Abstract
In the current study, a series of 4-chloromethyl-7-hydroxy-coumarin derivatives containing imidazolium, benzimidazolium, bisbenzimidazolium and quaternary ammonium salts were synthesized, characterized and the inhibition effects of the derivatives on human carbonic anhydrases (hCA I and hCA II) were investigated as in vitro. Structures of these coumarins were confirmed by FT-IR, (1)H NMR, (13)C NMR and LC–MS analyses. Structure activity relationship study showed that 3d (IC50: 79 μM for hCA I and 88 μM for hCA II) performed higher inhibitory activity than others.
Introduction
Coumarins are members of a class of compounds called benzopyrones and show various biological and pharmacological properties (Anand et al. Citation2012). Besides the numerous activities of coumarin compounds, they are reported as inhibitors of metalloenzyme carbonic anhydrase (Maresca et al. Citation2009, Maresca et al. Citation2010, Supuran Citation2010, Carta et al. Citation2012). Imidazole and their derivatives are generally used in the preparation of N-heterocyclic carbenes (NHC). Besides using in the synthesis of NHC's, antimicrobial and inhibitory properties of imidazolium salts and imidazole derivatives were reported (Hindi et al. Citation2009, CitationKhan et al. 2012). The quaternary ammonium salts exhibit antimicrobial activity against a wide range of bacteria, fungi and viruses (Tischer et al. Citation2012).
The metalloenzyme carbonic anhydrase (CA, EC 4.2.1.1) catalyzes a very simple but critically important physiological reaction: the involvement of the Carbonic anhydrase (CA) enzyme family, which catalyzes the physiological hydration of CO2 to yield bicarbonate and a proton, in many physiological/pathological processes opens up widespread opportunities for the development of diverse, specific inhibitors for clinical application (Supuran Citation2008, Thiry et al. Citation2006, McKiernan et al. Citation1997, Scozzafava et al. Citation2006).
The active site of most CAs contains a zinc ion (Zn2+), which is essential for catalysis. The CA reaction is involved in many physiological and pathological processes, including respiration and transport of CO2 and bicarbonate between metabolizing tissues and lungs; pH and CO2 homeostasis; electrolyte secretion in various tissues and organs; biosynthetic reactions such as gluconeogenesis, lipogenesis and ureagenesis; bone resorption; calcification; and tumorigenicity (Supuran et al. Citation2003, Smith and Ferry Citation2000, Stams and Christianson Citation2000, Pastorekova et al. Citation2004, Nishimori et al. Citation2007a, Citation2007b, Vullo et al. Citation2004, Nishimori et al. Citation2005a, Vullo et al. Citation2005a). Many of the CA isoenzymes involved in these processes are important therapeutic targets with the potential to be inhibited, to treat a range of disorders including edema, glaucoma, obesity, cancer, epilepsy and osteoporosis (Nishimori Citation2004, Vullo et al. Citation2003, Vullo et al. Citation2005b, Lehtonen et al. Citation2004, Nishimori et al. Citation2005b, Ashida and Brey Citation1995). Given the physiological importance of the CA, the metabolic impact of chemicals for crop production should receive greater importance.
The present study was carried out in order to synthesize, characterize and evaluate the biological activity of a new series of coumarin derivatives. In the current study, our main aim is to check whether the new derivatives may show higher inhibitory effect on CA enzymes with respect to the compounds previously investigated. The synthesized molecules have been characterized by various techniques including NMR, FT-IR and LC–MS. For in vitro inhibition studies, human erythrocyte carbonic anhydrase (CA I and CA II) isoenzymes were purified using Sepharose-4B l-tyrosine-sulphanilamide affinity chromatography. The inhibitory effects of novel compounds on the activities of purified human erythrocyte CA I and CA II isozymes were investigated.
Materials and methods
All reactions for the preparation of imidazolium and quaternary ammonium salts were carried out under argon using standard Schlenk-type flasks. Chemicals were obtained by Sigma Aldrich. DMF used as a solvent in the synthesis of imidazolium salts was dried using P2O5. N-methyl imidazole (2g), N-butyl imidazole (2h), N,N-dimethyl isopropyl amine (2i) and N,N-dimethyl benzyl amine (2j) were supplied commercially, used in the synthesis of imidazolium and quaternary ammonium salts and used without further purification.
Melting points were determined by Electrothermal-9200 melting point apparatus. FT-IR spectra were recorded on ATR unit in the range 400–4000 cm− 1 with Perkin Elmer Spectrum 100 Spectrophotometer. 1H-NMR and 13C-NMR were recorded using a Bruker AC300P FT spectrometer operating at 300.13 MHz (1H) and 75.47 MHz (13C). Chemical shifts are given in ppm relative to TMS, and coupling constants (J) in Hz. Elemental Analyses and LC–MS analyses were performed by IBTAM (Inonu University Scientific and Technological Research Central).
Synthesis of 4-chloromethyl-7-hydroxy-2H-chromen- 2-one (1)
4-chloromethyl-7-hydroxy-2H-chromen-2-one was synthesized by the procedure of Gumus et al. (Citation2010). Crude product was washed with diethyl ether and dichloromethane and dried under vacuum. Yellow solid, yield: 54%, mp:173–175°C; IR (cm− 1): 3207 (Ar-OH), 1682 (−C = O), 1603 (−C = C−); 1H-NMR (DMSO-d6, 300 MHz) δ/ppm:10.72 (1H, s, −OH) 7.69–7.67 (d, J = 8.8 Hz, 1H, CHC(OH)CH′CH″,), 6.85 (dd, 1H, CHC(OH)CH’CH″, JHH’ = 2.2 Hz, JH’H″ = 8.8 Hz), 6.80 (d, J = 2.2 Hz, 1H, CHC(OH)CH’CH″), 6.40 (s, 1H, −C = CH), 4.95 (s, 2H, −CH2Cl); 13C-NMR (DMSO-d6, 75 MHz) δ/ppm: 161.8, 160.6, 155.7, 151.4, 127.0, 114.1, 112.6, 109.8, 104.1, 42.5.
