Abstract
The aim of this study was to compare the rate of sperm DNA fragmentation (rSDF; increase of SDF over time) in fresh and gradient isolated frozen–thawed semen samples from male sperm donors of proven fertility. SDF was assessed in the two samples obtained from the same fifteen male donors after 0.5, 1.5, 4.5, 6, 24, 48, and 72 h of incubation in a humidified atmosphere of 5% CO2 in air at 37°C. Analysis was performed based on chromatin dispersion patterns evaluated using the Halosperm® kit. No significant differences in SDF were obtained when fresh and gradient-isolated frozen–thawed spermatozoa were compared at baseline. However, the rSDF shown by the two samples differed and gradient-isolation selected for sperm subpopulations showing a lower variance for SDF. At the individual level, sperm selection by density gradient purification in frozen–thawed samples did not improve the levels of SDF when compared with the values obtained in fresh samples at baseline.
Abbreviations | ||
rSDF: | = | rate of sperm DNA fragmentation |
SDF: | = | sperm DNA fragmentation |
ARTs: | = | assisted reproduction techniques |
IVF: | = | in vitro fertilization |
DGC: | = | density gradient-centrifugation |
SCD: | = | sperm chromatin dispersion |
T0: | = | time zero |
ROS: | = | reactive oxygen species |
INTRODUCTION
Some damaged DNA is present in mature human spermatozoa after ejaculation. However, sperm preparation for assisted reproduction techniques (ARTs) can increase the basal rate of DNA damage and impair other semen parameters [Donnelly et al. Citation2001; Dalzell et al. Citation2003; López-Fernández et al. Citation2007] with a subsequent reduction in fertility rates [Word et al. Citation2003]. Unfortunately, the mechanisms involved in sperm DNA fragmentation (SDF) in freshly ejaculated or processed samples are not clear [Jaattela Citation2002; Agarwal and Allamaneni Citation2005]. However, for many mammalian species it is known that the rate of sperm DNA fragmentation (rSDF) increases when the cells are exposed to temperature variations [Gosálvez et al. Citation2007; López-Fernández et al. Citation2007; Cortés-Gutiérrez et al. Citation2008]. Thus, the rSDF tends to increase after a period of incubation (usually hours) at 37°C in an atmosphere with a low concentration of CO2 [Gosálvez et al. Citation2008b]. The rSDF also varies between species and among different individuals within the same species [Gosálvez et al. Citation2007]. In human donors of proven fertility the highest rSDF is observed in the first 4–6 h of incubation at 37°C under in vitro fertilization (IVF) conditions. This generates a mean rSDF of about 8% of affected sperm nuclei per h [Gosálvez et al. Citation2008a].
Important factors affecting sperm cryopreservation are cooling, freezing, thawing, and the composition of cryoprotectants in the freezing extender [Holt and Medrano Citation1997; Donnelly et al. Citation2001]. It has been suggested that sperm DNA damage could be a consequence of the freeze–thaw process [Royere et al. Citation1988]. However, a direct relationship between these factors is not clearly established. For human spermatozoa, it has been demonstrated that freeze–thawing of fractionated samples is associated with plasmalemmal phosphatidylserine externalization, but not with fragmented DNA [Duru et al. Citation2001]. Although in most mammalian species the basal level of SDF after thawing does not increase when compared with that observed before freezing, the rSDF after thawing tends to be higher [López-Fernández et al. Citation2007; Citation2008]. In general, sperm motility, viability, and most motion characteristics are significantly affected in samples prepared by ‘swim-up’ [Esteves et al. Citation2000] or gradient-isolation techniques [McCann and Chantler Citation2002; Edelstein et al. Citation2008]. For example, swim-up isolates sperm with a greater ability to undergo hypo-osmotic swelling, but does not change the rate of spontaneous acrosome reaction, even though in vitro capacitation of spermatozoa selected by swim-up enhances the acrosome reaction rate [Esteves et al. Citation2000; Zaneveld et al. Citation1991]. Sperm morphology measures also improved significantly after preparation using swim-up strategies [Elberger et al. Citation2006].
The aim of this investigation was to analyze the rSDF in spermatozoa from donors with proven fertility, using an experimental model that emulated the steps of sperm handling as used for ART, comparing fresh sperm samples with frozen–thawed samples plus density gradient-centrifugation (DGC). The most parsimonious hypothesis to test was that the SDF values would be affected as a consequence of iatrogenic sperm damage produced after ex vivo sperm manipulation [Twigg et al. Citation1998]. A dynamic approach to measuring SDF over time in culture was used to assess this hypothesis.
RESULTS
SDF Dynamics in the Analyzed Sample
Gel Red staining provided high resolution images in the sperm chromatin dispersion (SCD) test. There were differences in the level of SDF between non-incubated sperm samples (fresh or DGC at baseline, ) and those incubated for some h. shows a sample analyzed at baseline time zero (T0), where most of the sperm heads exhibit the chromatin dispersion halo and contain putative normal DNA that expanded in the SCD test conditions. Heads with small or no halos suggests spermatozoa with fragmented DNA. shows a sample analyzed after 24 h of incubation, where the frequency of sperm nuclei displaying halos was reduced notably. It is interesting to stress that the combination of DNA denaturation plus protein depletion produce massive protamine removal while leaving other proteins such as those forming the flagellum relatively intact.
