Abstract
The method based on integrating the principles of solid dispersion and nanocrystal techniques was developed to prepare polymer crystals (PCs) of mebendazole (MBZ) and polyethylene glycol (PEG). Powder X-Ray diffraction (PXRD) of the PC crystals shows the required integrated crystalline and amorphous regions. The in vitro solubility studies showed a 32-fold increase in the solubility of the drug. Tests of dissolution of the PCs showed that the crystals have an enhanced dissolution rate in comparison to those in the MF. The results of the pharmacokinetic study showed a 2.12-fold increase in the bioavailability of the drug. Thus, the present study has proved the potential in enhancing solubility, dissolution, and bioavailability of the drug.
Introduction
Human echinococcosis is caused by the larval forms of the parasite Echinococcus granulosus or E. multilocuralis, which migrate to the different organs of the body through the systemic circulation (CitationAlbonico et al. 1999, CitationSafioleas et al. 2000). For patients infected with the larval forms, surgery has been the only available treatment, but it is often ineffective due to extensive secondary alveolar echinococcosis (CitationChaudhary et al. 2014). Thus, it is proposed that an effective treatment with chemotherapy is needed before or after the surgery (CitationGagandeep et al. 2014). For this purpose, benzimidazole derivatives have been used. Mebendazole (MBZ) is the only drug of choice in treatment of alveolar echinococcosis, which requires continuous treatment for at least 2 years and patient monitoring for 10 years (CitationGarg 2014). However, it is not successful because of its poor solubility (CitationGarg and Goyal 2014) and bioavailability (CitationGarg et al. 2014a), and recurrence in certain cases of alveolar echinococcosis cause death of the patient (CitationChiba et al. 1991). Therefore, it is necessary to increase the drug's GI absorption (CitationGarg et al. 2014c) and bioavailability (CitationGarg et al. 2014b) for the effective medical treatment of hepatic (CitationGarg et al. 2012) and alveolar echinococcosis (CitationGarg et al. 2013, CitationGoyal et al. 2013a). It is well known that various preparations of the solid dispersions and nanocrystalline formulations are used to enhance the solubility, dissolution, and bioavailability of the drug (CitationChing et al. 2008, CitationVan Eerdenbrugh et al. 2007). Thus, in the present technique, the principles of solid dispersion and nanocrystal techniques are integrated to develop polymer crystals (PCs) of MBZ. This technique involves heating of the polymer solution of drug and polymer in a vacuum oven for a particular period of time (CitationGoyal et al. 2013b, Citation2014a, Citation2014b). After that, the saturated solution is cooled at room temperature to obtain dried residue, which yields PCs of the drug and the polymer (CitationHussain et al. 2014, CitationJohal et al. 2014, CitationJoshi et al. 2014). In the PC system developed, polyethylene glycol (PEG 4000) is used as the hydrophilic carrier to improve solubility (CitationKataria et al. 2014) and dissolution rate (CitationKaur et al. 2014a, Citation2014b) of the crystals. Moreover, this is also proven to enhance their bioavailability (CitationKaur et al. 2014c, Citation2014d) by increasing their saturation solubility in GI fluids (CitationKaur et al. 2014e, Citation2014f, Citation2014g). In the system developed, the large difference in the melting point of the drug and polymer provides an added advantage of drug stability in the PC system (CitationKaur et al. 2014h, Citation2014i, CitationMalik et al. 2014). The amorphous and semi-crystalline nature of the drug and polymer in the PCs has been used extensively in PC preparation, for its wetting (CitationMarwah et al. 2014), solubilizing (CitationModgill et al. 2014), and surface-active properties (CitationMorie et al. 2014). The extent of absorption of polymer appears to depend on the molecular weight (CitationPabreja et al. 2014), and more complete absorption (CitationRohilla et al. 2014b) has been reported for PEGs with lower molecular weight (CitationRameez et al. 2008). However, the absorption is much more limited in the case of PEGs with higher molecular weight (CitationSharma et al. 2014a). Hence, PEG 4000 was empirically selected as the ideal polymer due its ease of melting, as it has a low melting point (CitationSharma et al. 2014b), surfactant property (CitationSharma et al. 2014c), and oral safety (CitationSingh et al. 2014a). This technique is designed not only for the management of human echinococcosis, but also has its application in the use of drug molecules with limited aqueous solubility (CitationSingh et al. 2014b, CitationRohilla et al. 2014a, CitationKalia et al. 2014).