General procedure for preparation of 1-alkylbenzimidazole and bisbenzimidazole compounds (2a–f)
1-alkylbenzimidazole and bisbenzimidazole compounds were synthesized by the procedure of Ozdemir et al. (Citation2005). With this procedure N-methyl benzimidazole (2a), N-butyl benzimidazole (2b), N-benzyl benzimidazole (2c), N-(3,4,5-trimethoxybenzyl) benzimidazole (2d), 1,1’-(1,4-butanediyl)bis(benzimidazole) (2e) and 1,1’-(1,5pentanediyl)bis(benzimidazole) (2f) were synthesized.
General procedure for preparation of coumarin bearing benzimidazolium salts (3a–d)
Ten millimoles of 1-alkylbenzimidazole (2a–d) was dissolved in dried DMF (5 mL) and 10 mmol of 4-chloromethyl-7-hyrdroxy-2H-chromen-2-one was added into the solution, and the mixture was heated for 48 h at 90°C. After 48 h, the mixture was cooled to room temperature and diethyl ether was added to the mixture and the precipitates were collected by filtration. Crude product was washed with acetone and ethanol and dried under vacuum.
1-methyl-3-(4-methyl-7-hydroxy-2H-chromen-2-one) benzimidazolium chloride (3a)
White solid, yield; 77%, mp:277–279°C; anal.cal.for.:C18H15ClO3N2; C:63.07, H:4.41, N:8.17, found: C:63.14, H:4.45, N:8.0; IR (cm− 1): 3240 (Ar-OH), 1700(−C = O), 1573 (C-N); 1H-NMR (DMSO-d6, 300 MHz) δ/ppm: 11.07 (s, 1H, Ar-OH), 9.93 (s, 1H, NCHN), 8.13–6.88 (m, 7H, ArH), 6.17 (s, 2H,-CH2N), 5.72 (s, 1H, −CH = C−), 4.14 (s, 3H, −CH3); 13C-NMR (DMSO-d6, 75 MHz) δ/ppm:162.5, 160.4, 155.4, 149.6, 144.3, 132.6, 131.5, 127.4, 127.2, 126.5, 114.4, 114.1, 113.8, 109.5, 108.6, 103.0, 46.8, 34.1. LC–MS (m/z):345.2 [MH+].
1-butyl-3-(4-methyl-7-hydroxy-2H-chromen-2-one) benzimidazolium chloride (3b)
White solid, yield: 68%, mp: 231–233°C; anal.cal.for.: C21H21ClO3N2; C:65.54, H:5.50, N:7.28, found: C:65.61, H:5.58, N:7.18; IR (cm− 1): 3245 (Ar-OH), 1709 (−C = O), 1567 (C-N); 1 H-NMR (DMSO-d6, 300 MHz) δ/ppm: 11.03 (s, 1H, Ar-OH), 10.01 (s, 1H, NCHN), 8.05–6.87 (m, 7H, ArH), 6.14 (s, 2H, -CH2N), 7.75 (s, 1H, −CH = C), 4.55 (t, J = 7.2 Hz, 2H, −CH2CH2CH2CH3), 1.99 (five, J = 7.3 Hz, 2H, CH2CH2CH2CH3), 1.38 (six, J = 7.5 Hz, 2H, CH2CH2CH2CH3), 0.97 (t, J = 7.3 Hz, 3H, CH2CH2CH2CH3); 13C-NMR (DMSO-d6, 75 MHz) δ/ppm: 162.8, 162.5, 160.4, 155.5, 149.4, 143.7, 131.8, 131.7, 127.4, 127.3, 126.4, 114.5, 114.1, 113.8, 109.5, 108.9, 103.1, 47.3, 46.9, 30.8, 19.6, 13.9. LC–MS (m/z): 387.2 [MH+].
1-benzyl-3-(4-methyl-7-hydroxy-2H-chromen-2-one) benzimidazolium chloride (3c)
White solid, yield: 62%, mp: 210–213°C, anal.cal.for: C24H19 ClO3N2; C:68.82, H:4.57, N:6.69, found: C:68.95, H:4.60, N:6.59; IR (cm− 1): 3230 (Ar-OH), 1705 (−C = O), 1563 (C-N); 1H-NMR (DMSO-d6, 300 MHz) δ/ppm: 11.39 (s, 1H, Ar-OH), 11.00 (s, 1H, NCHN), 8.10–6.72 (m, 12H, ArH), 6.15 (s, 2H, -CH2N), 5.82 (s, 2H, Ph-CH2-N), 5.79 (s, 1H, −CH = C); 13C-NMR (DMSO-d6, 75 MHz) δ/ppm:162.5, 160.4, 160.1, 155.5, 149.4, 144.1, 134.2, 132.0, 131.6, 129.5, 129.3, 129.0, 128.0, 127.6, 127.4, 126.4, 114.6, 114.4, 113.8, 109.5, 109.0, 103.1, 56.5, 47.1. LC–MS (m/z):421.2 [MH+].
