Abstract
We explored the molecular mechanisms of obesity and insulin resistance in patients with polycystic ovary syndrome (PCOS) using a human embryonic stem cell model (hESCs). Three PCOS-derived and one non-PCOS-derived hESC lines were induced into adipocytes, and then total RNA was extracted. The differentially expressed PCOS-derived and non-PCOS-derived adipocytes genes were identified using the Boao Biological human V 2.0 whole genome oligonucleotide microarray. Signals of interest were then validated by real-time PCR. A total of 153 differential genes were expressed of which 91 genes were up-regulated and 62 down-regulated. Nuclear receptor subfamily 0, group B, member 2 (NR0B2) was an up-regulated gene, and the GeneChip CapitalBio® Molecule Annotation System V4.0 indicated that it was associated with obesity and diabetes (Ratio ≥2.0X). Multiple genes are involved in PCOS. Nuclear receptor subfamily 0, group B, member 2 may play a role in obesity and insulin resistance in patients with PCOS.
Introduction
Polycystic ovary syndrome (PCOS) is a common endocrine disorder affecting fertility of women with an incidence of 5%-10% [Azziz et al. Citation2004]. The clinical manifestations of PCOS are complex. More than 50% of patients with PCOS have obesity and 50%–90% have insulin resistance. More and more studies have shown that adipose tissue plays an important role in PCOS [Dunaif et al. Citation2001], but little is known about the regulation of genes in adipose tissue. To begin to address this issue we examined the expression of lipoidosis-related genes in the patients with PCOS. A set of differentially expressed genes in adipocytes derived from induced PCOS and non-PCOS human embryonic stem cells (hESCs) was identified.
Results and Discussion
Differentiation of hESCs into adipocytes and the identification of adipocytes
Three days after induction, clones became loose and possessed an irregular edge. Five days after induction, lipid droplets were seen in the cytoplasm with the nucelus in the cellular center. Two weeks after induction, mature adipocytes were observed. An increasing number of lipid droplets gathered, and then cells became round or oval in shape and the nucelus localized to one side of the cells. Peak differentiation occurred 3–4 weeks after induction (). Lipid droplets were specifically stained with oil red O, whereas undifferentiated cells and intracellular non-lipid component were not stained with oil red O (). RT-PCR was consistent with the absence of peroxisome proliferator-activated receptor gamma (PPARγ)2 expression in pre-differentiated cells, but PPARγ2 was expressed in 3-week-induced cells ().
Figure 1. Differentiation of human embryonic stem cells (hESCs) into adipocytes and the identification of adipocytes. (A) Thawed clones before passage ×100. The thawed hESCs are incubated in an inactivated mixed feeder layer (1:1 of mouse embryonic fibroblasts: human foreskin fibroblasts) at 37°C in an atmosphere of 5% CO2 for two days. Next day, hESCs are observed under an inverted microscope. hESCs grow in colony, and are flat in shape with clear cell boundaries. (B) Adipocytes induced for 21 days ×40. Three days after induction, clones become loose with irregular edge. Five days after induction, lipid droplets are seen in cytoplasm with the nucelus in cellular center. Two weeks after induction, mature adipocytes occur. More and more lipid droplets gather, and then cells become round or oval in shape and the nucelus are pulled to one side of the cells. Differentiation peak occurs 21 after induction. (C) Oil red O staining ×40. After hESCs differentiate into mature adipocytes, cells become round with strong refractivity and lipid droplets gather. Lipid droplets in mature adipocytes can be specifically stained with oil red O, showing red color. (D) peroxisome proliferator-activated receptor gamma (PPARγ)2 mRNA expression detected by RT-PCR RNA in cells is extracted with Trizol, and then PPARγ2 mRNA expression is detected with β-actin mRNA as internal control. Lane 1 displays that there is no PPARγ2 expression in the pre-differentiated hESCs. Lane 2 displays that there is PPARγ2 expression in the differentiated adipocytes.
