Abstract
In the last few decades, various drug-delivery technologies have emerged and a fascinating part of this has been the development of nanoscale drug delivery devices. Nanoparticles (NPs) and other colloidal drug-delivery systems modify the kinetics, drug distribution in the body and release profile of an associated drug. Nanostructured lipid carriers (NLCs) have been reported to be an alternative system to emulsions, liposomes, microparticles, solid lipid nanoparticles (SLNs) and their polymeric counterparts due to their numerous advantages. This paper basically reviews the types of NLCs, mechanism of skin penetration, stability related issues along with their production techniques, characterisation and applications towards targeted drug delivery.
Introduction
Over the last 20 years, nanotechnology has practically made its influence in all technical fields, including pharmaceutics. Industry estimates suggest that approximately 40% of lipophilic drug candidates fail due to solubility and formulation stability issues, which has been solved by various novel and advanced lipophilic drug delivery technologies (CitationMishra et al. 2010). The lipids employed to prepare lipid nanoparticles are usually physiological lipids (biocompatible and biodegradable) with low acute and chronic toxicity (CitationMüller et al. 1996).
In 1960s the first safe parenteral fat emulsion (Intralipid) was developed by CitationWretlind (1981). Some other interesting carriers systems are the liposomes, multiple emulsions, sub-micron emulsion, niosomes, transferosomes, microsponge drug delivery system etc. Liposomes have been described for the first time by Bangham et al. in 1960s (CitationBangham and Horne 1964). Major obstacles for the development of liposomal formulations were limited physical stability of the dispersions, drug leakage, low targeting ability, non-specific clearance by mononuclear phagocytic system and difficulties in upscaling. Speiser and coworkers developed solid lipid microparticles (by spray drying) and ‘Nanopellets for peroral administration’. Nanosuspensions are the only colloidal particles composed of the drug and the emulsifier which can be prepared by ball milling or high-pressure homogenisation (HPH) (CitationEldem et al. 1991). Similar systems have been described by CitationDomb (1995) as ‘Lipospheres’. Polymeric nanoparticles and polymer nanocapsules, made from non-biodegradable and biodegradable polymers are another innovative carrier systems having high site-specific targeting and controlled release of the incorporated drugs (CitationPatel et al. 2011, CitationMehnert and Mäder 2001). At the beginning of 1990s, the advantages of solid particles, emulsions and liposomes were combined by the development of solid lipid nanoparticles (SLNs). SLNs are the solid, sub-micronic colloidal nano carriers with a size ranging from 1 to 1000 nm and consisting of physiological and biodegradable/biocompatible lipids, capable of incorporating both lipophilic and hydrophilic drugs within the lipid matrix in considerable amounts.
Recently, SLNs based on mixture of solid lipid and liquid lipids (so-called nanostructured lipid carries, NLCs), high amounts of lecithins, amphiphilic cyclodextrins and para-acyl-calix-(4)-arenes have been investigated (CitationMüller et al. 2002, CitationDate et al. 2007). Nanocarriers in various forms, have the endless opportunities in the area of drug delivery which have been recently investigated for their enormous potential. Nanotechnology, as defined by the National Nanotechnology Initiative, is the study and use of structures roughly in the size range of 1–100 nm. The overall goal of nanotechnology is the same as that of medicine: to diagnose as accurately and early as possible and to treat as effectively as possible without any side effects. Some of the important aspects of nanocarriers are highlighted in . Nanocarriers, on account of their higher ratio of surface area to volume, show improved pharmacokinetics and biodistribution of therapeutic agents and thus minimize toxicity by their preferential accumulation at the target site (CitationAlexis et al. 2008).
SLNs vs. NLCs
SLNs have been intensively studied as drug delivery systems for several routes of administration such as peroral, parenteral, dermal and topical delivery. The crystallinity of solid lipids affects the release properties of the SLNs derived which after their preparation; partially crystallize in high-energy modifications with many imperfections in the crystal lattice.
When a polymorphic transition to low-energy modification takes place during storage, the incorporated drug can be expelled from the lipid matrix. SLN have the better chances to be exploited as delivery system in many commercial products. However, there are also certain limitations of this system:
1) Payload for most of drugs is too low;
2) Drug expulsion during storage; and
3) High water content in the nanolipid dispersions.
To overcome drug expulsion during storage, use of lipid blends which do not form a highly ordered crystalline arrangement is needed. The matrix of NLCs is composed of mixture of spatially different lipid molecules, normally mixture of solid and liquid lipid, which makes more imperfection in the matrix to accommodate more drug molecules than SLN. Despite the presence of liquid lipid, NLC matrix is solid at room/body temperature. NLCs adopt mixtures of a solid lipid and liquid lipid and remain in the solid state by controlling the content of liquid lipid. NLCs can more strongly immobilize drugs and prevent the particle from coalescing by virtue of the solid matrix compared to emulsions. NLCs also have the advantages of SLNs including low toxicity, biodegradation, drug protection, slow release and avoidance of organic solvents in production (CitationLiu and Wu 2010, CitationDas and Chaudhary 2011). An overview of various colloidal nanocarriers is shown in .
Advantages of NLCs:
➢ Better physical stability,
➢ Ease of preparation and scale-up,
➢ Increased dispersability in an aqueous medium,
➢ High entrapment of lipophilic drugs and hydrophilic drugs,
➢ Controlled particle size,
➢ An advanced and efficient carrier system in particular for lipophilic substances,
➢ Increase of skin occlusion,
➢ Extended release of the drug,
➢ One of the carriers of choice for topically applied drugs because their lipid components have an approved status or are excipients used in commercially available topical cosmetic or pharmaceutical preparations,
➢ Small size of the lipid particles ensures close contact to the stratum corneum thus enhancing drug penetration into the mucosa or skin,
➢ Improve benefit/risk ratio,
➢ Increase of skin hydration and elasticity (CitationMüller et al. 2009), and
➢ These carriers are highly efficient systems due to their solid lipid matrices, which are also generally recognized as safe or have a regulatory accepted status (CitationAraújo et al. 2009).
In SLNs, the drug is mainly dispersed in molecular form, for example, located in between the fatty acid chains of the glycerides whereas in NLCs, blend of solid and liquid lipids are used and due to their differences in structure they cannot fit together very well to form a perfect crystal. This arrangement creates a lot of imperfections in matrix leading to accommodation of more drugs in molecular form and amorphous clusters (CitationDomingo and Saurina 2012, CitationMüller et al. 2009).