1-(3,4,5-trimethoxybenzyl)-3-(4-methyl-7-hydroxy-2H-chromen-2-one) benzimidazolium chloride (3d)
White solid, yield: 68%, mp:267–268°C, anal.cal.for: C27H25 ClO6N2; C:63.72, H:4.95, N:5.50, found: C63.74, H:4.96, N:5.47; IR (cm− 1): 3220 (Ar-OH), 1731 (−C = O), 1562 (C-N); 1H-NMR (DMSO-d6, 300 MHz) δ/ppm: 11.04 (s, 1H, Ar-OH), 10.08 (s, 1H, NCHN), 8.21–6.87 (m, 9H, ArH), 6.16 (s, 2H, -CH2N), 5.74 (s, 1H, -CH = C), 5.72 (s, 2H, Ph-CH2-N), 3.77 (s, 6H, Ar-OCH3(m-position)), 3.65 (s, 3H, Ar-OCH3 (p- position)); 13C-NMR (DMSO-d6, 75 MHz) δ/ppm: 162.8, 162.5, 160.4, 155.5, 153.7, 149.5, 143.8, 138.2, 131.9, 131.6, 129.3, 127.6, 127.4, 126.4, 114.7, 114.3, 113.8, 109.5, 108.9, 107.0, 103.1, 60.5, 56.5, 51.0, 47.0, 36.3. LC–MS (m/z):511.2 [MH+].
General procedure for preparation of coumarin bearing bisbenzimidazolium salts (3e, f)
Five millimoles of bisbenzimidazole (2e, f) was dissolved in dried DMF (5 mL) and 10 mmol of 4-chloromethyl- 7-hyrdroxy-2H-chromen-2-one was added into the solution, and the mixture was heated for 72 h at 90°C. After 72 h, the mixture was cooled to room temperature and diethyl ether was added to the mixture, and the precipitates were collected by filtration. Crude product was washed with acetone and ethanol and dried under vacuum.
3,3’-bis(4-methyl-7-hydroxy-chromen-2-one)- 1,1'buthylenedi(benzimidazolium)dichloride (3e)
White-yellow solid, yield: 48%, mp: 303–304°C; anal.cal.for: C38H32Cl2O6N4; C:64.14, H:4.53, N:7.87, found: C:64.15, H:4.55, N:7.93; IR (cm− 1): 3244 (Ar-OH), 1722 (−C = O), 1567 (C-N); 1H-NMR (DMSO-d6, 300 MHz) δ/ppm: 11.03 (s, 1H, Ar-OH), 10.22 (s, 1H, NCHN), 8.24–6.88 (m, 7H, ArH), 6.19 (s, 2H, −CH2N), 5.74 (s, 1H, −CH = C), 4.67, (t, 2H, −NCH2CH2−), 2.11 (t, 2H, −NCH2CH2−); 13C-NMR (DMSO-d6, 75 MHz) δ/ppm: 162.5, 160.4, 155.4, 144.0, 131.8, 131.7, 127.5, 127.3, 126.5, 114.5, 114.3, 113.8, 109.5, 108.7, 103.1, 47.0, 36.3, 25.8. LC–MS (m/z):713.2 [MH+].
3,3’-bis(4-methyl-7-hydroxy-chromen-2-one)-1,1'penthylenedi(benzimidazolium)dichloride (3f)
White-yellow solid, yield: 51%, mp: 252–255°C; anal.cal.for: C39H34Cl2O6N4; C:64.55, H:4.72, N:7.72, found: C:64.57, H:4.72, N:7.81; IR (cm− 1): 3205 (Ar-OH), 1722 (−C = O), 1568 (C-N); 1H-NMR (DMSO-d6, 300 MHz) δ/ppm: 11.05 (s, 1H, ArOH), 10.27 (s, 1H, NCHN), 8.21–7.79 (m, 7H, ArH), 6.02 (s, 2H, −CH2N), 5.74 (s, 1H, −CH = C), 4.59 (t, 2H, −NCH2CH2CH2−), 1.95 (2H, −NCH2CH2CH2−), 1.45 (2H, −NCH2CH2CH2−); 13C-NMR (DMSO-d6, 75 MHz) δ/ppm: 162.5, 160.4, 155.5, 143.9, 131.8, 131.6, 127.5, 127.3, 126.5, 114.5, 114.2, 113.8, 109.5, 108.8, 103.1, 47.3, 36.3, 31.2, 23.4. LC–MS (m/z):727.6 [MH+].
General procedure for preparation of coumarin bearing imidazolium salts (3g, h)
Ten millimoles of 1-alkylimidazole (2g, h) was dissolved in dried DMF (5 mL) and 10 mmol of 4-chloromethyl-7- hydroxy-2H-chromen-2-one was added into the solution, and the mixture was heated for 24 h at 90°C. After 24 h, the mixture was cooled to room temperature and diethyl ether was added to the mixture, and the precipitates were collected by filtration. The crude product was washed with acetone and ethanol and dried under vacuum.
1-methyl-3-(4-methyl-7-hydroxy-2H-chromen-2-one) imidazolium chloride (3g)
White solid, yield: 72%, mp: 262–264°C, anal.cal.for: C14H13ClO3N2; C:57.44, H:4.48, N:9.57, found: C:57.55, H:4.51, N:9.50; IR (cm− 1): 3310 (Ar-OH), 1705 (−C = O), 1575 (C-N); 1H-NMR (DMSO-d6, 300 MHz) δ/ppm: 11.09 (s, 1H, Ar-OH), 9.36 (s, 1H, NCHN), 7.89–6.87 (m,5H, ArH, N-CH = CH-N), 5.81 (s, 3H), 3.91 (s, 3H, −CH3); 13C-NMR (DMSO-d6, 75 MHz) δ/ppm: 162.8, 162.5, 160.4, 155.4, 150.2, 138.2, 126.2, 124.7, 123.5, 113.9, 109.5, 103.1, 48.8, 36.6. LC–MS (m/z):295.1 [MH+].
