Abstract
We report a easy approach for the immobilization of glucose oxidase enzyme (GOx) on nanoparticles. Nanoparticles-modified polystyrene-ANH2 (PSA) with some salicylaldehyde derivatives were synthesized by means of condensation and investigated the enzymatic properties of GOx immobilized on there. Modified polystyrenes were characterized using IR spectra, gel permeation chromatography, and scanning electron microscopy. All the characteristics of the immobilized glucose oxidase (PSA-SalH)-GOx, (PSA-SalCH3)-GOx, and (PSA)-GOx enzyme showed except one of them. Immobilized GOx on to (PSA) showed two optimum pH, other immobilized polymers were one optimum.
Introduction
In recent years, we have witnessed extensive efforts in incorporating enzymes with various nanostructured materials, such as immobilization on inorganic or organic nanoparticles (CitationÇolak et al. 2012, CitationLuhana et al. 2012) and encapsulation of the enzyme into sol-gel materials (CitationDavid et al. 2011). Polymeric nanoplatforms have attracted considerable attention due to their applications, including as stabilizers in dispersions and drug delivery carriers (CitationBlandino et al. 2001, CitationBozkır et al. 2012). Immobilization of enzymes onto polymeric platforms is the most useful strategy to improve the operational stability of biocatalysts (CitationBhatti and Saleem 2009). Other benefits are obtained as well, such as better operational control, ease of product recovery without catalyst contamination (CitationBhatti and Saleem 2009). CitationUnnikrishnan et al. (2013) immobilized glucose oxidase enzyme (GOx) onto graphene oxide without any cross-linking agents or modifiers. CitationZhou et al. (2012) immobilized GOx on a support composed of graphene oxide (GO) and concanavalin A (Con A) and proposed that the amino groups of Con A were covalently attached to the carboxyl groups of pre-activated GO and then GOx was immobilized on the GO-Con A. Polymers such as polystyrene (PSt) can be covalently linked with enzymes, resulting in surfactant-like giant molecules that have the ability to form catalytic molecular layers at the interface of aqueous/organic biphasic systems (CitationZhu and Wang 2004, CitationGe et al. 2009).
GOx is a commercially important enzyme which has application in pharmaceutical industry as a biosensor for the enzymatic determination of glucose in diabetes and the fermentation of liquor, and in the food industry for the removal of glucose and shelf life of various products (CitationBozkır et al. 2012, CitationHanf and Koehler 2006). Although Gox has attracted interests in the varying process, this enzyme is unstable due to its complex molecular structure. Therefore, a number of immobilization techniques have recently been investigated to improve its stability. To date, different methods have been used for enzyme immobilization (CitationJaneek 1993, CitationMoyo et al. 2012). Of course, the active center of the enzyme must be protected during the formation of this bond. GOx is covered with carbohydrate chains. A carbohydrate chain has hydroxyl groups. Oxygen in hydroxyl groups is connected by hydrogen bonds to polymer-based macromolecule support including O, N, and S atoms.
As is known, enzyme immobilization by adsorption occurs via interactions such as hydrogen bonding and Van Der Waals forces between the support and the enzyme (CitationGuisan 2012). Polymer-based macromolecule supports having electronegative groups like oxygen, nitrogen, and sulfur may be useful for increasing the enzyme stability via hydrogen bonding attachment (CitationGuisan 2012). Therefore, the surface on which the enzyme is immobilized plays several vital roles such as retaining of tertiary structure in the enzyme through hydrogen bonding.