Limitations with lipid nanoparticles:
Despite the great potential of NLCs in targeted delivery, they face certain limitations like:
➢ Cytotoxic effects related to the nature of matrix and concentration,
➢ Irritative and sensitising action of some surfactants,
➢ Application and efficiency in case of protein and peptide drugs and gene delivery systems still need to be better exploited, and
➢ Lack of sufficient preclinical and clinical studies with these nanoparticles in case of bone repair (Schäfer- Korting et al. 2007).
Types of NLCs
Depending on the various production techniques and the composition of the lipid blends, different types of NLCs are obtained. The basic idea is to provide a certain nanostructure for the lipid matrix so as to increase the pay-load for active compounds and reduce the expulsion of compound during storage. The three types of NLCs can be summarised as:
The imperfect type,
The amorphous type, and
The multiple types.
Drug loading capacity in SLNs is limited due to the formation of the lipid crystal. Drug expulsion has been observed due to an ongoing crystallisation process towards a perfect concentration; therefore by avoiding crystallisation, one can avoid these obstacles which is realised in the NLC type 2. The lipid matrix is solid but not crystalline, rather it is in an amorphous state (as shown in ). This can be achieved by mixing special lipids, for example, hydroxyoctacosanyl hydroxystearate with iso-propylmyristate. The form of fine droplets incorporated into the third type of NLC is a multiple system; comparable to w/o/w emulsions, that is oil-in-solid lipid-in-water dispersion. The solid lipid matrix contains tiny liquid oil nano-compartment particles. This type of NLC uses the fact that for a vast category of drugs, the solubility in oils is higher than their solubility in solid lipids (Uner and Yener 2007).
Table I. Types of NLCs (CitationWestesen et al. 1997).
Techniques of production
HPH
HPH has been used as a reliable and powerful technique for the large-scale production of NLCs, lipid drug conjugate, SLNs, and parenteral emulsions. The lipid is pushed with high pressure (100–2000 bars) through a very high shear stress, resulting in disruption of particles down to the sub-micrometer or nanometer range. Normally the lipid contents are in the range of 5–10%. In contrast to other preparation technique, high pressure homogenisation does not show scaling up problem. Homogenisation may be performed either at elevated temperature (hot homogenisation) or below room temperature (cold homogenisation) (CitationSchwarz et al. 1994).
Hot homogenisation technique
In this technique the drug along with melted lipid is dispersed under constant stirring by a high shear device in the aqueous surfactant solution of same temperature. The pre-emulsion obtained is homogenised by using a piston gap homogeniser and the obtained nanoemulsion is cooled down to room temperature where the lipid recrystallises and leads to formation of nanoparticles (Citationzur Mühlen et al. 1998).
Cold homogenisation technique
Cold homogenisation is carried out with the solid lipid containing drug. Cold homogenisation has been developed to overcome the problems of the hot homogenisation technique such as, temperature mediated accelerated degradation of the drug payload, partitioning and hence loss of drug into the aqueous phase during homogenisation. The first step of both the cold and hot homogenisation methods is the same. In the subsequent step, the melt containing drug is cooled rapidly using ice or liquid nitrogen for distribution of drug in the lipid matrix as shown in the . Cold homogenisation minimises the thermal exposure of the sample (CitationGasco 1993).
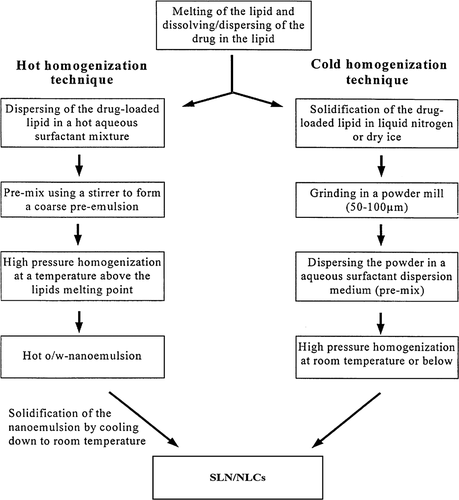
Microemulsion technique
In this technique, the lipids are melted and drug is incorporated in molten lipid. A mixture of water, co-surfactant(s) and the surfactant is heated to the same temperature as the lipids and added under mild stirring to the lipid melt. A transparent, thermodynamically stable system is formed when the compounds are mixed in the correct ratios for microemulsion formation. Thus the microemulsion is the basis for the formation of nanoparticles of a requisite size. This microemulsion is then dispersed in a cold aqueous medium under mild mechanical mixing of hot microemulsion with water in a ratio in the range 1:25–1:50. This dispersion in cold aqueous medium leads to rapid recrystallisation of the oil droplets (CitationMoulik and Paul 1998).
Surfactants and co-surfactants include lecithin; biliar salts alongwith alcohols such as butanol. Excipients such as butanol are less commonly used due to their regulatory aspects. The microemulsion is prepared in a large, temperature-controlled tank and then pumped from this tank into a cold water tank for the precipitation step (CitationGohla and Dingler 2001).
Solvent emulsification-evaporation technique
In solvent emulsification-evaporation method, the lipophilic material and hydrophobic drug are dissolved in a water immiscible organic solvent and emulsified in an aqueous phase using high speed homogeniser. The efficiency of fine emulsification is improved by immediately passing the coarse emulsion through microfluidiser Further the organic solvent is evaporated by mechanical stirring at room temperature and reduced pressure (e.g. rotary evaporator) leaving lipid precipitates nanoparticles (as shown in the ) (CitationShahgaldian et al. 2003).
Solvent emulsification-diffusion technique
This technique can be applied both for the aqueous and oily phase where solvent used must be partially miscible with water. Initially, both the solvent and water are mutually saturated in order to ensure the initial thermodynamic equilibrium of both liquids. During the heating process in order to solubilise the lipid, saturation step is performed at the same temperature. Then the lipid and drug were dissolved in water saturated solvent and this organic phase is stirred using mechanical stirrer. After the formulation of o/w emulsion, water in typical ratio from 1:5 to 1:10, is added to the system in order to allow solvent diffusion into the continuous phase, thus leading to the aggregation of the lipid in the nanoparticles. Both the phases have to be maintained at the same elevated temperature while the diffusion step is performed at room temperature (CitationHu et al. 2002, CitationTrotta et al. 2003).