1-butyl-3-(4-methyl-7-hydroxy-2H-chromen-2-one) imidazolium chloride (3h)
White solid, yield: 63%, mp: 180–184°C, anal.cal.for: C17H19ClO3N2; C: 60.99, H:5.72, N:8.37, found: C:61.04, H:5.77, N:8.29. IR (cm− 1): 3240 (Ar-OH), 1710 (−C = O), 1567 (C-N); 1H-NMR (DMSO-d6, 300 MHz) δ/ppm: 11.03 (s, 1H, Ar-OH), 9.44 (s, 1H, NCHN), 7.93–6.85 (m, 5H, ArH, N-CH = CH-N), 5.82 (s, 1H, −CH = C), 5.78 (s, 2H, −CH2N), 4.22 (t, J = 7.3 Hz, 2H, −CH2CH2CH2CH3), 1.81 (five, J = 7.3 Hz, 2H, −CH2CH2CH2CH3), 1.24 (six, J = 7.5 Hz, 2H −CH2CH2CH2CH3), 0.91 (t, J = 7.3 Hz, 3H, CH2CH2CH2CH3); 13C-NMR (DMSO-d6, 75 MHz) δ/ppm: 162.5, 160.4, 155.5, 149.9, 137.7, 126.2, 123.7, 123.5, 113.8, 109.9, 109.4, 103.1, 49.4, 48.9, 34.6, 19.4, 13.8. LC–MS (m/z):345.2 [MH+].
Synthesis of N-(4-methyl-7-hydroxy-2H-chromen-2-one)-N-isopropyl-N,N-dimethylammonium chloride (3i)
4-chloromethyl-7-hydroxy-2H-chrome-2-one (2.5 mmol) was dissolved in CH3CN (10 mL) and N,N-dimethyl-N-isopropyl amine (2.5 mmol) was added into the solution, and the mixture was refluxed for 72 h. CH3CN was evaporated under vacuum. The crude product was extracted with hot water which was evaporated under vacuum. Brown solid, yield: 10%, mp:204–207°C; anal.cal.for: C15H20ClO3N; C:60.50, H:6.77, N:4.70, found: C:60.59, H:6.87, N:4.59; IR (cm− 1): 3345 (Ar-OH), 1705 (−C = O), 1605 (−C = C−); 1H-NMR (DMSO-d6, 300 MHz) δ/ppm: 7.99–7.96 (d, J = 8.8 Hz, 1H, CHC(OH)CH’CH″,), 6.91 (dd, 1H, CHC(OH)CH’CH″, JHH’ = 2.2 Hz, JH’H″ = 8.8 Hz), 6.84 (s, J = 2.2 Hz, 1H, CHC(OH)CH’CH″), 4.64 (s, 2H, −CH2N), 4,08(seven, J = 6.5 Hz, 1H NCH(CH3)2), 2.96 (s, 6H, N(CH3)2), 1.46 (d, J = 6.5 Hz, 6H, NCH(CH3)2). 13C-NMR (DMSO-d6, 75 MHz) δ/ppm: 162.3, 160.0, 155.9, 142.8, 127.7, 119.7, 113.7, 111.8, 103.2, 68.5, 58.2, 47.6, 16.9. LC–MS (m/z):300.2 [MH+].
Synthesis of N-(4-methyl-7-hydroxy-2H-chromen-2- one)-N-benzyl-N,N-dimethylammonium chloride (3j)
4-chloromethyl-7-hydroxy-2H-chromen-2-one (3.75 mmol) was dissolved in DMF (10 mL) and N,N-dimethyl-N-benzyl amine (3.75 mmol) was added into the solution, and the mixture was heated for 24 h at 60°C. DMF was evaporated under vacuum. The crude product was extracted using distilled water which was evaporated under vacuum, too. The oily crude product was washed with THF and precipitated. White solid, yield: 16%, mp:194–196°C. anal.cal.for: C19H20ClO3N: C:65.99, H:5.83, N:4.05, found: C:66.07, H:5.89, N:3.96; IR (cm− 1): 3357 (Ar-OH), 1704 (−C = O), 1615 (−C = C−); 1H-NMR (DMSO-d6, 300 MHz) δ/ppm: 10.99 (s, 1H, Ar-OH), 8.21–6.85 (m, 8H, ArH), 6.62 (s, 1H, −CH = C), 4.90 (s, 2H, −CH2N), 4.86 (s, 2H, Ph-CH2N), 3.03 (s, 6H, N(CH3)2); 13C-NMR (DMSO-d6, 75 MHz) δ/ppm: 162.0, 160.1, 156.0, 142.5, 133.7, 131.5, 129.9, 129.4, 129.2, 128.4, 113.6, 112.0, 103.2, 67.5, 61.8, 49.5.
