Abstract
Two morphologically similar species of opossum from the genus Didelphis–Didelphis virginiana and Didelphis marsupialis–cooccur sympatrically in Mexico. High intraspecific variation complicates their morphological discrimination, under both field and museum conditions. This study aims to evaluate the utility and reliability of using DNA barcodes (short standardized genome fragments used for DNA-based identification) to distinguish these two species. Sequences of the cytochrome c oxidase subunit I (Cox1) mitochondrial gene were obtained from 12 D. marsupialis and 29 D. virginiana individuals and were compared using the neighbor-joining (NJ) algorithm with Kimura's two-parameter (K2P) model of nucleotide substitution. Average K2P distances were 1.56% within D. virginiana and 1.65% in D. marsupialis. Interspecific distances between D. virginiana and D. marsupialis varied from 7.8 to 9.3% and their barcode sequences formed distinct non-overlapping clusters on NJ trees. All sympatric specimens of both species were effectively discriminated, confirming the utility of Cox1 barcoding as a tool for taxonomic identification of these morphologically similar taxa.
Introduction
Opossums of the genus Didelphis are New World marsupials that have a wide geographical distribution extending from southern Canada to central Argentina, from sea level to above 3000 m. They can live in widely diverse habitats including scrubland, temperate forest, rainforest, tropical evergreen, and tropical deciduous forest (Cerqueira and Lemos Citation2000; Ventura et al. Citation2002). There are six recognized species: Didelphis albiventris (from Venezuela and Guyana to central Argentina); Didelphis aurita (Brazil, Paraguay, and Argentina); Didelphis imperfecta (Venezuela, southern Surinam, French Guyana, and northern Brazil); Didelphis pernigra (Colombian Andes, Venezuela, Ecuador, Peru, and Bolivia); Didelphis marsupialis (extends from Mexico to Peru, Bolivia, and Brazil); and Didelphis virginiana (ranges from southern Canada to northern Costa Rica; Gardner Citation2005).
Two of these species occur in Mexico (Ramírez-Pulido et al. Citation2005), D. virginiana occupies almost the entire country except Baja California and the Central Plateau, and D. marsupialis is restricted to the coast of the Gulf of Mexico from southern Tamaulipas to northern Oaxaca, Chiapas, and the Peninsula of Yucatán. Thus, D. marsupialis is sympatric throughout its distribution with D. virginiana (CitationAranda 2000; ). Despite the information reported by Gardner (Citation1973), the taxonomic discrimination between these species is difficult even in museum-stored vouchers because the morphological diagnostic characters suggested by that author exhibit high intraspecific variation. Their discrimination is even more complicated in field-caught live individuals, leading to taxonomic misidentification during field surveys. Furthermore, factors such as animal age and the experience of the animal handler can influence the species delimitation (St-Pierre et al. Citation2006).
Figure 1. Geographical distribution of the Virginia Opossum (D. virginiana) and the Common Opossum (D. marsupialis) in Mexico (modified from Gardner Citation1973). Numbers correspond to specimen localities described in .
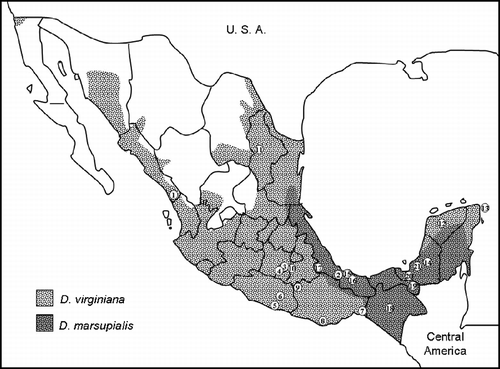
Some studies have reported that these two species of opossum can be distinguished through the use of external characters such as the hair color of the cheeks, the guard hair pattern, the extent of black color on the base of the tail, and head and body length–tail length ratio (Allen Citation1901; Davis Citation1944; Aranda 2002). However, Ruiz-Piña and Cruz-Reyes (Citation2002) could not differentiate among D. virginiana and D. marsupialis in Yucatán using the cheek coloration (white in D. virginiana and yellow in D. marsupialis) because several individuals had mixed hair color. In addition, Emmons (Citation1990) stated that the identification of these species based on external morphology alone is problematic because these characters are polymorphic, obscuring differences between species in areas of sympatric occurrence.
