Abstract
Using the rabbit as an animal model, this study evaluated the long-term effect of silver nanoparticles (NPs) administered intravenously (0.6 mg/kg bw) on reproductive activity and sperm quality. Semen analysis was performed by optical microscopy and sperm motility evaluation by computer assisted sperm analyzer (CASA). Mitochondria oxygen consumption, light and transmission electron microscopy of rabbit testis and ejaculated sperm were also carried out. Throughout the experiment NP-treated rabbits showed higher seminal reactive oxygen species (ROS), less motile sperm, and lower curvilinear velocity and oxygen consumption than control animals. In contrast, libido, serum testosterone, sperm concentration, and semen volume were hardly affected by NPs. Transmission electron microscopy analysis did not show any evident morphological damage in testes; however, Ag NPs are visible in spermatids and ejaculated sperm. These preliminary results show that Ag NPs can reach the testes, compromising sperm motility, sperm speed, and acrosome and mitochondria shape and function.
Introduction
Exposure to nanomaterials has greatly increased because of the expansion of nanotechnology. As a consequence of this widespread use, nanoparticles (NPs) are likely to be ubiquitous in the environment and can enter the human body by inhalation, ingestion, skin adsorption, and, when used for biomedical applications, by intravenous injection [Cattaneo et al. Citation2010]. Nanoparticles differ from bulk material in their chemical and physical properties and may have different toxic profiles [Gornati et al. Citation2009]. For example, air pollution studies have shown that particles that are not toxic in the micrometer range may become toxic in the nanometer range, raising special concerns for human health [Warheit et al. Citation2007].
Most nanotoxicological research is performed in vitro and, consequently, cannot consider tissue distribution, accumulation in selected organs, clearance or diffusion across biological barriers such as the blood-brain barrier and blood-testis barrier (BTB). Kwon and coworkers [Citation2008] stated that inhaled magnetic NPs penetrate the testis despite the BTB. Intravenously injected Au [De Jong et al. Citation2008] and Ag [Lankveld et al. Citation2010] NPs have also been shown to reach the testis. Some damaging effects on spermatogenesis and histopathological changes in the testes have also been observed in mouse offspring after maternal subcutaneous injections of TiO2 NPs [Ema et al. Citation2010]. Moreover, Asare and colleagues [Citation2012] have recently shown that AgNPs are particularly cytotoxic and genotoxic for testicular cells. Of course, all these data taken together raise concerns about the potential risks for reproductive health of using NPs. Since little information is available regarding the in vivo toxic effects of metal NPs on the male reproductive apparatus and sperm physiology, further studies using well-characterized materials are needed to evaluate the potential reproductive effect of nanomaterials.
Silver nanoparticles are widely employed in catalysis, optics, and biosensing; however, their most common use is in medicine and hygiene as biocides due to the notable activity against bacteria, fungi, and viruses [Silver et al. Citation2006]. Silver is more toxic to microorganisms than other metals; at the same time, it exhibits a relatively low toxicity and a low propensity to induce microbial resistance [Kim et al. Citation2007]. Undoubtedly, AgNPs represent a new generation of antimicrobials [Rai et al. Citation2009], exerting more efficient antimicrobial activity than silver ions and other silver salts. Silver nanoparticles can be used in several medical devices (catheters, bone and dental cements, anticonceptional foams) to prevent microbial infections [Chen et al. Citation2010].
As AgNPs are by far the most common nanoscale product, their release into the environment and a reasonable possibility of coming into contact with them is expected. Therefore, a better understanding of AgNP toxicology is needed to correctly evaluate the hazards and to responsibly balance the risks and benefits.
In this context, we have undertaken the current investigation to evaluate the effect of AgNPs on the main reproductive functions and sperm quality. We have chosen the rabbit as a model organism for the possibility to easily collect ejaculated semen for a continuous assessment of sperm traits without having to sacrifice the animal [Foote and Carney Citation2000].
Results
Health and semen characteristics after NP exposure
No deaths related to AgNPs occurred during the trial period. The administration of NPs had no effect on the body weight (control 3.79 kg vs. AgNPs 3.80 kg) or on rectal temperature (control 39.05°C vs. AgNPs 39.4°C). The baseline semen characteristics of rabbit show a standard semen quality (sperm concentration: 385 ± 29 × 106/mL; motile cells 78 ± 2%; curvilinear velocity (VCL) 218 ± 38 μm/sec −37). Seven days after i.v. injection, a lower percentage of motile cells and VCL, which is a measure of sperm vigor, were found in the rabbits treated with NPs than in the control rabbits (). This negative effect was partly abated within 42 days; but thereafter sperm traits progressively declined until the end of experiment.