Experimental
Materials
Mebendazole was procured from Cadila Pharmaceuticals Pvt. Ltd. J&K, India, as a free gift sample. PEG 4000 was purchased from HiMedia Laboratories Pvt. Ltd., Mumbai. All other reagents were of the highest grade commercially available and were used without further purification.
Preparation of polymer crystals
In this technique, a polymeric solution of PEG 4000 and MBZ was heated in a vacuum oven (1555A/1-LABCO, India) for (3–4 h). The concentrated solution was then allowed to cool at room temperature for (6–8 h). The dried residue obtained was passed through a 100-mesh sieve, which was the required optimized formulation. This technique, once developed, was optimized for various parameters, namely the pH of the PC and MBZ solution, temperature of the vacuum oven, variation in weight of the polymer against the constant weight of the drug, variation in weight of the drug against the constant weight of the polymer, and the growth time of the PCs.
Characterization of polymer crystals
Morphology
The morphology of the PCs prepared was also examined by scanning electron microscopy [(SEM) EVO M-10 (Zeiss)] and transmission electron microscopy [(TEM) HITACHI-8100)]. In this technique, a small amount of sample was placed on the sample holder coated with gold-palladium alloy in SEM, and a holder containing copper in TEM, with a sputter coater. Accelerated voltage of 15 and 90 KV was employed to take the SEM and TEM images at 300000X respectively.
Characterization by powder X-ray diffraction
X-ray diffraction is one of the most important characterization tools to analyze the potential changes in the crystalline structure of drug during formulation and development. In this technique, samples of pure drug (DG), polymer (PR), and optimized polymer crystal (PC) were analyzed by the X-ray diffractometer system (XPERT-PRO), at Punjab University, Chandigarh, with Cu Kα radiation at a wavelength of 1.542 Å, generated at 40 mA and 45 kV. The scanning speed was 10°/min. from 5° to 50° of 2θ, with a step size of 0.017°.
Differential scanning calorimetry
Differential scanning calorimetry (DSC) is a thermoanalytical technique which is used to study the degree of crystallinity and polymorphic transitions or thermal transitions involving energy changes during the process of formulation. The phase transitions of MBZ and the prepared PC system were analyzed by differential scanning calorimetry (DSC Q10V9.0 Build 275, TA Instruments, MDU, Rohtak) at a heating rate of 10°C/min, from 20 to 300°C.
Drug content and percentage yield
To calculate the drug content, PCs equivalent to 60 mg of MBZ were dissolved in a suitable quantity of formic acid, filtered with a filter paper of pore size of 0.45 μm, suitably diluted with formic acid, and analyzed by UV-Vis spectrophotometer. Similarly, the percentage yield of each formulation was determined according to the total recoverable final weight of PCs and the total original weights of MBZ and PEG 4000 used.
Solubility studies
Solubility study of optimized PCs were determined by spiking an excess amount of developed formulation in three different media of specific pH levels [0.1 N HCL (pH 2.0), phosphate buffer (pH 2.0), and distilled water] to obtain a saturated solution. Sealed tubes were agitated on a shaking incubator (LSB -100 5RE, Daihan India) at 90 rpm for 24 h at 25°C. After filtration through a 0.1 μm membrane filter (Millipore Corporation), the filtrates were suitably diluted and analyzed on a UV spectrophotometer at their corresponding λmax.
