Abstract
Early-identified severe/profound hearing loss (HL) following universal newborn hearing screening (UNHS) has been associated with improved speech and language outcomes. However, speech outcome reports have typically been based on broad measures of speech intelligibility and/or singleton consonant accuracy, with little known about production of consonant clusters. Using a prospective design, the range and accuracy of consonant clusters produced by a homogenous cohort of 12 children early-identified with severe/profound HL aged 3- and 4-years were examined. All children demonstrated bilateral aided thresholds within a range of 15–25 dB HL across all frequencies, were optimally amplified with cochlear implants (11/12) or hearing aids (1/12), and attended auditory-verbal (AV) early intervention. Standardized speech and language assessments were administered. Consonant clusters were strategically sampled in single-word and conversational speech contexts. All standard scores for speech, receptive, and expressive language were within normal limits. All children produced consonant clusters commensurate with expectations for typically-developing hearing peers at 3- and 4- years-of-age. Children's production of phonetically complex morphophonemes (final consonant clusters marking grammatical morphemes) was also in keeping with developmental expectations. Factors which contributed to these encouraging outcomes require further investigation.
Introduction
The introduction of universal newborn hearing screening (UNHS), along with the availability of advanced technological and surgical innovations, have heralded significantly improved speech and language outcomes for children with congenital severe/profound hearing loss (HL) (e.g., CitationErtmer, Kloiber, Jung, Kirleis, & Bradford, 2012; CitationFulcher, Purcell, Baker, & Munro, 2012; CitationMay-Mederake, 2012; CitationWie, 2010). Of the research considering speech outcomes, reports have been based on broad measures of speech relative to children with later-identified HL (e.g., CitationFulcher et al., 2012; CitationYoshinaga-Itano, 2003; CitationYoshinaga-Itano, Coulter, & Thomson, 2001), or the accuracy of consonants and/or vowels (e.g., CitationDornan, Hickson, Murdoch, Houston, & Constantinescu, 2010; CitationErtmer et al., 2012). Across the speech outcome research, no study has described the range and accuracy of initial and final consonant clusters produced by children with early identified severe/profound HL—an important speech production skill historically considered challenging for children with HL (CitationOsberger & McGarr, 1982). If the benefits of early identification, particularly when accompanied by oral education, are to be recognized, further speech outcome research is needed. The study reported in the current paper addresses this need by describing the speech production skills, including the consonant clusters produced by children with early-identified severe/profound HL enrolled in auditory-verbal (AV) therapy. Literature on the speech outcomes of children with early- identified severe/profound HL is provided, followed by an overview of the extant literature on the acquisition and importance of consonant clusters for speech. Previous research on the production of consonant clusters by children with severe/profound HL is also considered.
Speech outcomes of children with early-identified severe/profound HL
Research on the speech production skills of children with early-identified severe/profound HL has been encouraging. The unintelligible speech previously noted for children with severe/profound HL (e.g., CitationGeffner, 1980) has more recently been described as intelligible for increasing proportions of this population. CitationGeers (2004), for example, reported that 57% of children with profound HL who had received a cochlear implant prior to age 2 years demonstrated normal speech intelligibility by 8 or 9 years. Other speech outcome research has been similarly encouraging. For instance, CitationErtmer et al. (2012) reported that a group of 11 children with severe/profound HL (eight of whom were identified post-UNHS) who were enrolled in oral education programs “showed substantial progress in [singleton] consonant acquisition” (p. 342).
In a study comparing the speech intelligibility and phonetic inventory size of children with HL identified post-UNHS (referred to as the screen group) vs children with HL not identified via UNHS (referred to as the no-screen group), CitationYoshinaga-Itano et al. (2001, p. 527) reported that “the children in the screen group had significantly better speech intelligibility than the children in the no-screen group and significantly more consonant blends”. The accuracy and range of different types of consonant clusters (blends) produced by the children was not reported. CitationFitzpatrick, Crawford, Ni, and Durieux-Smith (2011) reported that around half of 32 children with severe/profound HL (including children with early and later identified HL) achieved standard scores within the normal range using the Goldman- Fristoe Test of Articulation-2 (GFTA-2) (CitationGoldman & Fristoe, 2000). Collectively, such reports suggest that children with early-identified HL can develop clear, intelligible speech like their typically- developing hearing peers.
However, not all speech outcome research has been so encouraging. For example, CitationYoshinaga-Itano (2003, p. 204) reported that, when “screened”, children aged between 9–59 months were examined in discrete groups according to degree of HL, those diagnosed with severe/profound HL were rated by the phonetician, parent, and parent-infant provider as having “almost always unintelligible speech”. Similarly, although CitationErtmer et al. (2012) noted that, while the children with severe/profound HL in their study demonstrated improved consonant accuracy, the children's speech was “still delayed compared to age-matched peers” (p. 342). CitationFitzpatrick et al. (2011, p. 610) also noted that “just over half (11 of 21) of the children in the profound group and in the severe group (five of 11) exhibited speech production skills more than 1 SD below the mean for age-matched peers”. Along similar lines, CitationSininger, Grimes, and Christensen (2010) reported that 44 infants and toddlers with early-identified mild-to-profound bilateral hearing loss performed significantly below age-matched hearing peers on standardized testing. The reasons for this diversity in speech outcome research warrants further discussion.
Factors complicating the conduct and interpretation of speech outcome research
A closer inspection of the speech outcome research regarding children with early identified severe/profound HL would suggest that the findings have been complicated and influenced by the heterogeneous characteristics of the children involved in the research (CitationGeers, Nicholas, & Moog, 2007). Specifically, within and across studies, children have varied with respect to type of HL—congenital vs acquired (e.g., CitationErtmer et al., 2012); degree of HL—mild-to-profound (e.g., CitationFitzpatrick et al., 2011; CitationYoshinaga-Itano et al., 2001); hearing devices—hearing aids (HA) and/or cochlear implants (CI), without reference to functional accessibility to speech sounds (e.g., CitationDornan et al., 2010; CitationSininger et al., 2010); CI devices and programming strategies—older vs newer technology (e.g., CitationGeers, 2004); communication mode—oral, total communication, sign (e.g., CitationYoshinaga-Itano, 2003); and varied aided thresholds (e.g., CitationErtmer et al., 2012).
One way to address the heterogeneity of participants in speech outcome research is to report findings according to children's functional access to sound. For instance, CitationMadell and Flexer (2008) suggest that a bilateral level of aided amplification of at least 20–25 dB HL across all frequencies (regardless of device or device combination) is sufficient for good auditory access to all speech phonemes. Presumably, if children meet this standard of optimal access to sound, then they have the potential to develop clear, intelligible speech commensurate with their typically-developing peers.
