Abstract
Background and purpose The most frequently used bones for mechanical testing of orthopedic and trauma devices are fresh frozen cadaveric bones, embalmed cadaveric bones, and artificial composite bones. Even today, the comparability of these different bone types has not been established.
Methods We tested fresh frozen and embalmed cadaveric femora that were similar concerning age, sex, bone mineral density, and stiffness. Artificial composite femora were used as a reference group. Testing parameters were pullout forces of cortex and cancellous screws, maximum load until failure, and type of fracture generated.
Results Stiffness and type of fracture generated (Pauwels III) were similar for all 3 bone types (fresh frozen: 969 N/mm, 95% confidence interval (CI): 897–1,039; embalmed: 999 N/mm, CI: 875–1,121; composite: 946 N/mm, CI: 852–1,040). Furthermore, no significant differences were found between fresh frozen and embalmed femora concerning pullout forces of cancellous screws (fresh frozen: 654 N, CI: 471–836; embalmed: 595 N, CI: 365–823) and cortex screws (fresh frozen: 1,152 N, CI: 894–1,408; embalmed: 1,461 N, CI: 880–2,042), and axial load until failure (fresh frozen: 3,427 N, CI: 2,564–4290; embalmed: 3,603 N, CI: 2,898–4,306). The reference group showed statistically significantly different results for pullout forces of cancellous screws (2,344 N, CI: 2,068–2,620) and cortex screws (5,536 N, CI: 5,203–5,867) and for the axial load until failure (> 7,952 N).
Interpretation Embalmed femur bones and fresh frozen bones had similar characteristics by mechanical testing. Thus, we suggest that embalmed human cadaveric bone is a good and safe option for mechanical testing of orthopedic and trauma devices.
Human cadaveric specimens are widely used in studies analyzing the mechanical properties and efficacy of orthopedic and trauadevices (Park et al. Citation2010). Storage of fresh human cadaveric bones at a temperature of –20°C is a commonly used and accepted method for preservation of bone. Many studies have shown that freezing does not alter the mechanical properties of human bone (Pelker et al. Citation1983, Goh et al. Citation1989, Linde and Sorensen Citation1993, Matter et al. Citation2001, van Haaren et al. Citation2008), although some changes have been shown compared to fresh bone (Sonstegard and Matthews Citation1977, Pelker et al. Citation1983).
In Europe, there is some difficulty in obtaining a sufficient number of fresh frozen bones. Furthermore, they are expensive and need excessive storage space—and there is also the risk of transmitting pathogens to investigators (Sterling et al. Citation2000, van Haaren et al. Citation2008).
Human cadaveric specimens are often obtained from anatomical institutes. The cadavers have usually been embalmed with a formaldehyde solution for at least 1 year before usage. Some studies have investigated the effects of embalming cadaveric bones regarding their mechanical properties (McElhaney et al. Citation1964, Pelker et al. Citation1983, Roe et al. Citation1988, Goh et al. Citation1989, Linde and Sorensen Citation1993, Currey et al. Citation1995).
Most of the above studies used animal bones for mechanical testing, and had a maximum storage period of ≤ 100 days (McElhaney et al. Citation1964, Pelker et al. Citation1983, Roe et al. Citation1988, Goh et al. Citation1989, Currey et al. Citation1995). There have been few studies on long-term effects (van Haaren et al. Citation2008), and most of the studies using human bones have concentrated on small bone segments (Linde and Sorensen Citation1993, Currey et al. Citation1995, Burkhart et al. Citation2010).
Another bone type that is commonly used is the artificial composite bone (Cristofolini et al. Citation1996, Zdero et al. Citation2008). Concerning the handling and mechanical properties, cadaveric specimens simulate reality best (Burkhart et al. Citation2010). In comparison, composite bones differ in some of their mechanical characteristics (Cristofolini et al. Citation1996).