Preparation of haemolysate and purification from blood red cells
Blood samples (25 mL) were taken from healthy human volunteers. They were anticoagulated with acid-citrate- dextrose, centrifuged at 2000 g for 20 min at 4°C and the supernatant was removed. The packed erythrocytes were washed three times with 0.9% NaCl and then haemolysed in cold water. The ghosts and any intact cells were removed by centrifugation at 2000 g for 25 min at 4°C, and the pH of the haemolysate was adjusted to pH 8.5 with solid Tris-base. The 25 mL haemolysate was applied to an affinity column containing L-tyrosine-sulphonamide-Sepharose-4B (Arslan et al. Citation1996) equilibrated with 25mMTris–HCl/0.1M Na2SO4 (pH 8.5). The affinity gel was washed with 50 mL of 25 mM Tris–HCl/22 mM Na2SO4 (pH 8.5). The human CA (hCA) isozymes were then eluted with 0.1 M NaCl/25 mM Na2HPO4 (pH 6.3) and 0.1 M CH3COONa/0.5 M NaClO4 (pH 5.6), which recovered hCA-I and hCA-II, respectively. Fractions of 3 mL were collected and their absorbance was measured at 280 nm.
CA enzyme assay
CA activity was measured by the Maren method which is based on determination of the time required for the pH to decrease from 10.0 to 7.4 due to CO2 hydration (Maren Citation1960). The assay solution was 0.5 M Na2CO3/0.1 M NaHCO3 (pH 10.0) and Phenol Red was added as the pH indicator. CO2-hydratase activity (enzyme units (EU)) was calculated by using the equation t0-tc/tc where t0 and tc are the times for pH change of the nonenzymatic and the enzymatic reactions, respectively.
In vitro inhibition studies
For the inhibition studies of coumarin, different concentrations of these compounds were added to the enzyme. Activity percentage values of CA for different concentrations of each coumarin were determined by regression analysis using Microsoft Office 2000 Excel. CA enzyme activity without a coumarin solution was accepted as 100% activity.
Results and discussion
For evaluating the CA inhibitory activity, all synthesized compounds () were subjected to CA inhibition assay with CO2 as substrate. The results showed that all synthesized compounds (3a–f) inhibited the hCA I and II enzyme activity. The inhibition values of 1–3f analogues against CAs are summarized in . We have determined the IC50 values of 79–620 μM for hCA I and 88–698 μM for hCA II, respectively. Among the compounds synthesized, 3d was found to be the most active one for CAs (IC50:79 μM for hCA I and 88 μM for hCA II).
Scheme 1. Synthesized Salts. Reagents and Conditions; (i) 2a–d, DMF, 90°C, 48 h (Karatas et al. Citation2013). (ii) 2e–f, DMF, 90°C, 72 h. (iii) 2g–h, DMF, 90°C, 24 h. (iv) 2j, DMF, 60°C, 24 h. (v) 2j, CH3CN, reflux, 72 h.
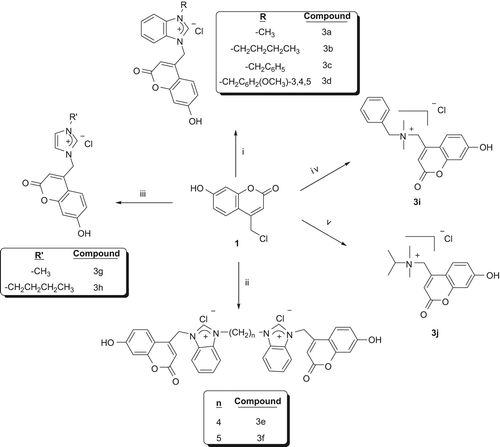
Table I. IC50 values of novel coumarin compounds.
Coumarins/thiocoumarins may possess various tautomeric forms, such as the zwitterionic benzo (thio) pyrylium phenoxides, which may bind within the CA active site similarly to phenols (Ebbesen et al. Citation2009), that is, by anchoring to the zinc-bound water molecule/hydroxide ion (CitationNair et al. 1994). It was reported that the coumarin-binding site in CAs may interact with diverse compounds, such as the antiepileptic drug lacosamide, which inhibits mammalian CAs I–XV, with inhibition constants in the range of 331–4.56 μM (Temperini et al. Citation2010).
The natural product (Vu et al. Citation2008) coumarin 6-(1S-hydroxy-3-methylbutyl)-7-methoxy-2H-chromen-2-one, as well as structurally related, simple coumarin derivatives possessing various substitution patterns at the heterocyclic ring, (Smith and Ferry Citation2000) was shown to be hydrolyzed within the CA active site with formation of 2-hydroxy- cinnamic acids, which possess significant CA inhibitory properties and bind in a completely unprecedented manner to the enzyme, not interacting with the Zn(II) ion as the sulphonamide/sulphamate/sulphamide type of inhibitors (Maren Citation1960). It was reported that the coumarin lacks classical zinc-binding functional groups and exhibits an unusual binding mode that may be exploited to design isoform–selective CA inhibitors. The inhibitor exhibits an extended two-arm conformation that effectively plugs the entrance to the active site (Vu et al. Citation2008). The present investigation reported that coumarin derivatives (3a–f) had potent inhibitory effects on the hCA I and II.
It has been reported that the novel coumarin derivatives containing sulphonamide were shown to exhibit inhibition effect against the hydratase activities of hCA-I, hCA-II (IC50 values of 4.7, 9.6 and 25.3 μM) and dog CA (Vullo et al. Citation2004, Bayram et al. Citation2007). Our inhibition values were closer to theirs. Gupta and Kumaran (Citation2005) reported that uncharged compounds cannot be made selective for cytosolic or membrane-bound isozyme since in both the cases the compounds appear to follow the same mechanism of inhibition. However, for the charged compounds, the polarizability of the molecule seems to greatly favour the inhibition of the membrane-bound enzyme, and hence they can be made selective for this enzyme by enhancing their polarizability, which is found to play no role in the inhibition of cytosolic enzymes (Gupta and Kumaran Citation2005). Barros et al. reported that the reaction of 20 aromatic/heterocyclic sulphonamides containing a free amino, imino, hydrazino or hydroxyl group, with 8-quinoline-sulphonyl chloride afforded a series of water-soluble (as hydrochloride or triflate salts) compounds. The new derivatives were assayed as inhibitors of the zinc enzyme CA, and more precisely of three of its isozymes, CA I, II (cytosolic forms) and IV (membrane-bound form), involved in important physiological processes. Some of the best inhibitors synthesized were topically applied as 2% water solutions onto the eye of normotensive and glaucomatous albino rabbits, when strong and long-lasting intraocular pressure (IOP) lowering was observed with many of them. This new compound is quite water soluble as hydrochloride salt, behaves as a strong CA II inhibitor and fared better than the parent molecule in lowering IOP in experimental animals (Borras et al. Citation1999). Therefore, our new water-soluble coumarins can be effective inhibitors for CA isozymes.
