Jiang, J. D., and Kong, W. J. 2014. Anti-oxidant and anti-inflammatory activities of berberine in the treatment of diabetes mellitus. Evid.-Based Complement Alt. Med. 2014:289264
- Lin, W. C., and Lin, J. Y. 2011. Five bitter compounds display different anti-inflammatory effects through modulating cytokine secretion using mouse primary splenocytes in vitro. J. Agric. Food Chem. 59:184–92
- Los, M., Droge, W., Stricker, K., et al. 1995. Hydrogen peroxide as potent activator of T-lymphocyte functions. Eur. J. Immunol. 25:159–165
- Lou, T., Zhang, Z., Xi, Z., et al. 2011. Berberine inhibits inflammatory response and ameliorates insulin resistance in hepatocytes. Inflammation 34:659–667
- Luster, M. I., Dean, J. H., and Germolec, D. R. 2003. Consensus workshop on methods to evaluate developmental immunotoxicity. Environ. Health Perspect. 111:579–583
- Maemura, K., Zheng, Q., Wada, T., et al. 2005. Reactive oxygen species are essential mediators in antigen presentation by Kupffer cells. Immunol. Cell. Biol. 83:336–343
- Marinova, E. K., Nikolova, D. B., Popova, D. N., et al. 2000. Suppression of experimental autoimmune tubulointerstitial nephritis in BALB/c mice by berberine. Immunopharmacology 48:9–16
- Matsue, H., Edelbaum, D., Shalhevet, D., et al. 2003. Generation and function of reactive oxygen species in dendritic cells during antigen presentation. J. Immunol. 171:3010–3018
- Munder, M., Eichmann, K., Morán, J. M., et al. 1999. TH1/TH2-regulated expression of arginase isoforms in murine macrophages and dendritic cells. J. Immunol. 163:3771–3777
- Noori, S., Taghikhani, M., Hassan, Z. M., et al. 2010. Tehranolide molecule modulates the immune response, reduce regulatory T-cell and inhibits tumor growth in vivo. Mol. Immunol. 47:1579–1584
- Piganelli, J. D., Flores, S. C., Cruz, C., et al. 2002. A metalloporphyrin-based superoxide dismutase mimic inhibits adoptive transfer of autoimmune diabetes by a diabetogenic T-cell clone. Diabetes 51:347–355
- Rahnama, M., Mahmoudi, M., Zamani Taghizadeh Rabe, S., et al. 2014. Evaluation of anti-cancer and immunomodulatory effects of carnosol in a Balb/c WEHI-164 fibrosarcoma model. J. Immunotoxicol 12:231–238
- Riahi, B., Rafatpanah, H., Mahmoudi, M., et al. 2010. Immunotoxicity of paraquat after subacute exposure to mice. Food Chem. Toxicol. 48:1627–1631
- Riahi, B., Rafatpanah, H., Mahmoudi, M., et al. 2011. Evaluation of suppressive effects of paraquat on innate immunity in Balb/c mice. J. Immunotoxicol. 8:39–45
- Sarna, L. K., Wu, N., Hwang, S. Y., et al. 2010. Berberine inhibits NADPH oxidase-mediated superoxide anion production in macrophages. Can. Physiol. Pharmacol. 88:369–378
- Schieke, S. M., McCoy J. P., Jr and Finkel, T. 2008. Coordination of mitochondrial bioenergetics with G1 phase cell cycle progression. Cell Cycle 7:1782–1787
- Sena Laura, A., Li, S., Jairaman, A., et al. 2013. Mitochondria are required for antigen-specific T-cell activation through reactive oxygen species signaling. Immunity 38:225–236
- Smith-Garvin, J. E., Koretzky, G. A., and Jordan, M. S. 2009. T-Cell activation. Annu. Rev. Immunol. 27:591–619
- Tsang, C. M., Cheung, Y. C., Lui, V. W., et al. 2013. Berberine suppresses tumorigenicity and growth of nasopharyngeal carcinoma cells by inhibiting STAT3 activation induced by tumor associated fibroblasts. BMC Cancer 13:619
- Xu, L., Liu, Y., and He, X. 2005. Inhibitory effects of berberine on the activation and cell cycle progression of human peripheral lymphocytes. Cell. Mol. Immunol. 2:295–300