A lot of studies on PSt-supported-Schiff base, oxidation, and ion selectivity can be found in the literature. Studies on polymers having Schiff bases have been increasing in recent years. Schiff bases have −CH = N− group. −CH = N− group has sp2 hybridized orbital. The presence of a lone pair of electrons in a sp2 hybridized orbital of nitrogen atom of the azomethine group is biologically important. The azomethine group of the Schiff bases is involved in many different biological processes such as decarboxylation, transamination, electron transfer, etc. (CitationSarı et al. 2012). To investigate the effect of hydrogen bond on enzyme immobilization, polymeric support involving salicylaldehyde derivatives was prepared and it is a derivative involving −CH3 group that has electron-donating effect. To prepare such a polymeric support, the polystyrene-ANH (PSA) reacted with salicylaldehyde and 5-methyl-2-hydroxybenzaldehyde.
Materials and methods
Materials
GOx (β-D-glucose: oxygen-l-oxidoreductase, EC 1.1.3.4) from Aspergillus niger was purchased from Sigma Chemical Company (SIGMA, 49180). Its molecular weight and pI was 160,000 Da and 4.2, respectively. 4-aminoantipyrene (4-AAP), phenol, PSA, salicylaldehyde, and 5-methyl-2-hydroxybenzaldehyde were purchased from Sigma (St. Louis, MO). All the other chemicals used in this work were provided by Merck and Sigma-Aldrich and used without further purification. All other materials were reagent grade (Sigma-Aldrich Company). IR spectra were recorded on a Mattson-5000 FT-IR instrument in KBr pellets. The GPC measurements were recorded on a Waters 1500 Series Gel permeation chromatography (GPC). Scanning electron microscopy of the Au-Pd-coated compounds was done using a JEOL JEM 100 CX II scanning electron microscope (JEOL, Peabody, MA) equipped with a Link analytical system. The electron energy used was 20 keV.
Synthesis of support nanoplatforms attached Schiff bases
The polymeric-Schiff bases (PSA–Sch) were prepared by reacting of polystyrene-ANH2 (PSA) (1 g, 100–200 mesh, 0.8–1.2 mmol/g −NH2 loaded, 1% cross-linked (Aldrich)) in hot DMF (15 mL) with salicylaldehyde and its derivatives (5-Methyl-2-hydroxybenzaldehyde, 1.0 mmol) in DMF (10 mL) (). Aldehyde solutions were slowly added dropwise on amine solutions while stirring through 30 min. Then the reaction mixture was boiled and stirred under a reflux condenser ca. 3 h, at 70°C. After the mixture was cooled to the room temperature, modified polymers were poured into the acetone and washed by adding acetone. The resulting clear color product was filtered and dried in the oven and kept in desiccator over anhydrous CaCl2.
Immobilization of GOx on (PSA), (PSA-SalH) and (PSA-SalCH3)
The (PSA-Sch) polymers (0.0125 g) were placed in a 15 mL DMF: water solution (9:6) of 0.010 gL− 1 of Gox at 30°C in a shaking water bath for 2 h. The immobilized polymer was separated and the free enzyme was removed by washing with phosphate buffer (pH: 7, 15 mL). The immobilized enzymes were freshly used and then stored at 4°C. Saturation ratio was determined as 97.13% from absorbance value in 498 nm.
Assay for enzyme activity measurement
A colorimetric method based on Trinder's reaction was used for the determination of glucose concentration (CitationTrinder 1969). Glucose is enzymatically oxidized to gluconic acid and hydrogen peroxide in the presence of GOx. The hydrogen peroxide reacts with 4-aminoantipyrene (4-AAP) and phenol to form pink-colored quinoneimine dye, which has absorption maximum at 498 nm (A498). The following reaction was started by adding 16 mg glucose, after pre-incubating at 30°C for 15 min. This mixture was removed after incubating under continuous stirring at 30°C for 75 min, and then was transferred to quartz cuvette for measurement.
The following recipe was used for free enzyme/immobilized enzyme assay: 4 mL studied buffer (pH 3–8) + 10 mg 4-aminoantipyrene + 20 mg phenol + 0.5 mg of horse radish peroxidase (HRP) + 0.010 g/L, 6 mL free glucose oxidase/immobilize glucose oxidase in studied buffer + 16 mg glucose.