Melting dispersion method
In melting method, drug and solid lipid are melted in an organic solvent regarded as oil phase, and simultaneously water phase is also heated to the same temperature as oil phase. Subsequently, the oil phase is added to a small volume of water phase and the resulting emulsion is stirred at high speed for few hours. Finally, it is cooled down to room temperature to yield nanoparticles (CitationReithmeier et al. 2001).
High shear homogenisation and/or ultrasonication technique
This technique is one of the less frequently studied methods for the production of lipid nanoparticles. First, the core material is melted followed by the addition of phospholipids along with an aqueous medium, and finally dispersing the melted material at increased temperature by mechanical stirring or ultrasonication. Particle size reduction of the core lipid emulsion with soya lecithin is carried out with the help of ultrasonic energy (CitationPuglia et al. 2008).
Solvent injection
The basic principle of the solvent injection method is similar to the solvent diffusion method. In case of solvent injection method, lipids are dissolved in a water-miscible solvent (e.g. acetone, isopropanol and methanol) or water-miscible solvent mixture and quickly injected into an aqueous solution of surfactants through an injection needle (CitationSchubert and Müller-Goymann 2003). The advantages of this method are the easy handling and fast production process without technically sophisticated equipment (e.g. high-pressure homogeniser). However, the main disadvantage is the use of organic solvents (CitationMüller et al. 2002).
Double emulsion technique
In double emulsion technique the drug (mainly hydrophilic drugs) is dissolved in aqueous solution, and further emulsified in melted lipid. The primary emulsion is stabilised by adding stabiliser that is dispersed in aqueous phase containing hydrophilic emulsifier, which is followed by stirring and filtration. Double emulsion technique avoids the necessity to melt the lipid for the preparation of peptide-loaded lipid nanoparticles and the surface of the nanoparticles could be modified in order to sterically stabilise them by means of the incorporation of lipid-PEG derivatives (CitationDate et al. 2007).
Drug encapsulation in NLCs
There are three ways of incorporating or encapsulating drug within the lipid nanoparticles or NLCs. They are homogenous matrix of solid solution, drug-enriched shell and drug-enriched core (CitationDas and Chaudhary 2011).
(a) Homogenous matrix of solid solution: In this method of encapsulation, the drug is homogenously dispersed into the lipid matrix of the particles and the drug release occurs by diffusion process.
(b) Drug-enriched shell; In this method, the drug is concentrated on to the outer most layer or shell of the lipid nanoparticles. This type of nanoparticles exhibit burst release of the drug due to precipitation and solubilisation mechanism.
(c) Drug-enriched core: In this method, prolonged release is observed due to the saturation solubility of drug in the lipid.
Mechanism of skin penetration and drug release in NLCs
In general, the rate of drug release depends on various factors like the solubility of drug, desorption of the surface bound/adsorbed drug, drug diffusion through the nanoparticles matrix, nanoparticles matrix erosion/degradation and combination of erosion/diffusion process. Thus solubility, diffusion and biodegradation of the matrix materials govern the release process. This type of release can also be triggered by an impulse when the particles are administered (as shown in ). The reason behind higher drug loading is the highly unordered lipid structures found in NLCs. NLCs of certain structures can be triggered this way (CitationRadtke and Müller 2001).
The development of a less ordered solid lipid matrix is desired for a sufficiently high drug-load. In general, the drug can be located in between the fatty acids or in between the lipid layers and also in imperfections (e.g. amorphous). In case of spatially very similar lipid compared to the more or less highly ordered matrix molecules, especially when mono acid highly purified glycerides such as tristearin is used, drug load is very limited and drug expulsion occurs within hours or a few days (CitationWestesen et al. 1997).
Materials employed in formulation of NLCs
The nanolipid carriers for topical application to skin are made up of a variety of lipids such as:
i. Glycerol behenate (Compritol® 888 ATO),
ii. Glycerol palmitostearate (Precirol® ATO 5), and
iii. Cetyl palmitate (wax).
In case of NLCs, at room temperature liquid lipids such as medium chain triglycerides (Miglyol® 812) are frequently used. Alternatively oleic acid, one of the frequently used penetration enhancers in semisolid vehicles applied to the skin, may enhance drug uptake further. They have a mean particle size ranging from 50 to 1000 nm where nanodispersions contain 5–40% of lipid. The preparations of higher concentration are of semisolid appearance and are cosmetically acceptable. Depending on the type and concentration of the lipid, 0.5–5% surfactants are added for physical stabilisation of the particles. Various lipids and surfactants used for preparation of lipid nano carriers are illustrated in and , respectively.
Table II. Lipids used for preparation of nanocarrier (CitationMehnert and Mäder 2001).
Table III. List of surfactants used for preparation of lipid nanocarrier (CitationMehnert and Mäder 2001).
Role of surfactant and lipid in formulation development
The properties and concentrations of surfactant greatly affect the quality and efficacy of nanolipid carrier and lipid nanoparticles. These surface active agents are preferentially located in interfacial regions where they lower the interfacial tension between lipid and aqueous phases because of their amphiphilic nature. Ionic surfactant, sodium deoxycholate, having low emulsification efficiency can be employed to increase the nanoparticles charge which is related to the improvement of electrostatic repulsion leading to improved physical stability of the colloidal system. Non-ionic emulsifier, especially Poloxamer 188, offers additional steric stabilisation effect avoiding aggregation of the fine particles in the colloidal system (CitationLombardi Borgia et al. 2005, CitationKovacevic et al. 2011).
Stability of nanostructured lipid dispersions
NLCs may contain additional colloidal structures, such as micelles, mixed micelles, liposomes and nanoemulsions which contribute to their stability. There are also some major stability issues during storage, such as particle size enhancement, gelation of the dispersion and drug expulsion from the lipid matrix. Gelation takes place due to formation of the network and lipid bridges between the particles. The physical stability of these dispersions is generally investigated by measurement of particle size (Photon correlation spectroscopy, PCS; Laser diffraction, LD), zeta potential (ZP) and thermal analysis (Differential scanning calorimetry, DSC). Several studies indicated physical stability of SLNs dispersion more than 1 year (CitationKakkar et al. 2011).