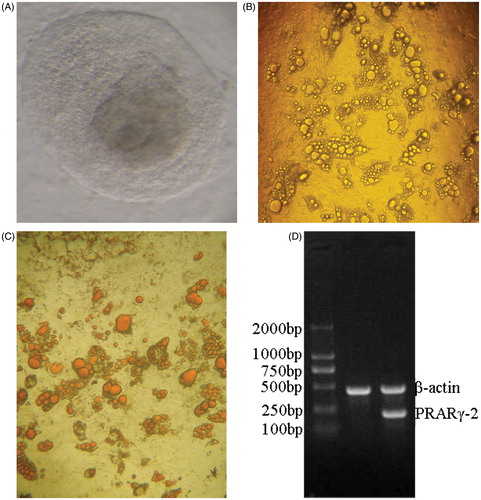
Differential expression of genes in adipocytes induced from PCOS-derived and non-PCOS-derived hESCs
The extracted total RNA had an A260/A280 value of greater than 1.8 and the ratio of the 28S to 18S rRNAs as determined by agarose formaldehyde denaturing gel electrophoresis, was greater than 2:1. This indicated that the total RNA was of sufficient quality for microarray hybridization. Upon hybridization each parameter for each sample was in line with the standards of quality control. The external standard, and internal standard were within the normal range and the negative control was negative. The repeatability of the house-keeping gene showed that the ratio of CV was less than 0.3. The overlap of signals did not exceed 3%. The detection rate was normal. If the transcription product ratio of sample to control was ≥2.0, the gene was regarded as differentially expressed. Namely a positive ratio of Cy5/Cy3 >2.0 or <0.5 was regarded as up-regulated or down-regulated (). Using these parameters, 153 differentially expressed genes were identified. A total of 91 genes were up-regulated and 62 genes were down-regulated and were considered as a function of known PCOS associations. These included glycometabolism, lipid metabolism, and steroid hormones, and were summarized in .
Figure 2. Pseudo-color diagrams of microarray hybridization of three polycystic ovary syndrome (PCOS)-derived adipocytes and verification of differential genes with RT-PCR. (A) cy5 is indicated with red color and cy3 with green color. For the signal in a point, if cy3 is strong, the point is indicated with green color; if cy5 is strong, the point is indicated with red color; if the intensity is similar between cy5 and cy3, the point is indicated with yellow color. Panels a, b, and c, indicate the microarray hybridization diagrams of three PCOS-derived adipocytes after comparison with non-PCOS-derived adipocytes. (B) Verification of differential genes with RT-PCR. Lane 1–4: house-keeping gene glyceraldehyde-3-phosphate dehydrogenase with 1st-cDNA as templates in experimental group 1, 2, and 3, and control group, respectively. Lane 5–8: UDP glucuronosyltransferase 2 family, polypeptide B28 gene with 1st-cDNA as templates in experimental group 1, 2, and 3, and control group, respectively. Lane 9–12: nuclear receptor subfamily 0, group B, member 2 gene with 1st-cDNA as templates in experimental group 1, 2, and 3, and control group, respectively. Lane 13–16: GALNT9(UDP-N-acetyl-alpha-D-galactosamine:polypeptide N-acetylgalactosaminyltransferase 9) gene with 1st-cDNA as templates in experimental group 1, 2, and 3, and control group, respectively. Lane 17–20: hydroxy-delta-5-steroid dehydrogenase, 3 beta- and steroid delta-isomerase 2 gene with 1st-cDNA as templates in experimental group 1, 2, and 3, and control group, respectively. Lane 21–24: tetraspanin 8 gene with 1st-cDNA as templates in experimental group 1, 2, and 3, and control group, respectively. Lane 25–28: major histocompatibility complex, class II, DR beta 3 gene with 1st-cDNA as templates in experimental group 1, 2, and 3, and control group, respectively. Marker TaKaRa DL2000, band size are 100 bp, 250 bp, 500 bp, 750 bp, 1000 bp, 2000 bp from the top to the bottom, respectively. NR0B2: nuclear receptor subfamily 0, group B, member 2.
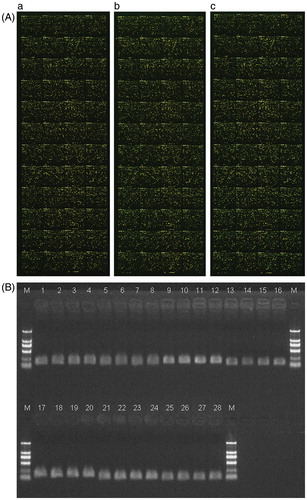
Table 1. Glycometabolism, lipid metabolism, and steroid hormone-related genes.