FIGURE 1 Sperm DNA fragmentation as visualized after application of the SCD test and staining using Gel Red. (a) Fresh sperm sample processed at baseline. Yellow arrows highlight sperm nuclei containing damaged DNA. (b) Fresh sperm sample after 24 h incubation. Green arrows show highly degraded sperm nuclei.
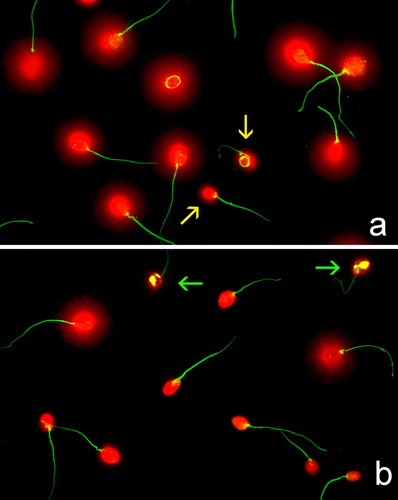
We started by checking baseline SDF in every donor, using fresh and DGC samples. Basal indices ranged from 8% to 49% and from 9% to 42%, respectively. SDF dynamics were analyzed for each donor, by quantifying SDF in both treatment groups over time. summarizes the results showing an increase in SDF with time. This was observed in every individual, irrespective of treatment (either fresh or DGC). However, differences were observed in the rSDF among individuals and between sample treatments. For example, for fresh samples (black lines in ) there was a steady increase in time for some donors, whereas in others there was little increase during the first 4–6 h but then a sudden rise. DGC semen samples (red lines in ) showed much more homogeneous SDF distributions over time than the fresh samples (black lines).
FIGURE 2 Graphic representation of different aspects of the dynamics of sperm DNA fragmentation. (a) Each line reflects SDF dynamics for one out of 15 donors. SDF was quantified in both fresh (black lines) and gradient-isolated (red lines) semen samples at time 0 and after 0.5, 1.5, 4.5, 6, 24, 48, and 72 h incubation at 37°C. (b) Box-and-whisker plots used to compare SDF distributions between semen samples (fresh in black, gradient-isolated in red) over time. Note the larger dispersion of data in fresh samples. Both treatment distributions tend to disperse after 24 h. (c) Mean±standard error is plotted for both fresh (black) and gradient-isolated (red) at the various incubation times. Interpolated lines have been superimposed on the graph.
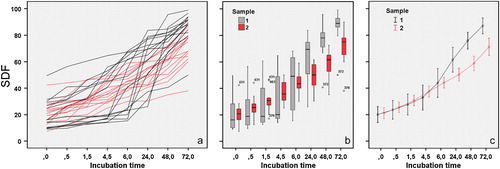
Box-and-whisker plots were used to compare the distribution of SDF data between semen samples over time (). There was a greater dispersion of SDF values in fresh than in DGC samples. Asymmetry and bias were common in most fresh sample distributions. In contrast, SDF data from the DGC samples tended to be more symmetrically distributed. The Kolmogorov–Smirnov test was used to check for normality. The distributions of the SDF values in the DGC samples (except for T1.5, P>0.05) approximated a normal distribution. In comparison, normality was rejected for most fresh sample distributions (apart from T24 and T48, P<0.05). We did not need to use arcsine transformation to normalize percentage data as most were in the range of 30–70%. Mann–Whitney U tests showed no significant difference between samples from T0 to T6 but statistically significant differences (P<0.05) were revealed from T24 on. As the fresh and DGC samples had been obtained from the same 15 donors, the Wilcoxon's signed rank test for paired data was also performed. In this case, significant differences between samples were not evident at T24 (P=0.078), but arose after T48 (P<0.05). shows the trend.
As mentioned above, differences in the patterns of SDF increase were apparent among individuals and between samples (fresh and DGC). To study these differences, we quantified the rSDF during two time intervals: from T0 to T6 (rSDF1) and from T6 to T24 (rSDF2, ). These times were selected because differences between fresh and DGC samples became evident from T24, but no differences were found up to T6. The rSDF1 ranged from 0.4 to 7.7% in fresh samples (mean±SE 3.98±1.96) and from 1.9 to 6.5% in DGC samples (3.83±0.63). (fresh samples) and (DGC samples) show the differences in rSDF1 among individuals and between semen samples. The lines in these figures join SDF values at T0 and after T6. We derived the rSDF1 value by subtracting these two SDF values and dividing by the elapsed time (6 h). Thus, differences in the slopes of the lines reflect differences in the rSDF1 among individuals within either fresh () or DGC () samples. There were greater differences in slopes in fresh than in DGC samples. Thus, although fresh and DGC samples showed similar rSDF1 values, the DGC samples appeared more homogeneous and predictable (see differences in standard errors and also for comparison of the distribution of rSDF1 values). The rSDF2 ranged from 0.1% to 3.1% per h in fresh samples (0.97±0.51% per h) and from 0.02 to 0.8% in DGC samples (0.34±0.14% per h). (fresh) and (DGC) highlight the differences in rSDF2 among individuals and between samples. In this case, the lines join SDF values at T6 and after T24. We derived the rSDF2 value by subtracting these two SDF values and dividing by the elapsed time (18h). Again, DGC seemed to render each individual's semen samples more predictable (see differences in standard errors and for comparison of the distribution of rSDF1/rSDF2 values).