We compared fresh frozen human femora and long-period embalmed femora concerning stiffness, maximum axial load until fracture, type of fracture generated, and pullout strength of cortical and cancellous bone screws. Artificial femora were used as a non-osteoporotic reference group.
Materials and methods
Bone types
6 embalmed human cadaveric femora were obtained from the Institute of Anatomy and Cell Biology of Philipps University, Marburg. They were taken from human cadavers that had been embalmed with a solution consisting of 96% ethanol and < 2% fomaldehyde. During perfusion, about 15 L of the solution was passed through the femoral artery. Human cadavers were stored there for a period of at least a year before usage. The bones were stored in towels soaked in the above solution, at a temperature of 6°C. 6 human fresh frozen cadaveric femora were obtained commercially from the Anatomy Gifts Registry (Glen Burnie, MD). The bones were sealed in biohazard bags and refrigerated at –20°C. Both the embalmed bones and the fresh frozen bones were whole-femur bone specimens with the soft tissues stripped off. Before use, all bones were placed at room temperature for 24 h. Embalmed bones were kept wet with towels soaked in 96% ethanol and < 2% formaldehyde, and fresh frozen bones were kept wet with saline-soaked towels during testing. A radiograph of all specimens was taken prior to testing, to exclude pathologies. As an artificial bone model, 6 large composite femora were used (Sawbones Fourth Generation, model no. 3402, left side; Pacific Research Laboratories Inc., Vashon, WA).
Bone mineral density
We used peripheral quantitative computed tomography (pQCT) measurements to compare the bone mineral density (BMD) of cadaveric models. For pQCT measurements, a Stratec XCT 2000 system was used (Stratec Medizintechnik GmbH, Pforzheim, Germany). The point of measurement for cortical BMD was set 8 cm below the greater trochanter. Cancellous BMD measurements were performed at the distal metaphysis.
All bone groups were cut in 2 pieces to a length of 30 cm, measured from the top of the femoral head to obtain 2 separate bone specimens for axial and pullout testing. The femoral shaft of the proximal part was placed in special containers filled with Technovit 3040 (Heraeus, Wehrheim, Germany) for further fixation.
Mechanical testing materials
Synthes stainless steel self-tapping cortical bone and cancellous bone screws were used (Synthes, Paoli, PA). The cortex screws had a 4.5-mm thread diameter, an 8-mm head, 1.25-mm pitch, and a core diameter of 3.1 mm. The cancellous bone screws had a 6.5-mm thread diameter, a full thread, an 8 mm head, 1.75-mm pitch, and a core diameter of 3.0 mm. A Synthes 3.2-mm drill bit was used for insertion of all screws. For axial drilling, a fixed-angle drilling machine was used. Mechanical testing was performed with an Instron 5566 materials testing system (Instron, Canton, MA) using axial pullout and loading forces.
Mechanical testing
Pullout testing. 18 Synthes self-tapping cortical screws were inserted into the distal diaphyseal region, 3 cm proximal to the metaphysis. The screws were inserted bicortically to depths of 2 mm past the far cortex. The 18 Synthes self-tapping cancellous bone screws were inserted at the crossing point of the maximum distance between the femoral condyles and the extension of the anatomical axis of the bone. All drilling holes were proximal to the notch region. The pilot holes were also prepared with a 3.2-mm Synthes drill bit using the same drilling machine. The drilling depth was 60 mm. All screws were inserted monocortically. Screw insertion was continuous without any intermission. A depth gauge, sensitive down to 0.05 mm, was used to ensure the depth of insertion. All screws were inserted by a single investigator (TT). Pullout testing was performed using an Instron 5566 materials testing system.
Mechanical testing was performed with a method consistent with the recommendations of the American Society for Testing and Materials (ASTM) (ASTM Citation1996). The screws were pulled out with a fixture that slipped over the screw head, which was attached to the load frame of the Instron 5566. An inserted load train employed a universal joint that was essential in ensuring that only axial pullout forces were applied to the screws () (Battula et al. Citation2008, Schoenfeld et al. Citation2008). Testing was performed under displacement control at a rate of 1 mm/s. Load and displacement data were collected at 30 Hz.