Structure Activity Relationship (SAR)
From this study, some opinions about SAR were emerged. First, all salts showed higher carbonic anhydrase inhibitor activity than 4-chloromethyl-7-hydroxycoumarin obviously. When we investigate factors that effect the inhibitory activity of the synthesized compounds, two factors were revealed; molecule size and electronic effects. When compounds (3a–d) containing imidazolium salts are compared, it can be said that the inhibitory activity increased by the size of the molecule. When coumarin derivatives containing benzimidazolium salts (3a–d) and imidazolium salts (3g–h) were compared, benzimidazolium salts showed higher inhibitory activity than imidazolium salts (3a > 3g, 3b > 3h). When bisbenzimidazolium salts (3e, 3f) and benzimidazolium salts (3a, 3b) are compared, the effect of molecule size on the inhibitory activity again can be seen (3e, f > 3a, b). When benzimidazolium salts 3c and 3d are compared, the effect of molecule size on the inhibitory activity again can be seen. Electron-donating methoxy group-bearing benzimidazolium salt (3d) is the most active salt in synthesized compounds. In this study, we only synthesized 7-hydroxy coumarin derivatives. If we want to see the role of coumarin skeleton on inhibitory activity, we can compare synthesized salts with the literature. Recently our group synthesized 7,8-dihydroxycoumarin-bearing benzimidazolium salts and their inhibitory effect on CA (4, 5, 6, 7) was evaluated () (Karatas et al. Citation2013). If we compare 3a–d salts with their 7,8-dihydroxy analogues (4–7); 7,8 –dihydroxy coumarin derivatives showed higher inhibitory effect obviously ().
Figure 1. 7,8-dihydroxy coumarin benzimidazolium salts synthesized by our group (Karatas et al. Citation2013).
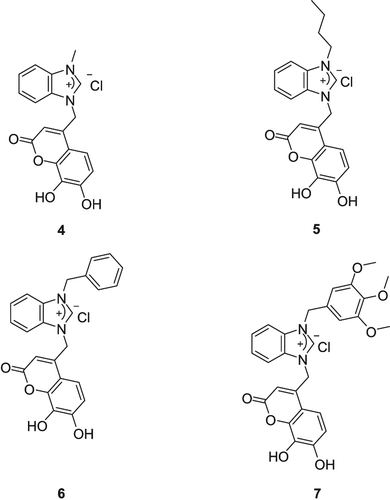
Table II. IC50 values of coumarin compounds (Karatas et al. Citation2013).
In summary, enzyme inhibition is more important issue for drug design and biochemical applications (Aydemir and Kavrayan Citation2009, Gencer and Arslan Citation2011, Demir et al. Citation2012, Bytyqi-Damoni et al. Citation2012, Sayin et al. Citation2012, Cicek et al. Citation2012, Gokce et al. Citation2012, Sonmez et al. Citation2011, Arslan et al. Citation2012, Gencer et al. Citation2012a, Citation2012b, Demirel and Tarhan Citation2004). The results showed that the newly synthesized coumarin derivatives inhibited the hCA I and II enzyme activity. Therefore, our results suggested that new coumarin derivatives are likely to be adopted as candidates to treat glaucoma and may be taken for further evaluation in vivo studies.
Acknowledgments
Special thanks to Dr. Fatih Sonmez for his help and advice during the study.
Declaration of interest
The authors report no conflicts of interest. The authors alone are responsible for the content and writing of the paper.
References
- Anand P, Singh B, Singh N. 2012. A review on coumarins as acetylcholinesterase inhibitors for Alzheimer's disease. Bioorg Med Chem. 20:1175–1180.
- Arslan O, Nalbantoglu B, Demir N, Ozdemir H, Küfrevioglu OI. 1996. A new method for the purification of carbonic anhydrase isozymes by affinity chromatography. Turk J Med Sci. 26:163–166.
- Arslan M, Gencer N, Arslan O, Guler OO. 2012. In vitro efficacy of some cattle drugs on bovine serum paraoxonase 1 (PON1) activity. J Enzyme Inhib and Med Chem. 27:722–729.
- Ashida M, Brey P. 1995. Role of the integument in insect defense: pro-phenol oxidase cascade in the cuticular matrix. Proc Natl Acad Sci USA. 92:10698–10702.
- Aydemir T, Kavrayan D. 2009. Purification and characterization of glutathione-s-transferase from chicken erythrocyte. Artif Cells Blood Substit Immobil Biotechnol. 37:92–100.
- Bayram T, Arslan O, Ugras HI, Cakir U, Ozensoy O. 2007. Purification of carbonic anhydrase from dog erytrocytes and investigation of in vitro inhibition effects by various compounds. J Enzyme Inhib Med Chem. 22:739–744.
