Abstract
The understanding of the early mammalian development is a prerequisite for the advancement of in vitro fertilization and improvement of derivation and culturing of embryonic stem cells. While, whole genome transcriptomic analysis on bovine blastocysts has identified genes active in early development, little information is available about the protein complement of early embryos. Modern, sensitive proteomic technology (nano HPLC tandem mass spectrometry) allowed us to describe the proteome of the scarce blastocoel fluid and cell material of expanded bovine blastocysts isolated by micromanipulation. From two independent replicates, 23 proteins were identified in the blastocoel fluid while 803 proteins were identified in the remaining cell material. The proteins were grouped into categories according to their gene ontology (GO) terms by which proteins involved in cell differentiation, cell proliferation, development, and reproduction could be derived. Proteins classified in these categories could be candidates for further functional studies to understand pluripotency and early mammalian development.
Introduction
The mammalian blastocyst is the early unimplanted embryo consisting of a spherical and fluid filled aggregation of 50–140 cells [Hardy et al. Citation1989]. It consists of an outer layer of differentiated trophectoderm (TE) cells that gives rise to placental tissue as well as an undifferentiated inner cell mass (ICM), which gives rise to the developing embryo [Pedersen et al. Citation1986]. The function and composition of the blastocoel fluid, bathing both the TE and ICM, remains largely unknown. Originally, it was suggested that the cavity solely provides a compartment for migration of cells in the process of gastrulation [Stableford Citation1949]. However, later studies demonstrated that the blastocoel fluid may directly support the development of the blastocyst. In an elegant study, Pedersen and Spindle injected donor 8-cells and morulas into the blastocoel cavity of giant chimeric blastocysts formed by the aggregation of 8–10 embryos at the 4–8-cell stage, to show that the injected embryos develop into normal blastocysts with their own blastocoel cavity [Pedersen and Spindle Citation1980]. This finding strongly supports the suggestion that the blastocoel fluid plays a critical role in supporting cellular processes during pre-implantation development other than merely providing a compartment for cell migration.
The ICM from a series of mammalian species can be isolated to form an undifferentiated cell line of embryonic stem cells (ESC) with the potential to develop in vitro into any cell type present in the adult body. Due to the far-reaching potential of stem cells in regenerative medicine [Mountford Citation2008], human ESC have gained growing interest ever since the first derivation of a human ESC cell line in 1998 [Thomson et al. Citation1998] and later the first derivation of induced pluripotent stem cells (iPSC) from a human adult skin biopsy [Takahashi et al. Citation2007]. Embryonic stem cells and iPSC technologies are also explored for utilization in livestock research, breeding, and transgenesis with a special focus on bovine and porcine cells. Pluripotent cells may be used to improve traits of agricultural importance, genetically manipulate species for the production of biomedical models, and potentially provide a model to test novel iPSC therapies [Lu et al. Citation2012]. However, it remains a challenging task to derive and culture stable pluripotent cell lines from large domestic animals [Ezashi et al. Citation2012; Telugu et al. Citation2010].
Evaluation of the protein profile of the blastocoel fluid would potentially advance our understanding of whether the fluid influences blastocyst development and more specifically on the regulation of proliferation of cells in the ICM. Furthermore, specific protein components in the blastocoel fluid would be candidates to use in culture media for both pre-implantation embryos and ESC/iPSC. Although several studies were performed in the 1970s on the physiochemical properties of especially rabbit blastocoel fluid [Borland et al. Citation1977; Cross Citation1973, Citation1974; Varma et al. Citation1979], the protein composition of the fluid is scarcely described. Nevertheless, the blastocoel fluid is expected to constitute a combination of proteins originating from the maternal environment through trans-cellular transport and/or through the release of components from ICM and TE cells of the blastocyst [Dardik and Schultz Citation1991]. Other prospects for examinations of blastocoel fluid are to select the best embryo for transfer after in vitro fertilization (IVF) procedures in humans [D'Alessandro et al. Citation2011], and also to identify genetic diseases in individuals before transfer, known as pre implantation genetic diagnosis or PGD [Palini et al. Citation2013].
Despite recent advances in various proteomic technologies, knowledge of the general mammalian blastocyst proteome remains very limited. The latest review in the field by Katz-Jaffe and McReynolds [Citation2013] lists the main hurdles to be the combined effects of limited template, low protein concentration, deficient platform sensitivity, and limited protein database information. Recently, our group published the first proteomic study on the human blastocoel fluid and blastocyst cells [Jensen et al. Citation2012]. In the present study we present a proteomic analysis of the blastocoel fluid and remaining cells of bovine blastocysts.