Figure 1. Material testing. A. Pauwels III fracture of a human cadaveric specimen after axial loading. The bone was fixed in a metal cylinder. Axial compression was performed via an artificial acetabulum. B. Osteosynthesis failure after pullout testing of a cortex screw.
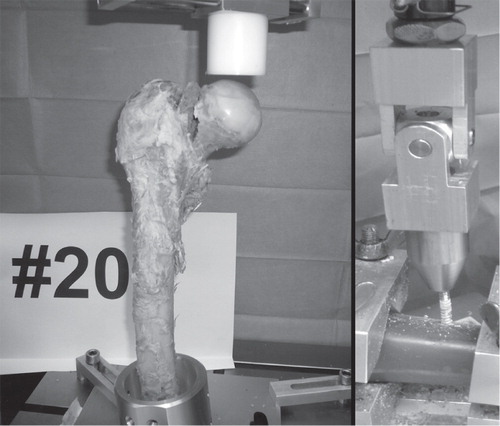
Stiffness testing. The parts of the proximal femur that had been embedded in Technovit were statically fixed in a metal cylinder with an angle of 7° to ensure physiological conditions. The femoral head was loaded through a congruently shaped cast acetabulum with spherical excavation. This artificial acetabulum was freely movable in the horizontal plane, to allow movements of the femoral head during loading (). Baseline intact stiffness was calculated (in N/mm) for each specimen. For this purpose, the femora were tested with 20 compressive cyclic loads at 0.25 Hz, moving between 200 and 1,000 N. Thus, the specimens were kept in the elastic part of the load-displacement curve, which ensured that they did not sustain permanent damage. The stiffness (in N/mm) was extrapolated from the slope of the curves of cycles 2–20. To minimize imprecision, the data from the first cycle were not used. This test setup has already been approved in other studies (Muller et al. Citation2011).
Axial load testing until failure. The setup for axial load testing until bone failure was equivalent to the setup for stiffness testing, but no cyclic loads were used. Testing was conducted under a continuously increasing load of 50 N/s. The testing was ended if a maximum load of 8,000 N had been reached or if a fracture of the bone occurred. All fractures were documented by video and still photography (). The fractures were classified using the Pauwels classification for femoral neck fractures (Pauwels Citation1935).
Statistics
Data are presented as mean (SD). They were analyzed with one-way analysis of variance (ANOVA) and with Tukey’s comparison post-test or a t-test for continuous variables when normal distribution was assumed. We used the Kruskal-Wallis test and Dunn’s comparison post-test for continuous data for analysis of datasets that included “off-scale” data that were not normally distributed. 95% confidence intervals (CIs) were determined. We used GraphPad Prism 5 software for Windows (GraphPad Software Inc., La Jolla, CA).
Results
Specimen data
Both cadaveric bone groups had a sex distribution of 5 females to 1 male specimen. 4 right human femora and 2 left human femora were used in each group; all of the composite bones were left femora. Mean age in the embalmed group was 85 (74–96) years and in the fresh frozen group it was 86 (73–95) years. There was no significant difference in age distribution (p = 0.4). Furthermore, there was no significant difference in the cortical BMD of fresh frozen bone (1,177 mg/cm3, CI: 1,135–1,317) and that of embalmed bone (1,176 mg/cm3, CI: 1,128–1,222) bone, or in the values of cancellous bone mineral density for both bone models (fresh frozen: 184 mg/cm3, CI: 165–200; embalmed: 170 mg/cm3, CI: 156–184). Values for total length, diameter at half of the bone length, and the caput-collum-diaphyseal (CCD) angle are given in .