FIGURE 3 Graphic representation for the rSDF. (a, b) Fresh samples. (c, d) Gradient-isolated samples. Two time intervals were used for comparison: (a, c) from 0 to 6 h (rSDF1) and (b, d) from 6 to 24 h (rSDF2). The mean±standard error is shown for each case. See text for a more detailed explanation. (e) Box-and-whisker plots used to compare rSDF1 and rSDF2 data distributions between semen samples. Note the larger data dispersion observed for fresh samples.
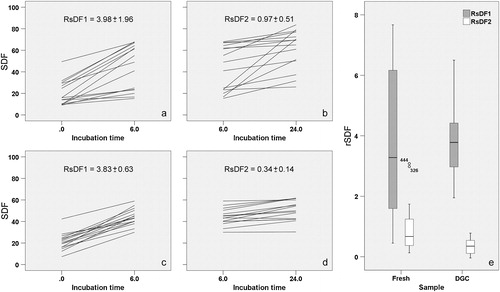
We checked for differences in the two rSDF values between treatments at the two time intervals (). Wilcoxon's signed rank test for paired data showed no differences in rSDF1 between treatments. However, we must stress the already mentioned larger dispersion of rSDF1 data in fresh samples. On the contrary, significant differences were found for rSDF2 between treatments (P<0.05); DGC samples showed lower rSDF2 values than fresh samples. We also compared rSDF1 with rSDF2 within samples. Significant differences were found in both fresh and DGC samples (P<0.01). Thus, a significant decrease in the rSDF was observed after T6 in both treatments, although this was more noticeable in the DGC samples.
We tested if the SDF index at T0 affected the rate of sperm DNA fragmentation. No association between SDF at T0 and rSDF1 was found for either treatment (ρ=0.29, P>0.05 for both samples). Likewise, no statistically significant correlation was found in rSDF between fresh and DGC for the two time intervals (ρ=0.07, P>0.05 for rSDF1; ρ=0.10, P>0.05 for rSDF2).
Individual SDF Dynamics
We tested whether DGC affected all individuals in the same manner. We looked for a consistent pattern at T0 by comparing basal SDF indexes in fresh and DGC semen samples. The present data do not seem to show a consistent pattern for the quality of DNA recovered after DGC. Thus, in some individuals (6 out of 15) SDF values for DGC samples were lower at T0 than those among fresh samples (), while in others (9 out of 15) the relationship was in the opposite direction (). However, the Spearman correlation analysis showed no significant association between fresh and DGC SDF indexes at T0 (ρ=0.15, P>0.05).
FIGURE 4 A graphic representation of the basal level of SDF and after 6 h of incubation for each donor. Continuous line: gradient-isolated sperm. Discontinuous line: fresh sperm. (a) Representation of the rSDF in six donors where the level of DNA damage after gradient isolation was lower than in fresh samples. (b) Representation of the rSDF in nine donors where the level of DNA damage after gradient isolation was higher than in fresh samples.
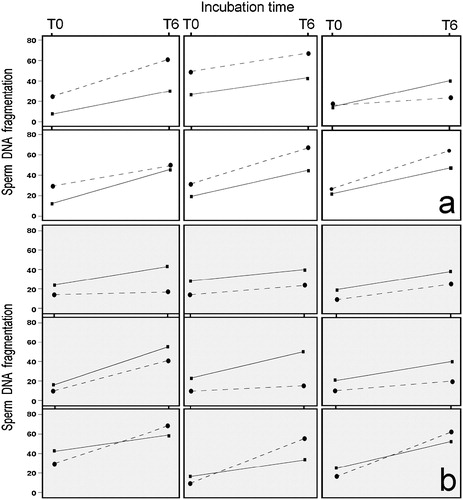
DISCUSSION
The present experiments were indicative of no significant effect at baseline on DNA fragmentation when fresh and DGC semen samples were compared after incubation in similar conditions. However, the SDF dynamics shown by the two types of samples differed. Density gradient selection of a sperm subpopulation tended to reduce the variance in SDF. Moreover, in some individual donors, DGC spermatozoa gave lower baseline SDF values than in the fresh samples; but in other individuals the level of damaged DNA after DGC was higher. Freeze–thawing plus gradient isolation seemed to render individual semen samples more predictable and stable in culture, although it did not always diminish the SDF.
