Abstract
Prostasomes, vesicles present in human semen, are known to play a role in male fertility. However, the mechanisms involved are still poorly understood. The present study looks at the direct influence of different concentrations of prostasomes on human sperm function in conditions supporting capacitation in vitro. Five million Percoll selected spermatozoa were incubated for 3 h at 370C under an atmosphere of 5% CO2 in air, in 100 µl Biggers Whitten Whittingham's medium (BWW) containing polyvinyl alcohol (PVA, 1 mg/ml) and bovine serum albumin (BSA; 3 mg/ml) in the absence or presence of increasing concentrations of prostasomes (expressed in terms of their cholesterol content: 15; 30; 45 nmoles per 100 µL of incubation medium). After in vitro exposure of spermatozoa to prostasomes, our data indicate that i) tyrosine phosphorylation intensity of the 107 KDa protein band was dose dependently lower and ii) the percentage of viable and progressive motile spermatozoa was unchanged and the percentage of non-progressive motility decreased. In addition, the incubation of prostasomes with spermatozoa resulted in an enrichment of their lipid content. Our experiments suggest that adhesion of prostasomes to spermatozoa could be responsible for the decrease in Tyrosine phosphorylation and the alteration of the mean curvilinear velocity (VCL) and the average path velocity (VAP).
Introduction
Prostasomes are small membrane-bound vesicles produced by the prostate gland and found in human seminal plasma. Their lipid composition is peculiar: cholesterol (Chol) and sphingomyelin (SM) are present in high amounts whereas phosphatidylcholine (PC) is less abundant [Arvidson et al. Citation1989; Arienti et al. Citation1998a]. They have an unusually high Chol to phospholipid ratio compared to that of human spermatozoa (1.7 versus 0.42) [Saez et al. Citation2000; Force et al. Citation2001]. Prostasomes have a range of small molecules (GDP, ADP, and ATP) and ions (Ca2+ and Zn2+) and different proteins including enzymes, transport structural proteins, and signal transduction proteins [Utleg et al. Citation2003]. Recently, it has been demonstrated that human prostasomes contain chromosomal DNA [Ronquist et al. Citation2009].
Although these particles have been described previously [Ronquist et al. Citation1978], their specific physiological roles, especially their relevance to reproductive function, are still poorly understood [Burden et al. Citation2006]. However, there is now a widespread consensus that prostasomes directly influence sperm function. Indeed, a pH-dependent, protein-dependent fusion between prostasomes and spermatozoa has been described which occurs at acidic pH 4-5 but not at pH 7.5 [Arienti et al. Citation1997]. Yet ejaculates with pH values > 7 allow an adhesion but no fusion of prostasomes to spermatozoa [Ronquist et al. Citation1990]. By such an interaction, prostasomes would directly influence spermatozoa behavior and protect them in the ejaculate. For instance, it has been suggested that the presence of prostasomes prevents premature acrosome reaction by delivering their Chol content to spermatozoa [Arienti et al. Citation1998a]. In addition, prostasomes may be considered as a system for counteracting the negative effect of acidic pH medium on sperm motility [Arienti et al. Citation1999] and they promote progressive motility of spermatozoa rendered immotile by buffer washing [Fabiani et al. Citation1994]. Also, Siciliano et al. (2008) demonstrated that prostasome-like vesicles stimulate the acrosome reaction of boar spermatozoa. These findings led to the hypothesis of a transfer of molecules from vesicles to membrane sperm [Siciliano et al. Citation2008].
Protein tyrosine phosphorylation is, by far, the most extensively studied type of post-translational modification in spermatozoa. This type of protein modification has been associated with some aspects of human spermatozoa functions such as capacitation and motility [Visconti et al. 1998]. Indeed, the increase in protein tyrosine phosphorylation occurring during sperm capacitation is well documented. Among tyrosine-phosphorylated proteins, we have previously demonstrated a strong correlation between tyrosine phosphorylation intensity of a 107 KDa protein band and the percentage of live acrosome reacted human spermatozoa in response to the A23187 calcium ionophore [Picherit-Marchenay et al. Citation2004].
The present study was undertaken to determine, in vitro, the role of prostasomes on the tyrosine phosphorylation of the 107 kDa protein and on some parameters of sperm quality (motility and motion). Additionally, lipid analysis was undertaken to evaluate a possible interaction of prostasomes with spermatozoa.
Results
The phosphorylation intensity of a 107 KDa protein band is diminished by prostasomes
After incubation of spermatozoa under capacitating conditions, an increase in tyrosine phosphorylation pattern was observed with the 107 KDa band identified as the main phosphotyrosine containing protein. The incubation of spermatozoa with different concentrations of prostasomes led to a decrease of the tyrosine phosphorylation pattern and most specifically to a decrease of the 107 KDa protein band (a). This effect was found significant with 300 µM and 450 µM (b). Indeed, prostasomes at a concentration of 300 µM significantly inhibit, by 56%, the phosphorylation of the 107 KDa protein band. This effect was enhanced with a higher concentration of prostasomes (74% of inhibition observed with 450 µM).