The accurate identification of these species of opossum is also needed because their highly similar morphology may lead them to have similar ecological niches in sympatric areas (Gardner Citation1973). In fact, these mammals are the most important reservoirs of the protozoan Trypanosoma cruzi that causes Chagas disease in the Mexican tropics (Huante-Magaña et al. Citation1990). Therefore, researchers need efficient methods to identify these species of opossum and understand their ecological roles as zoonotic disease reservoirs.
DNA barcodes have recently been proposed as a tool to facilitate species identification. This technique is based on the premise that DNA sequence diversity from short standardized regions of the genome can provide a “biological barcode” (Hajibabaei et al. Citation2006), where species are delimited by a particular sequence or by a tight cluster of very similar sequences (Ward et al. Citation2005). Recent works suggest that a 648 base pair (bp) region of the mitochondrial gene encoding the cytochrome c oxidase subunit I (Cox1) respiratory chain protein might serve as a DNA barcode for the identifications of animal species (Hebert et al. Citation2004a).
There are no data in the literature on the use of this molecular marker to recognize opossum species. However, research on bats and rodents has shown that DNA barcoding can accurately discriminate species (Clare et al. Citation2007; Borisenko et al. Citation2008). On the other hand, although reports show that within the monophyletic genus Didelphis, D. virginiana is the sister group to the clade containing D. marsupialis and D. albiventris (Patton et al. Citation1996; Palma Citation2003), and that D. virginiana is genetically divergent from D. marsupialis (Voss and Jansa Citation2003), the two species can be reliably identified using DNA barcoding approach across their ranges, including areas of sympatry. Therefore, the present study aims to distinguish these two marsupial species using Cox1 sequences.
Materials and methods
Samples, DNA extraction, amplification, and sequencing
Tissue samples (liver and kidney) from D. virginiana and D. marsupialis were collected from specimens trapped in the field and through loans from mammal collections (). All voucher specimens are housed in the Colección Nacional de Mamíferos of Instituto de Biología (IB) at the Universidad Nacional Autónoma de México in Mexico City. DNA was extracted following the manufacturer's protocol of the DNeasy Blood and Tissue Kit (Quiagen, México City, Distrito Federal, México), and its concentration was measured with a spectrophotometer. Extracted DNA was visualized with ethidium bromide through electrophoresis in 1% agarose gels.
Table I. Sequence origin of the Cox1 and the voucher museum specimen of the Virginia Opossum (D. virginiana), the Common Opossum (D. marsupialis), and other marsupial species.
A fragment of 647 bp of Cox1 was amplified through a PCR using the universal primers LepF1_t1 (5′-TGTAAAACGACGGCCAGTATTCAACCAATCATTCATAAAGATATGG-3′) and LepR1_t1 (5′-AGGAAACAGCTATGACTAGACTTCGGATGTCCAAAAAATCA-3′; Ivanova et al. Citation2007). The final reagent concentrations in a 25 μl volume were 1 × buffer, 2.5 mM MgCl2, 1 unit Taq polymerase (Invitrogen, Carlsbad, California, USA), 200 μM each dNTP (Promega, Pittsburgh, Pennsylvania, USA), 0.4 μM each primer, and 50 ng DNA. The optimum PCR conditions were initial denaturation at 94°C for 3 min; five cycles of 94°C for 30 s, 50°C for 40 s, 72°C for 60 s; 30 cycles of 94°C for 30 s, 60°C for 40 s; and a final extension at 72°C for 5 min. PCR products were verified through visualization on 1.5% w/v agarose gels and purified using a QIAquick PCR purification kit (Qiagen) following the manufacturer's instructions. DNA was sequenced bidirectionally in both directions using the IB automated sequencer (ABI Prism 3100 Genetic Analyzer; Applied Biosystems, Inc., México City, Distrito Federal, México) and the primers LepF1_t1 and LepR1_t1.