The oral AV therapy approach adopts this principle of homogeneity based on functional access to sound in their management of children with HL (CitationAG Bell, 2011). Specifically, the decision regarding the type of amplification device(s) recommended for a child (e.g., bilateral hearing aids, or binaural/bimodal stimulation via CI and hearing aid in the contralateral ear, or a unilateral CI, or bilateral CIs) is dependent on the amount of consistent functional access to speech sounds demonstrated by the child (CitationAG Bell, 2011). In the current study, we report on speech production skills of a group of children early identified with severe/profound HL where all children presented within the recommended range of functional access to speech sounds (10 children via bilateral CIs; one child via CI + HA; one child via bilateral HAs). We focus specifically on the children's production of consonant clusters.
Consonant clusters—Why are they important to investigate?
One of the features of clear, intelligible speech is the ability to produce consonant clusters. Consonant clusters are sequences of two or more consonants within a syllable, typically occurring at the beginnings or ends of words such as plant /plænt/ (e.g., initial consonant cluster /pl/ and final consonant cluster /nt/). Consonant clusters are important to study for a number of reasons. First, consonant clusters are evident in many of the world's languages (CitationGreenberg, 1978). Second, one third of English monosyllables commence with a consonant cluster (e.g., blue /blu/; star /sta/; street /stɹit/) (CitationLocke, 1983). Third, consonant clusters dominate word-final position (e.g., last /last/; jump /ʤʌmp/) (CitationLocke, 1983) and are particularly important for marking phonetically complex morphophonemes—grammatical morphemes realized by consonant clusters, such as the non-finite plural (e.g., boats /boʊts/) and possessive (e.g., dog's /dɒɡz/) morphemes, as well as finite third person present singular (e.g., eats /its/) and past tense (e.g., asked /askt/) morphemes (CitationPaul & Shriberg, 1982). Fourth, the accurate use of consonant clusters has been associated with improved expressive language outcomes (particularly given their role in marking grammatical morphophonemes) and overall long-term superior literacy development (CitationOverby, Trainin, Smit, Bernthal, & Nelson, 2012). Fifth, difficulty with the production of consonant clusters (e.g., reduction of clusters from two or three elements to one, such as star /sta/ realized as [ta]) is common in pre-school-aged children with speech sound disorder (CitationHodson & Paden, 1981). Finally, and perhaps most importantly for the current study, the production of consonant clusters, and in particular complex morphophonemes, has been reported to be difficult for children with severe/profound HL (CitationGeers, Moog, Biedenstein, Brenner, & Hayes, 2009; CitationOsberger & McGarr, 1982).
Consonant clusters—Typical acquisition
Relative to singleton consonants, consonant clusters take longer to acquire (CitationHaelsig & Madison, 1986; CitationMcLeod, van Doorn & Reed, 2001a; CitationPriester, Post, & Goorhuis-Brouwer, 2011; CitationWaring, Fisher, & Aitkin, 2001). For instance, in a longitudinal study of 16 typically-developing Australian-English speaking children aged 2;0–2;11, CitationMcLeod, van Doorn, and Reed (2001b) reported a mean percentage of consonant clusters correct of 29.5% (SD = 21.2). This figure rises to 88.1% for children aged 4;0–4;11 (CitationWaring et al., 2001), and then 94.5% for children aged 5–12 years (CitationMcLeod & Arciuli, 2009).
As children learn to produce consonant clusters, a range of errors is possible. The most common error is cluster reduction, whereby two or three elements in the cluster are reduced to one or two (e.g., blue /blu/ → [bu]; street /stɹ/ → [st]) (CitationSmit, 1993). In a study of 50 English-speaking children aged 2;10–5;2 years, CitationHaelsig and Madison (1986) reported that the frequency of occurrence of cluster reduction decreased from 30% at age 3-years to 10% by 4;0 years. CitationGrunwell (1987) suggests that the process of cluster reduction disappears by ˜ 4-years, with errors after this time being cluster simplifications whereby one or more of the consonants comprising the cluster is in error (e.g., tree /tɹi/ → [twi]). Such cluster simplifications are typically the result of age- appropriate errors on later developing singleton consonants including /s/ and /ɹ/ (CitationMcLeod & Arciuli, 2009).
Consonant clusters in children with severe/profound HL
Of the research examining the consonant cluster abilities of children with severe/profound HL, the children have frequently been either later identified, or a combination of early and later identified. For example, one long-term study which examined consonant cluster acquisition in children who used a CI reported that, of nine children aged between 7–10-years (mean CA = 9;9) who received CIs ≤ age 5 years, the mean percentage correct production of words within conversational speech was 92% for monophthongs, 89% for diphthongs, 82% for consonants, 43% for consonant clusters and 61% for whole words (CitationBlamey, Barry, Bow, Sarant, Paatsch, & Wales, 2001). Blamey et al. concluded that the children demonstrated considerable speech development gains previously not seen in children with profound HL. While encouraging, it was also noted that the participants’ progress was considerably slower relative to children with typical hearing. The possibility that their participants were not early diagnosed given that the range of hearing aid fitting was 9–40 months and that cochlear implantation ranged from 2;6–5;2 must also be considered. The enrolment into auditory-oral (six participants) or total communication (three participants) intervention programs may also have influenced these findings. No mention of functional access to sound was provided. Given that overall percentage of consonant clusters correct was reported, it was unknown whether there were any differences in the children's productions of word-initial vs word-final consonant clusters. Furthermore, it was unknown whether any of the clusters produced by the children were complex morphophonemes.
Word initial consonant cluster acquisition has been the focus of a small number of studies involving children with early diagnosed congenital profound HL who receive CIs prior to 5 years (CitationChin, 2002, Citation2007). CitationChin (2002) studied initial consonant cluster production by 12 children who had used a CI for a minimum of 5 years and either used oral or total communication—a combination of oral and sign. Chin reported that all children had patterns of acquisition similar to typically-developing children with respect to production of word initial clusters, although at delayed chronological ages. For example, the percentagr consonant cluster correct ranged from 0–89%, with a mean of 48 (SD = 36%) at a minimum chronological age of 6;4, with children using oral communication significantly outperforming children using total communication. In an extension to his 2002 study, CitationChin (2007) examined the variation in consonant clusters produced by children who used a cochlear implant using the framework of Optimality Theory. The results of this study similarly indicated that “the realization of consonant clusters by children with cochlear implants is ... not chaotic or random, but ... reflects general structural principles that constrain variation in all linguistic systems” (CitationChin, 2007, p. 9S). The accuracy of the children's productions with respect to developmental expectations was not noted. While it is possible that some of the participants in CitationChin's (2002, 2007) studies had typically-developing speech, it is also possible that some of the children were not using the full range of initial consonant clusters expected for their age. Factors that may have influenced findings in both of CitationChin's (2002, 2007) studies included participants with various ages of onset of HL (Range: 0–2;1), various ages of cochlear implantation (Range: 1;4–6;1), and various ages of assessment (Range: 6;4–11;1 in 2002; 6;4–16;5 in 2007). Finally, while CitationChin's (2002, 2007) research focused on initial consonant clusters, little is known about the ability of children with early identified severe/profound HL to produce final consonant clusters.