Results and Discussion
In this study, we isolated and analyzed the blastocoel fluid and remaining cells from 20 + 11 bovine blastocysts using micromanipulation as shown in . The expanded blastocyst with a clear blastocoel cavity was fixed on a holding pipette. The micropipette was inserted and blastocoel contents aspirated until collapse. The contents were then subjected to nano-HPLC tandem mass spectrometry.
Figure 1. Isolation of bovine blastocoel fluid. (A) A mixed population of blastocysts with arrested embryos (▪), expanded (○), and hatched blastocysts (•). Notice the empty zona pellucida (□). (B) Collection of expanded blastocysts with a clear blastocoel cavity chosen for micromanipulation. (C) A single blastocyst is fixed on a holding pipette with the inner cell mass (ICM) pointing towards the top of the holding pipette. (D) The micropipette is inserted in the blastocoel cavity. (E) Suction applied and the blastocoel is aspirated until the blastocyst collapses.
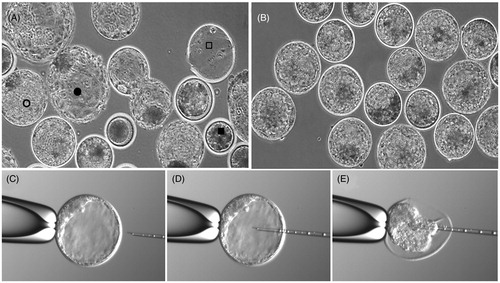
An overview of the number of proteins identified is presented in Venn-diagrams in . A total of 99 proteins could be identified in the blastocoel fluid; 23 of these were present in both samples and are described in . Fourteen of these 23 proteins could also be found in human blastocoel fluid [Jensen et al. Citation2012] and these common proteins are marked with an asterisk in the table. Of the 99 blastocoel proteins, 58 could also be identified in the bovine cell material which further supports the identity of these proteins.
Figure 2. Venn diagrams over protein identifications in bovine blastocoel fluid and blastocyst cells with an overview of the proteins in each biological replicate. Figure not to scale.
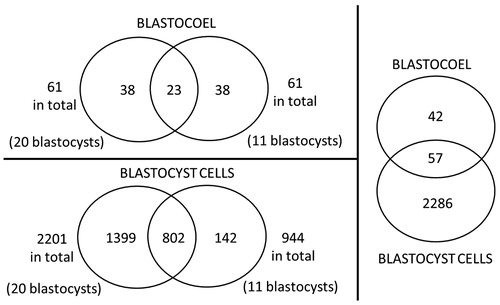
Table 1. Proteins identified in the bovine blastocoel fluid.
In the blastocyst cell material, 2,341 unique proteins could be identified. The large pool of cells from 20 blastocysts contributed 2,201 protein identifications while the smaller pool of 11 blastocysts contributed 944 protein identifications. There was an overlap of 802 proteins between the two biological replicates and these proteins were analyzed further using the ProteinCenter software. Here, the blastocyst proteins were grouped according to their cellular function using gene ontology (GO) terms, and the distribution is presented in a pie chart in . Proteins from the categories cell differentiation, cell proliferation, development and reproduction were retrieved and these are presented in Supplemental . Proteins from these categories could be of potential interest in the support of stem cell- and embryo culture and selected proteins are described in .
Figure 3. The distribution of gene ontology (GO) terms of the proteins from the bovine blastocyst cells. A protein can appear in more than one category.
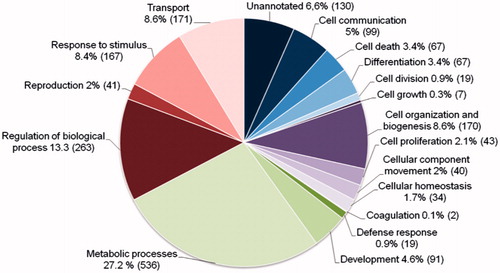
Table 2. Selected proteins from the bovine blastocyst cells with the gene ontology (GO) terms cell differentiation, cell proliferation, development, and reproduction.
Comparing the present bovine data set to the human blastocyst proteomic data set [Jensen et al. Citation2012] nine proteins were found to be unique to the bovine blastocoel fluid: Alpha-S1-casein, Annexin, Beta-casein, Beta-lactoglobulin, Casocidin-I, Histone H1.1, METTL3 protein, Proline-rich protein P-B, and Ubiquitin C. An overlap of 309 proteins was found comparing the protein identifications from the cell samples of the two species. These proteins are listed in Supplementary Table 1 and a selection of the proteins is presented in . Seventeen proteins were identified in the native culture medium (BlastAssist®) and since the only protein source in this medium is human serum albumin (HSA), the identified medium proteins must be co-purified proteins from the HSA production.