Table 1. Main seminal characteristics of rabbit semen before and at different time periods VCL in control and AgNP treated bucks.
The oxygen consumption of sperm was deeply affected by NP administration and followed a trend similar to that observed for the kinetic traits of semen: a short-term effect (7–14 days) followed by a partial recovery (42 days) and with a further decline in the long term (63–126 days). Seminal reactive oxygen species (ROS) and sperm concentration were affected only in the short term after NP treatment (7 and 21 days); successively, the values were similar to those of the controls. Silver nanoparticles reduced the percentage of normal sperm, whereas acrosome-reacted sperm increased at almost every sampling time. Semen volume, libido, and blood testosterone were not affected by the administration of AgNPs.
Testis and sperm ultrastructure
The testicular tissue from control rabbits did not differ from the tissue of NP-treated bucks at any observation point. Light microscopy of AgNP-treated rabbits showed tubules with a normal germinal epithelium at the different collection times (data not shown). Even 126 days after AgNP administration the testis was normal. No histopathological changes were observed. Sertoli and Leydig cells had also normal morphology (data not shown). During the study period spermatogonia, spermatocytes, and spermatids were present and they exhibited normal morphology. However, the presence of NPs was clearly highlighted in the testis ultrasection. shows the cytoplasm of a Sertoli cell rich in NPs and an elongated spermatid with NPs into the nucleus. These particles were absent in the controls.
Figure 1. Transmission electron microscopy (TEM) images of rabbit testicular tissue and ejaculated sperm (day 21 from nanoparticles (NPs) injection). A) Particles were evident in testicular tissue (arrows) and inside the cytoplasm of Sertoli cell and into the normal nucleus of an elongated spermatid. B–E) In ejaculated sperm, aggregates of NPs (arrows) were inside the cytoplasmic residue (B) and the sections of nuclei and axoneme (C). Sometimes NPs were localized in the acrosome that appeared broken and partially reacted (D) or swollen (E) and inside the mitochondria (E). A: acrosome; N: nucleus; Ch: chromatin; CR: cytoplasmic residue; M: mitochondria. A, C) Bar 1 µm; B, D, E) Bar 300 nm.
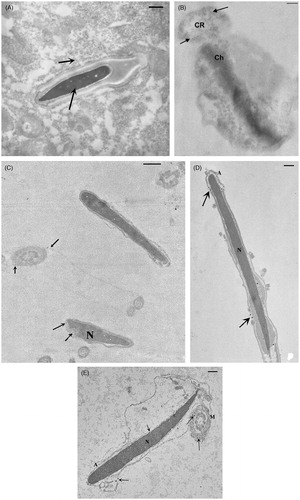
Ejaculated sperm from AgNP-treated rabbits and controls were analyzed by transmission electron microscopy (TEM) at the different days ( and ). Ultrastructural sperm damage, mainly concerning the acrosome and mitochondria integrity, was observed in AgNP-treated rabbits at every sampling date except day 42. The presence of NPs could be seen in semen samples in cytoplasmic residues () but also in the sections of nucleus, acrosome, and axoneme (). Sometimes, acrosomes appeared swollen, empty, or absent () and mitochondria swollen () and the cristae were not clearly detectable. The plasma membrane, axoneme assembly, and nuclear shape were rarely altered. Control rabbits showed a vast majority of sperm with a normal ultrastructure and particles were never observed in semen samples.
Discussion
The effect of nanomaterials on reproductive function is becoming an important part of nanoscience research [Ema et al. Citation2010]. The in vitro studies revealed that NPs impaired mitochondrial and membrane functions of spermatogonial stem cells, induced necrosis and apoptosis [Braydich-Stolle et al. Citation2005], damaged primary testicular cells [Asare et al. Citation2012], and reduced the motility of sperm [Wiwanitki et al. Citation2009] and the production of steroid hormones by Leydig cells [Kim et al. Citation2006].
The in vivo studies of engineered NPs confirmed the in vitro results and indicated that the harmful effect is related to their chemical composition, size, dose, means of administration, and duration of exposure.