In summary, although it has been reported that children with severe/profound HL are capable of producing initial consonant clusters, it is unknown whether the range and accuracy of consonant clusters produced by children with early-identified severe/profound HL can be commensurate with expectations for typically-developing hearing peers of the same chronological age. Given that research on the speech outcomes of children with early- identified HL has been complicated by inclusion of children with varying characteristics (e.g., age of diagnosis, age of fitting of individually optimized amplification technologies, various intervention approaches, and communication modes), research designed to examine the consonant cluster abilities of these children would benefit from an investigation of a relatively homogenous cohort of children.
Thus, the purpose of the current study was to extend the existing knowledge regarding consonant clusters produced by children with early identified severe/profound HL by examining the accuracy and range of 2- and 3-element initial and final consonant clusters (including complex morphophonemes) produced by a homogenous cohort of 3- and 4-year old UNHS children with early identified severe/profound HL in single-word and conversational speech contexts. All children were enrolled in AV therapy by age 6 months and had received optimized devices for hearing. All children demonstrated bilateral aided thresholds within a range of 15–25 dB HL across all frequencies, regardless of whether they had optimal access to sound using bilateral CIs, a single CI with contralateral HA, or bilateral HA's. Thus, an homogenous feature of the group of children related to functional access to the sounds of speech rather than the choice of device per se.
Method
This was a prospective investigation of the acquisition of consonant clusters in an homogenous cohort of Australian children with early-identified bilateral congenital severe/profound sensorineural HL.
Participants
Inclusion criteria for the participants included diagnosis of HL, amplification and enrolment in AV intervention prior to ≤ 6 months of age. Potential participants were identified prospectively via initial enrolment procedures at The Shepherd Centre following early identification of HL post-local UNHS services (CitationNSW State Government Health, 2011). describes the exclusion criteria and participant flow. The final cohort consisted of 12 children: eight children aged 3-years (five males/three females) and eight children aged 4-years (six males/two females). Of these participants, four children were available at both 3- and 4-years-of-age. All participants satisfied the Joint Committee on Infant Hearing (CitationJCIH, 2007) best practice guidelines. Frequently referred to as the 1-3-6 guidelines, this position statement recommends UNHS by 1 month-of-age, verification of HL/amplification by 3-months and commencement with early intervention services by 6 months-of-age. All children had commenced oral AV early intervention at one of five regional sites of The Shepherd Centre, NSW (http://www.shepherdcentre.org.au) prior to the age of 6 months. Early diagnosis and state-of-the-art audiologic management using technology which is consistently worn and monitored at all five city and regional Shepherd Centres are essential components of the AV therapy approach (CitationAG Bell, 2011). Audiology services as well as weekly playgroups and parent support groups are available at each centre. Children with severe/profound HL typically receive weekly 1–1 AV therapy sessions. Collaborative teamwork of family, audiologists, auditory-verbal therapists, and parent support groups are integral to the AV approach. Weekly full-team meetings facilitate conscientious monitoring of progress and optimal device fitting for each individual child.
Figure 1. Process of exclusion for participants. HL, Hearing Loss; S/P, Severe/Profound; M, male; F, female; AV, auditory-verbal; y, years. Framework based on format proposed by CitationVan Kleeck, Schwarz, Fey, Kaiser, Miller, & Weitzman (2010) and CitationBoutron, Moher, Altman, Schulz, Ravaud, & for the, C. G. (2008). aDegree of hearing impairment based on CitationClark (1981): Severe/Profound > 65 dB SPL bilaterally. bEight children were assessed at 3 and 4 years, with four children assessed at both 3 and 4 years.
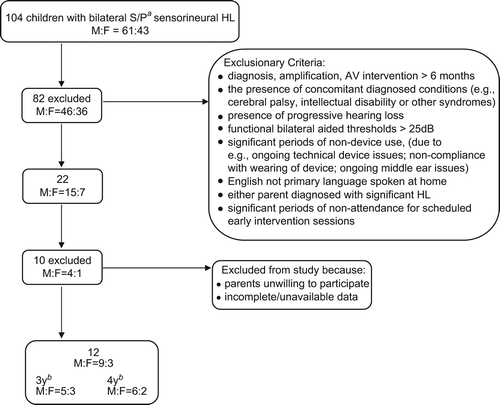
The average age of diagnosis was 4.42 (SD = 3.80) weeks (Range = 1–12 weeks). The average age of bilateral hearing aid fitting was 1.96 (SD = 1.14) months (Range = 1–4 months). All children were fitted with bilateral amplification devices at the time of assessments. Bilateral aided thresholds for all children were checked immediately prior to each assessment and were found to meet the inclusionary recommended criteria of a range of at least 20–25 dB HL across all frequencies (CitationMadell & Flexer, 2008). All children with profound HL (n = 10) wore bilateral CIs. The average age of initial CI activation was 10.64 (SD = 4.32) months (Range = 6–18 months). All participants using a CI (n = 11) had their first CI < 18 months, with 9/11 receiving their first CI ≤ 12 months-of-age. Ten participants had bilateral CIs prior to their first assessment at 3 years. The average age of the second CI activation was 29.6 (SD = 10.16) months. One child with severe/profound HL wore a unilateral CI with a HA in the contralateral ear. Auditory checks indicated the child was receiving the recommended bilateral aided access or better, at 15–25 dB HL. Another child with severe/profound HL wore bilateral hearing aids, as functional auditory responses similarly indicated appropriate aided benefit within the recommended 20–25 dB HL range utilizing this form of amplification. The children's hearing status, bilateral aided threshold ranges, cochlear implantation age of activation, gender, SES and maternal education levels are reported in . For purposes of maintaining anonymity, all children were de-identified, with pseudonyms replacing actual names.
Table I. Demographics and characteristics of participants.
Other factors contributing to the homogeneity of the group included; all participants had congenital HL with no significant deterioration in hearing during the period of research; all participants with severe/profound HL had trialled hearing aid amplification prior to CI candidacy, with the average age of bilateral HA fitting being 1.95 months; all CI devices were the same model (viz., COCHLEAR Nucleus Freedom), programmed with the ACE speech coding strategy; all hearing aids were the same generation of technology supplied through the Australian government, Australian Hearing-National Acoustic Laboratories (AH-NAL) and programmed according to NAL prescriptions (CitationDillon, 2006); case files indicated consistent use of amplification devices by all participants. In addition, all participants scored within-normal-limits (WNL ≥ 85) on standard scores for receptive, expressive, and total language on The Preschool Language Scale-4 (CitationZimmerman, Steiner, & Pond, 2002) and on The Peabody Picture Vocabulary Test, Fourth Edition (PPVT™-4) (CitationDunn & Dunn, 2007) at 3 and 4 years-of-age. The study was conducted in accordance with the ethical standards stipulated by the University of Sydney Ethics committee for research involving human subjects (Protocol Number 9551).