Verification of differentially expressed genes by RT-PCR
Since PCOS is mainly associated with glycometabolism, lipid metabolism, and steroid hormones, we selected six glycometabolism, lipid metabolism, and steroid hormone-related genes (nuclear receptor subfamily 0, group B, member 2 (NR0B2), (hydroxy-delta-5-steroid dehydrogenase, 3 beta- and steroid delta-isomerase 2), (tetraspanin 8), (major histocompatibility complex, class II, DR beta 3), (UDP glucuronosyltransferase 2 family, polypeptide B28), and (UDP-N-acetyl-alpha-D-galactosamine:polypeptide N-acetylgalactosaminyltransferase 9)) to be analyzed in this study. The six genes were validated with RT-PCR. The RT-PCR results were consistent with the results of microarray showing similar trends in up-regulation or down-regulation ( and ). This demonstrates that the results of microarray were reliable.
Table 2. Results of RT-PCR and microarray.
Polycystic ovary syndrome is associated with many factors and has various clinical manifestations. Jennifer et al. [2003] analyzed the differential gene in theca cells between patients with PCOS and normal individuals using a microarray strategy and identified new candidate genes that might be involved in the genetic etiology of PCOS. Diao et al. [Citation2004] found the differential genes in ovarian tissue between patients with PCOS and normal individuals, which is conducive to further exploration of the pathogenesis of PCOS. Haouzi et al. [Citation2012] and Huang et al. [Citation2013] compared the differential genes in cumulus cells and found three differential genes associated with embryo development, providing a new strategy for screening ovum and embryo in patients with PCOS.
Obesity and insulin resistance are common in patients with PCOS. A great deal of attention has been paid to obesity, because adipocytes are a target cell of insulin and the severity of insulin resistance is in direct proportion to body mass index [Venkatesan et al. Citation2001]. Weight reduction is an important measure to reduce the level of androgens and promote ovulation in patients with PCOS. More and more studies have shown that adipose tissue plays an important role in PCOS [Dunaif et al. Citation2001], but little attention has been given to the regulation of adipose tissue genes. Therefore, the biological functions of obesity-related genes may reveal the pathogenesis of PCOS. In this study, PCOS-derived and non-PCOS-derived hESCs were induced into adipocytes, then the differential genes were identified with microarray. The transcripts associated with adipocyte metabolism were then analyzed. This study provides a basis for clarifying the pathogenesis of obesity in patients with PCOS.
Induction efficiency of PCOS-derived hESCs into adipocytes
Differentiation of hESC into adipocytes is a complex process which is regulated by many factors. The exact molecular mechanism is not completely clear. In this study, although we used the substances which have strong effects on hESC differentiation into adipocytes, the differentiation rate was only 50%. With the rapid development of molecular biology technology, adipocyte differentiation will be further investigated. Various biological factors involved in hESC differentiation into adipocytes will become an investigative focus, which will be conducive to revealing PCOS pathogenesis.
Polycystic ovary syndrome may affect many kinds of cells, tissues, and organs. PCOS, a polygenic disease, is closely associated with multiple gene mutations. There are 37 PCOS-related genes [Diamanti-Kandarakia and Piperi Citation2005], such as fertility-related genes, glycometabolism and energy balance-related genes, steroid hormone metabolism-related genes, and insulin-related genes. In this study, we identified six differential genes, NR0B2, HSD3B2, TSPAN8, HLA-DRB3, UGT2B28, and GALNT9 in the adipocytes induced from hESCs in order to clarify PCOS pathogenesis.
In the patients with PCOS, obesity-related genes mainly include the luteinizing hormone gene, the obesity gene, and the follicle stimulating hormone gene. At present, the reported PCOS-related genes, modifier genes, are only associated with the severity of clinical manifestation. It is unlikely that PCOS is a single gene defect and is likely the result of multiple factors. The pathway may include modifiers which individually fail to play a key role in PCOS pathogenesis but together have a significant effect. For example, PCOS may be caused by a few key genes and some minor genes under multiple factors such as environment, nutrition, and an unhealthy lifestyle (e.g., hyperphagia or little exercise).