The first point we wish to discuss is the putative negative effects that freeze–thawing might have on DNA integrity. This clarification is necessary given the ambiguous results found in the literature. Some have suggested that the highly stable DNA is fragmented by freeze–thawing [Royere et al. Citation1988; Sakkas et al. Citation1999; Hammadeh et al. Citation2001]. However, other authors have differed [Steele et al. Citation2000]. Duru et al. [Citation2001] concluded that freeze–thawing of human sperm samples was associated with membrane changes, as revealed by membrane translocation of phosphatidylserine, while having no major impact on DNA fragmentation. In fact, when sperm samples are analyzed for SDF immediately after freeze–thawing and compared with the values obtained in fresh semen samples, the differences are not dramatic, or are even nonexistent [López-Fernández et al. Citation2007; Citation2008]. In the present experiments, this tendency for low SDF values was also observed at baseline after freeze–thawing plus gradient isolation (DGC samples). Our impression, according to the results obtained from several mammalian species, is that the actual situation could be a mixture of both circumstances, and an effective increase in SDF is not detected when the sperm are analyzed rapidly after thawing. The presence of newly produced single-stranded and/or double-stranded DNA breaks, is not a direct consequence of low temperatures by themselves. Instead, freeze–thawing induces changes in the chromatin (DNA plus proteins) that trigger DNA's predisposition for fragmentation, and the effect is manifested later. The most parsimonious hypothesis to test is that an indirect effect is produced on the DNA because alterations in the natural DNA/nuclear protein interactions are caused by the freezing conditions. The outcome is that the rSDF after freeze–thawing is higher than that observed in fresh semen samples; this is true for most species [López-Fernández et al. Citation2008; Gosálvez et al. Citation2009]. Moreover, the membranes must be first affected [Duru et al. Citation2001] and later a trigger for DNA damage occurs suddenly. This could be one reason why sperm viability and sperm DNA fragmentation behave as independent parameters [Gosálvez et al. Citation2008a].
The second point is the benefit of gradient-isolation techniques after thawing for ART in the context of DNA fragmentation. In fact, the SDF dynamics that were examined differed between fresh and DGC samples and it seems that a strong selection was produced in the subpopulation separated by density gradient centrifugation after thawing. This selection tended to diminish the variance in the range of values observed when the rSDF of DGC sperm was compared with fresh samples. Interestingly, these results are similar to those obtained by Hammoud et al. [Citation2007] where density gradient preparation resulted in a reduction on intra-individual variation of sperm motility. Our results suggest that only certain sperm subpopulations were selected, but that they behaved in a similar fashion for rSDF. Gradients or swim-up separation techniques have been used routinely in processing spermatozoa mainly because the recovery, in terms of numbers of motile spermatozoa, spermatozoa with normal morphology, and acrosome-reacted spermatozoa could help predict fertilization success [Burkman Citation1984; Howard et al. Citation1993; Bielsa et al. Citation1994; Elberger et al. Citation2006]. The methodology likely selects sperm populations with better motility from poor quality semen samples. This is based on the notion that highly motile spermatozoa move actively in the direction of the sedimentation gradient and can therefore penetrate the boundary quicker than poorly motile or immotile cells. Using this rationale, one would expect highly motile sperm cells to be enriched in the soft pellet fraction. When examining the ability of gradients to isolate spermatozoa with normal chromatin structure, the percentage of sperm improved in the 90% fraction [Golan et al. Citation1997; Sakkas et al. Citation2000]. Moreover, spermatozoa with more compacted chromatin are more likely to be present in the 90% fraction. In those studies, such gradients appeared to enrich for spermatozoa with less intermediate nucleoprotein content and more mature nucleoproteins of the protamine 2 family, a feature not observed with the swim-up separation technique.
In cats, a significant finding was that swim-up spermatozoa from teratozoospermic ejaculates were still incapable of penetrating the zona pellucida compared with spermatozoa from normospermic animals. When morphologically normal swim-up spermatozoa from both normospermic and teratospermic males were compared, the level of tyrosine phosphorylated protein was significantly lower in teratospermic samples. This result suggests that diminished phosphorylation present in the sperm from teratospermic forms may compromise capacitation and the ability to undergo an acrosome reaction [Pukazhenthi et al. Citation1996]. The effect of in vitro capacitation on the acrosomal enzymes of human spermatozoa was assessed in human spermatozoa by testing their ability to penetrate denuded hamster oocytes [Mack et al. Citation1983]. Interestingly, although no alteration in enzyme activity occurred after 4 h of incubation, some enzymes such as acid phosphatase decreased twofold after 8 h of capacitation and small decreases were noted in acrosin and proacrosin. This change in the level of enzyme activity might parallel the dynamic behavior of DNA fragmentation observed in the present study. Unfortunately, these physiological changes will not be obvious when using conventional techniques for sperm selection in ART. Together, these findings suggest that a certain level of synchronicity affects different programmed processes related to sperm capacitation before sperm death.