Figure 1. Inhibition of the 107 KDa protein band phosphorylation (on tyrosine residues) by different concentrations of prostasomes. Western blot analysis indicates that incubation of spermatozoa with increasing concentrations of prostasomes induces a dose-dependent inhibition of tyrosine phosphorylation of the 107 KDa protein band (Fig. 1a). The lane NI SPZ corresponds to non-incubated spermatozoa therefore non-capacitated spermatozoa. MW stands for molecular weigh markers and SPZ for spermatozoa. The 107 KDa protein band was quantified by densitometry (Fig. 1b) and the results expressed as % of control (spermatozoa incubated without prostasomes). A significant effect was observed with 300 μM and 450 μM of prostasomes. In figure 1b, the values are means ± SEM, n = 5. *significantly different from control (SPZ + 0 μM prostasomes); p < 0.05.
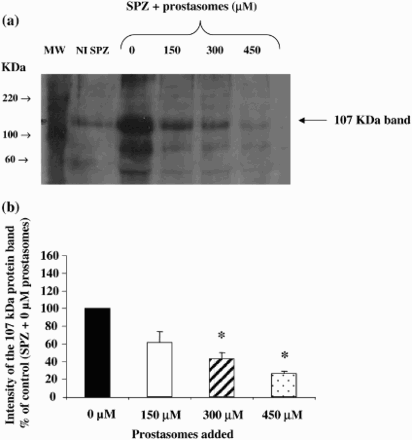
Effect of prostasomes on human sperm motility and motion parameters
The proportion of progressive motile spermatozoa was not significantly modified by any concentration of prostasomes tested (150, 300, and 450 µM) (). However, all concentrations of prostasomes used induced a significant decline in the proportion of non-progressive motile spermatozoa compared with a control (p < 0.05). This effect was significantly accentuated at a prostasome concentration of 300 µM (p < 0.05 compared with 150 µM). For all the concentrations of prostasomes tested, the decrease in the proportion of the non progressive motile spermatozoa was accompanied by a significant increase in the proportion of immotile spermatozoa. Addition of prostasomes to spermatozoa also increased the velocity values of sperm as judged by motion analysis (). All three concentrations of prostasomes used had a similar promoting effect on VCL (p < 0.05). For the VAP, 150 and 300 µM had a significant effect which was more potent than that observed with 450 µM (). LIN and ALH parameters were not significantly modified whatever the concentration of prostasomes utilized (data not shown).
Figure 2. Effect of different concentrations of prostasomes on sperm motility. The sperm motility of spermatozoa incubated in the absence or presence of prostasomes (150, 300 and 450 μM) was analyzed by CASA. The results indicate that addition of prostasomes induced a significant decline of non-progressive motile spermatozoa which was accompanied by an increase of the proportion of immotile spermatozoa. The values are means ± SEM, n = 11. * significantly different (p < 0.05) compared with control (SPZ + 0 μM prostasomes). † significantly different (p < 0.05) compared with 150 μM of prostasomes.
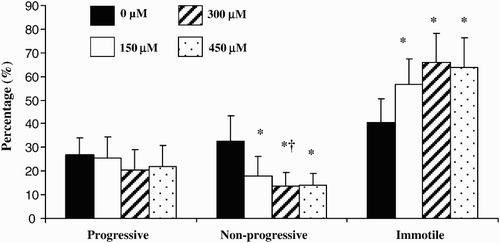
Figure 3. Effect of different concentrations of prostasomes on sperm motion parameters. The addition of prostasomes to spermatozoa resulted in an increase of VAP and VCL. For VAP, only 150 and 300 μM of prostasomes had a significant effect whereas all 3 three concentrations analyzed had a promoting effect on VCL. The table indicates the mean values measured for each parameter considered. The values are means ± SEM, n = 10. * significantly different (p < 0.05) compared with control (SPZ + 0 μM prostasomes). A table with raw data (means ± SEM) for VAP, VSL and VCL (expressed in μm/s) obtained from the CASA analysis is presented.
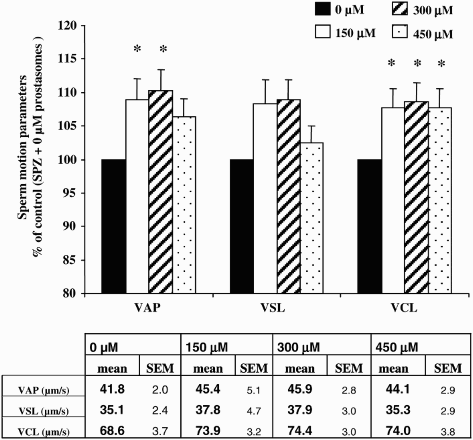
It is important to point out that sperm viability was not significantly modified after incubation with prostasomes (control: 80.25% ± 4.5%; 150 µM of prostasomes: 80.5% ± 7.4%; 300 µM of prostasomes: 78% ± 3.3%; 450 µM of prostasomes: 77.5% ± 7.7% with p values of 0.91; 0.19, and 0.18, respectively).
Existence of a putative adhesion of prostasomes with spermatozoa
The HPTLC technique was used in order to evaluate the putative adhesion of prostasomes to spermatozoa. Lipid analysis on supernatant and pellets (spermatozoa) was performed at the end of the incubation period as indicated in supplemental –3 available in the electronic version of the manuscript. These included prostasomes alone (150, 300, and 450 µM) which was used as a control (see below) and spermatozoa control and spermatozoa incubated with different concentrations of prostasomes (150, 300, and 450 µM).
The analysis of prostasomes incubated alone () was undertaken as a control and indicated that a negligible amount of lipids deriving from ‘free’ prostasomes could be found in the pellet fractions after their centrifugation (9%, 7.3%, and 6.6% with 150, 300, and 450 µM, respectively; see ).