Table 3. Selected proteins identified in both human and bovine blastocyst cells.
Blastocoel proteins and pluripotency
In the search of proteins to support the undifferentiated growth of pluripotent stem cells (ESC and iPSC), the proteins in the blastocoel fluid are of obvious interest as they bathe the pluripotent ICM of the blastocyst while the undifferentiated cells proliferate. The study of human blastocoel fluid provided potential protein candidates such as various vitamin-binding proteins and specific heat shock proteins [Jensen et al. Citation2012]. It is well-known that bovine pluripotency in part is supported by factors different from those in humans [Maruotti et al. Citation2012], and it was therefore expected that previously unknown bovine blastocyst proteins of interest would be identified. However, not one of the nine proteins found to be unique to the bovine blastocoel fluid have any known relation to pluripotency or early developmental biology. Further functional studies are required to identify whether they function in early development.
Proteins found in the blastocoel fluid could potentially improve the production of stable bovine ESC or iPSC lines if added either alone or in combination to the culture medium during isolation and/or maintenance of the cells. The production of stable porcine ESC/iPSC lines as well as large numbers of porcine blastocysts has similarly proven to be a challenging task. While the developmental processes up until the hatching blastocyst are very alike between humans, cattle, and pigs, the post-hatching progression and placentation are substantially different between humans and the livestock species. Candidate proteins for improvement of culture media for porcine ESC/iPSC lines therefore may be found in both the human and bovine blastocoel proteomes, although the bovine results may be more relevant to the pig developmental biology.
It is clear that we have only identified a few of the most abundant proteins present in the blastocoel fluid in this bovine study. While the concentration of a specific protein is important for the biological effect, it is likely that a low-abundance protein could have an essential effect on cellular pluripotency or differentiation. However, such low abundance proteins could not be detected in this study. Isolation and analysis of blastocoel fluid is technically challenging and a larger study is needed to reveal more proteins in the important fluid of the mammalian blastocyst.
Development and signaling
In and Supplementary Table 1, proteins involved in cell differentiation, cell proliferation, development and reproduction are listed. For many proteins, this study is the first to link their expression to the mammalian blastocyst. Although our study does not provide understanding about the effects and kinetics of each blastocyst protein identified, the knowledge of which proteins are present in the blastocyst provide an important platform to qualify further functional studies. The recent progress in the mapping of the bovine embryo transcriptome [Chitwood et al. Citation2013; Driver et al. Citation2012; Filliers et al. Citation2011; Mamo et al. Citation2012] are invaluable to support this work.
Specifically in the GO category development, 89 proteins were identified in the bovine blastocyst cell material. Several of these development-associated proteins are known to have functions in cytoskeletal organization and cell adhesion and thus may exert functions in modulation of cell shape and migration in the developing embryo. Examples of such proteins from the present data are Drebrin, Desmoplakin, Fibronectin, Filamin-A, Integrin beta-1, Junction plakoglobin, Lactadherin, Transgelin-2, and Tubulin-folding cofactor B. Few proteins were identified with known roles in cellular signaling, examples are STAT3 protein and Catenin delta-1.
Surprisingly, none of the major proteins known to be involved in embryo-maternal signaling were detected in the blastocyst cells in our study (e.g. Interferon Tau, Interleukin 1B, TNF alpha, or IGF binding proteins [Munoz et al. Citation2012; Staggs et al. Citation1998; Wolf et al. Citation2003]). These proteins may be present in undetectable concentrations or may appear at later stages of development. Interferon Tau has been detected in other studies at the blastocyst stage [Johnson et al. Citation2006; Kimura et al. Citation2004; Kubisch et al. Citation2003] and this indicates that we probably have been unable to detect the known embryo-maternal signaling components in our study with the available blastocyst material and proteomic technology.