Roles of NR0B2 in obesity and insulin resistance
Nuclear receptor subfamily 0, group B, member 2 identified by Real-time PCR in this study, a small heterodimer partner gene (SHP), is located on 1p36.1 and consists of two exons and one intron. The NR0B2 protein is one of the members of the nuclear receptor superfamily. Since the ligand of this receptor has not been discovered, it is an orphan nuclear receptor [Seol et al. Citation1996]. It is an atypical nuclear receptor because it is very different from other traditional nuclear receptors in structure and function. It mainly inhibits transcriptional activation of other nuclear receptors [Båvner et al. Citation2005]. The NR0B2 proteins are involved in regulating more than 40 different target genes which are associated with adipogenesis, glycometabolism, steroidogenesis, and cholesterol metabolism [Delerive et al. Citation2004]. Peroxisome proliferator-activated receptor gamma, a kind of transcription factor, is a key factor in the regulation of adipocyte differentiation [Watanabe et al. Citation2004], and plays an important role in gene regulation of fatty acid metabolism and glycometabolism [Lefebvre et al. Citation1997]. Nuclear receptor subfamily 0, group B, member 2 enhances the transcription activity of PPARγ directly through its combination with PPARγ, and the C-terminal of NR0B2 is important for PPARγ activity [Nishizawa et al. Citation2002]. HNF-1a, HNF-4a, HNF-6a, and other transcription factors form a regulatory circuit to control the transcription of hepatocytes and pancreatic islet cells. HNF-4a dysregulation may lead to diabetes. Nuclear receptor subfamily 0, group B, member 2 is located in the center of the transcription circuit regulated by hepatocyte nuclear factor (HNF) in pancreatic islets, so NR0B2 may play an important role in the transcription of pancreatic islet cells and hence insulin response [Gupta et al. Citation2005].
In 2001, Nishigori et al. [Citation2001] reported that a NR0B2 gene mutation was associated with mild obesity in Japanese. In addition, the level of fasting insulin and newborn weight are increased in patients with this NR0B2 mutation. Han et al. [Citation2004, Citation2005] have reported that the NR0B2 gene is related to birth weight, and it may play a role in the intrauterine growth of the fetus. Hung et al. [Citation2003] and Mitchell et al. [Citation2003] showed that the NR0B2 gene mutation is not the main cause of obesity, but it may induce insulin resistance through affecting insulin secretion. In 2008, Enya et al. [Citation2008] described a NR0B2 gene defect that was involved in early mild obesity and type 2 diabetes, but the exact mechanism that NR0B2 gene affected obesity and type 2 diabetes was unclear.
There has been considerable debate about whether NR0B2 is involved in obesity and insulin resistance. This may be that the NR0B2 gene mutation rate and the effects of NR0B2 on obesity and insulin resistance are associated with population differences. Large sample multi-center studies from different populations will be needed to confirm whether the NR0B2 gene is involved in PCOS. To begin we will investigate the localization and expression of NR0B2 and the effect of the SHP NROB2 motif in adipocytes of adult patients with PCOS.
Materials and Methods
All study methods were approved by the Institutional Review Board and Ethics Committee of the First Affiliated Hospital of Zhengzhou University. All the subjects enrolled into the study gave written formal consent to participate.
Cell lines
Three PCOS-derived and one non-PCOS-derived hESC lines were established and stored in the Reproductive Medical Center, the First Affiliated Hospital of Zhengzhou University between 2008 and 2009 [Li et al. Citation2012; Wang et al. Citation2012].
Reagents
KnockOut DMEM, KnokOut serum succedaneum, Insulin Selenium (ITS), DMEM medium, and α-MEM medium were purchased from Gibco Company (Grand Island NY, USA). High glucose DMEM medium, non-essential amino acids (NEAA), HEPES were purchased from Hyclone Company (Logan, UT, USA). Trypsin, EDTA, mitomycin C, dimethyl sulfoxide (DMSO), gelatin, glutamime, β-mercapto-ethanol (2-ME), bFGF, dexamethasone, insulin, 1-methyl-3-isobutyl xanthine (IBMX), and Oil Red O were purchased from Sigma Company (Carlsbad, CA, USA). BCIP/NBT was purchased from Millpore Company (Billerica, MA, USA). SSEA-4 (813-70) was purchased from Santa Cruz (CA, USA). Rosiglitazone was purchased from Cayman Company (Michigan, USA). Trizol kit was purchased from Invitrogen Company (Carlsbad, CA, USA). RT-PCR kit was purchased from Promega Company (Madison, WI, USA). FITC-labeled rabbit anti-mouse secondary antibody was purchased from Zhongshan Company (Beijing, China). DNA Marker was purchased from Saibaisheng Company (Beijing, China).