Data analysis
Sequences were edited and aligned manually using BioEdit v7.0.9 software (Hall Citation1999). Kimura's two-parameter (K2P) model of base substitution (Kimura Citation1980) was used to calculate genetic distances and a neighbor-joining (NJ) tree was constructed using the molecular evolutionary genetics analysis software (MEGA3; Tamura et al. Citation2007). Node support was tested with the bootstrap analysis at 1000 replicates. Trees were constructed using sequences of the gray four-eyed opossum (Philander opossum), Anderson's four-eyed opossum (Philander andersoni), and the brown four-eyed opossum (Metachirus nudicaudatus) for comparative purposes. In addition, the following sequences were obtained from the international database barcode of life database (BOLD) (http://www.barcodinglife.org; see ): GBMA 0523-06 D. virginiana; ABSMS 535-06 and ABSMS 588-06 D. marsupialis; ABSMS 559-06 white-eared opossum (D. albiventris); ABSMS 548-06 and ABSMS 070-06 Guianan white-eared opossum (D. imperfecta); ABSMS 363-06 Anderson's four-eyed opossum (P. andersoni); and ABSMS 569-06 brown four-eyed opossum (M. nudicaudatus).
Results and discussion
We obtained Cox1 sequences from 44 specimens; 41 from the genus Didelphis (12 D. marsupialis and 29 D. virginiana), and 3 from the genus Philander. Sequence length was about 657 bp, of which 498 sites were conserved, 56 sites were variable but uninformative, and 104 sites were variable and informative. No insertions, deletions, or stop codons were observed in any sequence. The average nucleotide frequencies were 27.6% adenine, 23.7% cytosine, 15.3% guanine, and 33.1% thymine.
We identified 14 different barcode sequences (haplotypes) that clustered in several haplogroups. The samples of D. virginiana formed two geographically segregated haplogroups, one for the USA (haplotype 1: GenBank accession number HQ451900) and the other for the range of this opossum in Mexico (haplotypes 2 and 3: GenBank HQ451898 and HQ451899, respectively; and ). Similarly, the NJ analysis clustered the samples of D. marsupialis into two haplogroups; one from Veracruz and Chiapas (haplotypes 4 and 5: GenBank HQ451901 and HQ451902, respectively) and another one resulting from three haplotypes from Mexico (haplotype 6: GenBank HQ451903) and Central and South America (haplotypes 7 and 8, respectively). As reported in other papers, it is not uncommon to observe several haplotypes per species due to the high mutation rate in mtDNA as the source of genetic variation (Nabholz et al. Citation2009). For example, Hajibabaei et al. (Citation2006) reported an average of eight barcode sequences per species in tropical butterflies. The other six haplotypes corresponded to D. albiventris (haplotype 9), D. imperfecta (haplotype 10), P. opossum (haplotypes 11 and 12: GenBank HQ451904 and HQ451905, respectively), P. andersoni (haplotype 13), and M. nudicaudatus (haplotype 14). The grouping of haplogroups by species received high bootstrap support, 81% for D. marsupialis and 99% for D. virginiana, reflecting that the sequence divergences were much greater between species than within them (), similar to reports for insects (Hajibabaei et al. Citation2006).
Figure 2. NJ tree of 49 Cox1 sequences of opossum species of the genera Didelphis, Philander, and Metachirus using K2P genetic distances. Numbers above the nodes are bootstrap support values based on 1000 replicates. *Sequence downloaded from BOLD (http://www.boldsystems.org).