The long-term storage of lipid dispersions leads to aggregation and shell formation as reported in case of SLNs (CitationFreitas and Müller 1999a). In case of highly concentrated NLC dispersions the particles form a ‘pearl-like network’, thus undergoing collision and perikinetic flocculation. After the administration of NLCs and their dilution with gastrointestinal fluid, the network is destroyed releasing single, non-aggregated particles. Lipid particle dispersions were produced at identical surfactant concentration, but with low lipid content (below 30%, outside patent coverage) and with 35% lipid. The low particle dispersion aggregated during storage time, the gel-like NLC dispersion remained stable during storage and, after dilution, single particles were obtained showing no size increase (CitationFreitas and Müller 1999b). Freely diffusible nanoparticles in low concentration dispersion can collide and aggregate (upper), while in highly concentrated dispersions the particles are fixed in a network, where further dilution with water releases non-aggregated definite nanoparticles (as shown in ).
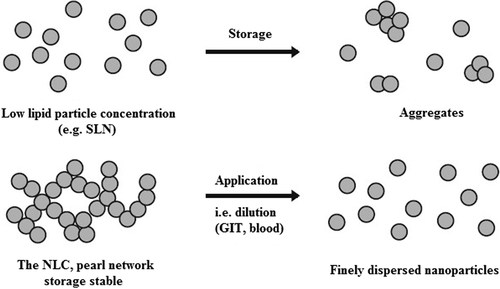
Strategies employed for overcoming the issues related to stability of NLCs
Polyethylene glycol
In general, surface modification of colloidal particles by coating with a hydrophilic substance like polyethylene glycol (PEG) reported to bring following benefits:
Providing good physical stability and dispersability of colloids,
Improving presence of colloids in blood circulation for systemic use,
Increasing stability of colloids in body fluids such as gastrointestinal (GI) fluids,
Acceleration of colloid transport across the epithelium,
Modulation of interaction of colloids with mucosa for specific delivery requirements and drug targeting,
Increasing biocompatibility and decreasing thrombogenicity of drug carriers, and
Providing reservoir function to colloid particles carrying hydrophobic drugs due to hydrophilic coating around the particles.
Spray drying
In addition to the optimised storage conditions, SLNs/NLCs dispersions can also be spray dried to increase their stability. However, melting point of the lipid matrix should be more than 70°C for spray drying.
Lyophilisation
Another efficient way to increase stability is lyophilisation. However, when SLN are lyophilised without cryoprotectant, the final product commonly results in the aggregation of particles. Some of the most widely used cryoprotectants are trehalose, sorbitol, glucose, sucrose, mannose and maltose. Schwarz and Mehnert reported trehalose as the most effective cryoprotectant in preventing particle growth (CitationTrotta et al. 2003).
A variety of drugs has been tested and incorporated in these versatile nano delivery systems for various biomedical applications, as enlisted in (CitationGupta and Vyas 2012, CitationVarshosaz et al. 2012, CitationJia et al. 2010, CitationHan et al. 2012, CitationZhao et al. 2012, CitationChen-yu et al. 2012, CitationAraújo et al. 2011, CitationGokce et al. 2012, CitationLiu et al. 2011, CitationPardeike et al. 2011, CitationZhang et al. 2011, CitationCirri et al. 2012, CitationSouza et al. 2011, CitationNam et al. 2011, CitationSanad et al. 2010, CitationNayak et al. 2010, CitationSchäfer-Korting et al. 2007, CitationTan et al. 2010, CitationDoktorovová et al. 2010, CitationAgrawal et al. 2010, CitationTaratula et al. 2013, CitationPathak and Nagarsenker 2009, CitationZhang et al. 2010, CitationFang et al. 2008, CitationJoshi and Patravale 2008).
Table IV. List of drugs incorporated in NLCs (CitationGupta and Vyas 2012, CitationVarshosaz et al. 2012, CitationJia et al. 2010, CitationHan et al. 2012, CitationZhao et al. 2012, CitationChen-yu et al. 2012, CitationAraújo et al. 2011, CitationGokce et al. 2012, CitationLiu et al. 2011, CitationPardeike et al. 2011, CitationZhang et al. 2011, CitationCirri et al. 2012, CitationSouza et al. 2011, CitationNam et al. 2011, CitationSanad et al. 2010, CitationNayak et al. 2010, CitationSchäfer-Korting et al. 2007, CitationTan et al. 2010, CitationDoktorovová et al. 2010, CitationAgrawal et al. 2010, CitationTaratula et al. 2013, CitationPathak and Nagarsenker 2009, CitationZhang et al. 2010, CitationFang et al. 2008, CitationJoshi and Patravale 2008).
Applications
NLCs as nanolipid carriers find potential application in various fields. The applications are divided in two broader aspects covering the therapeutic applications which include the various routes of administrations in drug delivery and the second part describes the applications in other fields including cosmetics, nutraceuticals, food, chemotherapy and gene delivery. These are discussed below:
Therapeutic applications
Topical delivery
Topical route has been greatly exploited for the drug delivery to dermal areas employing lipid based nanoparticles. In recent years, many studies and experiments have been performed on topical application of NLCs for their unique properties (CitationGupta and Vyas 2012, CitationHan et al. 2012, CitationChen-yu et al. 2012, CitationCirri et al. 2012, CitationNam et al. 2011). NLCs can enhance the apparent solubility of entrapped drugs, which can form high concentration gradient on skin to facilitate drug permeation. The nano-sized particles tightly adhere to the skin surface and release the drugs in a more controlled manner (CitationLiu et al. 2011). Therefore NLCs are used for topical application of various categories of drugs for improvement of penetration along with sustained release. Another benefit of NLCs for topical delivery of active compounds is the short time required to market these products.
Experimental studies have confirmed the significant improvement in therapeutic response and reduction in local side effects of acitretin NLCs loaded gel indicating its effectiveness in the topical treatment of psoriasis. The prepared NLCs were spherical in shape as shown in and the release study showed biphasic drug release pattern with an initial sustained release phase for up to 10 h followed by a steady drug release phase. indicates the release profile of acitretin suspension and acitretin NLC in phosphate buffer pH 7.4 containing 3% w/v sodium lauryl sulfate (CitationAgrawal et al. 2010).