Table 1. Different amounts of prostasomes incubated alone for 3 hours and the recovery of cholesterol in the pellet after centrifugation. The amount of cholesterol recovered before incubation and the percent of cholesterol recovered in the pellets after centrifugation is given.
In summary, centrifuging prostasomes in the absence of spermatozoa did not lead to a significant number of prostasomes in the pellets. However, the incubation of prostasomes with spermatozoa resulted in the addition of up to 10 times the amount of lipids as compared to a control (a). Our results indicated that the amounts of Chol and PC in the pellets were significantly higher for all the concentrations of prostasomes used (a), p < 0.05. For SM and phosphatidylethanolamine (PE), higher concentrations of prostasomes were needed to obtain a significant enrichment of the pellets (300 and 450 µM) (a), p < 0.05.
Figure 4. Pellets lipid composition after incubation of spermatozoa with increasing concentrations of prostasomes. Incubation of spermatozoa with increasing concentrations of prostasomes resulted in a significant enrichment of the pellets in Chol, PE, PC and SM (Fig. 4a). The values are means ± SEM, n = 7 (for Chol, PE and SM) and n = 4 (for PC). * significantly different (p < 0.05) compared with control (SPZ + 0 μM prostasomes), † significantly different (p < 0.05) compared with 150 μM. A picture of the resulting HPTLC plate of the analysis of lipids extracted from spermatozoa alone (control; SPZ + 0 μM prostasomes, lane 1) or spermatozoa incubated with different concentrations of prostasomes (150, 300, 450 μM, lanes 2-3-4 respectively) is shown (Fig. 4b). The amounts of standards spotted on the plate are for PC, PE, SM and Chol: 1, 2, 4, 6 and 8 μg and for PI and PS: 0.5, 1, 2, 3, 4 μg.
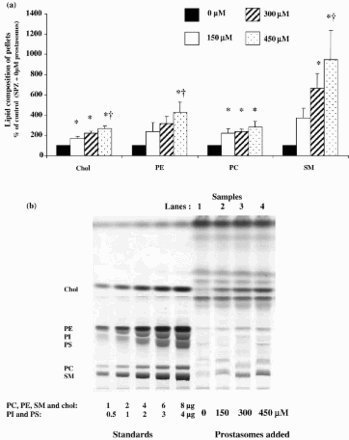
Supplemental Figure 1. Lipid analysis of the two fractions (supernatant and pellets: spermatozoa) obtained after centrifuging spermatozoa incubated with increasing amounts of prostasomes. This HPTLC plate allows better identification of the different sub-classes of lipids analyzed (SM = Sphingomyelin; PC = Phosphatidylcholin; PS = Phosphatidylserin; PI = Phosphatidylinositol; PE = Phosphatidylethanolamin; Chol = Cholesterol). In this new plate, the lines corresponding to PC and SM are more distinguishable. The SM band appears as a doublet and its migration depends on its fatty acid composition which is different from the fatty acid composition of the standards.
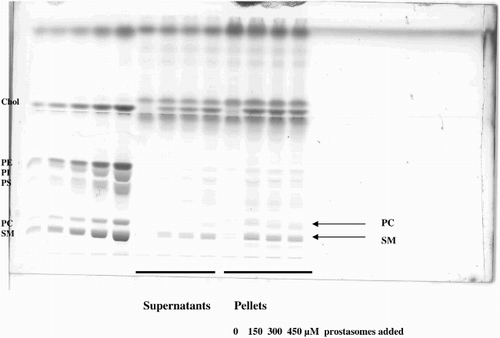
Supplemental Figure 2. Identification of lipid subclasses by bi-dimensional HPTLC. The identification of each spot corresponding to the different sub-classes of lipids was confirmed by bi-dimensional analysis.
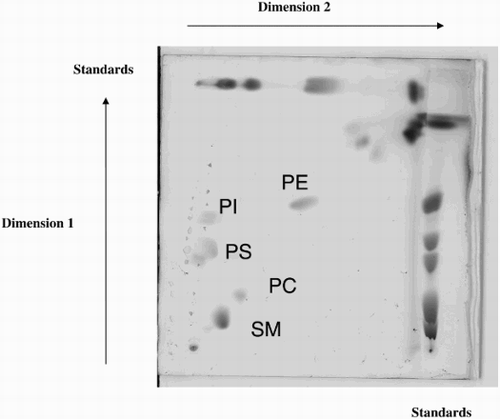
Supplemental Figure 3. Lipid analysis of different fractions in the absence or presence of lipid standards. A new HPTLC plate on which different samples were spotted, is presented in order to explain the non alignment of the lipid classes of the samples with the standards in Figure 4b which is due to the fact that the standards used in our experiments were from various sources. The fatty acid composition (molecular species) of the subclasses (PC = Phosphatidylcholin; PE = Phosphatidylethanolamin; SM = Sphingomyelin; PI = Phosphatidylinositol; PS = Phosphatidylserin) of phospholipids of the standards was different from the composition of the subclasses of phospholipids extracted from human spermatozoa which explains the shift observed for some of the bands. Lanes 2, 4, 6, and 8 correspond to supernatant 100,000g; seminal plasma 10,000g; spermatozoa, and prostasomes. Lane 1 corresponds to the standards alone. Lanes 3, 5, 7, and 9 correspond to the same samples as the ones plated as lanes 2, 4, 6, and 8 with the difference that in each of them standards were added. When comparing lane 2 with lane 3, lane 4 with lane 5, lane 6 with lane 7, and lane 8 with lane 9, we can see that the bands corresponding to SM and PC are not necessarily at the same level and that a shift could be observed for some of the samples. This discrepancy is due to the difference of molecular species of phospholipids subclasses from standards versus samples.