Speech assessment measurements
The Goldman Fristoe Test of Articulation-2 (GFTA-2) (CitationGoldman & Fristoe, 2000) was administered at ages 3- and 4-years. The speech samples gathered using GFTA-2 were used to calculate the participants’ standard scores (SS) in addition to percentage of consonants correct (PCC) across initial, medial, and final position, and percentage of vowels correct (PVC), in a single word context. Given that the GFTA-2 does not sample a large range of initial or final consonant clusters, two additional speech samples were gathered from each participant—a single word sample of initial and final clusters using the Single Word Test of Consonant Clusters (CitationMcLeod & Hand, 1991), and a conversational speech sample. The Single Word Test of Consonant Clusters is a picture-based non-standardized elicitation tool comprising 72 words, and provides an opportunity to sample two instances of 36 consonant clusters (27 word-initial and nine word-final) commonly used in Australian-English (CitationMcLeod, 1997). The conversational speech task sampled both initial and final clusters (including those marking complex morphophonemes), during a naturalistic, semi-controlled context involving a set of Lego zoo animals and additional props each brought out in a consistent order while following a script (based on CitationMcLeod, 1997). The animals and props were strategically selected to sample consonant clusters in mono and polysyllabic words (see the Supplementary Appendix available online at http://informahealthcare.com/doi/abs/10.3109/17549507.2013.808698). A minimum of 100 utterances were recorded and analysed for each participant.
A descriptive speech intelligibility rating scale (SIR) was used to provide a qualitative rating of the children's speech intelligibility from the conversation samples (CitationAllen, Nikolopoulos, Dyar, & O’Donoghue, 2001). Consensus ratings were agreed upon after independent ratings by the first author, the child's therapist, and the child's parent. This scale is reported to have a high level of inter-observer reliability by the developers and consists of five categories: 1 = connected speech is unintelligible up to 5 = connected speech is intelligible to all listeners.
All speech samples were video-recorded using a SONY Digicam HDR SR1E optimally positioned in order to capture visual and audio records for all participants. Real-time, broad phonetic transcriptions of all speech samples were carried out by the first author. Ten per cent of the GFTA-2, Single Word Test of Consonant Clusters (CitationMcLeod & Hand, 1991) and conversational speech samples were re-transcribed by the first and second authors. Based on point-by-point agreement, inter-judge reliability was 94.7%, while intra-judge reliability was 99.2%. These values were comparable to others reported in the literature (e.g., CitationFlipsen & Parker, 2008).
Analysis of consonant clusters
The Computer Aided Speech and Language Analysis (CASALA) program (CitationBlamey, 1997) was used to analyse all speech samples. All speech transcriptions were entered into the computer by hand then analysed by the program, with printouts provided for interpretation. From this information, the participants’ PCC and PVC were computed for the GFTA-2 (CitationGoldman & Fristoe, 2000). Independent and relational inventories of consonant clusters in initial and final positions were generated for all participants using both the Single Word Test of Consonant Clusters (CitationMcLeod & Hand, 1991) and conversation samples. An independent inventory of consonant clusters was defined as the consonant clusters produced by a child, irrespective of the correct production in the adult phonology. Relational inventories comprised only correctly produced clusters. Clusters were included if they occurred at least twice, with marginal clusters (occurring once) also identified (in keeping with CitationMcLeod et al., 2001b). Cluster categories produced, such as C+ /w/ (e.g., queen [kwin]); C + /l/ (e.g., clean [klin]); C + /r/ (e.g., cream [krim]); /s/ + C (e.g., star [sta]), and three element clusters (e.g., 3 CCC street [strit]) were identified in keeping with CitationMcLeod et al. (2001a) and CitationPowell (1995). Inventories of consonant clusters were further categorized with respect to whether they were initial or final word position, with complex morphophonemes identified from the final position inventories.
Statistical analysis
Descriptive statistics are reported for independent and relational inventory analyses. Wilcoxon Signed Rank Tests for initial vs final consonant cluster accuracy at chronological ages of 3- and 4-years were applied to both sampling contexts. Effect size is reported using non-parametric effect size (d-np) (CitationFlipsen, 2003).
Results
Overall speech production skills: Percentage of singleton vowels (PVC) and consonants (PCC) correct within GFTA-2 and conversation samples
Findings for PVC and PCC for single words in GFTA-2 suggest that any consonant cluster errors would not be due to atypical or delayed performance in singleton vowel and consonants across all participants. Descriptive statistics for the PVC and PCC for single words in GFTA-2 indicate results commensurate with reports of typically-developing Australian children (CitationMcLeod, 2007). Specifically, the mean PVC at age 3-years for GFTA-2 was 98% (SD = 1.7%; Range = 95–100), while the mean PCC at age 3-years was 81% (SD = 5.8%; Range = 77–91). Similarly, at age 4-years, the mean PVC was 99% (SD = 0.9; Range = 98–100) while the mean PCC was 90% (SD = 4.7%; Range = 79–94). PCC in conversation samples similarly followed typical developmental expectations in light of normative reports (in CitationMcLeod, 2007) with mean PCC at age 3-years being 85% (SD = 5.4%; Range = 75–92) and mean PCC at age 4-years being 91% (SD = 3.6%; Range = 87–98).
Speech intelligibility rating scales of conversation samples
All children were rated at either level 5 (connected speech is intelligible to all listeners. Child is understood easily in everyday contexts) or level 4 (connected speech is intelligible to a listener who has little experience of a deaf person's speech) (CitationAllen et al., 2001) for intelligibility of their conversation samples. At age 3-years, 38% of the children were rated at level 5, rising to 75% at age 4-years. These results compare favourably with results for children with CIs summarized by CitationFlipsen (2008).
Accuracy and range of initial and final consonant clusters in single word and conversational speech contexts
Data from show that all children followed typical developmental trends with respect to the accuracy of their production of consonant clusters (based on CitationHaelsig & Madison, 1986; CitationMcLeod et al., 2001a, b; CitationWaring et al., 2001) with steady increases in Percentage of Consonant Clusters Correct (PCCC) from 3- to 4-years in both initial and final position in single words (SW) and conversational speech (CS). In summary, the average PCCC at age 3-years was 61.75% (SD = 13.2; Range = 46–85) (SW) and 60.88% (SD = 19.6; Range = 25–85) (CS) for initial position, 82.63% (SD = 7.7; Range = 72–95) (SW) and 79% (SD = 10.8; Range = 67–94) (CS) in final position, and 69.13% (SD = 10.2; Range = 58–89) (SW) and 67.75% (SD = 12.3; Range = 46–85) (CS) overall. These results compare favourably with data from CitationMcLeod et al. (2001b), for typically-developing Australian children in the conversational speech context.
Table II. Percentage consonant clusters correct (PCCC) and phonological processes affecting production in single words (SW)a and PCCC in conversation.