In vitro drug release
The in vitro drug release profile was studied in 0.1N HCL (pH 2.0) using a dialysis bag. The dialysis membrane (pore size 12 KD, HiMedia) was exposed to running water for 12 h, to remove glycerin-based contents. The sulfur-based contents were removed by treating the tube with 0.3% (w/v) sodium sulfite at 70°C for 20 min. The dialysis membrane was washed with hot water at 70°C for 2 min and then exposed to a 0.2% (v/v) solution of sulfuric acid for 5 min. Acidification was followed by treatment with hot water to remove excess acid. The treated membrane was kept in alcohol until use for in vitro drug release studies. The in vitro drug release was performed using the activated dialysis membrane, described above. The dialysis bag was loaded with a preparation equivalent to 10 mg of the drug. The dialysis bag was then put in 50 ml of 0.1N HCL (pH 2.0) at 37 ± 1°C and stirred at 100 rpm. Samples (2 ml) were withdrawn at a predetermined time interval and replaced with the same volume of fresh medium; the withdrawn samples were assayed for drug content by measuring absorbance at the 289 nm wavelength for MBZ using a UV spectrophotometer (Shimadzu, Japan).
Pharmacokinetic study
Animal care and handling procedures were in accordance with the guidelines for animal use. The guidelines for animal use in experiments were followed as stipulated by the CPCSEA (Committee for Prevention, Control, and Supervision of Experimental Animals, Approval No. IAEC/CPCSEA/2014/177/Meeting no/10). The study protocol was approved by the animal care and use committee, ISF College of Pharmacy, Punjab Technical University. In this study, seven white rabbits of either sex (average weight 1.0–1.5 kg) were divided into three groups. The final formulation (FF) and marketed formulation (MF) equivalent to 20 mg/kg were administered orally. At predetermined time intervals, 1.0 ml of blood was collected and plasma was separated by centrifuging at 3000 rpm for 10 min (R-24C, Remi, India). For bioanalytical development, 200 μl of blank plasma was spiked with 200 μl of MBZ working test solutions, and then 200 μl of internal standard (atorvastatin) at a concentration of 200 μg/ml was added in each Eppendorf tube. The resultant plasma solution was vortexed for 15 min. To precipitate plasma proteins, ACN was added and the mixture was vortexed further for 2 min and centrifuged at 10,000 rpm for 10 min. After centrifugation, 1.0 mL of the supernatant was collected and diluted to 2 ml with the mobile phase. A 20 μl aliquot of the final preparation was auto-injected into the HPLC column. Responses (peak height) for major peaks were measured and quantity of MBZ in the formulation was calculated. The non-compartmental pharmacokinetic parameters were calculated using the Kinetica® software, version 3.0 (Thermo Fischer Scientific Inc.). The data from FF and MF were compared for statistical significance by one-way analysis of variance (ANOVA). The statistical significance of means was then compared by the multiple-range method of least significant difference.
Result and discussion
The preparation of PCs was relatively simple and the cooled masses of PCs were frangible enough to be ground easily. shows the optimized parameters of the developed PCs.
Table I. Optimized parameters for the development of PCs using the integrated principles of the solid dispersion and nanocrystal techniques.
In , optimized values for the developed PCs show the desired parameters which were optimized to develop PCs with enhanced solubility, dissolution, and bioavailability.
Estimation of solubility in distilled water, 0.1N HCL, and phosphate buffer pH (2.0)
The values for saturation solubility of MBZ in distilled water, 0.1 N HCL of pH (2.0), and phosphate buffer of pH (2.5) were found to be (71.06, 91.78, and 90.17) μg/ml respectively, which increased to (2273.92, 3854.76, and 3429) μg/ml for distilled water (32-fold increase), 0.1N HCL (42-fold increase) and phosphate buffer (38-fold increase) respectively. The results show significant increase in the solubility of the drug using the PC system. It was found that the solubility profile of PCs is better in presence of 0.1N HCL and phosphate buffer, as compared to that in distilled water. This might be due to the high solubility of the PC system in the acidic environment, as already proven in the pH-dependent solubility studies of the drug by Brook et al. (2007).
Drug content and percentage yield
MBZ assay in the PC formulation was almost 100%, and the percentage yield was greater than 93%. This method was relatively more feasible for the preparation of MBZ-PEG 4000 polymer crystals. The results of the drug content analysis and percentage yield state that MBZ was uniformly distributed in the PCs and the higher yield shows relatively lower process loss.