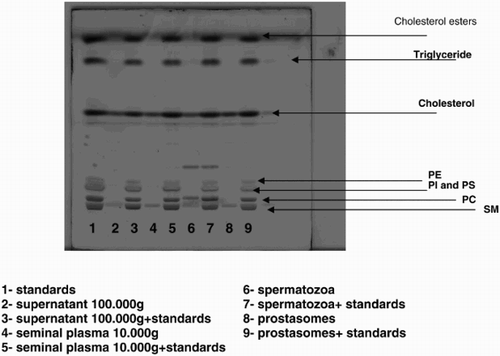
Discussion
Prostasomes are vesicles secreted by the prostate gland which sizes varying from 50 to 500 nm in diameter. These vesicles share similarities with exosomes which present an electron dense appearance and a diameter of 50 to 500 nm. Their Chol to phospholipid ratio can be as high as 2 and sphingomyelin is the major phospholipid constituent. Exosomes are secreted by the epididymal epithelium in an aprocrine way [Sullivan et al. Citation2005]. Therefore it is possible that the vesicles we isolated from the ejaculates contain exosomes and exosomes-like vesicles.
It is likely that prostasomes may have several functional activities as reflected by their size heterogeneity. Indeed, prostasomes have been described to possess multiple properties (immunosuppressive, antioxidant, and antibacterial) and to influence sperm functions (motility, capacitation, and acrosome reaction). In fact, the structural heterogeneity and protein composition of prostasomes/exosome-like vesicles, which is likely to reflect heterogeneity in function, has recently been characterized to a degree [Poliakov et al. Citation2009].
Some of the modifications observed during human spermatozoa capacitation include an increased activity of adenylyl cyclase, elevation of cyclic adenosine monophosphate (cAMP), stimulation of PKA [Lefièvre et al. Citation2002], and increased protein serine/threonine and tyrosine phosphorylation [O'Flaherty et al. Citation2004]. Our experiments have been done in a capacitating incubation medium which contains bicarbonate and calcium, agents known to induce capacitation [Flesch et al. Citation2001; Hoshi et al. Citation1982, Roberts et al. Citation2003]. In addition, Percoll gradient centrifugation, which was used to prepare spermatozoa, contains BSA, an acceptor of Chol described as an inducer of capacitation in vitro [Roberts et al. Citation2003; Choi et al. Citation2003; Bedu-Addo et al. Citation2005].
It has been suggested that, during capacitation, changes take place at the plasma membrane level that are responsible for initiating intracellular signal transduction. Indeed, efflux of Chol from the plasma membrane induces a cascade of events leading to an increase in protein phosphorylation of a variety of substrates [Visconti et al. Citation1999; Osheroff et al. Citation1999]. Our experiments indicated that in non capacitating conditions, the intensity of the phosphorylation of the 107 KDa band was weak. However, incubating the spermatozoa in capacitating conditions (in the absence of prostasomes) led to a tremendous increase in the phosphorylation status of the 107 KDa protein band which was counteracted by the presence of prostasomes.
We hypothesized that the decrease of tyrosine phosphorylation of the 107 kDa band which reflects an anti-capacitating state is linked to an enrichment of Chol (brought by prostasomes) which would prevent capacitation of spermatozoa until they are exposed to Chol acceptors in the female reproductive tract. Our lipid analysis results are in agreement with this hypothesis since a significant enrichment of spermatozoa in Chol was observed when prostasomes were added.
After incubation of spermatozoa with prostasomes for 3 h, we observed a loss of the percentage of motile sperm specially spermatozoa with non progressive motility. Yet, several other studies [Arienti at al. 1999; Carlsson et al. Citation1997] have reported that prostasomes have a positive impact on motility, this could reflect the differences in experimental conditions between our study and other studies (length of incubation, incubation medium particularly pH conditions, ratio of spermatozoa/prostasomes, experimental determination of sperm motility, and the sperm subpopulation used).
It is important to highlight that some of the promoting effects of prostasomes on sperm cell motility were obtained in different experimental settings such as conditions which have a deleterious effect on spermatozoa. Indeed, the effect of prostasomes was analyzed on spermatozoa rendered immotile by buffer washing and it was demonstrated that in these conditions, prostasomes were able to restore the loss of forward motility [Fabiani et al. Citation1994; Wang et al. Citation2001]. In the same way, prostasomes were able to counteract the negative effects of the acidic value of the incubation medium on sperm motility [Arienti et al. Citation1999]. In our study, prostasomes were added to Percoll-selected spermatozoa which, unlike in previous studies, represents a sperm selected subpopulation which displays a good functional quality (motility and viability) together with an ability to undergo capacitation associated tyrosine- phosphorylation [Buffone et al. Citation2004].
The effect of prostasomes on motion parameters was also studied. Sperm velocities were increased and the most sensitive parameters were VAP and VCL whereas no significant differences were observed for VSL. One of the explanations for increased VCL and VAP could be linked to the discrepancy between non-progressive and progressive motility. Indeed, the percentage of non-progressive motile spermatozoa was decreased by prostasomes whereas the percentage of the progressive motile spermatozoa was unchanged. As a result, in the motile sperm population there is enrichment in progressive motile spermatozoa which would explain the increase in their mean swimming speed. ALH and LIN were unchanged suggesting that in the conditions reported here prostasomes affect neither the spermatozoa swimming pattern nor their trajectory.