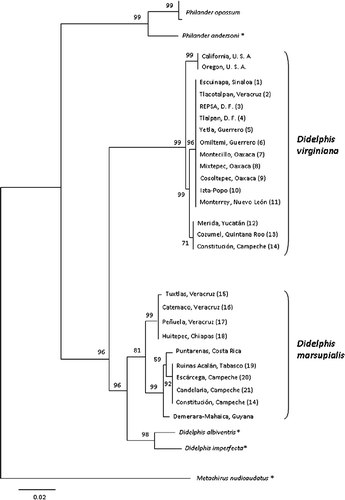
Genetic distances also indicate that these opossum species are different. The K2P genetic distance within barcode sequences in D. virginiana varied from 0.5 to 2.2% with an average of 1.56%, whereas in D. marsupialis they varied from 0.2 to 2.7% with an average of 1.65% (). These distances are higher than those reported within Neotropical bat species (0.6%; Clare et al. Citation2007), and fishes and birds, where the intraspecific average K2P genetic distances were 0.39 and 0.43%, respectively (Hebert et al. Citation2004a; Ward et al. Citation2005). However, values found by us are similar to those reported by Lissovsky et al. (Citation2007) for the Northern Pika (Ochotona hyperborea) because intraspecific distances were 2.44–2.90% (average = 2.78%).
Table II. Average genetic distances between opossum species of the genera Didelphis, Philander, and Metachirus estimated according to the K2P model of nucleotide substitution for barcode sequences.
DNA barcoding suggests that any species that splits into two or more groups with high bootstrap support and inter-group sequence divergence above 2% might represent a species complex (Hebert et al. Citation2004b). The intraspecific genetic distances recorded by us suggest that these opossum species might not include cryptic species, which facilitates comparisons between them at the specific level. Intraspecific variation within D. virginiana and D. marsupialis, however, might be reflecting merged phylogeographic variants or ancestral polymorphisms, as reported for other taxa (Hajibabaei et al. Citation2006).
The genetic distances between D. marsupialis and D. virginiana varied from 7.8 to 9.3%. This is consistent with average distances between congeners (7.8%) reported by Clare et al. (Citation2007) in Neotropical bat species, although Peropteryx leucoptera and Peropteryx kappleri showed almost 20% of sequence divergence. Among other vertebrates, Ward et al. (Citation2005) and Hebert et al. (Citation2004a) reported similar divergence levels within genera in birds (7.93%) and in fishes (9.93%). Therefore, the genetic distance found between D. marsupialis and D. virginiana, above 2%, supports the notion that they are different and recognizable species from the DNA barcode point of view.
The K2P distance percentages between the Didelphis species and other genera were even greater: 11.1–12.6% between D. marsupialis and P. opossum, and 17.7–19.5% between D. marsupialis and M. nudicaudatus; similarly, 12.1–13.2% between D. virginiana and P. opossum, and 20.3–21.8% between M. nudicaudatus and D. virginiana. Likewise, Ward et al. (Citation2005) reported divergence values of 15.5% among fish species within families.
Therefore, observed patterns of DNA barcode diversity and genetic distance in our dataset clearly separate D. marsupialis and D. virginiana in areas of their sympatric occurrence and show no evidence of mitochondrial introgression (). These results may thus complement the information provided by Gardner (Citation1973) to successfully distinguish these New World marsupials.
For example, we recorded D. marsupialis barcoded where the maxilo-frontal suture of the skull is anterior to the lacrimal bone; similarly, in barcoded D. virginiana this suture may be lined up either with the maxillary and frontal bones or anterior to the lacrimal bone too. Furthermore, four specimens from Yucatán, Campeche, and Oaxaca labeled as D. marsupialis after morphological identification in museums turned out to be D. virginiana according to our barcode analysis.
In addition, after examining almost 100 specimens from an area of sympatry in the Peninsula of Yucatán, Ruiz-Piña and Cruz-Reyes (Citation2002) reported that the external morphological characters are highly variable. They expected to find D. virginiana and D. marsupialis displaying white and yellow cheeks, respectively, as Gardner (Citation1973) reported. However, they karyotyped specimens of D. virginiana (eight out of 20 autosomal chromosomes are acrocentric) and D. marsupialis (20 out of 20 autosomal chromosomes are acrocentric), and found that each species may have individuals with either white or yellow cheeks; they then concluded that morphology could not tell these taxa apart. On the other hand, cheek color variation may not be a consequence of hybridization due to their differences in chromosome morphology (Gardner Citation1973).