By age 4-years, participants in the current study achieved an average PCCC of 82% (SD = 11.9; Range = 75–98) (SW) and 78.63% (SD = 13.0; Range = 58–96) (CS) for initial position, 96.25% (SD = 7.1; Range = 82–100) (SW) and 88.13% (SD = 9.2; Range = 76–100) (CS) in final position, and 86.5% (SD = 9.1; Range = 70–8) (SW) and 83% (SD = 10.5; Range = 70–99) (CS) overall. These results are slightly lower than results reported by CitationWaring et al. (2001), who reported average PCCC scores of 88.1% for children with typical hearing aged 4;0–4;11. However, different sampling task conditions make direct comparisons difficult.
Wilcoxon Signed Rank Test results indicated PCCC for final consonant clusters were significantly better than PCCC for initial consonant clusters within single words at 3-years (Median PCCC Final = 81.50, IQR = 13, Median PCCC Initial = 59.50, IQR = 20, Z = −2.521, p = .012, d-np = .89) and 4-years-of-age (Median PCCC Final = 100.00, IQR = 9, Median PCCC Initial = 82.00, IQR = 18, Z = −2.380, p = .017, d-np = .76). A marginal effect was observed between the PCCC for final consonant clusters in conversation and the PCCC for initial consonant clusters at age 3-years (Median PCCC Final = 78.00, IQR = 22, Median PCCC Initial = 58.5, IQR = 29, Z = −1.960, p = .050, d-np = .70), while a significant difference was observed at age 4-years, with final consonant cluster accuracy being superior to initial consonant cluster accuracy in conversation (Median PCCC Final = 88.50, IQR = 24; Median PCCC Initial = 80.00, IQR = 22, Z = −2.035; p = .042; d-np = .48). This finding of final consonant clusters being significantly more accurate relative to initial consonant clusters was consistent with previously reported trends and suggested that the children's production of consonant clusters was in keeping with those of typically-developing hearing children (CitationMcLeod et al., 2001a, b).
also shows that the range of errors observed across the children's attempted productions of consonant clusters, the percentage of cluster reduction (e.g., spoon /spun/ = [pun]), cluster simplification (e.g., grass /ɡɹas/ = [ɡwas]), and the rare occurrence of cluster deletion (e.g., frog /fɹɒɡ/ = [ɒɡ]) appeared to follow trends for typical consonant cluster acquisition (CitationHaelsig & Madison, 1986). The process of cluster reduction had reduced to ˜ 10% by 4-years for all participants, also along the lines of CitationHaelsig and Madison (1986) findings. In addition, the range of errors in clusters appeared to be a result of developmentally appropriate singleton consonant errors or error patterns (CitationSmit, 1993). For example, if a child exhibited the age-appropriate phonological process of gliding on singleton consonants, gliding was evident in clusters containing /ɹ/ (e.g., [ɡwin] for green /ɡɹin/).
The number of independent and relational clusters produced in both the Single Word Test of Consonant Clusters (CitationMcLeod & Hand, 1991) and conversational speech samples are presented in and . There are two opportunities to accurately produce 27 different initial consonant clusters and nine different final clusters in the Single Word Test of Consonant Clusters. Christina, for example, was able to produce 20/27 initial clusters at age 3-years, which increased to 26/27 at age 4-years. Of the 27 initial clusters, 22 are 2-element clusters, with five containing 3-elements. Of the 20 accurately produced initial clusters, Christina was able to accurately produce four 3-element initial clusters, viz., /skɹ, skw, spɹ, stɹ/ by age 3-years. Results suggested that the children's production of consonant clusters within single words in both initial and final positions were in keeping with those of typically-developing hearing children (CitationMcLeod et al., 2001a, b). The number of clusters in the relational inventories for each child at 3- and 4-years produced within the conversational samples also appeared to be similar to those of typically- developing hearing children, of a similar chronological age (CitationBarlow, 2004; CitationDyson, 1988).
Table III. Number of consonant clusters in participants’ independent and relational consonant cluster inventories in single-words.a
Table IV. Number of initial and final consonant clusters, including phonetically complex morphophonemes in relational inventories in conversational speech.
indicates the number and type of accurate final consonant clusters produced from the conversational speech samples, including the percentage of final clusters marking complex morphophonemes. In summary, an average of 58.9% of final clusters were morphological markers at age 3-years, rising to 62.1% at age 4-years. Additionally, the range of final clusters in the relational inventories for each child at 3- and 4-years appeared to be similar to those of typically-developing hearing children, of a similar chronological age (CitationBarlow, 2004; CitationDyson, 1988).
presents the range of initial and final consonant clusters produced accurately by the participants using the Single Word Test of Consonant Clusters (CitationMcLeod & Hand, 1991) compared to the results reported by CitationSmit, Hand, Freilinger, Bernthal, and Bird (1990) for a large study involving typically-hearing children. Final cluster percentages were not available for the CitationSmit et al. (1990) data. If age of acquisition is defined as 75% correct and age of mastery as 90% correct (CitationPriester et al., 2011) it can be seen that, at age 3-years, 25 2-element initial clusters were acquired by the children in the current study, with 11 mastered; three 3-element initial clusters were acquired, and one (/skw/) was mastered. At age 4-years, 30 2-element initial clusters were acquired, with 21 mastered; five 3-element initial clusters were acquired, with four mastered. If individual percentages for all initial clusters are compared to CitationSmit et al. (1990) findings, then data for children in the current study compared favourably for each cluster.
Table V. Percentage correct responses for initial and final consonant clusters for children in the current study and CitationSmit et al. (1990) at 3- and 4-years-of-age.a,b
Given that the children's inventories were quite extensive, we illustrate the range of final consonant clusters in the inventories for two children. Using the results from the Single Word Test of Consonant Clusters (CitationMcLeod & Hand, 1991), one child (pseudonym Alana) produced the maximum relational inventory of 10 final clusters at 3-years and 10 final clusters at 4-years. In conversation, she produced 13 final clusters at 3-years and 18 at 4-years. Moreover, within the final clusters relational inventory for conversation, 9/13 were morphological markers (/ŋk dz ks lp lz nd nt ps st tl ts kst nts/) at 3-years, and 14/18 (/ŋk dz fs ks ld lz mp mz nʤ nd ns nt nz ps st ts vz nts/) at 4-years. The range of markers included both finite (e.g., past tense in missed realized as [mɪst], regular third person singular in wants realized as [wɒnts]), non-finite (e.g., possessive in William's realized as [wɪljəmz] and plural in clouds realized as [klаʊdz]). Similarly, Winslow had a final consonant cluster relational inventory of 12 at 3-years and 18 at 4-years, during conversational speech. Within these inventories, 6/12 at 3-years (/ʃt ls lz pz ts ksd/) and 11/18 at 4 years (/ʃt dz ft gz ks lz nz ps pz ts nts/) were complex morphophonological markers. Overall, the smallest final consonant cluster relational inventory within conversation was 6 (Range = 6–13) at 3-years and 6 (Range = 6–19) at 4-years. Also of interest was the ability of 75% of 3- and 4-year olds to include at least one final 3-element morphological marker cluster (e.g., /nts/ as in wants [wɒnts]).