The incubation of prostasomes with spermatozoa resulted in the addition (on average) of 3-4 times the amount of lipids as compared to the control. Indeed, centrifuging prostasomes in the absence of spermatozoa did not lead to a significant quantity of prostasomes in the pellets. Prostasomes by themselves are too light to enter the pellet but they will be pelleted if they adhere to the denser sperm. These results are in favour of a massive adhesion of prostasomes to spermatozoa. Our results are supported by the literature that indicates that prostasomes have the ability to interact with other cells. Indeed, a pH dependent adhesion of prostasomes to leukocytes but without fusion has also been reported [Arienti et al. Citation1998b]. In addition, Delves et al. [2005; 2006] have demonstrated an inhibitory effect of prostasomes isolated from human semen on angiogenesis using either HUVEC cells or rat aortic rings as model systems. Their results suggest an important role of lipid transfer rather than the implication of proteins. The lipid transfer mechanism is not fully understood and it has been suggested that the exchange of Chol between prostasomes and spermatozoa takes place through the aqueous phase or at the time of vesicle sperm collision [Cross and Mahasreshti Citation1997].
More recently it has been suggested that some of the protein components of prostasomes such as the beta-galactoside-binding protein (galectin-3) could be involved in the regulation of sperm function [Jones et al. Citation2010].
In conclusion, our results indicate that prostasomes by interacting and transferring some of their lipid components to the spermatozoa might prevent premature capacitation. Hence, premature capacitation could be a causal factor of male subfertility as prematurely capacitated spermatozoa will undergo a spontaneous acrosome reaction and consequently will possess a relatively lower fertilizing ability.
Materials and methods
Sperm preparation
Semen samples were obtained from men referred to our reproductive biology laboratory (CHU, Clermont Ferrand, France) for routine semen analysis before in vitro fertilization or for semen analysis screening. The ejaculates were collected by masturbation after a recommended sexual abstinence of 2-3 days. Immediately after liquefaction (30 min at 37oC) sperm parameters were determined in accordance with World Health Organization (WHO) guidelines [World Health Organization Citation1999]. Surplus semen samples within the normal limits set down by the WHO were used for experimental analysis. In accordance with appropriate ethical guidelines, our study used discarded human spermatozoa. In this situation the approval from an Institutional Review Board or equivalent oversight was not necessary at the time the study was performed in French legislation.
Spermatozoa were separated from seminal plasma on a two-step discontinuous Percoll gradient, using 95 and 47.5% Percoll created by diluting an isotonic Percoll preparation (designated 100% Percoll) with T6 medium (99.4 mmol/l NaCl, 1.42 mmol/l KCl, 0.47 mmol/l MgCl2, 0.36 mmol/l Na2HPO4, 1.78 mmol/l CaCl2, 25 mmol/l NaHCO3, 24.9 mmol/l sodium lactate, 0.47 mmol/l sodium pyruvate, 5.56 mmol/l glucose; pH 7.4) containing 0.3% BSA. Liquefied semen was carefully laid on the top gradient and the sample spun at 500 x g for 30 min. Then, the purified population of spermatozoa (from 95% Percoll layer) was recovered and washed with T6 medium.
Preparation of prostasomes
Prostasomes were obtained from human semen and purified according to Ronquist and Brody [1985]. Briefly, liquefied ejaculates were centrifuged for 10 min at 1,000 x g, then the supernatant was collected and centrifuged again at 10,000 x g for 20 min to eliminate all possible contaminating cells. The new supernatant was subsequently subjected to preparative ultracentrifugation at 110,000 x g for 2 hours (h) to pellet the prostasomes. The pellet was suspended in 2.5 ml of 30 mM Tris-HCl buffer, pH 7.6 containing 130 mM NaCl and chromatographed on a Sephadex G200 column. Elution was performed with the Tris-HCl buffer. The eluted fractions were pooled, ultracentrifuged for 3 h at 110,000 x g. The prostasomes were resuspended in NaCl 0.9% and stored at −20oC.
Lipids were extracted from the prostasomes and their Chol content was determined as previously described by Saez et al. [1998]. Quantities of prostasomes used for experiments were then expressed in terms of Chol.
Incubation procedure
Spermatozoa were resuspended at 5 million in 100 µL of BWW medium containing PVA (1 mg/mL) and essentially fatty acid free BSA 0.3% supplemented or not with different concentrations of prostasomes and incubated for 3 h at 370C under an atmosphere of 5% CO2 in air. In addition, prostasomes alone were incubated in the same conditions. These conditions (3 h of incubation) were guided by characteristics of our experimental model previously used [Picherit-Marchenay et al. Citation2004] and by data of literature which indicated that protein tyrosine phosphorylation appears as a last event of capacitation and is not significant for the first 2 h of incubation under capacitating conditions [O'Flaherty et al. Citation2006]. The concentrations of prostasomes (15, 30, and 45 nmoles of Chol / 100 µL of incubation medium: 150, 300, and 450 µM) were chosen to frame physiological values of Chol content in seminal plasma (around 300 µM).
For each condition (spermatozoa incubated in the absence or presence of increasing concentrations of prostasomes) the incubation was performed in duplicate. The first fraction was used for the sperm motion and lipid analyses while the second fraction was utilized for Western blotting analysis.