Effect of pH and temperature on activity of free and immobilized GOx
Optimum pH for free and immobilized GOx was determined by measuring the activity of free and immobilized enzymes in buffers of different pH values ranging from 3 to 10. The buffers used were: pH: 3–4 (CH3COONa/CH3COOH); pH: 5 (NaH2PO4/H3PO4); pH: 6 (Na2HPO4/NaH2PO4); pH: 7–9 (NaH2PO4/Na2HPO4); pH: 10 (Na2B4O7/NaOH). In case of temperature studies, free and immobilized enzymes were incubated in the reaction mixtures at different temperatures ranging from 25°C to 90°C. The activities of free and immobilized enzyme were plotted against respective temperature. In our study, CH3COONa/CH3COOH, Na2HPO4/NaH2PO4, and NaH2PO4/Na2HPO4 were used for pH 4, pH 6, and pH 7, respectively.
Effect of substrate
The activities of the free and immobilized enzymes with various substrate concentrations were plotted as the Lineweaver–Burk graphs to calculate maximum reaction rate (Vmax) and Michaelis–Menten constant (Km) values (). The Vmax value defines the maximum velocity when all of enzyme is saturated with substrate. Km, the substrate concentration at which an enzyme reaches ½ Vmax, reflects the effective characteristic of the enzyme and depends upon both partitioning and diffusion (CitationSarı et al. 2012).
Figure 4. Kinetic parameters of free and immobilized GOx (♦:Free enzyme, ▲: (PSA-SalH), ■: (PSA-SalCH3), x:(PSA)).
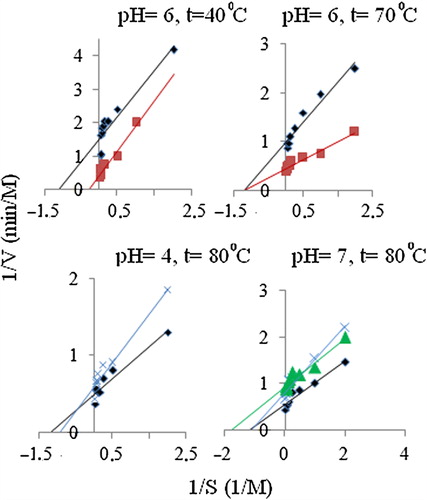
For optimum pH = 4 and 80°C temperature: To determine the extent at which immobilization affects the enzyme activity, Km and Vmax were determined at optimum pH and 40°C temperature (CitationJaneek 1993). Free and immobilized enzymes were incubated with different substrate concentrations (0.5–40 mM) in phosphate buffer of pH 4 and assayed for enzyme activity at 40°C, recommended temperature for enzyme assays.
For optimum pH = 6 and 40°C/70°C temperature: To determine the extent at which immobilization affects the enzyme activity, Km and Vmax were determined at optimum pH and 40°C/70°C temperature (CitationJaneek 1993). Free and immobilized enzymes were incubated with different substrate concentrations (0.5–40 mM) in phosphate buffer of pH 6 and assayed for enzyme activity at 40°C/70°C, recommended temperature for enzyme assays.
For optimum pH = 7 and 80°C temperature: To determine the extent at which immobilization affects the enzyme activity, Km and Vmax were determined at optimum pH and 80°C temperature (CitationJaneek 1993). Free and immobilized enzymes were incubated with different substrate concentrations (0.5–40 mM) in phosphate buffer of pH 8 and assayed for enzyme activity at 80°C, recommended temperature for enzyme assays.
Storage stability and reusability of immobilized enzyme
Storage stability experiments were carried out to determine the stabilities of immobilized enzymes after storage in dry conditions at + 4°C during the 8 months. The enzyme activity was measured every 7 days. Observed results were compared to the initial activities. To evaluate the reusability, the GOx-immobilized polymeric supports were also washed with buffer solution after any run and re-introduced into a fresh solution. Reaction cycles under the conditions (pH = 6, t = 40°C/70°C; pH = 4, t = 80°C; pH = 7, t = 80°C) described above were performed. The enzyme activity was measured at every 4 h.