Proteins in the human and bovine blastocyst cells
A total of 309 of the 802 internally validated bovine blastocyst proteins were also identified in the human blastocyst cells [Jensen et al. Citation2012]. The development of the embryo up until the blastocyst stage is rather similar between human and cattle; however, there are differences in the developmental kinetics and in the subsequent early developmental steps. The blastocyst is formed on day 5–6 in humans and day 7–8 in cattle, and further the implantation of human blastocysts takes place shortly after the blastocyst is formed while the bovine blastocyst continues to grow and later elongate extensively for about three weeks of detached development [Blomberg et al. Citation2008]. The bovine conceptus size increases more than 1000-fold during the elongation [Maddox-Hyttel et al. Citation2003] and this is accomplished by an increase in cell number and accompanying protein synthesis [Degrelle et al. Citation2005; Thompson et al. Citation1998]. Furthermore, bovine placentation is synepitheliochorial, which means that the uterine epithelium is modified by invasion and fusion of trophoblast cells; however, it shows very little invasiveness [Wooding Citation1992]. It is therefore not surprising that the protein expression is not identical between the two species. However, human and bovine embryos can be successfully cultured up until the blastocyst stage in the same culture medium (e.g. BlastAssist®) and the expression of many of the basic housekeeping and conserved proteins are expected to be similar.
Our data can be used to pinpoint molecular differences in the developmental pathways between the two species in support of comparative studies. Such studies, preferably including the mouse embryo, are important with perspectives in both evolutionary biology and comparative physiology in a field where data regarding culture conditions and molecular functions often are extrapolated between the model-species cow and mouse to the human pre-implantation embryo.
Technical considerations
A day seven expanded bovine blastocyst with a size of around 200–250 μm is expected to contain 2–8 nl of blastocoel fluid. The collection of this fluid by micromanipulation is technically challenging and it is difficult to obtain sufficient clean sample material for a comprehensive proteomic mapping of the proteins in the fluid. In our study we examined two samples of pooled blastocoel fluid and even though the first sample contained double the amount of blastocoel fluid compared to the second (from 20 and 11 blastocysts, respectively), the resulting number of proteins identified are the same (61 proteins in each sample). Reasons for these results can be due to handling difficulties during the micromanipulation procedure leading to a smaller fluid yield per blastocyst or loss of sample material during their preparation for proteomic analysis. The yield from the proteomic analysis can also vary with such small samples because we are working at the limit of detection for the proteomic equipment. Blastocoel fluid from a larger number of blastocysts will be needed to obtain a full protein profile for the blastocoel fluid.
Regarding the cell material, it seems that the number of protein identifications increases proportionately when the number of blastocysts are increased (944 proteins in 11 blastocysts compared to 2,201 proteins in 20 blastocysts). That 802 of the 944 proteins from the small sample were also detected in the larger sample means that 85% of the cellular protein identifications could be reproduced. Analyzing a larger number of blastocysts, possibly without initial isolation of blastocoel fluid, would potentially provide a more detailed proteomic description of the bovine blastocyst.
To summarize, we report to have identified 23 proteins in two replicates of bovine blastocoel fluid and 803 proteins in the corresponding replicate blastocyst cell material. To our knowledge, this study is the first proteomic study of bovine blastocoel fluid and blastocyst cells and the data will supplement the recent advances in bovine embryo transcriptomics in improving the understanding of early mammalian development. This will potentially support progress in the field of in vitro fertilization and stem cell derivation and culture in both livestock animals and humans.
Materials and Methods
Production and handling of bovine blastocysts
Production of bovine embryos from slaughterhouse material with the sole purpose of research, does not require ethical approval by Danish law. Bovine blastocysts were produced at ORIGIO, Denmark and micromanipulation and sample collection was performed at the fertility clinic at Herlev Hospital, Denmark. Reagents and media were purchased from Sigma Aldrich (Brøndby, Denmark) unless otherwise stated. All media were pre-warmed and pre-equilibrated in a CO2 incubator before use.
Follicle aspiration and in vitro maturation (IVM)
Bovine ovaries, originating mainly from Danish dairy cows and heifers, were obtained from a local slaughterhouse (Herlufmagle Slagteri, Herlufmagle, Denmark), and transported to the laboratory within 3 h of retrieval at 29–32 °C in a thermo container. The temperature from ovary collection throughout all manipulations outside the incubator was kept within this temperature range.
The ovaries were rinsed twice in 0.9% saline and antral follicles with surface diameters between 3 and 15 mm were evacuated by use of a vacuum pump connected to an 18-G needle into a 50 ml tube containing 140 µl heparin (5,000 IE/ml, Leo Pharma, Ballerup, Denmark) to prevent clotting. The cumulus–oocyte complexes (COCs) were washed three times in TCM-199 wash medium supplemented with 50 µg/ml gentamycine and 0.5 mg/ml BSA. After retrieval and wash, the COCs were washed once in IVM medium consisting of DMEM supplemented with 5% FBS, 15 IE/ml PMSG-hCG (Suigonan, VetPharm, New York, USA) 1 mM Pyruvate, 50 ng/ml EGF and 50 µg/ml gentamycine before being transferred to the same medium for in vitro maturation in groups of 35–50 COCs in 4-well dishes (NUNC, Roskilde, Denmark) for 22.5–24.0 h at 35.8 °C in 5% CO2 in air and in saturated humidity.