Some authors [Komatzu et al. Citation2008; Morishita et al. Citation2012] showed that magnetic and silica NPs can penetrate the BTB and become distributed in the gonads without apparent tissue alteration. Other in vivo studies have demonstrated that nanosize metals can accumulate in the testis, impairing endocrine and reproductive functions [Thompson and Bannigan Citation2008]. Although several reports documented an in vivo toxicity of different nanoparticles, the effect of silver nanoparticles is currently unclear and only limited data is available on the effect of AgNPs on ejaculated sperm and male fertility. Accordingly, we investigated the long-term effect of AgNPs on the main reproductive functions of a mammal model (rabbit). Using this model, we can conduct longitudinal studies with a continuous assessment of the semen traits without having to sacrifice the animals. The AgNPs were intravenously administered to rabbit bucks at a dose of 0.6 mg/kg of body weight without any visible effect on the health status of rabbits. The dose is comparable to that suggested [Lankveld et al. Citation2010], where 1 mg/kg of body weight did not cause significant toxic effects. We used intravenous administration as a route of exposure to bypass skin, intestinal, or pulmonary absorption that, although more realistic, would complicate data interpretation. Moreover, the intravenous administration of NPs is the most relevant method to simulate biomedical exposure by humans. Gromadzka-Ostrowska et al. [Citation2012] intravenously injected rats with 5 and 10 mg/kg bw and calculated that the dose of AgNPs used as a drug (Abraxane®) in the therapy of breast cancer is about 7 mg/kg bw. Thus, the dose of AgNPs used in this study is ten times smaller than the NP doses used in the clinic.
In this study, we showed that AgNPs reproductive functions (sperm velocity, acrosome status, oxygen consumption, and ROS production) without producing significant variations of semen volume, concentration, libido, testosterone, and evident morphological alteration of Sertoli and Leydig cells. Komatzu et al. [Citation2008] using different nanomaterials (TiO2, carbon black, and diesel exhaust NPs) found that NPs were taken up by Leydig cells and affected their viability, proliferation, and gene expression.
Spermatogenesis occurs in a safe environment because of the BTB which is formed by adjacent Sertoli cells near the basement membrane, and should prevent harmful substances from reaching developing germ cells, most notably postmeiotic spermatids [Su et al. Citation2011].Theoretically, NPs should not penetrate the BTB, but TEM analysis showed that AgNPs cross the BTB and can be found in spermatids and in the ejaculated sperm, confirming what has been reported for other NPs [Yoshida et al. Citation2009]. How the NPs can penetrate the BTB is a complex and debated topic [Zhou and Yang Citation2012]. Park et al. [Citation2010] in mice showed that small-sized AgNPs (22 and 42 nm) caused an accumulation of Ag within the testes and NPs possibly penetrated the BTB, whereas large-sized AgNPs (323 nm) did not. Interestingly, inflammatory cytokines, B-lymphocyte distribution, and IgE production increased when small-sized AgNPs were administered. These results suggest that penetration of the BTB may not be solely dependent on particle size but may also involve an inflammatory response that weakens the integrity of the BTB. Because of inflammatory responses, the size of the BTB intracellular gap might become larger after treatment with NPs. Such an assumption is consistent with our results and explains why NP particles can penetrate the BTB whereas larger nanoparticles still keep out because the size is larger than the size of the BTB gaps.
Whether additional NPs arrive in the sperm during the transit in the epididymis and/or via accessory glands still remains to be determined. However, human sperm in vitro exposed to high concentrations of AgNP (125–500 µM), showed that the first hypothesis should be prevalent since NPs required a relatively short lag time (60–120 minutes) to affect kinetic traits [Moretti et al. Citation2013]. Although our data do not reveal in detail how AgNPs affect sperm physiology we can hypothesize different mechanisms of action on the basis of time-dependent effects.
Due to the early occurrence of sperm damage, it is reasonable to hypothesize that AgNPs impacted mainly on epididymis rather than on testes. Similarly, Gromadzka-Ostrowska et al. [Citation2012] demonstrated, in rats, a stronger impact of AgNPs on the epididymis than on testes which increase free radical production and reduce antioxidant potential in the epididymis [Foster et al. Citation2010]. To the best of our knowledge, there is no detailed information on the effects of a low AgNPs dose on ejaculated sperm, testes, epididymis, and on other accessory organs.