Under the experimental conditions used in this trial, there was no difference in the rSDF1 between fresh and DGC samples. However, there was a larger variance for the fresh samples. By contrast, DGC samples showed a lower rSDF2 than fresh samples. When rSDF1 was compared with rSDF2, significant differences were observed in both fresh and DGC samples. Thus, a significant decrease in the rSDF was observed after 6 h in both semen samples, though this was more noticeable in the DGC samples. Thus, after gradient-isolation, the factors associated with DNA damage were less active, probably because the sperm debris resulting from a normal ejaculation, which is inherent to each individual, was removed. A potential cause for the observed differences could arise from oxidative stress associated with the generation of reactive oxygen species (ROS) [Aitken and Clarkson Citation1987]. In fact, decreased temperature leads to an increase in ROS in spermatozoa [Wang et al. Citation1997]. However, this does not explain the reduced variance for DNA damage observed after gradient isolation in the present experiment. If ROS are the stressing factors, after gradient isolation ROS effects on DNA would be lower than that acting before. However, the problem of sperm DNA damage cannot be reduced to such a simplistic interpretation and, in addition to the cell damage produced by variable levels of ROS, induction of damage to sperm membranes by lipid peroxidation [Aitken and Clarkson Citation1988; Sikka et al. Citation1995] must also be considered. In addition to the release of acrosomal enzymes, which might act negatively on chromatin structure once the sperm membranes have been affected, this perturbation of membrane structure could in turn disrupt several cellular mechanisms including ion transport and signal transduction pathway(s) [Sikka et al. Citation1995].
Finally, it should be stressed that DNA fragmentation is an important cause of the rapid decline of sperm quality, as sperm DNA tends to degrade very quickly after thawing. Although gradient isolation tends to select some sperm subpopulations, the tendency for DNA damage is still present. Sperm DNA exhibitied high rates of degradation in DGC and freshly ejaculated sperm samples. In fact, given the high rate for SDF observed during the first 4–6 h of incubation, it seems highly probable that any sperm sample would contain 50% of sperm cells with damaged DNA after that period of temperature recovery. Additionally, this investigation has shown that different sperm samples exhibit different sperm DNA fragmentation dynamics. This tendency for inter-individual variation has also been reported in human [Gosálvez et al. Citation2009] and in other mammalian species such as horse [López-Fernández et al. Citation2007] and donkey [Cortés-Gutiérrez et al. Citation2008], which also exhibit rapid losses in sperm DNA quality. At the individual level, it is noteworthy that SDF recovered after gradient isolation of frozen–thawed samples could be improved with respect to that obtained in fresh samples in some cases. This is not the general rule. The fact that no correlation was found between SDF at T0 in fresh and DGC samples is in accord with the results obtained by others where discordances are found between SCSA/DFI analysis on net semen (equivalent to T0 in our experiment) and DGC samples and their respective predictive values of pregnancy outcome [Bungum et al. Citation2008]. Thus, the next question that should be addressed is: how is fertility affected when a decrease in the DNA quality is caused by the detrimental effects produced via sperm handling?
This dynamic analysis of sperm DNA fragmentation, unlike the static conception of DNA damage, opens the possibility of a more personalized semen assessment for each patient. In ART, it is important to select the most efficient semen samples by minimizing the severity of DNA damage. This can be aided, for example, by being scientifically conscientious of the timing for using the sperm samples according to the dynamic changes that each semen sample presents. We therefore recommend that chromatin changes through time should be used as an additional parameter for the assessment of sperm quality after freeze–thawing or in every circumstance of semen handling for ART. This could be an additional viewpoint of semen quality assessment, which would be independent of the static value usually reported for each patient. Our results emphasize that if care is taken not to cause damage to the genetic component of the spermatozoa during semen handling the outcome of ART should be improved. We must be aware that as time elapses after sample analysis the frequency of sperm cells with fragmented DNA at the moment that the ART procedure is carried out could have increased significantly.
MATERIALS AND METHODS
Fifteen donors of proven fertility were included in this study at the Tambre Clinic (Madrid, Spain). Their semen samples were used in different ART programs. Semen specimens from each donor were divided into two aliquots. One was used as a fresh semen sample while the other was cryopreserved. For crypreservation a modified method of Nagase and Niwa [Citation1964] adapted to human spermatozoa was used [Nuñez-Calonge et al. Citation2007]. An equal volume of cryoprotector solution (Criosperm: TRES-TRIS egg yolk/7% glycerol) was added to the net sperm (volume/volume); the resulting solution was gently mixed and kept at 4°C for 45 min. After the refrigeration, 100 microliter drops were prepared and then placed on dry ice for 2 min. Each frozen drop was then placed in a 1.0 ml vial and then plugged in liquid nitrogen.