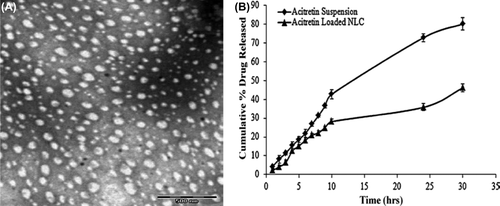
Idebenone loaded nanostructured lipid carriers (I-NLCs) were prepared for topical delivery of antioxidant idebenone and evaluation of its sun protection efficacy. Sun protection factor (SPF) value for I-NLCs was found to be 23 which represents that lipid nanocarriers (LNC) have standards of blocking of 94–96% of Ultraviolet-B rays (CitationSalunkhe et al. 2013). The potential of NLCs loaded with lipophilic calcipotriol and hydrophilic methotrexate for topical therapy of psoriasis was investigated. The study confirmed that NLC systems are a promising carrier for the topical delivery of anti-psoriatic drugs as revealed by enhanced skin permeation, negligible skin irritation and the compatibility of the two drugs (CitationLin et al. 2010). In another study anti-fungal drug ketoconazole loaded NLCs were physically more stable as compared to SLN as the SLN matrix was not able to protect the chemically labile ketoconazole against degradation under light exposure (CitationSouto and Müller 2005).
Oral delivery
NLCs have been proved as one of the beneficial systems for peroral administration of poorly water soluble drugs having low bioavailability. Another important feature is the high dispersivity of NLCs due to which they exhibit a high specific surface area for enzymatic attack by intestinal lipases. Other advantages of giving NLC in oral forms include increased drug loading; improved drug inclusion; patient compliance; high particle concentration and cream like consistency of the carrier.
The mechanisms involved in the absorption of the NLC from the intestine include direct uptake through the GI tract, increase in permeability by surfactants and decreased degradation and clearance. Besides this, the NLCs can also adhere on to the gut wall prolonging the residence time, and consequently the absorption. Poloxamer is involved in deforming the cell membrane and opening of the tight junction of intestinal epithelial cell, thus facilitating paracellular transport of NLCs. Poloxamer 407 also restrains p-glycoprotein efflux pump and increases NLC transport across the intestinal mucosa. Recently, it has been reported that enzyme activity of CYP3A could be inhibited by oleic acid (CitationZhuang et al. 2010, CitationTiwari and Pathak 2011). For example, Lovastatin, a cholesterol-lowering agent used for the treatment of patients with moderate hypercholesterolemia has been incorporated in NLCs that showed increased stability and clinical efficacy (CitationChen et al. 2010).
Parenteral delivery
The nano-drug delivery systems such as nanomicelles, nanoemulsions and nanoparticles has displayed a great potential in improved parenteral delivery of the hydrophobic agents since last two decades. NLC has been considered as an alternative to liposomes and emulsions due to improved properties such as ease in manufacturing, high drug loading, increased flexibility in modulating drug release profile, and alongwith these, their aqueous nature and biocompatibility of the excipients has enabled intravenous delivery of the drug with passive targeting ability and easy abolishment.
Another reported example is NLCs of artemether (Nanoject) that offers significant improvement in the anti-malarial activity and duration of action as compared to the conventional injectable formulation. Nanoject can be considered as a viable alternative to the current injectable intramuscular (IM) formulation (CitationJoshi et al. 2008, CitationJoshi and Müller 2009). Bufadienolides a class C-24 steroid also proved to be effective in terms of enhanced haemolytic activity and cytotoxicity with reduced side effects when incorporated in NLCs (CitationLi et al. 2010).
Ocular drug delivery
Ophthalmic drug delivery with long pre-corneal retention time and high penetration into aqueous humor and intraocular tissues is the key-limiting factor for the treatment of ocular diseases and disorders. Recent reports indicated that NLC could increase the ocular bioavailability of lipophilic drug, ibuprofen. Our previous research showed that NLC could improve the penetration of bioactive compounds into ocular tissues with a good ocular tolerance. Another approach is to increase the transcorneal passage of drugs by incorporating permeation enhancers into formulations like Gelucire 44/14 a type of solid lipid and Transcutol IP that could enhance drug corneal permeability to some extent while stearylamine could prolong the pre-corneal retention of drug; all the three materials could optimise the formulation of a NLC ocular drug delivery and the preparation showed higher bioavailability comparing with eye drops.
Mucoadhesive nanostructured lipid carrier modified by thiolated agent has also been evaluated as a promising carrier for ocular drug delivery in vitro and in vivo. The in vivo distribution investigation indicated that thiolated NLC could prolong pre-corneal residence time, and deliver high cyclosporine (CyA) level into eye tissues in ocular surface and anterior chamber (CitationShen et al. 2010, CitationLi et al. 2008).
Drug delivery to brain
Brain targeting not only increases the cerebro spinal fluid concentration of the drug but also reduces the frequency of dosing and side effects. The major advantages of this administration route are avoidance of first pass metabolism and rapid onset of action as compared to oral administration. LNC (e.g. NLC) of this generation are considered to be one of the major strategies for drug delivery without any modification to the drug molecule because of their rapid uptake by the brain, bioacceptability and biodegradability. Further, the feasibility in scale-up and absence of burst effect make them more promising carriers for drug delivery. In addition, NLC further enhanced the intranasal drug delivery of duloxetine in the brain for the treatment of major depressive disorder. Bromocriptine (BC) a dopamine receptor agonist has been also incorporated in NLCs for controlled delivery of drug to provide long-lasting therapeutic effects possibly extending BC half-life in vivo for the treatment of Parkinson's disease (CitationAlam et al. 2012, CitationEsposito et al. 2012).
Pulmonary drug delivery
Drug delivery via inhalation is also a potential route for the treatment of several pulmonary disorders having advantages over conventional (parenteral and oral) dosage forms like a) non-invasive b) circumventing first pass metabolism and systemic toxicity c) reduced frequent dosing and d) site specificity by directly reaching to the lung epithelium thereby enhancing local drug concentrations. In pulmonary drug delivery systems, surfactants and co-solvents are also often used to prepare stable formulations of highly lipophilic active ingredients.
Few attempts have been made to deliver anti-cancer agents using nanoparticles and liposomes via an inhalation route, but the major limitations being instability during nebulisation, biodegradability, drug leakage and adverse side effects of drug. The lipophilic COX-2 inhibitor, celecoxib, was successfully encapsulated in the NLC nanoparticles using mixture of solid and liquid lipids where most of the nebulised nanoparticles were able to deposit in the alveolar region of the mice lungs and also enhanced the celecoxib lung residence time (CitationPatlolla et al. 2010).