Sperm motion analysis
After incubation, the sperm suspensions were analyzed using a Computer Assisted Sperm Analysis system (CASA), Hamilton Thorne Sperm Analyser (HTM Ceros model V.12, Hamilton-Thorn Biosciences, Beverly, MA, USA). A three µL sperm sample was loaded into a pre-warmed disposable 20 µm deep slide (Leja, Niew-Vennep, Netherlands). The slide was placed on the stage of the HTM minitherm which stabilizes the temperature at 37oC.
Spermatozoa were classified according to their velocity: rapid (VAP > 25 µm/s), medium (5 µm/s < VAP < 25 µm/s), slow (VAP < 5 µm/s), and immotile. All spermatozoa with rapid and medium velocities were considered as spermatozoa with a progressive motility and spermatozoa with a slow velocity as spermatozoa with a non progressive motility.
In addition the following parameters were recorded: mean average path velocity (VAP: velocity of the smoothed average cell path, µm/s), mean curvilinear velocity (VCL: velocity of total distance between each sperm position, µm/s), mean straight line velocity (VSL: velocity between the straight line beginning and the end of the tracks, µm/s), amplitude of lateral head displacement (ALH: mean width of the head oscillation, µm) and mean percentage linearity (LIN = VSL/VCL, departure of the cell track from the straight line, %) of all motile spermatozoa.
Assessment of cell viability
Viability was measured in accordance with the WHO guidelines using an eosin-nigrosin test [Campbell et al. Citation1956]. This staining technique is based on the principle that dead cells with a damaged plasma membrane take up eosin. Nigrosin used as the counterstain makes the slides easier to analyze. A total of 200 cells were counted under a light microscope (magnification X 400) to evaluate the live spermatozoa (unstained) and the dead spermatozoa (stained red). Sperm viability was defined as the percentage of live cells.
Western blotting analysis
At the end of the incubation period, the sperm suspensions were centrifuged at 600 x g for 10 min, washed twice with PBS and solubilized as described by Ficarro et al. [2003] in Lysis buffer containing 50 mM Tris-HCl, pH 7.4, 150 mM NaCl, 0.5% sodium deoxycholate, 1% NP-40, 0.1% SDS supplemented with a protease inhibitor cocktail (Roche, Meylan, France) and phosphatase inhibitors diluted 1:100 (Sigma Aldrich, Saint Quentin Fallavier, France). Cell debris was pelleted at 14,000 x g and proteins from the supernatant were used to study protein expression. Reduced samples from sperm lysates (five million spermatozoa) were subjected to 8% SDS-PAGE. Prestained protein standards (Amersham Pharmacia Biotech, Orsay, France) were run in each gel as indicated by the manufacturer. The proteins were transferred onto nitrocellulose membranes (Hybond ECL; Amersham Pharmacia Biotech, Orsay, France) using the Mini Trans Blot electrophoretic transfer system (Bio-Rad Laboratories, Hercules, CA, USA). Blotted membranes were blocked overnight at 4oC in Phosphate Buffer Saline-Tween 20 (PBS-T; 0.1%) supplemented with 10% of heat-inactivated human serum. The membranes were then hybridized for 1 h at room temperature with an anti-phosphotyrosine antibody (P-Y69, mouse monoclonal IgG, 1 mg/ml; BD Biosciences Pharmingen, San Jose, CA, USA) diluted 1:700 in PBS-T supplemented with 1% human serum. After 3 washes of 20 min each with PBS-T, the blots were incubated for 1 h at room temperature with horseradish peroxidase-conjugated anti-mouse IgG antibody diluted 1:700 in PBS-T supplemented with 1% human serum, washed again in the same conditions and visualized with enhanced chemioluminescence reagents (Amersham Pharmacia Biotech, Orsay, France). Phosphotyrosine residues were detected by autoradiography with Fuji-film. A semi-quantitative analysis of the phosphotyrosine-containing protein band was performed by densitometry, using Sigma Scan Pro 5 software.
Lipid extraction and HPTLC analysis
The sperm suspensions incubated in the presence or absence of prostasomes were centrifuged at 600 x g for 10 min. The pelleted spermatozoa were washed with Phosphate Buffer Saline (PBS) and the lipids were extracted according to the method developed by Grizard et al. [2000].
HPTLC plates were washed with a mixture of chloroform-methanol (1:1; v/v) and heated for 10 min at 1100C. The lipid fractions were spotted on the plate (Linomat IV: CAMAG, Muttenz, Switzerland) and resolved sequentially with a mixture of methyl acetate; chloroform; n-propanol; methanol; 0.25% KCl in water (25:25:25:10:9; v/v) then hexane; diethylether; acetic acid (80:20:2; v/v). The plates were stained with 10% CuSO4 w/v in 8% H3PO4; v/v, charred at 160oC and the lipid quantification undertaken. Different amounts of standards were spotted on the same TLC plate (for Chol, SM, PE, and PC: 1; 2; 4; 6, and 8µg and for PI and PS: 0.5; 1; 2; 3, and 4 µg) in order to identify the different sub-classes of phospholipids. The quantification was performed using density measurements with SIGMA Scan Pro 5 software.
Statistical analyses
Values are presented as mean ± SEM. An ANOVA was performed to analyze the effect of different concentrations of prostasomes on i) the tyrosine phosphorylation intensity of the 107 KDa protein band, ii) sperm motility and the different parameters of sperm motion, and iii) the lipid content of the pellets recovered in the infranatant at the end of the incubation period. When a significant overall effect was detected, differences among individual means were assessed using the Fisher test. For all statistical tests, the level of significance was set at p < 0.05.