Results and discussion
Heterogenlic index and some of the physical properties of all studied polymer are given in . The elemental analyses can be considered compatible with the chemical formulas of the compounds due to polymers of different chain lengths (CitationBozkır et al. 2012). The weight average molecular weight (Mw) was suggested from element analyses. Molecular weight and molecular weight distribution (Mw/Mn) were determined by GPC. According to GPC, modified polymers have a very narrow Mn (PDI: 1.82, 1.09, and 1.08 for (PSA), (PSA-SalH) and (PSA-SalCH3), respectively).
Table I. Elemental analysis and important physical properties of modified polymer.
FTIR and Sem-Edx spectra of modified nanoplatform
IR Spectra of support nanoplatforms-attached Schiff bases (PSA-Sch): The characteristic peaks of IR spectra of (PSA), (PSA-SalH), and (PSA-SalCH3) support polymers are given in . Three overtone peaks showed in ca. 1940, 1870, and 1800 cm− 1 for all support polymers. IR bands in the 3433, 3013, 2920, 556, and 541 cm− 1 regions are characteristics of υ(OH) (for adsorbed H2O), υ(CH) aromatic, υ(CH) aliphatic, υ(CH) buckling out of plane, and υ(CH) buckling, respectively. Imine band was observed in between 1618 and 1622 cm− 1.
SEM images of APS and modified polymers are shown in . SEM images of modified polymers were not markedly different from those of APS. This image indicates that the protection structures are of modified polymers. presents the elemental compositions of synthesized (PSA), (PSA-SalH), and (PSA-SalCH3) obtained from EDX analysis. EDX is not the technique of choice for analyzing polymers because intensities of the carbon atom are often erratic in modified polymers. However, an EDX-spectrum gives an excellent elemental analysis for all elements in the Periodic Table above beryllium in modified polymer. shows that hydrogen atoms are not taken into account in the composition of the studied polymers. An increase in the amount of oxygen according to the EDX spectra, modifies the polymer with an aldehyde group.
Table II. SEM imaging (Mag 120 × and 500×) EDX spectra of studied polymer.
Immobilization studies: influence of pH on the enzyme activity
The maximum activity was obtained at pH 5.0 for the free enzyme (CitationSarı et al. 2012). (PSA)-GOx has two maximum activities at pH 4.0 and 7.0 which is different from earlier reports (CitationDong et al. 2011). However (PSA-SalH)-GOx and (PSA-SalCH3)-GOx have one maximum activity at pH 6.0 and 7.0, respectively. They are illustrated in .
As is known, the pH is one of the important parameter capable of altering enzymatic activities in aqueous solution. Immobilization of enzyme is likely to result in conformational changes of enzyme resulting in a variation of optimum pH. The reason for having two optimums of immobilized (PSA) may be due to the two active properties of different residues of enzyme. The active site of the enzyme contains three amino acid side chains that are intimately involved in catalysis: His516 with pKa = 6.9 and Glu412 with pKa = 3.4, which is hydrogen bonded to His559, with pKa > 8. The protonation of each of these residues has a strong influence on all rate constants in the catalytic mechanism (CitationWang et al. 2011).
The catalytic mechanism of Glu412 may be more effective than that of His516 and His559 in the optimum pH = 4. His516 from the enzyme contains effective catalytic mechanism in optimum pH 6 and 7. To our knowledge, two optimum states are not a common property.
Influence of temperature on the enzyme activity
The effect of temperature on the activity of free and immobilized GOxs is shown in . There is significant difference between the profiles of the temperature optimum for free and immobilized GOxs.