Discussion
The purpose of the current study was to investigate the accuracy and range of initial and final consonant clusters produced by a homogenous cohort of 3- and 4-year-old children with early identified congenital bilateral severe/profound HL. All children satisfied inclusion criteria which included receiving treatment according to the Joint Committee on Infant Hearing 1-3-6 guidelines (CitationJCIH, 2007). That is, all children were screened for hearing loss by 1 month-of-age, received verification of HL/amplification by 3 months, and commenced early intervention services by 6 months-of-age. In addition, all children participated exclusively in AV therapy, had no other diagnosed special needs, and utilized the same CI technology and programming strategies and/or optimal HA technologies by < 18 months-of-age. Bilateral aided thresholds for all children fell within the range of 15–25 dB HL across all frequencies, which has been described as sufficient for good auditory access to all speech phonemes (CitationMadell & Flexer, 2008).
In summary, the children's performance on a standardized test of articulation accuracy (GFTA-2: CitationGoldman & Fristoe, 2000) was within normal limits. Measures of phoneme accuracy (PCC and PVC) in single-word and conversational speech contexts indicated that the children's productions of singleton phonemes were appropriate for their age and as such would not have hampered their efforts to produce consonant clusters. Accuracy of consonant cluster production, based on PCCC scores from the Single Word Test of Consonant Clusters (CitationMcLeod & Hand, 1991), and range of consonant clusters produced, was consistent with expectations for typically-developing hearing children (CitationHaelsig & Madison, 1986; CitationMcLeod et al., 2001a, b; CitationWaring et al., 2001) across all participants at 3- and 4-years. The inclusion of conversational speech samples showed that not only was the accuracy of the children's PCCC scores consistent across the two different sampling conditions, but that they used a range of 2- and 3-element consonant clusters including complex morphophonemes. These results were encouraging not only because such markers have previously been reported as difficult for later diagnosed children with cochlear implants (CitationGeers et al., 2009), but also because they often contained high frequency phonemes (e.g., /s/) known to be acoustically challenging for children with severe/profound HL (CitationMoeller, Hoover, Putman, Arbataitis, Bohnenkamp, Peterson, et al., 2007; CitationOsberger & McGarr, 1982). Specifically, in our study an average of 58.9% of final consonant clusters at 3-years and 62.1% at 4-years were complex morphophonemes. Moreover, regarding initial clusters, in light of CitationChin's (2002) findings where a cohort of children aged 6;4–11;1 produced on average 75% PCCC in initial position, our current findings encouragingly indicate relatively better and more age-appropriate performance of an average 82% PCCC in initial position at age 4-years.
Since the process of acquiring consonant clusters is considered to be more challenging than single consonant attainment, a longer time period for acquisition is required (CitationHaelsig & Madison, 1986; CitationPriester et al., 2011). The age of mastery of the majority of consonant clusters for typically-developing children has been reported to be between 6–7-years with complete mastery between 8–9-years (CitationMcLeod & Arciuli, 2009). Data obtained in the current study indicate all participants were not only following regular stages of development but were doing so at typical chronological ages for acquisition or mastery (CitationMcLeod et al., 2001b; CitationSmit et al., 1990). This finding not only contrasts with previous reports about the production of consonant clusters by children with severe/profound HL (CitationBlamey et al. 2001; CitationChin, 2002, Citation2007), but the idea that typical patterns (but not necessarily age-appropriate) speech acquisition cannot be expected of children who use CIs (CitationErtmer & Goffman, 2011). Although encouraging, caution in the interpretation of the current data must be noted due to the small numbers of children in the sample sizes at each age.
The importance of thorough, ongoing functional evaluation in order to regularly check the benefit of the various amplification options is supported by the data from this study. Two children with severe/profound HL were not bilateral CI recipients. However, these children were able to achieve similar scores to those children with bilateral CIs. While it is widely recognized that children with congenital profound HL will typically receive significantly greater benefit from early receipt of bilateral CIs (e.g., CitationChing, Van Wanrooy, & Dillon, 2007; CitationYoshinaga-Itano, 2011), some children with severe/profound HL may do equally as well with a CI and contralateral HA or two hearing aids. Indeed, CitationChing et al. (2007) suggest that, for children with some degree of usable residual hearing, the optimal amplification fitting may be the binaural/bimodal option, with the CI providing enhanced high frequency stimulation, and the contralateral HA typically providing better quality low frequency information. The current Australian recommended practice for CI evaluation is to follow the 1-3-6 guidelines, followed by close monitoring of not only audiometric results but also the individual functional access to the sounds of speech (e.g., CitationMadell & Flexer, 2008). This approach is well supported within AV early intervention programs where regular liaison and close teamwork between audiologists and therapists is regarded as an essential component of service provision (see http://www.agbellacademy.org/principal-auditory.htm).
The value of early CI activation for children with congenital profound HL, between ages 1–2.5 years, has been indicated in other studies (e.g., CitationDornan et al., 2010), with early bursts in growth curves of consonant production accuracy reported. However, it has remained uncertain whether these early bursts could lead to normalized speech development by school entry, or, indeed, whether typical development could be possible as early as ages 3- or 4-years. Our findings support the notion that typical speech development is indeed possible as early as 3-years of age, particularly with regard to consonant cluster acquisition.
Our findings contrast with previous reports that have suggested that although children with early-identified HL perform better than children with late-identified HL, they typically perform significantly below age-matched hearing peers on measures of speech production, particularly in the pre-school years (e.g., CitationErtmer et al., 2012; CitationFitzpatrick et al., 2011; CitationSininger et al., 2010; CitationYoshinaga-Itano, 2003). Reasons for the positive speech outcomes of the children in the current study require consideration. First, relative to previous speech outcome research, the children in the current study were considerably more homogenous. Second, all the children received the recommended (CitationDornan et al., 2010, p.378) “international gold standard” treatment (CitationJCIH, 2007) involving identification by 1-month, confirmation of HL and amplification by 3-months and early intervention by 6-months. It is possible, although not provable, that both early identification and early AV early intervention contributed to the children's positive speech outcomes in some way.
According to CitationSharma (2010, p. 1) the first 18 months in a child's life is a period of “maximal synaptogenesis” in the auditory cortex—a period when the auditory system is plastic and undergoing considerable change and development. Sharma predicts that optimal access to listening within this time frame may equate with normalized speech and language development. Early optimal access to sound, as was the case for all the children in the current study, may not only provide a chance to avoid the previous reported delays in speech and language exhibited by children with severe/profound HL (e.g., CitationConrad, 1979; CitationOsberger & McGarr, 1982), but it may also offer the opportunity for typical speech development alongside that of hearing peers.