Frozen samples were thawed by immersion in a 37°C water bath for 30 s and then diluted to 10–15×106 spermatozoa/mL in IVF medium (MediCult, Jyllinge, Denmark). Fresh semen samples were diluted to the same concentration. Frozen-thawed aliquots were put through a two-step discontinuous density gradient separation in order to prepare DGC samples. Briefly, a 1 mL aliquot was gently overlaid on a column consisting of 1 mL of 45% and 1 mL of 90% density gradient material (Sperm grad-TM™, Vitrolife, Goteborg, Sweden) in a 15 mL conical tube, and centrifuged at 300×g for 15 min at room temperature. The pellets were washed once with 5 mL of Sperm Prep Medium (Medi-Cult, Jyllinge, Denmark), centrifuged at 300×g for 8 min and then resuspended in 0.5 mL of IVF medium (Medi-Cult, Jyllinge, Denmark) as in the washing step. The samples were then assessed under a phase contrast microscope for concentration and motility using a Makler chamber (Sefi Medical Instruments, Haifa, Israel). Two aliquots of fresh and DGC samples from the same individual were incubated in a humidified atmosphere of 5% CO2 in air at 37°C. The SDF index, the ratio of fragmented versus total spermatozoa in the analyzed sample, expressed as a percentage, was assessed at time 0 (baseline) and after 0.5, 1.5, 4.5, 6, 24, 48, and 72 h incubation in IVF medium (Medi-Cult, Jyllinge, Denmark).
The SDF was determined from chromatin dispersion patterns using the Halosperm® kit (Halotech DNA SL, Madrid, Spain; Conception Technologies, San Diego, CA, USA). According to the manufacturer, controlled denaturation of the DNA followed by the extraction of the nuclear proteins, gives rise to partially deproteinized nucleoids in which the DNA loops expand, forming halos of chromatin dispersion. However, the spermatozoa nucleoids whose DNA is fragmented, either do not develop a dispersion halo or the halo is minimal. The induction of DNA denaturation plus protein depletion, as used in the Halosperm protocol, differ from the protocols used for assessing DNA fragmentation in other experimental environments such as comets or chromatin diffusion tests resulting in the resolution of sperm nuclear matrix. The use of mild acid DNA denaturation enhances protein removal, thus producing massive protamine removal while leaving other proteins such as those forming the flagellum relatively intact. DNA halos from the SCD test were visualized by fluorescence microscopy after being stained with the DNA intercalating fluorochrome Gel Red (Biotium, Hayward, CA, USA; 10× in anti-fading Vectashield, Vector Laboratories, Burlingame, CA, USA) and scored automatically using a prototype sperm class analyzer/computer-assisted semen analysis system (SCA-CASA, Microptic SL., Barcelona, Spain). Gel Red provides quick staining with low levels of fading. Additionally, given its selectivity and efficiency in binding DNA, this fluorochrome produces a low level of background. This improves the efficiency of the CASA system for image interpretation. The SCA–CASA system discriminates spermatozoa that show a small and peripheral halo of chromatin dispersion (DNA fragmentation) from those exhibiting a large halo (normal DNA, ). This software was coupled to a Leica DMLA model motorized fluorescence microscope controlled with software for automatic scanning and image digitization (Leica Microsystems, Barcelona, Spain). A Leica EL6000 fluorescence light source equipped with a metal halide lamp and plan-Fluotar 40×lenses was used. Routinely each sample was subjected to double staining for simultaneous flagellum and DNA identification. This approach serves as an internal control to differentiate possible somatic cells from sperm cells. The double staining protocol used Gel Red for DNA and 2,7-dibrom-4-hydroxy-mercury-fluorescein for specific protein staining (Sigma-Aldrich, Barcelona, Spain). A charge-coupled device camera (Leica DFC350 FX, Leica Microsystems) with two independent green and red filters was used for image capture and storage as grey level TIFF files. Files were merged using Adobe-Photoshop CS3 (Adobe Systems Inc., Mountain View, CA, USA). A minimum of 800 spermatozoa was scored per sample. The percentage of spermatozoa with fragmented DNA based on the presence of small halo or the absence of halo was then calculated.
Statistical Analysis
SPSS v.15.0 for Windows (SPSS Inc., Chicago, IL, USA) was used for statistical analysis and graphics. Box-and-whisker plots were used to compare the distribution of SDF data between semen samples over time. The Kolmogorov–Smirnov test was used to check for normality of distribution. Differences between fresh and DGC samples were analyzed at the various incubation times, and evaluated using the non-parametric Mann–Whitney U test and Wilcoxon's signed rank test for paired data. Spearman's correlation coefficient was used to test associations between variables and P<0.05 was considered statistically significant.
ACKNOWLEDGMENTS
This work was supported by BFU 2007-66340/BFI (Ministerio de Educación y Ciencia, Spain).
Declaration of Interest: The authors report no conflicts of interest. The authors alone are responsible for the content and writing of the paper.
REFERENCES
- Agarwal, A. and Allamaneni, S. S. R. (2005) Alteraciones de la cromatina espermática en la etiopatogenia de la infertilidad masculina. Rev Int Androl 3:31–37.