In vitro fertilization (IVF)
After IVM, the groups of mature oocytes were transferred to new 4-well plates with TALP-IVF medium consisting of sterile water, 5 µg/ml phenol red, 0.5 mM MgCl2, 6H2O, 2 mM CaCl2, 2H2O, 0.4 mM NaH2PO4, 10 mM lactic acid, 25 mM NaHCO3, 3.2 mM KCl, 114 mM NaCl, 6 mg/ml BSA, 0.25 mM pyruvate, 30 µg/ml heparin, 20 µM penicillamine, 10 µM hypotaurine, 1 µM epinephrine, 0.25 mM Na-pyrovate, 0.6% BSA fraction V, and 50 µg/ml gentamycine. Frozen straws of semen from two different SDM bulls – Elvis and Haslund (Vikinggenetics, Randers, Denmark) were thawed, pooled, and washed twice in modified non-capacitation (mNCM) medium consisting of sterile water, 113.1 mM NaCl, 4.78 mM KCl, 1.19 mM KH2PO4, 1.19 mM MgSO4, 21.6 mM Na-lactate syrup, 5 µg/ml phenol red, 50 ug/ml gentamycine, and 0.5 mM NaHCO3. Sperm cells were added to the 4-well dish with oocytes in a final concentration of 2 × 106 sperm cells/ml. The oocytes were incubated for fertilization overnight at 38.8 °C in an atmosphere of 5% CO2 in air and in saturated humidity.
In vitro culture (IVC)
After IVF, cumulus cells and excess spermatozoa were removed by vortex agitation in wash medium for 2 min. The oocytes were washed twice in wash medium, counted, and washed once in IVC medium (BlastAssist, ORIGIO, Måløv, Denmark). Finally the oocytes were transferred to 4-well dishes with IVC medium covered with paraffin oil (ORIGIO). Twenty-five to 35 oocytes per well were incubated at 38.8 °C in an atmosphere of 6% CO2, 6% O2, and 90% N2 in saturated humidity. The blastocysts were evaluated at day 8 after fertilization and expanded but not hatched blastocysts were chosen for further sample preparation using micromanipulation.
Isolation of the blastocoel fluid and sample collection
The blastocoel fluid was isolated from the blastocyst cells by micromanipulation using Nikon Eclipse 2000 microscope (200X) with Narishige manipulators (Narishige, Tokyo, Japan). The pipettes used were Cook Micro-Injection Pipette (K-MPIP-3135) and Cook Holding Pipette (K-HPIP-2135). Three droplets of 5–10 µl IVC medium and a droplet of 3 µl PBS were prepared in an ICSI dish and covered with mineral oil. Five to ten blastocysts were transferred to each droplet with IVC medium and the dish was placed in the micromanipulator. The injection pipette was moved to the droplet of PBS and a small volume of PBS was taken into the pipette using the suction. This way the pipette was coated with PBS before sampling. In the media droplet, one blastocyst at the time was fixed on the holding pipette so that the ICM was placed in the holding-pipette side. The ICSI pipette was then gently lead in to the blastocyst cavity and suction applied to extract the fluid until the blastocyst collapsed (see ). The ICSI pipette was then moved to the PBS droplet where the blastocoel fluid was ejected. A small amount of PBS was left in the pipette and henceforth the blastocoel isolation was repeated on remaining blastocysts. When all blastocysts were emptied the dish was moved to a stereo microscope where the PBS/blastocoel droplet was transferred with a PBS-coated pipette tip to a low bind, protein free 500 µl tube (Eppendorf, Hamburg, Germany). Emptied blastocysts were collected in the smallest possible amount of medium and transferred to another low bind, protein free 500 µl tube with 5 µl PBS. Blastocoel and corresponding cell material from 21 + 11 bovine blastocysts were collected this way from two separate blastocyst in vitro productions. Both samples were immediately frozen at −80 °C. See the sampling procedure in and in the supplementary video (Supplementary Figure 1). The frozen samples were transported on dry ice for proteomic analysis at Odense University Hospital.