The acrosome cap appears as the most physiologically fragile organelle susceptible to damage and mitochondria, as confirmed by the lower oxygen consumption. Recent reports have identified the mitochondria [Braydich-Stolle et al. Citation2005; Hussain et al. Citation2005] as primary targets of AgNPs in other cell lines such as liver and neuroendocrine cells. Mitochondria are the main source of energy in the sperm; therefore, the reduction in kinetic traits is likely due to the harmful effect of silver NPs on sperm mitochondria. AshaRani et al. [Citation2009] proposed a mechanism of action for AgNPs involving disruption of the mitochondrial respiratory chain and the interruption of ATP synthesis [Asare et al. Citation2012]. In agreement with us, AgNPs were reported to act via ROS production and depletion of endogenous antioxidants, suggesting that oxidative stress might mediate its cytotoxicity.
It is widely known that sperm are very sensitive to excessive ROS production due to the high levels of unsaturated membrane and to the limited antioxidant power of the seminal plasma and of the spermatozoon itself. Proteins and enzymes with thiol groups (glutathione, thioredoxin, SOD, and thioredoxin peroxidase) are key components of the antioxidant defense of sperm and AgNPs may lessen their impact and increase ROS [Almofti et al. Citation2003; Hussain et al. Citation2005].
After 7 weeks from NP inoculation there was a partial recovery of semen traits. This can be explained by the fact that the resistance to harmful substances is affected by the stage of sperm differentiation: during the leptotene-zygotene phase sperm is more susceptible to exogenous substances [Liew et al. Citation2007] and is probably more resistant in other ones. In comparison, the impairment of reproductive function over the long term suggests that some injuries occurred during the epididymal transport and storage because the examination of testis showed a quite normal spermatogenesis.
In conclusion, it seems that the BTB of the rabbit male reproductive system does not guarantee complete sealing and permits the passage of AgNPs from blood to sperm and does not protect male gametes from their noxious effects. How these NPs might alter BTB function still remains to be determined. Alternatively, the early occurrence and the persistence of the noxious effect of NPs could be explained postulating a critical role for the epididymis, but the mechanism involved has not been analyzed and deserves further study. This is a major concern for the reproductive health of humans considering the postulated use of AgNPs which can access sperm via a variety of biomedical products such as contraceptive devices and other female hygiene items, which may result in fertility problems and warrants further investigation. Naturally, a single acute dose may not reflect what happens in cases of chronic toxicity and future studies should be performed and sperm function assessed.
Materials and Methods
Chemicals and preparation of NPs and ion mixtures
Stock solutions and samples were diluted with deionized water. The AgNPs were kindly provided by Cericol Colorobbia Research Center, Sovigliana Vinci, Firenze (Italy). The NPs suspensions were prepared by polyol-mediated synthesis using a bottom-up approach and an organometallic precursor of silver. By employing this procedure, metal NPs can be dispersed in water by means of a nucleation of metallic salts. The process involves an initial phase in which reagents are added in a high-temperature environment of a reaction containing a solvent, such as glycolic solvent medium, with a high capacity for complexing. The next step is the crystal growth phase, performed by mixing the reagents, including surfactants such as polyvinylpyrrolidone (PVP), at room temperature. The obtained NPs are stable for long periods of time without showing aggregation or changes in chemical characteristics. The concentration of the stock solutions of NPs was 1% in distilled H2O and PVP (<1%) as stabilizing agent.
To determine the NPs morphology, after a 10 min sonication, a drop of NP suspension was placed on a formvar-carbon coated grid. After 45 s the grid was dried with blotting paper and examined at the electron microscope (). The hydrodynamic dimensions were determined by Dynamic Light Scattering (Nano-S model by Malvern Instrument Ltd, UK) and the average size of 45 nm AgNPs resulted. Z-potential was −20 mV.
Animals, farming conditions, and approval of the experimental procedures
New Zealand White male rabbits selected for their high semen quality and high repeatability were enrolled in this study. Rabbits allow easy collection of ejaculated semen with a continuous assessment of the semen traits from the same subject [Foote and Carney Citation2000]. Semen was collected with an artificial vagina filled up with warm water at about 38°C.