Clonal expansion
The vitrification-cryopreserved three PCOS-derived and one PCOS-derived hESC lines in passage 20 were thawed. The thawed hESCs were incubated in an inactivated mixed feeder layer (1:1 of mouse embryonic fibroblasts: human foreskin fibroblasts) at 37 °C in an atmosphere of 5% CO2 for two d. The next day, medium was changed, and then the growth of hESCs was observed under an inverted microscope. One passage was performed every 4–7 d. Amplified clones were stored for future use.
Induction of adipocytes
The amplified ESC clones were incubated with adipogenic medium consisting of α–MEM, 10% SR, 1 μM/L of dexamethasone, 10 mg/L of insulin, 0.5 m M/L of IBMX, and 5 × 104 IU/L of penicillin/streptomycin for about 2 weeks. These ESCs were induced with 10μ M/L of rosiglitazone for about 2 weeks, and then observed under an inverted microscope.
Identification of adipocytes
Oil red O stain: After the removal of medium, cells were washed with PBS, fixed with 10% paraformaldehyde for 30 min, stained with diluted oil red O for 10 min. Cells were washed with 75% of alcohol to move redundant dyes, and then stained with hematoxylin for one min. After washed with PBS, cells were observed under an inverted microscope.
PPARγ2 mRNA expression detection with RT-PCR: RNA in adipocytes was extracted with Trizol; PPARγ2 mRNA expression was detected with RT-PCR. β-actin mRNA was used as the internal control to evaluate the relative expression level of PPARγ2 gene. The procedures were performed according to the manufacture’s instructions.
Screening of differential genes
The induced cells were washed with PBS, and then Trizol was added. When cell layer was completely dissolved, the sample was transferred into a 1.5 ml of RNAase-free centrifuge tube. Three PCOS-derived samples were marked with experimental group 1, 2, and 3, respectively; and one non-PCOS-derived sample with the control group. Differential genes were detected with human V 2.0 whole genome oligonucleotide microarray (35035 70 mer-Oligo DNAs from human genome bank of Operon Company) provided by Boao Biological Co., Ltd (Beijing, China) as follows.
Double-stranded cDNA synthesis: The dilution of purified poly(A+)RNA control was firstly prepared, then 1st-strand cDNA was synthesized with T7-Oligo (dT) as primer by reverse transcription, followed by the synthesis of 2nd-strand cDNA through primer extension based on E.coli DNA polymerase, finally double-stranded cDNA was purified with cDNA Cleanup Spin Column [MACHEREY-NAGEL (MN)] [Yang et al. Citation2002].
Synthesis of biotin-labeled cRNA: Synthesis of biotin-labeled cRNA was performed with a crystal® cRNA amplification kit. Taking a 0.2 ml-EP tube, 12 μl of double-strand cDNA, 4 μl of 10 × IVT Labeling Buffer, 12 μl of IVT Labeling NTP Mix, and 4 μl of IVT Labeling Enzyme Mix were added into the EP tube, and then DEPC-H2O was also added to reach 40 μl for incubation at 37°C for 16 h. Finally, cRNA was purified with Spin Column for future use.
cRNA fragmentation: A total of 40 μl including 20 μl of cRNA, 8 μl of 5 × Fragmentation Buffer, and 12 μl of DEPC-H2O was incubated at 94°C for 35 min, and then the sample was immediately placed on ice. A total of 2 μl of product underwent formaldehyde denaturing gel electrophoresis to detect fragmented distribution range of cRNA for chip hybridization.
Microarray hybridization: The hybridization fluid was incubated at 99°C for 5 min, and then 200 μl of Pre-Hybridization Mix was added followed by centrifugation at 60 rpm for 10 min at 45°C to remove insoluble matter. Chips and Pre-Hybridization Mix were centrifuged at 60 rpm for 16 h at 45°C.