The optimum temperature was not observed for free GOx at pH = 4 as it is denatured. But, surprisingly, the optimum temperature for immobilized GOx was found to be 80°C at pH = 4 for support (PSA) polymer. Furthermore GOx−(PSA) has shown optimum temperature 80°C at pH = 7. Also, at pH = 6, the dependence of the immobilized enzyme activities on temperature in a range of 20–90°C indicates that the optimal temperature for attaining highest activities of immobilized GOx to support (PSA-SalCH3) was 40°C and 70°C. (APS-SalH) has an optimum temperature of 80°C at pH 6. The results show that immobilized enzyme become more stable against heat and denaturing agents.
The storage stability and kinetic parameters (Km and Vmax)
The storage stability of an enzyme is one of its most important characteristics. Enzymes often lose their activity during the storage. Free and immobilized enzymes were stored in a dark bottle at + 4°C for 10 months. After 10 months, the free GOx and immobilized enzymes retained 92.30% and 89.78% of their original activity, respectively. High activitiy was found for modified polymer. This result suggests that the modified polymer-support confer a higher conformational stability upon the immobilized enzyme due to the formation of hydrogen bond of oxygen and nitrogen atoms (). The reusabilty was tested because of its importance for repeated applications in a batch reactor. Reusability is advantageous for cost reduction of the treatment (CitationSarı et al. 2012). Reuse of the immobilized enzyme was used three times in a day due to a long incubation time. After the third use of (PSA)-GOx, (PSA-SalH)-GOx, and (PSA-SalCH3)-GOx polymers the immobilized enzyme retained nearly 25, 8, 5% of their original activity at determinated optimum conditions. (PSA)-GOx is more stable than (PSA-SalH)-GOx and (PSA-SalCH3)-GOx due to condensation interaction between enzyme and PSA polymer ().
The activities of the free and immobilized enzymes with various substrate concentrations were plotted as the Lineweaver–Burk graphs to calculate Vmax and Km values (). The Vmax value defines the maximum velocity when all of enzyme is saturated with substrate. Km, the substrate concentration at which an enzyme reaches ½ Vmax, reflects the effective characteristic of the enzyme and depends upon both partitioning and diffusion (CitationAynacı et al. 2011).
Table III. Kinetic parameters for free and immobilized GOxs.
Kinetic parameters were studied for free GOx and immobilized glucose oxidase all optimum at pH = 4, 6, 7 and 8 (at t = 80°C, 40°C, 70°C and 80°C). The effect of the substrate concentration on the reaction rate was studied using varying initial concentrations (0.5–40 mM) of β-D-glucose substrate. The Km and the Vmax values of immobilized GOx were calculated from the Lineweaver–Burk plots (). For free GOx the Km/Vmax values were calculated for all optimum conditions by the Lineweaver–Burk plots. Km/Vmax values were calculated from the Lineweaver–Burk plots for immobilized GOx to the (PSA-SalH); (PSA-SalCH3); and (PSA) supports, 1.069/0.549 (pH = 7, t = 80°C) M/Mmin− 1; 0.706/3.703 and 2.216/0.813 (pH = 6, t = 40°C and pH = 6, t = 70°C) M/Mmin− 1; 1.641/1.031 and 1.266/0.917 (pH = 4, t = 80°C and pH = 4, t = 80°C) M/Mmin− 1, respectively.
Conclusions
In this study, we developed a novel strategy for the immobilization of GOx enzyme based on Schiff base-modified polymeric nanoplatform supports, which are synthesized from condensation of polymer containing −NH2 with furfuraldehyde derivatives. As is known, GOx is a dimeric protein and is covered with carbohydrate chains. A carbohydrate chain has hydroxyl groups (). In our study, the immobilized form of the enzyme was much more stable than that of the free enzyme. Furthermore, operational and storage stabilities of GOx dramatically improved following immobilization. These kinds of superior properties of our developed immobilization strategy would be of great advantage for various industrial applications, not only for GOx but also for other enzymes.
Declaration of interest
The authors report no declarations of interest. The authors alone are responsible for the content and writing of the paper.