Another source of success for children in this current study may lie in the particular type of AV therapy received by all participants from very early ages. It is possible that the exclusive focus on listening (auditory) and speech production (verbal) coupled with the high degree of parental involvement and training contributed to the children's range and accuracy of consonant clusters commensurate with reports of their typically-developing hearing peers. If the specific benefits of AV intervention are to be established, however, future research would need to examine management according to the 1-3-6 guidelines (CitationJCIH, 2007), whereby AV intervention is compared with other approaches to early intervention.
Positive outcomes aside, it is yet unknown whether the children in this current study will continue to develop high levels of speech intelligibility. Equally, further investigations are required to examine whether positive long-term language and literacy outcomes previously reported to be associated with age-appropriate consonant cluster acquisition (CitationOverby et al., 2012) do indeed eventuate. The ongoing detailed analysis and monitoring of speech and language progress of larger cohorts of children, who receive optimized amplification and/or cochlear implants in their first year of life within particular early intervention service models, will be important for informing our evidence base.
Conclusion
Parents of UNHS children diagnosed with severe/profound HL frequently express the desire for clear speech production for their children and the difficult dilemma of how best to facilitate this goal (CitationKluwin & Stewart, 2000). This study of a homogenous cohort of UNHS identified 3- and 4- year-old children diagnosed with congenital severe/profound HL, who all attended AV early intervention, who were all optimally amplified with functional access to the sounds of speech within the required 20–25 dB across all frequencies, and received CIs, if required, prior to 18 months-of-age, has shown that not only are these children capable of performing WNL on standardized tests of speech and language, but that they are capable of producing a range of 2- and 3-element initial and final consonant clusters at an age commensurate with their typically-developing hearing peers. For parents wanting to know whether age-appropriate speech is possible, the answer would appear to be yes. Intelligible, age-appropriate speech is possible for children with early identified severe/profound HL. The exact conditions which facilitate such encouraging outcomes are for future research.
http://informahealthcare.com/doi/abs/10.3109/17549507.2013.808698
Download PDF (174.9 KB)Declaration of interest: The authors report no conflicts of interest or financial relationships relevant to this article.
References
- Alexander Graham Bell (AG Bell), Association for Listening and Spoken Language. (2011). Auditory-verbal therapy. http://www.agbellacademy.org/principal-auditory.htm.
- Allen, C., Nikolopoulos, T., Dyar, D., & O’Donoghue, G. (2001). Reliability of a rating scale for measuring speech intelligibilty after pediatric cochlear implantation. Otology and Neurotology, 22, 631–633.
- Barlow, J. A. (2004). Consonant clusters in phonological acquisition: Applications to assessment and treatment. CSHA Magazine, 34, 10–13.
- Beveridge, A., & Weber, S. (2003). Income, education and occupation data analysis of 2000 and 2003 US Census Bureau. New York: Queens College Sociology Department.
- Blamey, P. (1997). Computer Aided Speech and Language Assessment: Version Student 2.0. Melbourne: University of Melbourne.
- Blamey, P., Barry, J., Bow, C., Sarant, J., Paatsch, L., & Wales, R. (2001). The development of speech production following cochlear implantation. Clinical Linguistics and Phonetics, 15, 363–382.
- Boutron, I. M. D. P., Moher, D. P., Altman, D. G. D., Schulz, K. F. P. M. B. A., Ravaud, P. M. D. P., & for the, C. G. (2008). Extending the CONSORT statement to randomized trials of nonpharmacologic treatment: Explanation and elaboration. Annals of Internal Medicine, 148, 295–309.
- Chin, S. (2002). Consonant cluster production by pediatric users of cochlear implants. The Volta Review, 102, 157–174.
- Chin, S. B. (2007). Variation in consonant cluster production by pediatric cochlear implant users. Ear and Hearing, 28 (Suppl.), 7S–10S.
- Ching, T., Van Wanrooy, E., & Dillon, H. (2007). Binaural-bimodal fitting or bilateral implantation for managing severe to profound deafness: A review. Trends in Amplification, 11, 161–192.
- Clark, J. G. (1981). Uses and abuses of hearing loss classification. American Speech-Language-Hearing Association, 23, 493–500.
- Conrad, R. (1979). The deaf child. London: Harper and Row.
- Dillon, H. (2006). What’s new from NAL in hearing aid prescriptions?. Hearing Journal, 59, 10–16.
- Dornan, D., Hickson, L., Murdoch, B., Houston, T., & Constantinescu, G. (2010). Is auditory-verbal therapy effective for children with hearing loss?. The Volta Review, 110, 361–387.
- Dunn, L. M., & Dunn, D. M. (2007). Peabody Picture Vocabulary Test (4th ed.). Circle Pines, MN: Pearson Assessments.
- Dyson, A. T. (1988). Phonetic inventories of 2- and 3- year old children. Journal of Speech and Hearing Disorders, 53, 89–93.
- Ertmer, D. J., & Goffman, L. A. (2011). Speech production accuracy and variability in young cochlear implant recipients: Comparisons with typically developing age-peers. Journal of Speech, Language, and Hearing Research, 54, 177–189.
- Ertmer, D. J., Kloiber, D., Jung, J., Kirleis, K., & Bradford, D. (2012). Consonant production accuracy in young cochlear implant recipients: Developmental sound classes and word position effects. American Journal of Speech-Language Pathology, 21, 342–353.
- Fitzpatrick, E., Crawford, L., Ni, A., & Durieux-Smith, A. (2011). A descriptive analysis of language and speech skills in 4- to 5-year-old children with hearing loss. Ear and Hearing, 32, 605–616.
- Flipsen, P. (2003). Articulation rate and speech-sound normalization failure. Journal of Speech, Language, and Hearing Research, 46, 724–737.
- Flipsen, P. (2008). Intelligibility of spontaneous conversational speech produced by children with cochlear implants: A review. International Journal of Pediatric Otorhinolaryngology, 72, 559–564.
- Flipsen, P., & Parker, R. (2008). Phonological patterns in the speech of children with cochlear implants. Journal of Communication Disorders, 41, 337–357.
- Fulcher, A., Purcell, A., Baker, E., & Munro, N. (2012). Listen up: Children with early identified hearing loss achieve age- appropriate speech/language outcomes by 3 years-of-age. International Journal of Pediatric Otorhinolaryngology, 76, 1785–1794.
- Geers, A. E. (2004). Speech, language, and reading skills after early cochlear implantation. Archives of Otolaryngology-Head and Neck Surgery, 130, 634–638.
- Geers, A. E., Moog, J. S., Biedenstein, J., Brenner, C., & Hayes, H. (2009). Spoken language scores of children using cochlear implants compared to hearing age-mates at school entry. Journal of Deaf Studies and Deaf Education, 14, 371–385.