Proteomic analysis
The proteins of the two replicates of the blastocoel fluid or the corresponding lysed cells collected in PBS were digested by the addition of 10 ng lysC (Wako Chemicals, Richmond, VA, USA) in 8 M urea followed by a reduction of the disulfide bonds using dithiothrethiol (DTT) and blocking of free cysteine with iodoacetamide. The samples were diluted to a urea concentration of 0.75 M and further proteolytically cleaved by adding 25 ng sequencing grade trypsin (Promega, Madison, WI, USA). The resulting peptide mixtures were purified using reversed phase miocrocolumns and further fractionated using hydrophobic interaction liquid interaction chromatography (HILIC) using the fraction collection option of the Thermo/Dionex Ultimate 3000 nano/capillary HPLC system (Thermo Scientific, Sunnyvale, CA, USA). Briefly, the peptide samples were loaded onto a custom made HILIC column packed with TSKgel Amide-80 column material (3 µm bead size, 10 cm length, 300 µm ID, Tosoh Bioscience LLC, PA, USA). The resulting fractions were analyzed by nano-LC MSMS analysis using a Dionex Ultimate 3000 nano HPLC coupled to a Thermo Scientific Orbitrap Q-Exactive mass spectrometer (Thermo Scientific, Bremen, Germany); briefly, the samples (5 µl) were loaded onto a custom made fused capillary pre-column (2 cm length, 360 µm OD, 75 µm ID) with a flow of 5 µl per min for 7 min. Trapped peptides were separated on a custom made fused capillary column (20 cm length, 360 µm outer diameter, 75 µm inner diameter) packed with ReproSil Pur C18 3-µm resin (Dr. Maish, Ammerbuch-Entringen, Germany) with a flow of 300 nl per min using a linear gradient from 95% solution A (0.1% formic acid) to 35% B (100% Acetonitrile in 0.1% formic acid) over 86 min, followed by 10 min at 90% B, and 14 min at 98% A at a flow rate at 300 nl per min. Mass spectra were acquired in positive ion mode applying automatic data-dependent switch between one Orbitrap survey MS scan in the mass range of 300 to 1,500 m/z followed by HCD fragmentation and Orbitrap detection of the ten most intense ions observed in the MS scan. Target value in the Orbitrap for MS scan was 1,000,000 ions at a resolution of 70,000 at m/z 200. Fragmentation in the HCD cell was performed at normalized collision energy of 30 eV. Ion selection threshold was set to 50,000–100,000 counts. Selected sequenced ions were dynamically excluded for 45 s.
Data analysis
A combined MASCOT-SEQUEST search was performed where peak lists (mgf files) were processed using the Proteome Discoverer 1.4, version 1.4.0.288. The search parameters were set to: MS accuracy 10 ppm, MSMS accuracy 0.1 Da for HCD data, with two missed cleavages allowed, fixed modification of cystein blocked with carbamidomethyl, and variable modifications; methionine oxidation and deamidated asparagines. Tandem mass spectra were searched against a matured and merged Swissprot-trEMBL bovine database downloaded on demand 16th of May 2012 from http://www.ebi.ac.uk. Proteins were inferred on a basis of at least one unique peptide identified with a high confidence (FDR < 1%). The proteins were grouped according to their associated functions in specific biological processes using GO terms provided by the bioinformatics tool ProteinCenter (http://www.proxeon.com/home/index.html). Human contaminants as keratins and trypsin were deleted from all data using the cRAP database as reference (http://www.thegpm.org/crap/). The background medium used for the blastocyst culture (BlastAssist®) was analysed as well and the identified proteins deleted from the blastocyst data. Only proteins identified in both analyses (biological replica) are presented in the results and discussion, however, the full lists of proteins can be found in Supplementary Table 1. The chosen bovine database search resulted in 790 uncharacterized proteins identified in the blastocyst material. The protein sequence of these proteins (identified by Uniprot search: www.uniprot.org) were subjected to a BLAST search (http://blast.ncbi.nlm.nih.gov/Blast.cgi-?PAGE=Proteins) to provide a protein identification.
Declaration of interest
Pernille Linnert Jensen and Lotte Stroebech were employed at ORIGIO A/S at the time of conducting the experimental work, however, no conflict of interest exists. This work was funded by the The Novo Nordic Foundation and The Lundbeck Foundation (STC), and the Danish Agency for Science, Technology and Innovation, Danish Ministry of Science, Innovation and Higher Education (PLJ).