Other applications
Cosmetics
Recently NLCs have been developed based on the controlled nanostructuring of particle matrix which provides immense advantages with respect to loading capacity and long term stability. The various forms in which NLC dispersions can be given are gel, cream, lotion, ointment. The beneficial aspects associated with these NLCs in cosmaceuticals are very broad which lies in, enhancing skin bioavailability of active ingredients, film formation and controlled occlusion, UV protection, penetration enhancement and epidermal targeting, enhancement of physical and chemical stability and in vivo skin hydration (CitationPardeike and Müller 2007).
NLCs greatly increased the in vitro SPF and erythemal UVA protection factor of oxybenzone more than six- and eight-fold, respectively, with fewer side effects. Investigations proved that NLC containing Cutanova Cream NanoRepair Q10 (Dr. Rimpler, Wedemark, Germany), was superior with regard to skin hydration in comparison to a conventional o/w cream having the same composition. NanoLipid Restore CLR (Chemisches Laboratorium, Dr. Kurt Richter, Berlin, Germany) is another semi-finished cosmetic product based on lipid nanoparticles. The easily oxidized black currant seed oil is incorporated in NLCs which are able to protect it against oxidation and enhance the stability of the final product. Another product Surmer (Dr. Rimpler, GmbH, Wedemark, Germany) increases the occlusion of a day cream without changing its light character, that is, achieving higher occlusive properties without having the glossy skin appearance. A prolonged release profile can also be obtained for the perfumes and insect repellents by incorporating them in NLCs (CitationPetersen et al. 2006).
Chemotherapy
Recent studies have shown that NLCs not only enhanced the efficacy and stability but also reduced side effects of many cytotoxic drugs. Different nanosystems have been developed with anti-cancer drugs, for example, the albumin–paclitaxel nanoparticles were approved in early 2005 in the chemotherapy for metastatic breast cancer; etoposide NLCs were found to be cytotoxic against human epithelial-like lung carcinoma cells; stabilisation and prolonged release of topotecan NLCs in treatment of refractory ovarian and small-cell lung cancer. Advantages of incorporating anti-cancer drugs in NLCs include high drug loading efficiency; prolonged release profile; increased chemical stabilisation; increased cytotoxicity.
As these NLCs avoid some potential problems associated with SLN, such as drug leakage during storage and decreased loading capacity. They act by prolonging the exposure of tumour cells to anti-tumour drug and enhancing permeability and retention effect to further increase the therapeutic effect (CitationBharali et al. 2009). It has also been reported that hyaluronic acid coated NLC could prolong the circulation time of paclitaxel (PTX) in blood and increase the accumulation of PTX in the tumour. The results of this experiment indicated that HA-NLCs (hyaluronic acid-coated, paclitaxel-loaded, nanostructured lipid carriers) showed higher anti-tumour efficacy and fewer side effects than Taxol® in B16-bearing Kunming mice as depicted in . The overall targeting efficiency of HA-NLC in the tumour was 14.46%, approximately 1.4 times that of Taxol® (CitationYang et al. 2013).
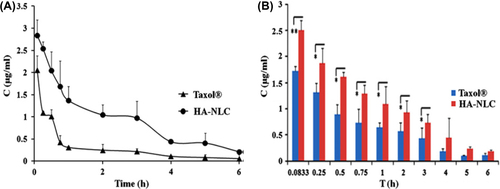
Nutraceuticals
Nutraceuticals are bioactive compounds, which provide medicinal or health benefits, including the prevention, and treatment of diseases. Among them, the carotenoids are one of the most important groups of natural pigments, because of their wide distribution in plant tissues, structural diversity and numerous functions. Carotene-LNC with highly antioxidant and significant anti-bacterial activities were successfully produced by using natural oils and a versatile high-shear homogenisation technique.
Hesperetin (5,7,3′-trihydroxy-4′-methoxy flavanone) belonging to flavonones which is useful in chemically induced mammary tumorigenesis, colon carcinogenesis, heart attack and blood pressure was also successfully encapsulated in NLCs that showed good acceptance, homogeneity, improved taste and enhanced therapeutic effects (CitationLacatusu et al. 2012, CitationFathi et al. 2013).
In food industry
Because of its good stability and high loading capacity, the NLCs are widely applied in the pharmaceutical field. It was seldom reported that the NLC was applied as a nutritional supplement carrier in food industry for the capsule and beverage preparations. However, there are certain difficulties related to the raw material supply, availability and environmental factors due to which there is still a great risk for food industry to invest in this area. Coenzyme Q10-loaded NLCs for food application were developed to enhance the physicochemical stability and bioavailability (CitationLiu et al. 2012).
Gene delivery and gene therapy
Transfer of genes to mammalian cells is the most challenging task to achieve efficient and safe gene therapy. Gene delivery systems are basically divided into two types, viz., viral and non-viral vectors. Viral vectors have been extensively investigated because of their high transfection efficiencies while non-viral vectors have the benefits of low immunogenicity and ease of preparation. However, their efficiency is not quite satisfactory. Colloidal particulate delivery systems like cationic liposomes, SLNs, nanoemulsions, micelles, and some of the polymer based vectors like poly-L-lysine, polyethylenimine (PEI), polyamidoamine dendrimer and chitosan, exhibit significant advantages as potential candidates for efficient non-viral gene delivery. Among them, cationic liposomes and PEI are the most extensively investigated where cationic liposomes form a complex with anionic DNA molecules and deliver DNA through endosomes after endocytosis of the complex (CitationZhang et al. 2008). Lipopolyplexes are used as nanomedicines for successful and efficient gene delivery. These are prepared by combination of gene (RNA/DNA), polycations, and lipids. They are mainly preferred for gene delivery in treatment of various cancers (CitationGarcía et al. 2012). Recently, CitationZhang et al. (2008) demonstrated the contribution of NLCs towards gene delivery, by evaluating the in vitro gene transfer properties of polycationic nanostructured lipid carrier (PNLC) loaded with triolein in human lung adenocarcinoma. Enhanced transfection efficiency of PNLC was observed, which proved that PNLC is an effective non-viral gene transfer vector. CitationZhu et al. (2013) explained the utility of folate nanoliposomes for targeted delivery of siRNA in metastatic neuroblastoma (CitationZhu et al. 2013). Similarly, NLCs are used as multifunctional carrier for targeted delivery of siRNA and anti-cancer drugs. Recently, CitationTaratula et al. (2013) demonstrated the higher efficiency of NLCs for tumour-targeted local delivery by inhalation of anti-cancer drugs and mixture of siRNAs for treatment of lung cancer with efficient suppression of tumour growth and prevention of adverse side effects on healthy organs (CitationTaratula et al. 2013).