Table 1. Dimensions of the different bone models. Values are mean (CI)
Mechanical testing
No statistically significant differences between the 3 test groups were found concerning stiffness. Fresh frozen bones and embalmed bones required significantly lower pullout forces for failure of cortex and cancellous screws than the composite group. Furthermore, lower axial loads could be used in these 2 groups for a fracture of the bone to occur, compared to composite bones. There was no statistically significant difference when comparing fresh frozen and embalmed bones with regard to pullout and axial loading ().
Table 2. Comparison of fresh frozen bone (FFB), embalmed bone (EB), and composite bone (CB) concerning stiffness, pullout forces for cortex and cancellous screws, and axial load until failure. Figures are mean (CI) and all p-values are given
shows a typical profile from pullout testing of a cortex screw in a plot of the force-displacement curve.
All 3 bone types showed Pauwels III fracture types when a fracture occurred. In the fresh frozen bone group and the embalmed bone group, a fracture could be generated for each specimen. However, only 1 of the composite bones had fractured before the maximum load of 8 kN was reached.
Discussion
To simulate physiological conditions in vivo, fresh bone—including the surrounding tissue—would be the best testing material. For mechnical testing, high numbers of bones are needed and testing often has to be performed over several days, which requires bone preservation. Changes in mechnical properties after preservation have been discussed in the literature, and they are the subject of some controversy. Sonstegard and Matthews (1997) found a decrease of 10% in stiffness after freezing of trabecular bones, and they suggested that the reason might be the trabecular damage caused by freezing-expansion of interstitial fluids. Pelker et al. (Citation1983) found a slight increase in stiffness after freezing. Panjabi et al. (Citation1985) and Linde and Sorensen (Citation1993) found similar mechanical properties after freezing bones for different periods of time. Before bone preservation, early post mortem changes lead to alteration in bone stiffness—especially in the first 24 hours (Linde and Sorensen Citation1993). These changes must be considered when interpreting in vitro results.
Besides fresh frozen bones, embalmed human cadaveric bones are common in mechanical studies. There are also varied and contradictory opinions in the literature as to whether embalmed bone reflects realistic physiological conditions. In experiments with cat bones, Goh et al. (Citation1989) found that formalin fixation caused a reduction in energy absorption and increased the brittleness of the bone. Currey et al. (Citation1995) reported a decrease in impact strength of bovine bone after fixation with formaldehyde. Wingerter et al. (Citation2006) showed similar results for fixation of rat bone with a 10% formalin solution. In contrast, van Haaren et al. (Citation2008) recently showed that fixation with formaldehyde had no effect on the mechanical properties of goat bones after a fixation period of up to 1 year, and they suggested that embalmed bones can be safely used for mechanical testing purposes.
There have been few studies on human bone tissue. Ohman et al. (Citation2008) analyzed small specimens extracted from 2 human femoral diaphyses. After a 4 week of fixation using a 4% formalin solution, they found that there were no significant differences in yield stress, ultimate stress, and hardness compared to the fresh bone. In addition, they found an increase in yield and ultimate stress after a fixation period of 8 weeks. Burkhart et al. (Citation2010) recently published similar results after comparing formalin-fixed and fresh human bones. They analyzed human specimens taken from the subtrochanteric region of the femur and reported an increase in stiffness values as well as axial and torsional loads after 6 weeks of fixation in 4% formalin solution. Another study found no change in stiffness after a storage time of 100 days after ethanol fixation (Linde and Sorensen Citation1993).
Zech et al. (2006) compared embalmed, fresh, and artificial human calcanei. Artificial calcanei had mechanical characteristics that were different from those of embalmed and fresh frozen cadaveric specimens, which does not support the use of artificial calcanei for mechanical implant testing. Fresh frozen and embalmed specimens appeared to be equally adequate for mechanical testing. Furthermore, Zech suggested that the use of paired specimens is not necessary because of the low variation in mechanical strength in unpaired cadaveric specimens. Cristofolini et al. (Citation1996) found that composite bones differ from cadaveric bones in some of their mechanical characteristics. Zdero et al. (Citation2008) compared artificial and human bones and they could not find any significant difference regarding screw pullout force and shear stress.