- Borras J, Scozzafava A, Menabuoni L, Mincione F, Briganti F, Mincione G, Supuran CT. 1999. Carbonic anhydrase inhibitors: synthesis of water-soluble, topically effective intraocular pressure lowering aromatic/heterocyclic sulfonamides containing 8-quinoline-sulfonyl moieties: is the tail more important than the ring?Bioorg Med Chem. 7: 2397–2406.
- Bytyqi-Damoni A, Genc H, Zengin M, Beyaztas S, Gencer N, Arslan O. 2012. In vitro effect of novel β-lactam compounds on xanthine oxidase enzyme activity. Artif Cells Blood Substit Immobil Biotechnol. 40:369–377.
- Carta F, Maresca A, Scozzafava A, Supuran CT. 2012. Novel coumarins and 2-thioxo-coumarins as inhibitors of the tumor-associated carbonic anhydrases IX and XII. Bioorg & Med Chem. 20:2266–2273.
- Cicek B, Ergun A, Gencer N. 2012. Synthesis and evaluation in vitro effects of some macro cyclic thiocrown ethers on erythrocyte carbonic anhydrase I and II” Asian. J Chem. 24:3729–3731.
- Demirel LA, Tarhan L. 2004. Dismutation properties of purified and GDA modified CuZnSOD from chicken heart. Artif Cells Blood Substit Immobil Biotechnol. 32:609–624.
- Demir D, Gencer N, Er A. 2012. Purification and characterization of prophenoloxidase from Galleria mellonella L. Artif Cells Blood Substit Immobil Biotechnol. 40:369–377.
- Ebbesen P, Pettersen EO, Gorr TA, Jobst G, Williams K, Kienninger J, et al. 2009. Taking advantage of tumor cell adaptations to hypoxia for developing new tumor markers and treatment strategies. J Enzyme Inhib Med Chem. 24:1–39.
- Gencer N, Arslan O. 2011. In Vitro Effects of Some Pesticides on PON1Q192 and PON1R192 Isoenzymes from Human Serum. Fresen Envir Bull. 20:590–596.
- Gencer N, Ergun A, Demir D. 2012a. In vitro effects of some pesticides on human erythrocyte carbonic anhydrase activity. Fresen Environ Bull. 21:549–552.
- Gencer N, Ergun A, Demir D. 2012b. In vitro effects of some anabolic compounds on erythrocyte carbonic anhydrase I and II. J Enzyme Inhib and Medicinal Chem. 27:208–210.
- Gokce B, Gencer N, Arslan O, Turkoglu SA, Alper M, Kockar F. 2012. Evaluation of in vitro effects of some analgesic drugs on erythrocyte and recombinant carbonic anhydrase I and II. J Enzyme Inhib Med Chem. 27: 37–42.
- Gumus A, Karadeniz S, Ugras HI, Bulut M, Cakir U, Gören AC. 2010. Synthesis, complexation, and biological activity studies of 4-aminomethyl-7,8-dihydroxy coumarines and their crown ether derivatives. J Heteroc Chem. 47:1127–1133.
- Gupta SP, Kumaran S. 2005. A quantitative structure–activity relationship study on some aromatic/heterocyclic sulfonamides and their charged derivatives acting as carbonic anhydrase inhibitors. J Enzyme Inhib Med Chem. 20:251–259.
- Hindi KM, Panzenr MJ, Tessier CA, Cannon CL, Youngs WJ. 2009. The medicinal applications of imidazolium carbene-metal complexes. Chem Rev. 109:3859–3884.
- Karatas MO, Alici B, Cakir U, Cetinkaya E, Demir D, Ergun A, Gencer N, Arslan O. 2013. Synthesis and carbonic anhydrase inhibitory properties of novel coumarin derivatives. J Enzyme Inhib Med Chem. 28:317–322.
- Khan KM, Shah Z, Ahmad VU, Ambreen N, Khan M, Taha M, et al. (2012). 6-Nitrobenzimidazole derivatives: potential phosphodiesterase inhibitors: synthesis and structure-activity relationship. Bioorg Med Chem. 20:1521–1526.
- Lehtonen J, Shen B, Vihinen M, Casini A, Scozzafava A, Supuran CT, et al. 2004. Characterization of CA XIII, a novel member of the carbonic anhydrase isozyme family. J Biol Chem. 279:2719–2727.
- Maren TH. 1960. A simplified micromethod for the determination of carbonic anhydrase and its inhibitors. J Pharm Exp Ther. 130:2629–2634.
- Maresca A, Temperini C, Vu H, Pham NB, Poulsen SA, Scozzafava A, Quinn RJ, Supuran CT. 2009. Nonzinc mediated inhibition of carbonic anhydrases: coumarins are a new class of suicide inhibitors. J Am Chem Soc. 131:3057–3062.
- Maresca A, Temperini C, Pochet L, Masereel B, Scozzafava A, Supuran CT. 2010. Deciphering the mechanism of carbonic anhydrase inhibition with coumarins and thiocoumarins. J Med Chem. 53:335–344.
- McKiernan JM, Buttyan R, Stifelman MD, Kutz AG, Chen MW, Olsson CA, Sawczuk IS. 1997. Expression of the tumor-associated gene MN: a potential biomarker for human renal cell carcinoma. Cancer Res. 57:2362–2365.
- Nair SK, Ludwig PA, Christianson DW. (1994). 2-site binding of phenol in the active-site of human carbonic-anhydrase-ii - structural implications for substrate association. J Am Chem Soc. 116:3659–3660.