Conclusion
The lipid nanoparticles like SLN, NLC, LNC, etc. have always been potential carrier systems with good therapeutic applications. The purpose of this work was to highlight the role of NLCs as a novel drug delivery system for various categories of drugs. They are the new generation, smart, flexible systems offering for enhanced drug loading, modulation of release and improved performance in producing final dosage forms such as creams, tablets, capsules and injectables. The article also emphasises on various production techniques, stability related issues along with the therapeutic and cosmetic applications of NLCs.
LNC (e.g. NLC) of this generation are considered to be one of the major strategies for drug delivery without any modification to the drug molecule because of their rapid uptake, bio-acceptability and biodegradability. The impact of these carrier systems is continuously increasing and thus has bright future prospects. However, their cytotoxic effects related to the nature of matrix and concentration, irritative and sensitising action of some surfactants are the areas of concern. Their application and efficiency in food, protein and peptide drugs, gene delivery systems and other fields still needs to be better exploited.
Acknowledgement
The authors are thankful to the Director, University Institute of Pharmacy, Pt. Ravishankar Shukla University, Raipur (C.G.), India for the present study and All India Council of Technical Engineering (AICTE) and Department of Science and Technology (DST-FIST-SR/FST/LSI – 013/2010) and University Grant Commission [UGC SAP – F.3_54/2011(SAP 2)] for financial assistance.
Declaration of interest
The authors report no declarations of interest. The authors alone are responsible for the content and writing of the paper.
References
- Agrawal Y, Petkar KC, Sawant KK. 2010. Development, evaluation and clinical studies of Acitretin loaded nanostructured lipid carriers for topical treatment of psoriasis. Int J Pharm. 401:93–102.
- Alam MI, Baboota S, Ahuja A, Ali M, Ali J, Sahni JK. 2012. Intranasal administration of nanostructured lipid carriers containing CNS acting drug: pharmacodynamic studies and estimation in blood and brain. J Psychiatr Res. 46:1133–1138.
- Alexis F, Rhee JW, Richie JP, Radovic-Moreno AF, Langer R, Farokhzad OC. 2008. New frontiers in nanotechnology for cancer treatment. Urol Oncol. 26:74–85.
- Araújo J, Gonzalez E, Egea MA, Garcia ML, Souto EB. 2009. Nanomedicines for ocular NSAIDs: safety on drug delivery. Nanomedicine. 5:394–401.
- Araújo J, Nikolic S, Egea MA, Souto EB, Garcia ML. 2011. Nanostructured lipid carriers for triamcinolone acetonide delivery to the posterior segment of the eye. Colloids Surf BBiointerfaces. 88:150–157.
- Bangham AD, Horne RW. 1964. Negative staining of phospholipids and their structural modification by surface active agents as observed in electron microscope. J Mol Biol. 8:660–668.
- Bharali DJ, Khalil M, Gurbuz M, Simone TM, Mousa SA. 2009. Nanoparticles and cancer therapy: a concise review with emphasis on dendrimers. Int J Nanomed. 4:1–7.
- Chen CC, Tsai TH, Huang ZR, Fang JY. 2010. Effects of lipophilic emulsifiers on the oral administration of lovastatin from nanostructured lipid carriers: physicochemical characterization and pharmacokinetics. Eur J Pharm Biopharm. 74:474–482.
- Chen-yu G, Chun-fena Y, Qi-luc L, Qi T, Yan-wei X, Wei-na L, Guang-xi Z. 2012. Development of a quercetin-loaded nanostructured lipid carrier formulation for topical delivery. Int J Pharm. 430:292–298.
- Cirri M, Bragagni M, Mennini N, Mura P. 2012. Development of a new delivery system consisting in ‘‘drug – in cyclodextrin – in nanostructured lipid carriers’’ for ketoprofen topical delivery. Eur J Pharm Biopharm. 80:46–53.
- Das S, Chaudhary A. 2011. Recent advances in lipid nanoparticle formulations with solid matrix for oral drug delivery. AAPS PharmSciTech. 12:62–76.
- Date AA, Joshi MD, Patravale VB. 2007. Parasitic diseases: liposomes and polymeric nanoparticles versus lipid nanoparticles. Adv Drug Deliv Rev. 59:505–521.
- Doktorovová S, Araújo J, Garcia ML, Rakovský E, Souto EB. 2010. Formulating fluticasone propionate in novel PEG-containing nanostructured lipid carriers (PEG-NLC). Colloids Surf BBiointerfaces. 75:538–542.
- Domb AJ. 1995. Long acting injectable oxytetracycline-liposphere formulations. Int J Pharm. 124:271–278.
- Domingo C, Saurina J. 2012. An overview of the analytical characterization of nanostructured drug delivery systems: towards green and sustainable pharmaceuticals: a review. Anal Chim Acta. 744:8–22.
- Eldem T, Speiser P, Hincal A. 1991. Optimization of spray-dried and congelated lipid micropellets and characterization of their surface morphology by scanning electron microscopy. Pharm Res. 8:47–54
- Esposito E, Mariani P, Ravani L, Contado C, Volta M, Bido S, et al. 2012. Nanoparticulate lipid dispersions for bromocriptine delivery: characterization and in vivo study. Eur J Pharm Biopharm. 80:306–314.
- Fang JY, Fang CL, Liu CH, Su YH. 2008. Lipid nanoparticles as vehicles for topical psoralen delivery: solid lipid nanoparticles (SLN) versus nanostructured lipid carriers (NLC). Eur J Pharm Biopharm. 70:633–640.
- Fathi M, Varshosaz J, Mohebbi M, Shahidi F. 2013. Hesperetin-loaded solid lipid nanoparticles and nanostructure lipid carriers for food fortification: preparation, characterization, and modeling. Food Bioprocess Tech. 6:1464–1475.