Our testing setup was chosen for comparison of 2 different and frequently used bone types: embalmed and fresh frozen bones. To simulate realistic conditions, we used the whole proximal part of the bone including the shaft for testing of axial loading forces in the physiological axis of the bone. The different unpaired human cadaveric femur bones were harvested from different donors; thus, we could not compare the different fixation techniques on a paired bone model. Zech et al. (Citation2006) found a low degree of variation in mechanical strength in similar unpaired cadaveric bone specimens. For a better comparison of the specimens, we chose a similar group of donors concerning sex and age distribution. All donors had had osteoporosis documented in their clinical history, but T-scores were not provided. The cortical BMD of embalmed and fresh frozen bones was therefore documented using pQCT measurements prior to mechanical testing.
The cortical and cancellous BMD of the different cadaveric specimens were similar, as were the stiffness and length measurements of the samples.
When comparing embalmed cadaveric bones to fresh frozen cadaveric bones, we found similar stiffness, axial load, and pullout force of cancellous and cortical bone screws. Slightly higher pullout forces for cancellous screws and lower pullout forces for cortical screws in fresh frozen bones can be explained by interindividual differences between the specimens used. There may be an effect of the procedure of embalming on the bones, especially concerning rigidity and brittleness. Van Haaren et al. (2008) and Zech et al. (Citation2006) did not find any significant differences between embalmed and fresh frozen animal and human bones regarding their mechanical properties. Goh et al. (Citation1989) found an increase in brittleness and Currey et al. (Citation1995) found a reduction in impact strength, while Burkhart et al. (2008) and Ohman et al. (Citation2008) found that cortical bones are more rigid after an embalming procedure. We found similar mechanics in embalmed and fresh frozen human cadaveric bones. They can therefore be recommended for biomechanical testing purposes.
As expected, the reference group of composite bones had higher values for all mechanical parameters tested although they showed the same Pauwels type of fracture (type III). These differences can be explained by the fact that these composite “bone analogs” are designed and optimized to be a model for “average” and non-osteoporotic human femora regarding mass density and stiffness distribution. This was why composite bones were used as a reference group in this study.
All the authors were fully involved in the study and in preparation of the manuscript. They all revised and approved the final manuscript. Creation of hypothesis and suggestion of test setup: TT, TM, and RPZ. Development of test setup: TT and TM. QCT measurements: TT and RPK. Anatomical supervision and preparation: TT, TM, EW, and SH. Fracture classification, supervision: TT, RPZ, and SH. Statistical analysis and writing of the manuscript: TT.
No external funding was used.
No competing interests declared.
- ASTM.Designation: F1691-96. Standard test method for determining axial pullout strength of medical bone screws. Book of ASTM Standards 1996;Philadelphia, PA: American Society for Testing and Materials.
- Battula S, Schoenfeld AJ, Sahai V, Vrabec GA, Tank J, Njus GO. The effect of pilot hole size on the insertion torque and pullout strength of self-tapping cortical bone screws in osteoporotic bone. J Trauma 2008; 64 (4): 990-5.
- Burkhart KJ, Nowak TE, Blum J, Kuhn S, Welker M, Sternstein W, Mueller LP, Rommens PM. Influence of formalin fixation on the biomechanical properties of human diaphyseal bone. Biomed Tech (Berl) 2010; 55 (6): 361-5.
- Cristofolini L, Viceconti M, Cappello A, Toni A. Mechanical validation of whole bone composite femur models. J Biomech 1996; 29 (4): 525-35.
- Currey JD, Brear K, Zioupos P, Reilly GC. Effect of formaldehyde fixation on some mechanical properties of bovine bone. Biomaterials 1995; 16 (16): 1267-71.
- Goh JC, Ang EJ, Bose K. Effect of preservation medium on the mechanical properties of cat bones. Acta Orthop Scand 1989; 60 (4): 465-7.