Abbreviations
BWW: | = | Biggers Whitten Whittingham's medium |
PVA: | = | polyvinyl alcohol |
BSA: | = | bovine serum albumin |
VCL: | = | mean curvilinear velocity |
VAP: | = | average path velocity |
Chol: | = | cholesterol |
SM: | = | sphingomyelin |
PC: | = | phosphatidylcholine |
LIN: | = | mean percentage linearity |
ALH: | = | amplitude of lateral head displacement |
PE: | = | phosphatidylethanolamine |
VSL: | = | straight line velocity |
HUVEC | = | : human umbilical vein endothelial cells. |
Acknowledgments
The authors gratefully acknowledge Marjorie Mollaret and Emilie Viennois for their technical help and Dr. Laurent Lagrost for helpful suggestions and discussion.
Declaration of interest: There is no conflict of interest that could be perceived as prejudicing the impartiality of the research reported.
References
- Arienti, G., Carlini, E., Nicolucci, A., Cosmi, E.V., Santi, F., and Palmerini, C.A. (1999) The motility of human spermatozoa as influenced by prostasomes at various pH levels. Biol Cell 91:51–54.
- Arienti, G., Carlini, E., and Palmerini, C.A. (1997) Fusion of human sperm to prostasomes at acidic pH. J Membr Biol 155(1):89–94.
- Arienti, G., Carlini, E., Polci, A., Cosmi, E.V., Palmerini, CA (1998a) Fatty acid pattern of human prostasome lipid. Arch Biochem Biophys 358:391–395.
- Arienti, G., Carlini, E., Saccardi, C., Palmerini, C.A. (1998b) Interactions between prostasomes and leukocytes. Biochim Biophys Acta 1425:36–40.
- Arvidson, G., Ronquist, G., Wikander, G., Ojteg, A.C. (1989) Human prostasome membranes exhibit very high cholesterol/phospholipid ratios yielding high molecular ordering. Biochim Biophys Acta 984(2):167–173.
- Bedu-Addo, K., Lefievre, L., Moseley, F.L., Barratt, C.L., Publicover, S.J. (2005) Bicarbonate and bovine serum albumin reversibly ‘switch’ capacitation-induced events in human spermatozoa. Mol Hum Reprod 11(9):683–691.
- Buffone, M.G., Doncel, G.F., Marin Brigiller, C.I., Vasquez-Levin, M.H., Calamera, J.C. (2004) Human sperm subpopulations: relationship between functional quality and protein tyrosine phosphorylation. Hum Reprod 19:139–146.
- Burden H.P., Holmes, C.H., Persad, R., Whittington, K. (2006) Prostasomes-their effects on human male reproduction and fertility. Hum Reprod Update 12(3):283–292.
- Campbell, R.C., Dott, H.M., Glover, T.D. (1956) Nigrosin eosin as a stain for differentiating live and dead spermatozoa, J Agric Sci 48:1–8.
- Carlsson, L., Ronquist, G., Stridsberg, M., Johansson, L. (1997) Motility stimulant effects of prostasome inclusion in swim-up medium on cryopreserved human spermatozoa. Arch Androl 38(3):215–221.
- Choi, Y.H., Landim-Alvarenga, F.C., Seidel, G.E. , Squires, E.L. (2003) Effect of capacitation of stallion sperm with polyvinylalcohol or bovine serum albumin on penetration of bovine zona-free or partially zona-removed equine oocytes. J Anim Sci 81(8):2080–2087.
- Cross, N.L., Mahasreshti, P. (1997) Prostasome fraction of human seminal plasma prevents sperm from becoming acrosomally responsive to the agonist progesterone. Arch Androl 39(1):39–44.
- Delves, G.H., Stewart, A.B., Lwaleed, B.A., Cooper, A.J. (2005) In vitro inhibition of angiogenesis by prostasomes. Prostate Cancer and Prostatic Dis 8(2):174–178.
- Delves, G.H., Goyal, A., Lwaleed, B.A., Cooper, A.J. (2006) Seminal prostasomes inhibit the angiogenesis activity of rat aortic rings. Prostate Cancer and Prostatic Dis 9(4): 444–447.
- Fabiani, R., Johansson, L., Lundkvist, O., Ulmsten, U., Ronquist, G. (1994) Promotive effect by prostasomes on normal human spermatozoa exhibiting no forward motility due to buffer washings. Eur J Obstet Gynecol Reprod Biol 57:181–188.
- Flesch, F.M., Brouwers, J.F., Nievelstein, P.F., Verkleij, A.J., Van Golde, L.M., Colenbrander, B., Gadella, B.M. (2001) Bicarbonate stimulated phospholipid scrambling induces cholesterol redistribution and enables cholesterol depletion in the sperm plasma membrane. J Cell Sci 114(Pt19):3543–3555.
- Ficarro, S., Chertihin, O., Westbrook, V.A., White, F., Jayes, F., Kalab, P., (2003) Phosphoproteome analysis of capacitated human sperm. Evidence of tyrosine phosphorylation of a kinase-anchoring protein 3 and valosin-containing protein/p97 during capacitation. J Biol Chem 278(13):11579–11589.
- Force, A., Grizard, G., Giraud, M.N., Motta, C., Sion, B., Boucher, D. (2001) Membrane fluidity and lipid content of human spermatozoa selected by swim-up method. Int J Androl 24(6):327–334.