In contrast, the specimens we barcoded were positively discriminated from localities within the range of sympatry: Peñuela, Tuxtlas, Tlacotalpan, and Catemaco in Veracruz; Huitepec in Chiapas; Candelaria, Constitución, and Escárcega in Campeche; Ruinas Acalán in Tabasco; Mérida in Yucatán; and Cozumel in Quintana Roo (). The NJ analysis assigned the samples from Peñuela, Tuxtlas, Catemaco, Huitepec, Ruinas Acalán, Escárcega, Candelaria, and Constitución to D. marsupialis, and the samples from Tlacotalpan, Constitución, Mérida, and Cozumel to D. virginiana. The sole collecting locality where both species were collected was Constitución in the state of Campeche; samples from this locality appear in both clusters ().
Our results support the notion that Cox1 barcode appears to be an effective tool for species recognition because it enables the rapid detection of deep intraspecific barcode divergence (Hajibabaei et al. Citation2006), and help to resolve taxonomic uncertainties in these opossum species. The barcode sequences we described display diagnostic sequence arrays for the barcode region as reported for other mammals (Clare et al. Citation2007). In conclusion, our results demonstrate that Cox1 barcodes may discriminate between samples of Common Opossum (D. marsupialis) and Virginia Opossum (D. virginiana) with sympatric distribution in Mexico. However, the application of DNA barcoding is no substitute for the full taxonomic analysis of morphological data, which should complement the analysis for final documentation of species richness (Hebert et al. Citation2004b).
Acknowledgements
The authors wish to thank the people, particularly Julieta Vargas who helped to collect field samples and curate specimens. Robert C. Dowler (ASUNHC), Loren K. Ammerman (ASUNHC), Silvia Hernández (FMVZ–UADY), and Consuelo Lorenzo (ECO-SC-M) graciously loaned opossum tissue samples. Comments and corrections by Jennifer Bain improved this manuscript. The Comisión Nacional para el Conocimiento y Uso de la Biodiversidad, Mexico partially financed this project through its program “Generación de Códigos de Barras del ADN de Especies Mexicanas”.
Declaration of interest: The authors report no conflict of interest. The authors alone are responsible for the content and writing of the paper.
References
- Allen JA. 1901. A preliminary study of the North American opossums of the genus Didelphis. Bull Am Mus Nat Hist. 14:149–188.
- Aranda M. 2002. Huellas y otros rastros de los mamíferos grandes y medianos de México. Xalapa: Instituto de Ecología, A. C.
- Borisenko AV, Lim BK, Ivanova NV, Hanner RH, Hebert PDN. 2008. DNA barcoding in surveys of small mammal communities: A field study in Suriname. Mol Ecol Resour. 8:471–479.
- Cerqueira R, Lemos B. 2000. Morphometric differentiation between Neotropical black-eared opossums, Didelphis marsupialis and D. aurita (Didelphimorphia, Didelphidae). Mammalia. 64:319–327.
- Clare EL, Lim BK, Engstrom MD, Egert JL, Hebert PDN. 2007. DNA barcoding of Neotropical bats: Species identification and discovery within Guyana. Mol Ecol Notes. 7:184–190.
- Davis WB. 1944. Notes on Mexican mammals. J Mammal. 25:370–403.
- Emmons LH. 1990. Neotropical rainforest mammals: A field guide. Chicago, IL: University of Chicago Press.
- Gardner AL. 1973. The systematics of the genus Didelphis (Marsupialia: Didelphidae) in North and Middle America. Special Pub Mus Texas Tech Univ. 4:1–81.
- Gardner AL. 2005. Order Didelphimorphia. In: Wilson DE, Reeder DM. editors. Mammal species of the world: A taxonomic and geographic reference. Baltimore, MD: Johns Hopkins University Press. p 3–18.