- Geers, A. E., Nicholas, J. G., & Moog, J. S. (2007). Estimating the influence of cochlear implantation on language development in children. Audiological Medicine, 5, 262–273.
- Geffner, D. (1980). Feature characteristics of spontaneous speech production in young deaf children. Journal of Communication Disorders, 13, 443–454.
- Goldman, R., & Fristoe, M. (2000). Goldman-Fristoe-Test of Articulation-2. Circles Pines, MN: American Guidance Service.
- Greenberg, J. H. (1978). Some generalizations concerning initial and final clusters. In J. Greenberg, C. Ferguson & E. Moravcsik (Ed.), Universals of human language: Phonology (pp. 243–279). Stanford, CA: Stanford University Press.
- Grunwell, P. (Eds). (1987). Clinical phonology (2nd ed.). London: Croom Helm.
- Haelsig, P., & Madison, C. (1986). A study of phonological processes exhibited by 3-, 4-, and 5-year-old children. Language, Speech, and Hearing Services in Schools, 17, 107–114.
- Hodson, B., & Paden, E. (1981). Phonological processes which characterize unintelligible and intelligible speech in early childhood. Journal of Speech and Hearing Disorders, 46, 369–373.
- Joint Committee on Infant Hearing (JCIH). (2007). Joint Committee on Infant Hearing: Principles and guidelines for early hearing detection and intervention programs (Position Statement). Pediatrics, 120, 898–921.
- Kluwin, T., & Stewart, D. (2000). Cochlear implants for younger children: A preliminary description of the parental decision process and outcomes. American Annals of the Deaf, 145, 26–32.
- Locke, J. L. (1983). Phonological acquisition and change. New York: Academic Press.
- Madell, J., & Flexer, C. (2008). Pediatric audiology: Diagnosis, technology, and management. New York: Thieme Medical Publishers.
- May-Mederake, B. (2012). Early intervention and assessment of speech and language development in young children with cochlear implants. International Journal of Pediatric Otorhinolaryngology, 76, 939–946.
- McLeod, S. (1997). Sampling consonant clusters: Four procedures designed for Australian children. The Australian Communication Quarterly, Autumn, 9–12.
- McLeod, S. (2007). Australian English speech acquisition. In S. McLeod (Ed.), The international guide to speech acquisition (pp. 241–256). Clifton Park, NY: Thomson Delmar Learning.
- McLeod, S., & Arciuli, J. (2009). School-aged children's production of consonant clusters. Folia Phoniatrica et Logopaedica, 61, 336–341.
- McLeod, S., & Hand, L. (1991). Single Word Test of Consonant Clusters. Sydney: The University of Sydney. http://athene.riv.csu.edu.au/˜smcleod/Consonant
- McLeod, S., van Doorn, J., & Reed, V. (2001a). Normal acquisition of consonant clusters. American Journal of Speech-Language Pathology, 10, 99–110.
- McLeod, S., van Doorn, J., & Reed, V. A. (2001b). Consonant cluster development in two-year-olds: General trends and individual difference. Journal of Speech, Language, and Hearing Research, 44, 1144–1171.
- Moeller, M. P., Hoover, B., Putman, C., Arbataitis, K., Bohnenkamp, G., Peterson, B., et al. (2007). Vocalizations of infants with hearing loss compared with infants with normal hearing: Part I – Phonetic development. Ear and Hearing, 28, 605–627.
- NSW State Government Health. (2011). NSW Statewide Infant Screening - Hearing (SWISH) Program. http://www.health.nsw.gov.au/initiatives/swish/index.asp.
- Osberger, M. J., & McGarr, N. S. (1982). Speech production characteristics of the hearing impaired. In N. Lass (Ed.), Speech and language: Advances in basic research and practice (pp. 227–267). New York: Academic Press.
- Overby, M. S., Trainin, G., Smit, A. B., Bernthal, J. E., & Nelson, R. (2012). Preliteracy speech sound production skill and later literacy outcomes: A study using the templin archive. Language, Speech, and Hearing Services in Schools, 43, 97–115.
- Paul, R., & Shriberg, L. D. (1982). Associations between phonology and syntax in speech-delayed children. Journal of Speech and Hearing Research, 25, 536–547.
- Powell, T. W. (1995). A clinical screening procedure for assessing consonant cluster production. American Journal of Speech-Language Pathology, 4, 59–65.
- Priester, G., Post, W., & Goorhuis-Brouwer, S. (2011). Phonetic and phonemic acquisition: Normative data in English and Dutch speech sound development. International Journal of Pediatric Otorhinolaryngology, 75, 592–596.
- Sharma, A. (2010). Plasticity, development and reorganization of central auditory system in children with hearing impairment. Paper presented at the Beyond Newborn Hearing Screening Conference, Cernobbio Italy.
- Sininger, Y. S., Grimes, A., & Christensen, E. (2010). Auditory development in early amplified children: Factors influencing auditory-based communication outcomes in children with hearing loss. Ear and Hearing, 31, 166–185.
- Smit, A., Hand, L., Freilinger, J., Bernthal, J., & Bird, A. (1990). The Iowa articulation norms project and its Nebraska replication. Journal of Speech and Hearing Disorders, 55, 779–798.
- Smit, A. B. (1993). Phonologic error distribution in the Iowa-Nebraska articulation norms project: Word-initial consonant clusters. Journal of Speech and Hearing Research, 36, 931.
- Van Kleeck, A., Schwarz, A. L., Fey, M., Kaiser, A., Miller, J., & Weitzman, E. (2010). Should we use telegraphic or grammatical input in the early stages of language development with children who have language impairments? A meta-analysis of the research and expert opinion. American Journal of Speech-Language Pathology, 19, 3–21.
- Waring, R., Fisher, J., & Aitkin, N. (2001). The articulation survey: Putting numbers to it. In L. Wilson, & S. Hewat (Eds.), Proceedings of the 2001 Speech Pathology Australia national conference: Evidence and innovation (pp. 145–151). Melbourne: Speech Pathology Australia.
- Wie, O. B. (2010). Language development in children after receiving bilateral cochlear implants between 5 and 18 months. International Journal of Pediatric Otorhinolaryngology, 74, 1258–1266.
- Yoshinaga-Itano, C. (2003). Universal newborn hearing screening programs and developmental outcomes. Audiological Medicine, 1, 199–206.
- Yoshinaga-Itano, C. (2011). Achieving optimal outcomes from EHDI. ASHA Leader Live, Early Intervention, September, 1–45.
- Yoshinaga-Itano, C., Coulter, D., & Thomson, V. (2001). Developmental outcomes of children with hearing loss born in Colorado hospitals with and without universal newborn hearing screening programs. Seminars in Neonatology, 6, 521–529.
- Zimmerman, I. L., Steiner, V. G., & Pond, R. E. (2002). Preschool Language Scales (4th ed.). San Antonio, TX: Psychological Corporation.