Cleaning, staining, and scanning of chips: Labeled DNA was dissolved in 80 μl of hybridization solution (3 × SSC, 5 × Denhart’s, 0.2% SDS, and 25% formamide) at 42°C overnight, and then respectively washed with the fluid containing 2 × SSC and 0.2% SDS at 42°C for 5 min, and 0.2 × SSC for 5 min. After drying, chips were scanned with LuxScan 10KA (Beijing Boao Company, Beijing, China). The image signals of chips were changed into digital signals with LuxScan 3.0 image analysis software. The linear correction was performed among chips followed by intra-chip normalization with Lowess method.
Screening standards for differential genes: False-positive rate was controlled within 5%. Compared with controlled sample, if the expression ratios are increased or decreased by more than 2 X, it is considered significant.
Verification of differential genes with RT-PCR
In this study, six genes which had a higher difference multiple, better repeatability, and specific chromosomal localization were validated with Real-time PCR. Of the six genes, five genes were up-regulated and one gene was down-regulated, which was consistent with the results of microarray. Primers were designed with Primer 5.0 ().
Table 3. Genes validated with real-time PCR and primer sequences.
The RT-PCR was performed with a 7700 fluorescent quantitative PCR instrument provided by ABI Company. The RT-PCR was carried out in a volume of 20 μl containing 1 μl of templates, 0.6 μl of upstream primer, 0.6 μl of downstream primer, 0.3 μl of Cap Taq enzyme (5U/μl), 10 μl of 2 × PCR Buffer for EvaGreen, 0.6 μl of 20 × EvaGreen, and water. The reaction conditions were as follows: 95°C for 5 min, 95°C for 15 s, 50–60°C for 15 s, 72°C for 20 s, 76°C for 3 s, 40 cycles. The fluorescent signals were collected in the second step of every cycle. The relative level of gene expression was calculated with 2−ΔΔCt formula.
Declaration of interest
This study was supported by the National Natural Science Foundation of China (NO. 31271605) and the Youth Innovation Fund of the First Affiliated Hospital of Zhengzhou University. The authors have no conflicts of interest. The authors alone are responsible for the content and writing of this paper.
Author contributions
Conceived and designed the study: FW, Y-pS; Performed the study: W-wL; Analyzed the data: H-jK, JL, X-mC; Wrote the manuscript: FW.
Abbreviations | ||
PCOS | = | polycystic ovary syndrome |
hESCs | = | human embryonic stem cells |
NR0B2 | = | nuclear receptor subfamily 0, group B, member 2 |
SHP | = | small heterodimer partner gene |
HNF | = | hepatocyte nuclear factor |
Accession number | ||
GEO number GSE52422 | = |
References
- Azziz, R., Woods, K.S., Reyna, R., Key, T.J., Knochenhauer, E.S., and Yildiz, B.O. (2004) The prevalence and features of the polycystic ovary syndrome in an unselected population. J Clin Endocrinol Metab 89:2745–9
- Båvner, A., Sanyal, S., Gustafsson, J.A., and Treuter, E. (2005) Transcriptional corepression by SHP: molecular mechanisms and physiological consequences. Trends Endocrinol Metab 16:478–88
- Delerive, P., Galardi, C.M., Bisi, J.E., Nicodeme, E., and Goodwin, B. (2004) Identification of liver receptor homolog-1 as a novel regulator of apolipoprotein AI gene transcription. Mol Endocrinol 18:2378–87
- Diamanti-Kandarakia, E., and Piperi, C. (2005) Genetics of polycystic ovary syndrome: searching for the way out of the labyrinthy. Hum Reprod Update 11:631–43
- Diao, F.Y., Xu, M., Hu, Y., Li, J., Xu, Z., Lin, M., et al. (2004) The molecular characteristics of polycystic ovary syndrome (PCOS) ovary defined by human ovary cDNA microarray. J Mol Endocrinol 33:59–72
- Dunaif, A., Wu, X., Lee, A., and Diamanti-Kandarakis, E. (2001) Defects in insulin receptor signaling in vivo in the polycystic ovary syndrome (PCOS). Am J Physiol Endocrinol Metab 281:E392–9