Animals were housed in individual cages with a photoperiod of 16 h light/day, at an intensity of 40 lux, and at temperatures ranging from 16°C to 25°C. Fresh water was constantly available. They were fed ad libitum with a standard diet composed of dehydrated alfa meal (40%), soybean meal (18%), barley (30%), wheat bran (10%), and minerals and vitamins (2%). Feed, purchased from Mignini s.p.a. (Petrignano, Italy) as pellet chow, had the following chemical composition: 17.5% crude protein, 15.5% crude fiber, 2.5% fat, and 6.2% ash. At 140 days of age, rabbits were trained for semen collection for about 2 w. During the training period, libido (defined as the time between the introduction of a female rabbit and the ejaculation) and several sperm traits of each rabbit buck were analyzed. This study was conducted in accordance with the Guiding Principles in the Use of Animals in Toxicology and was approved by the by the Animal Ethics Monitoring Committee of the University of Siena (CEL AOUS 21.10.09).
Study design
To investigate the effect of AgNPs on the reproductive traits of rabbit, 16 bucks were homogeneously assigned to two experimental groups (eight animals/treatment): the Treated group, treated with 0.6 mg/kg bw AgNPs, and the Control group, treated with physiological saline (0.9 % w/v NaCl). For both treatments, a volume of 2.0 mL was intravenously injected into the marginal vein of the right ear.
Semen samples were collected weekly from each rabbit buck before the treatment for 126 d. Semen traits were studied to determine spermiotoxicity of metal NPs as described below. During the trial, the health status of rabbits was assured daily by monitoring feeding and drinking behavior, live weight, and body temperature. After 21, 42, 84, and 105 d one rabbit/group was killed and at 126 days all the rabbits were killed and the reproductive organs removed and evaluated by TEM and light-microscopy.
Semen collection and sperm quality assessment
Semen samples were collected by means of an artificial vagina heated to 38°C with water, immediately transferred to the laboratory [Boiti et al. Citation2005], and subjected to the following analyses: 1) semen volume (ml) which was determined by graduate tubes; 2) sperm concentration (number of sperm × 106/ml) which was measured by means of a Thoma–Zeiss cell counting chamber with a 40x objective; 3) live spermatozoa (%) which were measured using the eosin/nigrosin exclusion test; and 4) kinetic characteristics which were analyzed by a computer assisted sperm analyzer (CASA) (model ISAS®4.0, Valencia, Spain) after appropriate dilution (1/20) with a modified Tyrode’s Albumin Lactate Pyruvate buffer at pH 7.4 and 296 mOsm/kg. This system consisted of a negative phase-contrast optic system (Olympus CH-2) equipped with a CCD Sony camera. The set-up parameters were established previously and the acquisition rate was set at 100 Hz [Castellini et al. Citation2011]. For each sample, two drops and six microscopic fields were analyzed for a total of 300 spermatozoa. Recorded sperm motion parameters were motility rate (percentage of motile sperm/total sperm) and VCL (µm/s, the sum of the incremental distances moved by the sperm in each frame along the sampled path divided by time).
The acrosome status of the spermatozoa was evaluated using a chlortetracycline (CTC) fluorescence assay [Kaul et al. Citation2001]. Briefly, 100 µL semen in CTC stock solution (100 µL) were fixed by adding 8 µL of 12.5% paraformaldehyde in 0.0.6 mg/kg Tris HCl buffer, pH 7.5. Slides were prepared by placing 10 µL of the fixed solution with one drop of 0.22 1,4-diazabicyclo[2.2.2]octane dissolved in glycerol/phosphate buffer saline. The slides were examined under an epifluorescence microscope (OLYMPUS - CH2 excitation filter 335–425 nm) and 300 spermatozoa per slide were counted.