Morphology
shows the scanning electron micrograph of PCs, which appeared as smooth, irregular-surfaced, and bodies with a homogeneous mass. The uniform surface of PCs indicates that MBZ was uniformly adsorbed into the polymer. This also signifies the capability of the developed system to successfully integrate MBZ with the polymer. The transition electron micrograph () shows polymer crystals appearing as smooth, elongated, and irregular rod-like surfaced bodies. Some uniformly elongated rod-like structures as seen in the picture are the characteristic features of the PCs, which proves the formation of PCs by the integrated system developed.
Powder X-ray diffraction
The powder X-ray diffraction (PXRD) peak pattern in , shows characteristic peaks of PEG 4000 polymer (PR) that appeared at 2θ equal to 11.6°, 16.4°,19.6°, 21.7°, 26.89°, and 29.3°. The diffraction spectrum of plain MBZ (DG) showed that the drug is more amorphous, moderately crystalline, and possesses sharp peaks at 2θ equal to 12.58°, 16.50°, 19.25°, 19.67°, 20.10°, 21.35°, and 25.68°. In case of PC peaks of MBZ- PEG 4000 system, there was a decrease in intensity of MBZ but the peaks remain at the same position, confirming the stability of the drug. The low intensity of peaks in the PCs at the same position of MBZ reflects mutual concentration of drug and polymer or mixing of the semi-crystalline and amorphous regions of the drug and the polymer. This could be attributed to the destruction of its crystal lattice, because of integration of drug into the carrier. The peaks associated to the carriers are lost with respect to the PCs, which indicates the desired extent of integration of drug and polymer.
Differential scanning calorimetry
of MBZ shows an apparent endothermic peak at 260°C corresponding to its melting point. The sharp MBZ peak was lost in PCs, as shown in . Shifting of the MBZ peak in PCs indicate the possibilities of the interaction between the MBZ and polymer. In case of the minor endothermic peak, the narrow melting point range between 212°C and 233°C corresponds to less crystalline nature of the developed FF, which may be due to the integrated semi-crystalline regions of the polymer. Endothermic peaks of DSC demonstrate that PCs are less crystalline with low interaction of the drug and polymer in the FF, which enhances the stability during storage.
In vitro release study
Dissolution studies of the MF and the developed FF were performed in 0.1N HCL pH (2.0) due to absorption of the drug from the acidic environment. shows that the MF has a maximum of 67% release, whereas the FF has a maximum of 86% release. Enhanced dissolution of MBZ from PCs could be related to the surface activity and the wetting effect, which may lead to reduced agglomeration and hence increased surface area and the solubilizing effect of PEG 4000. In the dry state, drug particles were in close contact or integrated with the polymer particles as a result of uniform mixing, as shown by SEM. When the mixture came in contact with water, the polymer particles might have hydrated rapidly (because of the high hydrophilic potency of PEG 4000) into the polymer solution. The resulting increased wettability of the drug particles and enhancement of the drug solubility in the diffusion layer surrounding the particles subsequently releases the drug into the medium.
Drug release kinetics of the optimized formulation
represents the release pattern of the optimized formulation, dictated by the water penetration rate (diffusion control) which follows the relation Q = K2 t1/2, where, Q is the percentage of drug release at time t and K2 is the diffusion rate constant. This indicates that the drug concentration in the matrix is initially much higher than the solubility of the drug. As edge effects are negligible, PCs follow unidirectional diffusion. Finally, this model concludes that the thickness of the formulation is much larger than the size of the drug molecules, and the diffusivity of the drug molecules is constant.