- Aitken, R. J. and Clarkson, J. S. (1987) Cellular basis of defective sperm function and its association with the genesis of reactive oxygen species by human spermatozoa. J Reprod Fertil 81:459–469.
- Aitken, R. J. and Clarkson, J. S. (1988) Significance of reactive oxygen species and antioxidants in defining the efficacy of sperm preparation techniques. J Androl 9:367–376.
- Bielsa, M. A., Andolz, P., Gris, J. M., Martínez, P. and Egozcue J. (1994) Andrology: Which semen parameters have a predictive value for pregnancy in infertile couples? Hum Reprod 9:1887–1890.
- Bungum, M., Spano, M., Humaidan, P., Eleuteri, P., Rescia, M. and Giwercman, A. (2008) Sperm chromatin structure assay parameters measured after density gradient centrifugation are not predictive for the outcome of ART. Hum Reprod 23:4–10.
- Burkman, L. J. (1984) Characterization of hyperactivated motility by human spermatozoa during capacitation: comparison of fertile and oligozoospermic sperm population. Arch Androl 13:153–165.
- Cortés-Gutiérrez, E. I, Crespo, F., Gosálvez, A., Dávila-Rodríguez, M. I., López-Fernández, C. and Gósalvez, J. (2008) DNA fragmentation in frozen sperm of Equus asinus: Zamorano-Leonés, a breed at risk of extinction. Theriogenology 69:1022–1032.
- Dalzell, L. H., Thompson-Cree, M., McClure, N., Traub, A. I. and Lewis, S. E. M. (2003) Effects of 24-hour incubation after freeze-thawing on DNA fragmentation of testicular sperm from infertile and fertile men. Fertil Steril 79:1670–1672.
- Donnelly, E. T., Mcclure, N. and Lewis S. E. M. (2001) Cryopreservation of human semen and prepared sperm: effects on motility parameters and DNA integrity. Fertil Steril 76:892–900.
- Duru, N. K., Morshedi, M. S., Schuffner, A. and Oehninger, S. (2001) Cryopreservation-Thawing of fractionated human spermatozoa is associated with membrane phosphatidylserine externalization and not DNA fragmentation. J Androl 22:646–651.
- Edelstein, A., Yavetz, H., Kleiman, Hauser, R., Botchan, A., Paz, G. and Yogev, L. (2008) Effect of long-term storage on deoxyribonucleic acid damage and motility of sperm bank donor specimens. Fertil Steril 90:1327–1330.
- Elberger, L., Grünwaldt, M. M., Divita, A. E., Arenas, G., Buxeda, P. S. and Polak de Fried, E. S. (2006) Sperm morphology before and after capacitation. Fertil Steril 86:S508.
- Esteves, S. C, Sharma, R. K., Thomas, A. J. and Agarwal, A. (2000) Effect of swim-up sperm washing and subsequent capacitation on acrosome status and functional membrane integrity of normal sperm. Int J Fertil Womens Med 45:335–341.
- Golan, R., Schochat, L. and Weissenberg, R. (1997) Evaluation of chromatin condensation in human spermatozoa: a flow cytometric assay using acridine orange satining. Molecular Hum Reprod 14:1039–1049.
- Gosálvez, J., Cortés-Gutierrez, E., López-Fernández, C., Fernández, J. L., Nuñez, R. and Caballero, P. (2009) Sperm deoxyribonucleic acid fragmentation dynamics in fertile donors. Fertil Steril 92:170–173.
- Gosálvez, J., Fernández, J. L., Gosálbez, A., Arroyo F., Agarwal, A. and López-Fernández, C. (2007) Dynamics of sperm DNA fragmentation in mammalian species as assessed by the SCD methodology. Fertil Steril 88:S365.
- Gosálvez, J., Cortés-Gutierrez, E., Nuñez, R., Fernández, J. L., Caballero, P., López-Fernández, C. and Holt, W. V. (2008a) A dynamic assessment of sperm DNA fragmentation versus sperm viability in proven fertile human donors. Fertil Steril (in press). doi:10.1016/j.fertnstert.2008.08.136
- Gosálvez, J., Nuñez, R., Caballero, P., Fernandez, J. L., Cortés-Gutierrez, E. and López-Fernández, C. (2008b) Fragmentación del ADN espermático, ¿un concepto estático o dinámico? Revista Iberoamericana de Fertilidad 25:195–206.
- Hammadeh, M. E., Dehn, C. H., Hippach, M., Zeginiadou, T., Stieber, M., Georg, T., Rosenbaum, P. and Schmidt, W. (2001) Comparison between computerized slow-stage and static liquid nitrogen vapour freezing methods with respect to the deleterious effect on chromatin and morphology of spermatozoa from fertile and subfertile men. Int J Androl 24:66–72.
- Hammoud, A. O., Gibson, M., Peterson, C. M. and Carrell, D. T. (2007) Effect of sperm preparation techniques by density gradient on intra-individual variation of sperm motility. Arch Androl 53:349–351.