Oxygen consumption
Oxidative phosphorylation and mitochondrial functionality were estimated by sperm oxygen consumption [Ferramosca et al. Citation2008]. Semen samples were centrifuged at 500 × g for 10 min and sperm were collected by resuspended pellets in isotonic salt medium (75 mM sucrose, 100 mM KCl, 2 mM K2HPO4, 0.6 mg/kg MgCl2, 20 mM Tris, pH 7,4). Sperm were then subjected to permeabilization with digitonin added slowly to a final concentration of 0.1 mg/mg protein. After incubation for 10 min at 0°C, sperm were recovered by centrifugation (250 × g for 10 min). Respiration (6 × 107 cells) was evaluated in 0.5 ml of 120.6 mg/kg KCl, 2 mM K2HPO4, 0.025% BSA, 20 mM Hepes, pH 7.4, and temperature equilibrated at 37°C for 15 min prior to adding the substrates (5 mM succinate and 0.1 mM ADP). The rate of oxygen consumption was determined using a fiber optic oxygen monitor (Instech, USA) equipped with a probe fitted into a thermostatic water-jacketed chamber with a capacity of 400 µl. An electromagnetic stirrer and a bar flea were used to mix the incubation medium. Oxygen was sensed by fluorescence quenching of an indicator dye trapped in a matrix at the tip of the probe as previously described [Macchioni et al. Citation2011]. The oxygen content of the starting medium was normalized, assuming a concentration of ∼190 nmol/ml at 37°C.
Hormonal assays
Testosterone plasma concentrations were assayed in duplicate samples using the Test-RIA-CT kit (DIAsource ImmunoAssays, Louvain-la-Neuve, Belgium). The detection limit was 0.05 pg/ml and the intra- and inter-assay coefficients of variation were <3.3 and <4.8%, respectively.
Radical oxygen species in seminal plasma
Radical oxygen species in the seminal plasma was assessed with a colorimetric assay (d-ROS, Diacron Grosseto, Italy) measured by a spectrophotometer (Shimadzu, model UV 5550, Kyoto, Japan), and expressed as mg hydrogen peroxide/100 mL.
Light and transmission electron microscopy of rabbit testis and ejaculated sperm
Light microscopy of rabbit testicular tissue
Rabbit testicular tissue was obtained from rabbits killed at days 21, 42, 84, 105, and 126 after NP treatment and from controls. Rabbit tissue was fixed in 10% buffered neutral formalin and embedded in paraffin for histology; 4-μm-thick sections of each specimen were stained with hematoxylin and eosin.
TEM of rabbit sperm and testicular tissue
Three small fragments (1 × 1 mm) were treated for conventional TEM. Samples were fixed in cold Karnovsky fixative and maintained at 4°C for 2 h. Fixed semen was washed in 0.1 mol/l cacodylate buffer (pH 7.2) for 12 h, postfixed in buffered 1% osmium tetroxide for 1 h at 4°C, then dehydrated and embedded in Epon Araldite. Ultra-thin sections, stained with uranyl acetate and lead citrate, were observed and photographed with a Philips EM208 TEM (Philips Scientifics, Eindhoven, The Netherlands).
Ejaculated sperm were obtained as described above from NP-treated and control rabbits. Samples at days 0, 7, 21, 42, 84, 105, and 126 were treated as reported for testicular tissue. Major submicroscopic sperm characteristics were recorded by trained examiners who were blinded to the experiment. A minimum of 300 sperm sections were analyzed for each sample; a sperm was considered altered when affected by anomalies related to the acrosome (altered, absent, reacted, empty, or swollen), the nucleus (altered), the chromatin (disrupted), the axoneme (coiled or disorganized), the mitochondria (swollen and badly assembled), or the plasma membrane (broken).
Statistics
All the seminal traits were statistically analyzed with a linear model comprising the effect of collection data (0-7-21-42-63-84-105-126 d), treatment (control vs. NPs) and the repeated effect of animals [StataCorp Citation2009]. The significance of difference were evaluated with t test (p < 0.05).
Declaration of interest
The authors declare that there is no conflict of interest that could be perceived as prejudicial to the impartiality of the present research. This research did not receive any specific grants from any funding agencies in the public, commercial, or not-for-profit sectors.
Author contributions
All the authors gave substantial contributions to research design, or the drafting of the paper, or revising it critically, and approval of the submitted version. In particular: Research design, rabbits managing, wrote the paper, data interpretations: CC; Rabbit managing, acquisition, analysis or interpretations of semen collection data: SR. Rabbit managing, acquisition, analysis or interpretations of semen collection data: SM; Research design, analysis or interpretations of semen collection data, hormonal and ROS assays: GB; Analysis or interpretations of oxygen consumption data: LM. Data interpretations, light and electron microscopy analysis: EM; Research design, wrote the paper, data interpretations, light and electron microscopy analysis: GC.