This work was supported by the Gazi University Research Fund (Project number: 05/2010-75 and 05/2012-68).
References
- Aynacı E, Sarı N, Tumturk H. 2011. Immobilization of β-galactosidase on novel polymers having Schiff bases. Artif Cells Blood Substit. 39:259–266.
- Bhatti HN, Saleem N. 2009. Characterization of glucose oxidase from Penicillium notatum. Food Technol Biotechnol. 47:331–335.
- Blandino A, Macías M, Cantero D. 2001. Immobilization of glucose oxidase within calcium alginate gel capsules. Process Biochem. 36:601–606.
- Bozkır E, Sarı N, Oğutcu H. 2012. Polystyrene containing carbinolamine/azomethine potentially useful as antimicrobial agent: synthesis and biological evaluation. J Inorg Organomet Polym. 22:1146–1155.
- Çolak Ö, Yaşar A, Çete S, Arslan F. 2012. Glucose biosensor based on the immobilization of glucose oxidase on electrochemically synthesized polypyrrole-poly(vinyl sulphonate) composite film by cross-linking with glutaraldehyde. Artif Cells Blood Substıt Biotechnol. 40:354–361.
- David AE, Yang AJ, Wang NS. 2011. Enzyme stabilization and immobilization by sol-gel entrapment. Methods Mol Biol. 679:49–66.
- Dong LC, Wang G, Xiao Y, Xu Y, Zhou X, Jiang H, Luo Q. 2011. Immobilization of glucose oxidase on a novel crosslinked chitosan support grafted with L-lysine spacers. Chem Biochem Eng Q. 25:395–402.
- Ge J, Lu D, Liu Z, Lui Z. 2009. Recent advances in nanostructured biocatalysts. Biochem Eng J. 44:53–59.
- Guisan JM. 2012. Immobilization of enzymes and cells, In: Methods in Biotechnology, 2nd ed. Totowa, NJ: Humana Press Inc., p. 4–9, 23–25.
- Hanf F, Koehler P. 2006. Studies on the effect of glucose oxidase in bread making. J Sci Food Agric. 86:1699–1704.
- Janeek Š. 1993. Strategies for obtaining stable enzymes. Process Biochem. 28:435–445.
- Luhana C, Bo XJ, Ju J, Guo LP. 2012. A novel enzymatic glucose sensor based on Pt nanoparticles-decorated hollow carbon spheres-modified glassy carbon electrode. J Nanopart Res. 14:1158, 2–9.
- Moyo M, Okonkwo JO, Agyei MN. 2012. Recent advances in polymeric materials used as electron mediators and immobilizing matrices in developing enzyme electrodes. Sensors (Basel). 12:923–953.
- Sarı N, Antepli E, Nartop D, Yetim NK. 2012. Polystyrene attached Pt(IV)–Azomethine, synthesis and immobilization of glucose oxidase enzyme. Int J Mol Sci. 13:11870–11880.
- Trinder P. 1969. Determination of glucose in blood using glucose oxidase with an alternative oxygen acceptor. Ann Clin Biochem. 6:24–25.
- Unnikrishnan B, Palanisamy S, Chen SM. 2013. A simple electrochemical approach to fabricate a glucose biosensor based on grapheme-glucose oxidase biocomposite. Biosens Bioelectron. 39:70–75.
- Wang X, Zhu KX, Zhou HM. 2011. Immobilization of glucose oxidase in alginate-chitosan microcapsules. Int J Mol Sci. 12: 3042–3054.
- Zhou L, Jiang Y, Gao J, Zhao X, Zhao L, Zhou Q. 2012. Oriented immobilization of glucose oxidase on graphene oxide. Bıochem Eng J. 69:28–31.
- Zhu G, Wang P. 2004. Polymer-enzyme conjugates can self-assemble at oil/water interfaces and effect interfacial biotransformations. J Am Chem Soc. 126:11132–11133.