- Nishimori I. 2004. Acatalytic CAs: carbonic anhydrase-related proteins. In: Supuran CT, Scozzafava A, Conway J, Eds. Carbonic Anhydrase—Its Inhibitors and Activators. Boca Raton (FL) USA: CRC Press, pp. 25–43.
- Nishimori I, Vullo D, Innocenti A, Scozzafava A, Mastrolorenzo A, Supuran CT. 2005a. Carbonic anhydrase inhibitors. The mitochondrial isozyme VB as a new target for sulfonamide and sulfamate inhibitors. J Med Chem. 48:7860–7866.
- Nishimori I, Vullo D, Innocenti A, Scozzafava A, Mastrolorenzo A, Supuran CT. 2005b. Carbonic anhydrase inhibitors: inhibition of the transmembrane isozyme XIV with sulfonamides. Bioorg Med Chem Lett. 15:3828–3833.
- Nishimori I, Minakuchia T, Onishia S, Vullob D, Cecchib A, Scozzafavab A, Supuran CT. 2007a. Carbonic anhydrase inhibitors: cloning, characterization, and inhibition studies of the cytosolic isozyme III with sulfonamides. Bioorg Med Chem. 15: 7229–7236.
- Nishimori I, Minakuchi T, Onishi S, Vullo D, Scozzafava A, Supuran CT. 2007b. Carbonic anhydrase inhibitors. DNA cloning, characterization, and inhibition studies of the human secretory isoform VI, a new target for sulfonamide and sulfamate inhibitors. J Med Chem. 50:381–388.
- Ozdemir I, Sahin N, Demir S, Cetinkaya B. 2005. In situ generated 1- alkylbenzimidazole-palladium catalyst for the Suzuki coupling of aryl chlorides. J Molec Catal. 234:181–185.
- Pastorekova S, Parkkila S, Pastorek J, Supuran CT. 2004. Carbonic anhydrases: current state of the art, therapeutic applications and future prospects. J Enzyme Inhib Med Chem. 19:199–229.
- Sayin D, Cakir DT, Gencer N, Arslan O. 2012. Effects of some metals on Paraoxonase activity from shark Scyliorhinus canicula. J Enzyme Inhib Med Chem. 27:595–598.
- Scozzafava A, Mastrolorenzo A, Supuran CT. 2006. Carbonic anhydrase inhibitors and activators and their use in therapy. Expert Opin Ther Pat. 16:1627–1664.
- Smith KS, Ferry JG. 2000. Prokaryotic carbonic anhydrases. FEMS Microbiol Rev. 24:335–366.
- Sonmez F, Sevmezler S, Atahan A, Ceylan M, Demir D, Gencer N, Arslan O, Kucukislamoglu M. 2011. Evaluation of new chalcone derivatives as polyphenol oxidase inhibitors. Bioorg Med Chem Lett. 21:7479–7482.
- Stams T, Christianson DW. 2000. The carbonic anhydrases—new horizons (Chegwidden WR, Carter ND, Edwards YH, Eds), pp. 159–174.
- Supuran CT. 2008. Carbonic anhydrases: novel therapeutic applications for inhibitors and activators. Nat Rev Drug Discov. 7:168–181.
- Supuran CT. 2010. Carbonic anhydrase inhibitors. Bioorg Med Chem Lett. 20:3467–3474.
- Supuran CT, Scozzafava A, Casini A. 2003. Carbonic anhydrase inhibitors. Med Res Rev. 23:146–189.
- Temperini C, Innocenti A, Scozzafava A, Parkkila S, Supuran CT. 2010. The coumarin-binding site in carbonic anhydrase accommodates structurally diverse inhibitors: the antiepileptic lacosamide as an example and lead molecule for novel classes of carbonic anhydrase inhibitors. J Med Chem. 53:850–854.
- Thiry A, Dogne JM, Masereel B, Supuran CT. 2006. Targeting tumor-associated carbonic anhydrase IX in cancer therapy. Trends Pharmacol Sci. 27:566–573.
- Tischer M, Pradel G, Ohlsen K, Hilzgrabe U. 2012. Quaternary ammonium salts and their antimicrobial potential: targets or nonspecific interactions?Chem Med Chem. 7:22–31.
- Vullo D, Franchi M, Gallori E, Pastorek J, Scozzafava A, Pastorekova S, Supuran CT. 2003. Carbonic anhydrase inhibitors: inhibition of the tumor-associated isozyme IX with aromatic and heterocyclic sulfonamides. Bioorg Med Chem Lett. 13:1005–1009.
- Vullo D, Franchi M, Gallori E, Antel J, Scozzafava A, Supuran CT. 2004. Carbonic anhydrase inhibitors. Inhibition of mitochondrial isozyme V with aromatic and heterocyclic sulfonamides. J Med Chem. 47:1272–1279.
- Vullo D, Voipiob J, Innocenti A, Riverab C, Rankib H, Scozzafavaa A, Kailab K, Supuran CT. 2005a. Carbonic anhydrase inhibitors. Inhibition of the human cytosolic isozyme VII with aromatic and heterocyclic sulfonamides. Bioorg Med Chem Lett. 15:971–976.
- Vullo D, Innocenti A, Nishimori I, Pastorek J, Scozzafava A, Pastorekova S, Supuran CT. 2005b. Carbonic anhydrase inhibitors. Inhibition of the transmembrane isozyme XII with sulfonamides-a new target for the design of antitumor and antiglaucoma drugs?Bioorg Med Chem Lett. 15:963–969.
- Vu H, Pham NB, Quinn RJ. 2008. Direct screening of natural product extracts using mass spectrometry. J Biomol Screen. 13: 265–275.