Author contributions
Conception and design, acquisition of data, data analysis and interpretation, manuscript writing, final approval of manuscript: PLJ; Acquisition of data, manuscript writing, final approval of manuscript: MLG; Conception and design, acquisition of data, data analysis and interpretation, manuscript writing, final approval of manuscript: HCB; Acquisition of data, manuscript writing, final approval of manuscript: JP; Conception and design, acquisition of data, data analysis and interpretation, manuscript writing, final approval of manuscript: LS; Conception and design, data analysis and interpretation, manuscript writing, final approval of manuscript: STC; Conception and design, data analysis and interpretation, manuscript writing, final approval of manuscript: CYA.
Abbreviations | ||
ESC | = | embryonic stem cells |
GO | = | gene ontology |
HILIC | = | hydrophobic interaction liquid interaction chromatography |
HPLC | = | high-performance liquid chromatography |
HSA | = | human serum albumin |
ICM | = | inner cell mass |
ICSI | = | intra cytoplasmic sperm injection |
iPSC | = | induced pluripotent stem cells |
IVF | = | in vitro fertilization |
IVM | = | in vitro maturation |
TE | = | trophectoderm |
Supplementary material available online
Supplementary Table 1
Supplementary Figure 1 (Video and Video Still)
Supplementary Material
Download PDF (36.5 KB)Supplementary Material
Download QuickTime Video (3.6 MB)Supplementary Material
Download MS Excel (1.2 MB)References
- Blomberg, L., Hashizume, K., and Viebahn, C. (2008) Blastocyst elongation, trophoblastic differentiation, and embryonic pattern formation. Reproduction 135:181–95
- Borland, R.M., Biggers, J.D., and Lechene, C.P. (1977) Studies on the composition and formation of mouse blastocoele fluid using electron probe microanalysis. Dev Biol 55:1–8
- Chitwood, J.L., Rincon, G., Kaiser, G.G., Medrano, J.F., and Ross, P.J. (2013) RNA-seq analysis of single bovine blastocysts. BMC Genomics 14:350
- Cross, M.H. (1973) Rabbti blastocoele pH. J Exp Zool 186:17–22
- Cross, M.H. (1974) Rabbit blastocoele bicarbonate: Accumulation rate. Biol Reprod 11:654–62
- D'Alessandro, A., Federica, G., Palini, S., Bulletti, C., and Zolla, L. (2012) A mass spectrometry-based targeted metabolomics strategy of human blastocoele fluid: A promising tool in fertility research. Mol Biosyst 8:953--8. Epub 2011 Oct 21
- Dardik, A., and Schultz, R.M. (1991) Protein secretion by the mouse blastocyst: Differences in the polypeptide composition secreted into the blastocoel and medium. Biol Reprod 45:328–33
- Degrelle, S.A., Campion, E., Cabau, C., Piumi, F., Reinaud, P., Richard, C., et al. (2005) Molecular evidence for a critical period in mural trophoblast development in bovine blastocysts. Dev Biol 288:448–60
- Driver, A.M., Penagaricano, F., Huang, W., Ahmad, K.R., Hackbart, K.S., Wiltbank, M.C., et al. (2012) RNA-Seq analysis uncovers transcriptomic variations between morphologically similar in vivo- and in vitro-derived bovine blastocysts. BMC Genomics 13:118
- Ezashi, T., Telugu, B.P., and Roberts, R.M. (2012) Induced pluripotent stem cells from pigs and other ungulate species: An alternative to embryonic stem cells? Reprod Domest Anim 47:92–7
- Filliers, M., De, S.W., Peelman, L., Goossens, K., Burvenich, C., Vandaele, L., et al. (2011) Laser capture microdissection for gene expression analysis of inner cell mass and trophectoderm from blastocysts. Anal Biochem 408:169–71
- Hardy, K., Handyside, A.H., and Winston, R.M. (1989) The human blastocyst: Cell number, death and allocation during late preimplantation development in vitro. Development 107:597–604
- Jensen, P.L., Beck, H.C., Petersen, J., Hreinsson, J., Wånggren, K., Laursen, S.B., Sørensen, P.D., Christensen, S.T., Andersen, C.Y. (2013) Proteomic analysis of human blastocoel fluid and blastocyst cells. Stem Cells Dev 22:1126--35. Epub 2012 Dec 26