- Hajibabaei M, Janzen DH, Burns JM, Hallwachs W, Hebert PDN. 2006. DNA barcodes distinguish species of tropical Lepidoptera. Proc Natl Acad Sci USA. 103:968–971.
- Hall TA. 1999. Bioedit: A user-friendly biological sequence alignment editor and analysis program for Windows 95/98/NT. Nucl Acids Res. 41:95–98.
- Hebert PDN, Stoeckle MY, Zemlak TS, Francis CF. Identification of birds through DNA barcodes. PLoS Biol. 2004a; 2:1657–1663.
- Hebert PDN, Penton EH, Burns JM, Janzen DH, Hallswachs W. Ten species in one: DNA barcoding reveals cryptic species in Neotropical skipper butterfly Astraptes fulgerator. Proc Natl Acad Sci USA. 2004b; 101:14812–14817.
- Huante-Magaña R, Piza-Bernal R, Tabárez-Hernández J, Liera-Romero F, Mata-Carbajal E, Matadamas N. 1990. Enfermedad de Chagas en Guerrero. Reporte de dos casos confirmados con xenodiagnóstico. Salud Púb Méx. 32:320–324.
- Ivanova NV, Zemlak TS, Hanner RH, Hebert PDN. 2007. Universal primer cocktails for fish DNA barcoding. Mol Ecol Notes. 7:544–548.
- Kimura M. 1980. A simple method for estimating evolutionary rates of base substitutions through comparative studies of nucleotide sequences. J Mol Evol. 16:111–120.
- Lissovsky AA, Ivanova NV, Borisenko AV. 2007. Molecular phylogenetics and taxonomy of the pika (Ochotona, Lagomorpha). J Mammal. 88:1195–1204.
- Nabholz B, Glémin S, Galtier N. 2009. The erratic mitochondrial clock: Variation size affect mtDNA diversity across birds and mammals. BMC Evol Biol. 9:54–67.
- Palma RE. 2003. Evolution of American marsupials and the phylogenetic relationships with Australian metatherians. In: Jones M, Dickerman C, Archer M. editors. Predators with pouches: The biology of carnivorous marsupials. Collingwood: CSIRO. p 21–29.
- Patton JL, dos Reis SF, da Silva MN. 1996. Relationships among didelphid marsupials based on sequence variation in the mitochondrial cytochrome b gene. J Mammal Evol. 3:3–29.
- Ramírez-Pulido J, Arroyo-Cabrales J, Castro-Campillo A. 2005. Estado actual y relación nomenclatural de los mamíferos terrestres de México. Acta Zool Mex (nueva serie). 21:21–82.
- Ruiz-Piña H, Cruz-Reyes A. 2002. The opossum Didelphis virginiana as a synanthropic reservoir of Trypanosoma cruzi in Dzidzilché, Yucatán, México. Mem Inst Oswaldo Cruz. 97:613–620.
- St-Pierre C, Ouellet JP, Dufresne F, Chaput-Bardy A, Hubert F. 2006. Morphological and molecular discrimination of Mustela erminea (ermines) and M. frenata (long-tailed weasels) in Eastern Canada. Northeast Nat. 13:143–152.
- Tamura K, Dudley J, Nei M, Kumar S. 2007. MEGA4: Molecular evolutionary genetics analysis (MEGA) software version 4.0. Mol Biol Evol. 24:1596–1599.
- Ventura J, Salazar M, Pérez Hernández R, López Fuster MJ. 2002. Morphometrics of the genus Didelphis (Didelphimorphia: Didelphidae) in Venezuela. J Mammal. 83:1087–1096.
- Voss RS, Jansa SA. 2003. Phylogenetic studies on didelphid marsupials II. Nonmolecular data and new IRBP sequences: Separate and combined analyses of didelphine relationships with denser taxon sampling. Bull Am Mus Nat History. 276:1–82.
- Ward RD, Zemlak TS, Innes BH, Last PR, Hebert PDN. 2005. DNA barcoding Australia's fish species. Philos Trans R Soc B Biol Sci. 360:1847–1857.