Pharmacokinetic study of MBZ
After oral administration of the FF and the MF, maximum plasma drug concentration was obtained in the case of FF. Tmax was found to be 2 h in the case of MF, and Cmax was 0.173 ± 0.20 μg/ml. These values for MF indicate its limited solubility throughout the G.I. tract. The Cmax value of 0.363 ± 1.01 μg/ml for the FF showed better solubility and bioavailability than the MF. A comparison of the values for Tmax (2 h) indicates the absorption of drug more from the stomach than from the small intestine. The high Cmax value of 0.363 ± 1.01 μg/ml for the FF (PCs) proved the efficacy in increasing the solubility, dissolution, and bioavailability of the drug. However, the clearance and half-life values of the FF and MF were not significantly different, as shown in . The significantly higher AUC and Cmax values shown in indicate the higher extent of absorption of FF because of the improved dissolution rate in the stomach. In summary, the FF resulted in much higher bioavailability compared with the MF, as reflected by both Cmax and AUC values. These results show that the MBZ was more readily available from the FF as compared to the MF, because of the high integration of drug with the polymer by the system developed. Thus, the integrated system developed results in enhanced solubility, which enhances the absorption of the MBZ.
Figure 6. Plasma concentration time curve following oral administration of the FF and MF in two (Test) groups of white rabbits. Data expressed as Mean ± SD; *p < 0.05, statistically significant (n = 6).
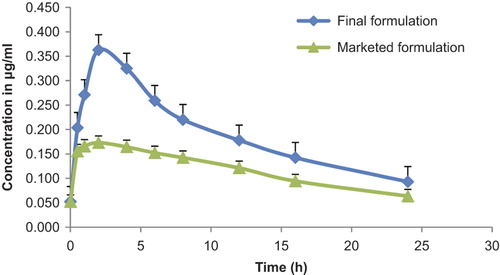
Table II. Pharmacokinetic parameters of the final PC system and the FF.
Conclusion
The PC system of MBZ was successfully developed and optimized in this study. The study has demonstrated that the integration of the PC system of MBZ into polymer changed the crystallinity of the drug, according to the type and amount of polymer. The formation of different crystalline and amorphous regions represents a suitable modification for improving the solubility, dissolution, and bioavailability of MBZ. The enhanced Cmax, and AUC profile of the developed FF indicate that it can be utilized for the safe and effective management of human echinococcosis. The developed formulation can reduce recurrence of this disease, as poor bioavailability of MBZ leads to the death of the patient. This approach proved the significant potential of the PCs developed, not only in the effective management of human echinococcosis, but also for further contribution to the usage of other BCS class 2 and BCS class 4 drugs.
Acknowledgement
Dr. Amit K. Goyal is thankful to the Department of Biotechnology (DBT), New Delhi, India (under IYBA scheme; BT/01/IYBA/2009 dated May 24, 2010).
Declaration of interest
The authors report no declarations of interest. The authors alone are responsible for the content and writing of the paper.
References
- Albonico M, Crompton DW, Savioli L. 1999. Control strategies for human intestinal nematode infections. Adv Parasitol. 42:277–341.
- Chaudhary S, Garg T, Murthy RS, Rath G, Goyal AK. 2014. Recent approaches of lipid-based delivery system for lymphatic targeting via oral route. J Drug Target. 22:1–12.
- Chiba Y, Kohri N, Iseki K, Miyazaki K. 1991. Improvement of dissolution and bioavailability for mebendazole, an agent for human echinococcosis, by preparing solid dispersion with polyethylene glycol. Chem Pharm Bull (Tokyo). 39:2158–2160.
- Ching AL, Liew CV, Chan LW, Heng PW. 2008. Modifying matrix micro-environmental pH to achieve sustained drug release from highly laminating alginate matrices. Eur J Pharm Sci. 33:361–370.
- Gagandeep, Garg T, Malik B, Rath G, Goyal AK. 2014. Development and characterization of nano-fiber patch for the treatment of glaucoma. Eur J Pharm Sci. 53:10–16.
- Garg T. 2014. Current nanotechnological approaches for an effective delivery of bio-active drug molecules in the treatment of acne. Artif Cells Nanomed Biotechnol. 1–8.
- Garg T, Goyal AK. 2014. Biomaterial-based scaffolds–Current status and future directions. Expert Opin Drug Deliv. 11:767–789.