- Holt, W. V. and Medrano, J. A. (1997) Assessment of boar sperm function in relation to freezing and storage. J Reprod Fertil Suppl 52:213–222.
- Howard, I. G., Donoghue, A. M., Johnston, L. A. and Wildt, D. E. (1993) Zona pellucida filtration of structurally abnormal spermatozoa and reduced fertilization in teratospermic cats. Biol Reprod 49:131–139.
- Jaattela, M. (2002) Programmed cell death: many ways for cells to die decently. Ann Med 34:480–488.
- López-Fernández, C., Crespo, F., Arroyo, F., Fernández, J. L., Arana, P., Johnston, S. D. and Gosálvez, J. (2007) Dynamics of sperm DNA fragmentation in domestic animals II. The stallion. Theriogenology 68:1240–1250.
- López-Fernández, C., Fernández, J. L., Gosálbez, A., Arroyo, F., Vázquez, J. M., Holt, W. V. and Gosálvez, J. (2008) Dynamics of sperm DNA fragmentation in domestic animals: III. Ram. Theriogenology 708:98–908.
- Mack, S., Bhattacharyya, A. K., Joyce, C., van der Ven, H. and Zaneveld, L. J. (1983) Acrosomal enzymes of human spermatozoa before and after in vitro capacitation. Biol Reprod 28:1032–1042.
- McCann, C. T. and Chantler, E. (2002) Properties of sperm separated using Percoll and IxaPrep density gradients. A comparison made using CASA, longevity, morphology and the acrosome reaction. Int J Androl 23:205–209.
- Nagase, H. and Niwa, T. (1964) Deep freezing of bull semen in concentrated pellet form. I. Factors affecting survival of spermatozoa. In V.I.C.A.R. Trento, Italy Vol 4, pp 410–415.
- Nuñez-Calonge, R., Cortes, S., Peramo, B. and Caballero, P. (2007) Influence of incubation of frozen-thawed testicular immotile sperm on the outcome of intracytoplasmatic sperm injection in non-obstructive azoospermic patients. Fertil Steril 88:S1360.
- Pukazhenthi, B. S., Wildt, D. E., Ottinger, M. A. and Howard, J. (1996) Compromised sperm protein phosphorylation after capacitation, swim-up, and zona pellucida exposure in teratospermic domestic cats. J Androl 17:409–419.
- Royere, D., Hamamah, S., Nicolle, J. C., Barthelemy, C. and Lansac, J. (1988) Freezing and thawing alter chromatin stability of ejaculated human spermatozoa: fluorescence acridine orange staining and Feulgen-DNA cytophotometric studies. Gamete Research 21:51–57.
- Sakkas, D., Manicardi, G. C., Tomlinson, M., Mandrioli, M., Bizzaro, D., Bianchi, P. G. and Bianchi, U. (2000). The use of two density gradient centrifugation techniques and the swim-up method to separate spermatozoa with chromatin and nuclear DNA anomalies. Hum Reprod 5:1112–1116.
- Sakkas, D., Mariethoz, E., Manicardi, G., Bizzarro, D., Bianchi, P. G. and Bianchi, U. (1999) Origin of DNA damage in ejaculated spermatozoa. Rev Reprod 4:31–37.
- Sikka, S. C., Rajasekaran, M. and Hellstrom, W. J. (1995) Role of oxidative stress and antioxidants in male infertility. J Androl 16:464–481.
- Steele, E. K., McClure, N. and Lewis, S. E. M. (2000) Comparison of the effects of two methods of cryopreservation on testicular sperm DNA. Fertil Steril 74:450–453.
- Twigg, J., Irvine D. S., Houston P., Fulton N., Michael L. and Aitken, R. J. (1998) Iatrogenic DNA damage induced in human spermatozoa during sperm preparation: protective significance of seminal plasma. Mol Hum Reprod 4:439–445.
- Wang, A. W., Zhang, H., Ikemoto, I., Anderson, D. J. and Loughlin, K. R. (1997) Reactive Oxygen Species Generation by Seminal Cells During Cryopreservation. Urology 49:921–925.
- Wood, S., Sephton, V., Searle, T., Thomas, K., Schnauffer, K., Troup, S., Kingsland, C. and Lewis-Jones, I. (2003) Effect on clinical outcome of the interval between collection of epididymal and testicular spermatozoa and intracytoplasmic sperm injection in obstructive azoospermia. J Androl 24:67–72.
- Yanagimachi, R. (1994) Mammalian fertilization. In: The Physiology of Reproduction, Knobil, E. and Neill, J. D. ( Eds), New-York, NY: Raven Press Ltd; pp. 189–317.
- Zaneveld, L. J. D., De Jong, C. J., Anderson, R. A. and Mack, S. R. (1991) Human sperm capacitation and the acrosome reaction. Hum Reprod 6:1265–1274.
- Zilli, L., Schiavonea, R., Zonnob, V., Storellia, C. and Vilellaa, S. (2003) Evaluation of DNA damage in Dicentrarchus labrax sperm following cryopreservation. Cryobiology 47:227–235.