- Johnson, K.M., Alvarez, X., Borkhsenious, O.N., and Kubisch, H.M. (2006) Nuclear and cytoplasmic localization of interferon-tau in in vitro-produced bovine blastocysts. Reprod Nutr Dev 46:97–104
- Katz-Jaffe, M.G., and McReynolds, S. (2013) Embryology in the era of proteomics. Fertil Steril 99:1073–7
- Kimura, K., Spate, L.D., Green, M.P., Murphy, C.N., Seidel, G.E. Jr., and Roberts, R.M. (2004) Sexual dimorphism in interferon-tau production by in vivo-derived bovine embryos. Mol Reprod Dev 67:193–9
- Kubisch, H.M., Rasmussen, T.A., and Johnson, K.M. (2003) Interferon-tau in bovine blastocysts following parthenogenetic activation of oocytes: Pattern of secretion and polymorphism in expressed mRNA sequences. Mol Reprod Dev 64:79–85
- Lu, Y., Mumaw, J.L., West, F.D., and Stice, S.L. (2012) Livestock induced pluripotent stem cells. Reprod Domest Anim 47:72–6
- Maddox-Hyttel, P., Alexopoulos, N.I., Vajta, G., Lewis, I., Rogers, P., Cann, L., et al. (2003) Immunohistochemical and ultrastructural characterization of the initial post-hatching development of bovine embryos. Reproduction 125:607–23
- Mamo, S., Rizos, D., and Lonergan, P. (2012) Transcriptomic changes in the bovine conceptus between the blastocyst stage and initiation of implantation. Anim Reprod Sci 134:56–63
- Maruotti, J., Munoz, M., Degrelle, S.A., Gomez, E., Louet, C., Diez, C., et al. (2012) Efficient derivation of bovine embryonic stem cells needs more than active core pluripotency factors. Mol Reprod Dev 79:461–77
- Mountford, J.C. (2008) Human embryonic stem cells: Origins, characteristics and potential for regenerative therapy. Transfus Med 18:1–12
- Munoz, M., Corrales, F.J., Caamano, J.N., Diez, C., Trigal, B., Mora, M.I., et al. (2012) Proteome of the early embryo-maternal dialogue in the cattle uterus. J Proteome Res 11:751–66
- Palini, S., Galluzzi, L., De, S.S., Bianchi, M., Wells, D., Magnani, M., et al. (2013) Genomic DNA in human blastocoele fluid. Reprod Biomed Online 26:603–10
- Pedersen, R.A. and Spindle, A.I. (1980) Role of the blastocoele microenvironment in early mouse embryo differentiation. Nature 284:550–2
- Pedersen, R.A., Wu, K., and Balakier, H. (1986) Origin of the inner cell mass in mouse embryos: Cell lineage analysis by microinjection. Dev Biol 117:581–95
- Stableford, L.T. (1949) The blastocoel fluid in amphibian gastrulation. J Exp Zool 112:529–46
- Staggs, K.L., Austin, K.J., Johnson, G.A., Teixeira, M.G., Talbott, C.T., Dooley, V.A., et al. (1998) Complex induction of bovine uterine proteins by interferon-tau. Biol Reprod 59:293–7
- Takahashi, K., Tanabe, K., Ohnuki, M., Narita, M., Ichisaka, T., Tomoda, K., et al. (2007) Induction of pluripotent stem cells from adult human fibroblasts by defined factors. Cell 131:861–72
- Telugu, B.P., Ezashi, T., and Roberts, R.M. (2010) The promise of stem cell research in pigs and other ungulate species. Stem Cell Rev 6:31–41
- Thompson, J.G., Sherman, A.N., Allen, N.W., McGowan, L.T., and Tervit, H.R. (1998) Total protein content and protein synthesis within pre-elongation stage bovine embryos. Mol Reprod Dev 50:139–45
- Thomson, J.A., Itskovitz-Eldor, J., Shapiro, S.S., Waknitz, M.A., Swiergiel, J.J., Marshall, V.S., et al. (1998) Embryonic stem cell lines derived from human blastocysts. Science 282:1145–7
- Varma, S.K., Dawood, M.Y., Haour, F., Channing, C., and Saxena, B.B. (1979) Gonadotropin-like substance in the preimplanted rabbit blastocyst. Fertil Steril 31:68–75
- Wolf, E., Arnold, G.J., Bauersachs, S., Beier, H.M., Blum, H., Einspanier, R., et al. (2003) Embryo-maternal communication in bovine - strategies for deciphering a complex cross-talk. Reprod Domest Anim 38:276–89
- Wooding, F.B. (1992) Current topic: The synepitheliochorial placenta of ruminants: Binucleate cell fusions and hormone production. Placenta 13:101–13