- Garg T, Kumar A, Rath G, Goyal AK. 2014a. Gastroretentive drug delivery systems for therapeutic management of peptic ulcer. Crit Rev Ther Drug Carrier Syst. 31: 531–557.
- Garg T, Rath G, Goyal AK. 2014b. Ancient and advanced approaches for the treatment of an inflammatory autoimmune disease-psoriasis. Crit Rev Ther Drug Carrier Syst. 31:331–364.
- Garg T, Rath G, Goyal AK. 2014c. Comprehensive review on additives of topical dosage forms for drug delivery. Drug Deliv.
- Garg T, Singh O, Arora S, Murthy R. 2012. Scaffold: a novel carrier for cell and drug delivery. Crit Rev Ther Drug Carrier Syst. 29:1–63.
- Garg T, Singh S, Goyal AK. 2013. Stimuli-sensitive hydrogels: an excellent carrier for drug and cell delivery. Crit Rev Ther Drug Carrier Syst. 30:369–409.
- Goyal AK, Rath G, Garg T. 2013a. Nanotechnological approaches for genetic immunization. DNA and RNA Nanobiotechnologies in Medicine: Diagnosis and Treatment of Diseases, 67–120.
- Goyal G, Garg T, Malik B, Chauhan G, Rath G, Goyal AK. 2013b. Development and characterization of niosomal gel for topical delivery of benzoyl peroxide. Drug Deliv.
- Goyal G, Garg T, Rath G, Goyal AK. 2014a. Current nanotechnological strategies for an effective delivery of drugs in treatment of periodontal disease. Crit Rev Ther Drug Carrier Syst. 31:89–119.
- Goyal G, Garg T, Rath G, Goyal AK. 2014b. Current nanotechnological strategies for treating glaucoma. Crit Rev Ther Drug Carrier Syst. 31:365–405.
- Hussain T, Garg T, Goyal AK, Rath G. 2014. Biomedical applications of nanofiber scaffolds in tissue engineering. J Biomater Tissue Eng. 4: 600–623.
- Johal HS, Garg T, Rath G, Goyal AK. 2014. Advanced topical drug delivery system for the management of vaginal candidiasis. Drug Deliv. 1–14.
- Joshi D, Garg T, Goyal AK, Rath G. 2014. Advanced drug delivery approaches against periodontitis. Drug Deliv. 1–15.
- Kalia V, Garg T, Rath G, Goyal AK. 2014. Development and evaluation of a sublingual film of the antiemetic granisetron hydrochloride. Artif Cells Nanomed Biotechnol. 1–5.
- Kataria K, Sharma A, Garg T, Goyal AK, Rath G. 2014. Novel technology to improve drug loading in polymeric nanofibers. Drug Deliv Lett. 4:79–86.
- Kaur M, Garg T, Narang RK. 2014a. A review of emerging trends in the treatment of tuberculosis. Artif Cells Nanomed Biotechnol. 1–7.
- Kaur M, Garg T, Rath G, Goyal AK. 2014b. Current nanotechnological strategies for effective delivery of bioactive drug molecules in the treatment of tuberculosis. Crit Rev Ther Drug Carrier Syst. 31:49–88.
- Kaur M, Malik B, Garg T, Rath G, Goyal AK. 2014c. Development and characterization of guar gum nanoparticles for oral immunization against tuberculosis. Drug Deliv.
- Kaur N, Garg T, Goyal AK, Rath G. 2014d. Formulation, optimization and evaluation of curcumin-beta-cyclodextrin-loaded sponge for effective drug delivery in thermal burns chemotherapy. Drug Deliv. 1–10.
- Kaur P, Garg T, Rath G, Murthy RS Goyal AK. 2014e. Surfactant-based drug delivery systems for treating drug-resistant lung cancer. Drug Deliv. 1–12.
- Kaur R, Garg T, Das Gupta U, Gupta P, Rath G, Goyal AK. 2014f. Preparation and characterization of spray-dried inhalable powders containing nanoaggregates for pulmonary delivery of anti-tubercular drugs. Artif Cells Nanomed Biotechnol. 1–6.