- Grizard, G., Sion, B., Bauchart, D., Boucher, D. (2000) Separation and quantification of cholesterol and major phospholipid classes in human semen by high-performance liquid chromatography and light-scattering detection. J Chromatogr B Biomed Sci Appl 740(1):101–107.
- Hoshi, K., Saito, A., Suzuki, M., Hayashi, K., Yanagimachi, R. (1982) Effects of calcium and magnesium on in vitro fertilization in human. Nippon Sanka Fujinka Gakkai Zasshi 34:1899–1906.
- Jones, J.L., Saraswati, S., Block, A.S., Litchi, C.F., Mahadevan, M., Diekman, A.B. (2010) Galectin-3 is associated with prostasomes in human semen. Glycoconj J. 27(2):227–36.
- Lefièvre, L., Jha, K.N., de Lamirande, E., Visconti, P.E., Gagnon, C. (2002) Activation of protein kinase A during human sperm capacitation and acrosome reaction. J Androl 23(5):709–716.
- O'Flaherty, C., de Lamirande, E., Gagnon, C. (2006) Positive role of reactive oxygen species in mammalian sperm capacitation: triggering and modulation of phosphorylation events. Free Radic Biol Med 41(4):528–540.
- O'Flaherty, C., de Lamirande, E., Gagnon, C. (2004) Phosphorylation of the Arginine-X-X (Serine/Threonine) motif in human sperm proteins during capacitation: modulation and protein kinase A dependency. Mol Hum Reprod 10(5):355–363.
- Osheroff, J.E., Visconti, P.E., Valenzuela, J.P., Travis, A.J., Alvarez, J., Kopf, G.S. (1999) Regulation of human sperm capacitation by a cholesterol efflux-stimulated signal transduction pathway leading to protein kinase A-mediated up-regulation of protein tyrosine phosphorylation. Mol Hum Reprod 5(11):1017–1026.
- Picherit-Marchenay, C., Bréchard, S., Boucher, D., Grizard, G. (2004) Correlation between tyrosine phosphorylation intensity of a 107 KDa protein band and A23187-induced acrosome reaction in human spermatozoa. Andrologia 36(6):370–377.
- Poliakov, A., Spilman, M., Dokland, T., Amling, C.L., Mobley, J.A. (2009) Structural heterogeneity and protein composition of exosome-like vesicles (prostasomes) in human semen. Prostate 69(2):159–67.
- Roberts, K.P., Wamstad, J.A., Ensrud, K.M., Hamilton, D.W. (2003) Inhibition of capacitation-associated tyrosine phosphorylation signaling in rat sperm by epididymal protein Crisp-1. Biol Reprod 69(2):572–581.
- Ronquist, G., Brody, I. (1985) The prostasome: its secretion and function in man. Biochim Biophys Acta 822(2): 203–218.
- Ronquist, G., Brody, I., Gottfries, A., Stegmayr, B. (1978) An Mg2+ and Ca2+-stimulated adenosine triphosphatase in human prostatic fluid. Andrologia 10(4):261–272.
- Ronquist, G., Nilsson, B.O., Hjertën, S. (1990) Interaction between prostasomes and spermatozoa from human semen. Arch Androl 24(2):147–157.
- Ronquist, K.G., Ronquist, G., Carlsson, L., Larsson, A. (2009) Human prostasomes contain chromosomal DNA. Prostate 69(7):737–43.
- Saez, F., Motta, C., Boucher, D., Grizard, G. (1998) Antioxidant capacity of prostasomes in human semen. Mol Hum Reprod 4(7):667–672.
- Saez, F., Motta, C., Boucher, D., Grizard, G. (2000) Prostasomes inhibit the NADPH oxidase activity of human neutrophils. Mol Hum Reprod 6(10):883–891.
- Siciliano, L., Marcianò, V., Carpino, A. (2008) Prostasome-like vesicles stimulate acrosome reaction of pig spermatozoa. Reprod Biol Endocrinol 30:6–5.
- Sullivan, R., Saez, F., Girouard, J., Frenette, G. (2005) Role of exosomes in sperm maturation during the transit along the male reproductive tract. Blood Cells Mol Dis 35(1):1–10.
- Utleg, A.G., Yi, E.C., Xie, T., Shannon, P., White, J.T., Goodlett, D.R., (2003) Proteomic analysis of human prostasomes. Prostate 56(2):150–161.
- Visconti, P.E., Ning, X., Fornes, M.W., Alvarez, J.G., Stein, P., Connors, S.A., Kopf, G.S. (1999) Cholesterol efflux-mediated signal transduction in mammalian sperm: cholesterol release signals an increase in protein tyrosine phosphorylation during mouse sperm capacitation. Dev Biol 214(2):429–443.
- Visconti, P.E., Kopf, G.S. (1998) Regulation of protein phosphorylation during sperm capacitation. Biol Reprod 59(1):1–6.
- Wang, J., Lundqvist, M., Carlsson, L., Nilsson, O., Lundkvist, O., Ronquist, G. (2001) Prostasome-like granules from PC-3 prostate cancer cell line increase the motility of washed human spermatozoa and adhere to the sperm. Eur J Obstet Gynecol Reprod Biol 96(1):88–97.
- World Health Organization (1999) WHO laboratory manual for the examination of human semen and sperm-cervical mucus interaction, 4th Edn. Cambridge University Press, Cambridge.