- Enya, M., Horikawa, Y., Kuroda, E., Yonemaru, K., Tonooka, N., Tomura, H., et al. (2008) Mutations in the small heterodimer partner gene increase morbidity risk in Japanese type 2 diabetes patients. Hum Mutat 29:E271–7
- Gupta, R.K., Vatamaniuk, M.Z., Lee, C.S., Flaschen, R.C., Fulmer, J.T., and Matschinsky, F.M. (2005) The MODY1 gene HNF-4alpha regulates selected genes involved in insulin secretion. J Clin Invest 115:1006–15
- Han, H-j., Wei, L-h., Wang, S-m., and Ji, L-n. (2004) Correlation between SHP gene distribution and birth weight. Chinese Journal of Obstetrics and Gynecology 39:491–3
- Han, H-j., Wei, L-h., Wang, S-m., and Ji, L-n. (2005) Association between small heterodimer partner gene and high birth weight infants. Chinese Journal of Perinatal Medicine 8:326–30
- Haouzi, D., Assou, S., Monzo, C., Vincens, C., Dechaud, H., and Hamamah, S. (2012) Altered gene expression profile in cumulus cells of mature MII oocytes from patients with polycystic ovary syndrome. Hum Reprod 27:3523–30
- Huang, X., Hao, C., Shen, X., Liu, X., Shan, Y., Zhang, Y., et al. (2013) Differences in the transcriptional profiles of human cumulus cells isolated from MI and MII oocytes of patients with polycystic ovary syndrome. Reproduction 145:597–608
- Hung, C.C., Farooqi, I.S., Ong, K., Luan, J., Keogh, J.M., and Pembrey, M. (2003) Contribution of variants in the small heterodimer partner gene to birthweight, adiposity, and insulin levels: mutational analysis and association studies in multiple populations. Diabete 52:1288–91
- Lefebvre, A.M., Peinado-Onsurbe, J., Leitersdorf, I., Briggs, M.R., Paterniti, J.R., and Fruchart, J.C. (1997) Regulation of lipoprotein metabolism by thiazolidinediones occurs through a distinct but complementary mechanism relative to fibrates. Arterioscler Thromb Vasc Biol 17:1756–64
- Li, P.F., Wang, F., Kong, H.J., Zhao, F., Bai, A.H., Chen, X.M., et al. (2012) Establishment of polycystic ovary syndrome-derived human embryonic stem cell lines. Gynecol Endocrinol 28:25–8
- Mitchell, S.M., Weedon, M.N., Owen, K.R., Shields, B., Wilkins-Wall, B., Walker, M., et al. (2003) Genetic variation in the small heterodimer partner gene and young-onset type 2 diabetes, obesity, and birth weight in U.K. subjects. Diabetes 52:1276–9
- Nishigori, H., Tomura, H., Tonooka, N., Kanamori, M., Yamada, S., Sho, K., et al. (2001) Mutations in the small heterodimer partner gene are associated with mild obesity in Japanese subjects. Proc Natl Acad Sci USA 98:575–80
- Nishizawa, H., Yamagata, K., Shimomura, I., Takahashi, M., Kuriyama, H., Kishida, K., et al. (2002) Small heterodimer partner, an orphan nuclear receptor, augments peroxisome proliferator-activated receptor gamma transactivation. J Biol Chem 277:1586–92
- Seol, W., Choi, H.S., and Moore, D.D. (1996) An orphan nuclear hormone receptor that lacks a DNA binding domain and heterodimerizes with other receptors. Science 272:1336–9
- Venkatesan, A.M., Dunaif, A., and Corbould, A. (2001) Insulin resistance in polycystic ovary syndrome: progress and paradoxes. Recent Prog Horm Res 56:295–308
- Wang, F., Kong, H.J., Kan, Q.C., Liang, J.Y., Zhao, F., Bai, A.H., et al. (2012) Analysis of blastocyst culture of discarded embryos and its significance for establishing human embryonic stem cell lines. J Cell Biochem 113:3835–42
- Watanabe, M., Houten, S.M., Wang, L., Moschetta, A., Mangelsdorf, D.J., Heyman, R.A., et al. (2004) Bile acids lower triglyceride levels via a pathway involving FXR, SHP, and SREBP-1c. J Clin Invest 113:1408–18
- Yang, Y.H., Dudoit, S., Luu, P., Lin, D.M., Peng, V., Ngai, J., et al. (2002) Normalization for cDNA microarray data: a robust composite method addressing single and multiple slide systematic variation. Nucleic Acids Res 30:e15