- Kaur R, Garg T, Malik B, Gupta UD, Gupta P, Rath G, Goyal AK. 2014g. Development and characterization of spray-dried porous nanoaggregates for pulmonary delivery of anti-tubercular drugs. Drug Deliv. 1–6.
- Kaur R, Garg T, Rath G, Goyal AK. 2014h. Advanced aerosol delivery devices for potential cure of acute and chronic diseases. Crit Rev Ther Drug Carrier Syst. 31:495–530.
- Kaur V, Garg T, Rath G, Goyal AK. 2014i. Therapeutic potential of nanocarrier for overcoming to P-glycoprotein. J Drug Target. 1–12.
- Malik R, Garg T, Goyal AK, Rath G. 2014. Polymeric nanofibers: targeted gastro-retentive drug delivery systems. J Drug Target. 1–16.
- Marwah H, Garg T, Goyal AK, Rath G. 2014. Permeation enhancer strategies in transdermal drug delivery. Drug Deliv. 1–15.
- Modgill V, Garg T, Goyal AK, Rath G. 2014. Permeability study of ciprofloxacin from ultra-thin nanofibrous film through various mucosal membranes. Artif Cells Nanomed Biotechnol. 1–6.
- Morie A, Garg T, Goyal AK, Rath G. 2014. Nanofibers as novel drug carrier –An overview. Artif Cells Nanomed Biotechnol. 1–9.
- Pabreja S, Garg T, Rath G, Goyal AK. 2014. Mucosal vaccination against tuberculosis using Ag85A-loaded immunostimulating complexes. Artif Cells Nanomed Biotechnol. 1–8.
- Rameez S, Alosta H, Palmer AF. 2008. Biocompatible and biodegradable polymersome encapsulated hemoglobin: a potential oxygen carrier. Bioconjug Chem. 19:1025–1032.
- Rohilla R, Garg T, Bariwal J, Goyal AK, Rath G. 2014a. Development, optimization and characterization of glycyrrhetinic acid-chitosan nanoparticles of atorvastatin for liver targeting. Drug Deliv. 1–8.
- Rohilla R, Garg T, Goyal AK, Rath G. 2014b. Herbal and polymeric approaches for liver-targeting drug delivery: novel strategies and their significance. Drug Deliv. 1–17.
- Safioleas M, Misiakos EP, Kakisis J, Manti C, Papachristodoulou A, Lambrou P, et al. 2000. Surgical treatment of human echinococcosis. Int Surg. 85:358–365.
- Sharma A, Garg T, Aman A, Panchal K, Sharma R, Kumar S, Markandeywar T. 2014a. Nanogel-an advanced drug delivery tool: Current and future. Artif Cells Nanomed Biotechnol. 1–13.
- Sharma R, Garg T, Goyal AK, Rath G. 2014b. Development, optimization and evaluation of polymeric electrospun nanofiber: a tool for local delivery of fluconazole for management of vaginal candidiasis. Artif Cells Nanomed Biotechnol. 1–8.
- Sharma R, Singh H, Joshi M, Sharma A, Garg T, Goyal AK, Rath G. 2014c. Recent advances in polymeric electrospun nanofibers for drug delivery. Crit Rev Ther Drug Carrier Syst. 31:187–217.
- Singh B, Garg T, Goyal AK, Rath G. 2014a. Recent advancements in the cardiovascular drug carriers. Artif Cells Nanomed Biotechnol. 1–10.
- Singh H, Sharma R, Joshi M, Garg T, Goyal AK, Rath G. 2014b. Transmucosal delivery of Docetaxel by mucoadhesive polymeric nanofibers. Artif Cells Nanomed Biotechnol.
- Van Eerdenbrugh B, Froyen L, Martens JA, Blaton N, Augustijns P, Brewster M, Van Den Mooter G. 2007. Characterization of physico-chemical properties and pharmaceutical performance of sucrose co-freeze-dried solid nanoparticulate powders of the anti-HIV agent loviride prepared by media milling. Int J Pharm. 338:198–206.