- Linde F, Sorensen HC. The effect of different storage methods on the mechanical properties of trabecular bone. J Biomech 1993; 26 (10): 1249-52.
- Matter HP, Garrel TV, Bilderbeek U, Mittelmeier W. Biomechanical examinations of cancellous bone concerning the influence of duration and temperature of cryopreservation. J Biomed Mater Res 2001; 55 (1):0-4.
- McElhaney J, Fogle J, Byars E, Weaver G. Effect of Embalming on the Mechanical Properties of Beef Bone. J Appl Physiol 1964; 19: 1234-6.
- Muller T, Topp T, Kuhne CA, Gebhart G, Ruchholtz S, Zettl R. The benefit of wire cerclage stabilisation of the medial hinge in intramedullary nailing for the treatment of subtrochanteric femoral fractures: a biomechanical study. Int Orthop 2011; 35 (8): 1237-43.
- Ohman C, Dall’Ara E, Baleani M, Van Sint Jan S, Viceconti M. The effects of embalming using a 4% formalin solution on the compressive mechanical properties of human cortical bone. Clin Biomech (Bristol. Avon) 2008; 23 (10): 1294-8.
- Panjabi MM, Krag M, Summers D, Videman T. Biomechanical time-tolerance of fresh cadaveric human spine specimens. J Orthop Res 1985; 3 (3): 292-300.
- Park K, Kim K, Choi YS. Comparison of mechanical rigidity between plate augmentation leaving the nail in situ and interlocking nail using cadaveric fracture model of the femur. Int Orthop 2010; 35 (4): 581-5.
- Pauwels F. Der Schenkelhalsbruch. Ein mechanisches Problem. Beilagheft Z Orthop Chir 1935;Stuttgart:Enke 63.
- Pelker RR, Friedlaender GE, Markham TC. Biomechanical properties of bone allografts. Clin Orthop 1983; (174): 54-7.
- Roe SC, Pijanowski GJ, Johnson AL. Biomechanical properties of canine cortical bone allografts: effects of preparation and storage. Am J Vet Res 1988; 49 (6): 873-7.
- Schoenfeld AJ, Battula S, Sahai V, Vrabec GA, Corman S, Burton L, Njus GO. Pullout strength and load to failure properties of self-tapping cortical screws in synthetic and cadaveric environments representative of healthy and osteoporotic bone. J Trauma 2008; 64 (5): 1302-7.
- Sonstegard DA, Matthews B. Mechanical property dependence on storage technique and locale of knee joint trabecular bone. Trans orthop Res Soc 1977; 2:283.
- Sterling TR, Pope DS, Bishai WR, Harrington S, Gershon RR, Chaisson RE. Transmission of Mycobacterium tuberculosis from a cadaver to an embalmer. N Engl J Med 2000; 342 (4): 246-8.
- van Haaren EH, van der Zwaard BC, van der Veen AJ, Heyligers IC, Wuisman PI, Smit TH. Effect of long-term preservation on the mechanical properties of cortical bone in goats. Acta Orthop 2008; 79 (5): 708-16.
- Wingerter S, Calvert G, Tucci M, Benghuzzi H, Russell G, Puckett A. Mechanical strength repercussions of various fixative storage methods on bone. Biomed Sci Instrum 2006; 42: 290-5.
- Zdero R, Olsen M, Bougherara H, Schemitsch EH. Cancellous bone screw purchase: a comparison of synthetic femurs, human femurs, and finite element analysis. Proc Inst Mech Eng H 2008; 222 (8): 1175-83.
- Zech S, Goesling T, Hankemeier S, Knobloch K, Geerling J, Schultz-Brunn K, Krettek C, Richter M. Differences in the mechanical properties of calcaneal artificial specimens, fresh frozen specimens, and embalmed specimens in experimental testing. Foot Ankle Int 2006; 27 (12): 1126-36.