Abbreviations | ||
NPs | = | nanoparticles |
ROS | = | reactive oxygen species |
BTB | = | blood-testis barrier |
VCL | = | curvilinear velocity |
TEM | = | transmission electron microscopy |
PVP | = | polyvinylpyrrolidone |
CASA | = | computer assisted sperm analyzer |
CTC | = | chlortetracycline |
Acknowledgments
The authors thank Dr Ivan Mičetić of ECSIN for help with TEM analysis of AgNPs.
References
- Almofti, M.R., Ichikawa, T., Yamashita, K., Terada, H., and Shinohara, Y. (2003) Silver ion induces a cyclosporine a-insensitive permeability transition in rat liver mitochondria and release of apoptogenic cytochrome c. J Biochem 134:43–9
- Asare, N., Instanesa, C., Sandberga, W.J., Refsnesa, M., Schwarzea, P., Kruszewskib, M., et al. (2012) Cytotoxic and genotoxic effects of silver nanoparticles in testicular cells. Toxicology 291:65–72
- AshaRani, P.V., Prakash Hande, M., and Valiyaveett, S. (2009) Anti-proliferative activity of silver nanoparticles. BMC Cell Biology 10:65
- Boiti, C., Castellini, C., Besenfelder, U., Theau-Clément, M., Liguori, L., Renieri, T., et al. (2005) Guidelines for the handling of rabbit bucks and semen. World Rabbit Sci 13:71–91
- Braydich-Stolle, L., Hussain, S., Schlager, J.J., and Hoffman MC. (2005) In vitro cytotoxicity of nanoparticles in mammalian germline stem cells. J Toxicol Sci 88:412–19
- Castellini, C., Dal Bosco, A., Ruggeri, S., and Collodel, G. (2011) What is the best frame rate for evaluation of sperm motility in different species by Computer-Assisted Sperm Analysis. Fertil Steril 96:24–7
- Cattaneo, A.G., Gornati, R., Sabbioni, E., Chiriva-Internati, M., Cobos, E., Jenkins, M.R., et al. (2010) Nanotechnology and human health: Risks and benefits. J Appl Toxicol 30:730–44
- Chen, L., Zheng, L., Lv, Y., Liu, H., Wang, G., Ren, N., et al. (2010) Chemical assembly of silver nanoparticles on stainless steel for antimicrobial applications. Surf Coat Technol 204:3871–5
- De Jong, W.H., Hagens, W.I., Krystek, P., Burger, M.C., Sips, A.J.A.M., and Geertsma, R.E. (2008) Particle size-dependent organ distribution of gold nanoparticles after intravenous administration. Biomaterials 29:1912–19
- Ema, M., Kobayashi, N., Naya, M., Hanai, S., and Nakanishi, J. (2010) Reproductive and developmental toxicity studies of manufactured nanomaterials. Reprod Toxicol 30:343–52
- Ferramosca, A., Focarelli, R., Piomboni, P., Coppola, L., and Zara, V. (2008) Oxygen uptake by mitochondria in demembranated human spermatozoa: A reliable tool for the evaluation of sperm respiratory efficiency. Int J Androl 31:337–45
- Foote, R.H., Carney, E.W. (2000) The rabbit as a model for reproductive and developmental toxicity studies. Reprod Toxicol 14:477–93
- Foster, W.G., Maharaj-Briceño, S., and Cyr, D.G. (2010) Dioxin-induced changes in epididymal sperm count and spermatogenesis. Environ Health Perspect 118:458–64
- Gornati, R., Papis, E., Di Gioacchino, M., Sabbioni, E., Dalle Donne, I., Milzani, A., et al. (2009) In vivo and in vitro models for nanotoxicology testing. In Nanotoxicity: From in vivo and in vitro models to health risks. Sahu, S.C. and Casciano, D.A., ed. Chichester, UK: John Wiley and Sons Ltd, Ch 15
- Gromadzka-Ostrowska, J., Dziendzikowska, K., Lankoff, A., Dobrzyńska, M., Instanes, C., Brunborg, G., et al. (2012) Silver nanoparticles effects on epididymal sperm in rats. Toxicol Lett 214:251–8
- Hussain, S.M., Hess, K.L., Gearhart, J.M., Geiss, K.T., and Schlager, J.J. (2005) In vitro toxicity of nanoparticles in BRL 3A rat liver cells. Toxicol In Vitro 19:975–83
- Kaul, G., Sharma, G.S., Singh, B., and Gandhi, K.K. (2001) Capacitation and acrosome reaction in buffalo bull spermatozoa assessed by chlortetracycline and pisum sativum agglutinin fluorescence assay. Theriogenology 55:1457–68
- Kim, J.S., Kuk, E., Yu, K.N., Kim, J.H., Park, S.J., Lee, H.J., et al. (2007) Antimicrobial effects of silver nanoparticles. Nanomedicine 3:95–101
- Kim, J.S., Yoon, T.J., Yu, K.N., Kim, B.G., Park, S.J., Kim, H.W., et al. (2006) Toxicity and tissue distribution of magnetic nanoparticles in mice. Toxicol Sci 89:338–47
- Komatzu, T., Masako, T., Kubo-Irie, M., Shimizu, T., Suzuki, K.I., Nihei, Y., et al. (2008) The effects of nanoparticles on mouse testis Leydig cells in vitro. Toxicol in Vitro 22:1825–31
- Kwon, J.T., Hwang, S.K., Jin, H., Kim, D.S., Minai-Tehrani, A., Yoon, H.J., et al. (2008) Body distribution of inhaled fluorescent magnetic nanoparticles in the mice. J Occup Health 50:1–6
- Lankveld, D.P.K., Oomen, A.G., Krystek, P., Neigh, A., Troost-de Jong, A., Noorlander, C.W., et al. (2010) The kinetics of the tissue distribution of silver nanoparticles of different sizes. Biomaterials 31:8350–61
- Liew, S.H., Meachem, S.J., and Hedger, M.P. (2007) A stereological analysis of the response of spermatogenesis to an acute inflammatory episode in adult rats. J Androl 28:176–85
- Macchioni, L., Davidescu, M., Mannucci, R., Francescangeli, E., Nicoletti, I., Roberti, R., et al. (2011) H2O2 disposal in cardiolipin-enriched brain mitochondria is due to increased cytochrome c peroxidase activity. Biochim Biophys Acta 1811:203–8
- Moretti, E., Terzuoli, G., Renieri, T., Iacoponi, F., Castellini, C., Giordano, C., et al. (2013) In vitro effect of gold and silver nanoparticles on human spermatozoa. Andrologia 45:392–6
- Morishita, Y., Yoshioka, Y., Satoh, H., Nojiri, N., Nagano, K., Abe, Y., et al. (2012) Distribution and histologic effects of intravenously administered amorphous nanosilica particles in the testes of mice. Biochem Biophys Res Commun 420:297–301
- Park, E.J., Bae, E., Yi, J., Kim, Y., Choi, K., Sang Hee Lee, S.H., et al. (2010) Repeated-dose toxicity and inflammatory responses in mice by oral administration of silver nanoparticles. Environ Toxicol Pharmacol 30:162–8
- Rai, M., Yadav, A., and Gade, A. (2009) Silver nanoparticles as a new generation of antimicrobials. Biotechnol Adv 27:76–83
- Silver, S., Phung, L.T., and Silver, G. (2006) Silver as biocides in burn and wound dressings and bacterial resistance to silver compounds. J Ind Microbiol Biotechnol 33:627–34
- StataCorp (2009) Stata Statistical Software: Release 11. College Station, TX: StataCorp LP
- Su, L., Mruk, D.D., and Cheng, C.Y. (2011) Drug transporters, the blood-testis barrier, and spermatogenesis. J Endocrinol 208:207–23
- Thompson, J., Bannigan, J. (2008) Cadmium: Toxic effects on the reproductive system and the embryo. Reprod Toxicol 25:304–15
- Warheit, D.B., Born, P.J., Hennes, C., and Lademann, J. (2007) Testing strategies to establish the safety of nanomaterials: Conclusions of an ECETOC workshop. Inhal Toxicol 19:631–43
- Wiwanitki, V., Sereemaspun, A., and Rojanathanes, R. (2009) Effect of gold nanoparticles on spermatozoa: The first world report. Fertil Steril 91:7–8
- Yoshida, S., Hiyoshi, K., Ichinose, T., Takano, H., Oshio, S., Sugawara, I., et al. (2009) Effect of nanoparticles on the male reproductive system of mice. Int J Androl 32:337–42
- Zhou, L., Yang, W.X. (2012) Nanoparticles and spermatogenesis: How do nanoparticles affect spermatogenesis and penetrate the blood–testis barrier. Nanomedicine 7:579–96