- Freitas C, Müller RH. 1999a. Correlation between long-term stability of solid lipid nanoparticles (SLN) and crystallinity of the lipid phase. Eur J Pharm Biopharm. 47:125–132.
- Freitas C, Müller RH. 1999b. Stability determination of solid lipid nanoparticles (SLN) in aqueous dispersion after addition of electrolyte. J Microencapsul. 16:59–71.
- García L, Urbiola K, Düzgüneş N, Tros de Ilarduya C. 2012. Lipopolyplexes as nanomedicines for therapeutic gene delivery. Methods Enzymol. 509:327–338.
- Gasco MR. 1993. Method for producing solid lipid microspheres having a narrow size distribution. US Pat. No. 5250236.
- Gohla SH, Dingler A. 2001. Scaling up feasibility of the production of solid lipid nanoparticles (SLN). Pharmazie. 56:61–63.
- Gokce EH, Korkmaz E, Dellera E, Sandri G, Bonferoni MC, Ozer O. 2012. Resveratrol-loaded solid lipid nanoparticles versus nanostructured lipid carriers: evaluation of antioxidant potential for dermal applications. Int J Nanomed. 7:1841–1850.
- Gupta M, Vyas SP. 2012. Development, characterization and in vivo assessment of effective lipidic nanoparticles for dermal delivery of fluconazole against cutaneous candidiasis. Chem Phys Lipids. 165:454–461.
- Han F, Yin R, Che X, Yuan J, Cui Y, Yin H, Li S. 2012. Nanostructured lipid carriers (NLC) based topical gel of flurbiprofen: Design, characterization and in vivo evaluation. Int J Pharm. 439:349–357.
- Hu FQ, Yuan H, Zhang HH, Fang M. 2002. Preparation of solid lipid nanoparticles with clobetasol propionate by a novel solvent diffusion method in aqueous system and physicochemical characterization. Int J Pharm. 239:121–128.
- Jia L, Zhang D, Li Z, Duan C, Wang Y, Feng F, et al. 2010. Nanostructured lipid carriers for parenteral delivery of silybin: biodistribution and pharmacokinetic studies. Colloids Surf BBiointerfaces. 80: 213–218.
- Joshi M, Pathak S, Sharma S, Patravale V. 2008. Design and in vivo pharmacodynamic evaluation of nanostructured lipid carriers for parenteral delivery of artemether: nanoject. Int J Pharm. 364:119–126.
- Joshi M, Patravale V. 2008. Nanostructured lipid carrier (NLC) based gel of celecoxib. Int J Pharm. 346:124–132.
- Joshi MD, Müller RH. 2009. Lipid nanoparticles for parenteral delivery of actives. Eur J Pharm Biopharm. 7:161–172.
- Kakkar V, Singh S, Singla D, Kaur IP. 2011. Exploring solid lipid nanoparticles to enhance the oral bioavailability of curcumin. Mol Nutr Food Res. 55:495–503.
- Kovacevic A, Savic S, Vuleta G, Müller RH, Keck CM. 2011. Polyhydroxy surfactants for the formulation of lipid nanoparticles (SLN and NLC): effects on size, physical stability and particle matrix structure. Int J Pharm. 406:163–172.
- Lacatusu I, Badea N, Ovidiu O, Bojin D, Megha A. 2012. Highly antioxidant carotene-lipid nanocarriers: synthesis and antibacterial activity. J Nanopart Res. 14:902.
- Li F, Weng Y, Wang L, He H, Yang J, Tang X. 2010. The efficacy and safety of bufadienolides-loaded nanostructured lipid carriers. Int J Pharm. 393:203–211.
- Li X, Nie SF, Kong J, Li N, Ju CY, Pan WS. 2008. A controlled-release ocular delivery system for ibuprofen based on nanostructured lipid carriers. Int J Pharm. 363:177–182.
- Lin YK, Huang ZR, Zhuo RZ, Fang JY. 2010. Combination of calcipotriol and methotrexate in nanostructured lipid carriers for topical delivery. Int J Nanomed. 5:117–128.
- Liu CH, Wu CT. 2010. Optimization of nanostructured lipid carriers for lutein delivery. Colloids Surf APhysicochem Eng Aspects. 353: 149–156.
- Liu D, Liu Z, Wang L, Zhang C, Zhang N. 2011. Nanostructured lipid carriers as novel carrier for parenteral delivery of docetaxel. Colloids Surf B Biointerfaces. 85:262–269.
- Liu GY, Wang JM, Xia Q. 2012. Application of nanostructured lipid carrier in food for the improved bioavailability. Eur Food Res Technol. 234:391–398.
- Lombardi Borgia S, Regehly M, Sivaramakrishnan R, Mehnert W, Korting HC, Danker K, et al. 2005. Lipid nanoparticles for skin penetration enhancement—correlation to drug localization within the particle matrix as determined by fluorescence and parelectric spectroscopy. J Control Release. 110:151–163.
- Mehnert W, Mäder K. 2001. Solid lipid nanoparticles: production, characterization and applications. Adv Drug Deliv Rev. 47:165–196.
- Mishra B, Patel BB, Tiwari S. 2010. Colloidal nanocarriers: a review on formulation technology, types and applications toward targeted drug delivery. Nanomedicine. 6:9–24.
- Moulik SP, Paul BK. 1998. Structure, dynamics and transport properties of microemulsions. Adv Colloid Interface Sci. 78:99–195.
- Müller RH, Maassen S, Weyhers H, Mehnert W. 1996. Phagocytic uptake and cytotoxicity of solid lipid nanoparticles (SLN) sterically stabilized with poloxamine 908 and poloxamer 407. J Drug Target. 4:161–170.
- Müller RH, Mader K, Gohla S. 2009. Lipid nanoparticles (SLN, NLC) in cosmetic and pharmaceutical dermal products. Int J Pharm. 366:170–184.
- Müller RH, Radtke M, Wissing SA. 2002. Solid lipid nanoparticles (SLN) and nanostructured lipid carriers (NLC) in cosmetic and dermatological preparations. Adv Drug Deliv Rev. 54:131–155.
- Nam SH, Ji XY, Park JS. 2011. Investigation of tacrolimus loaded nanostructured lipid carriers for topical drug delivery. Bull Korean Chem. 32:956–960.
- Nayak AP, Tiyaboonchai W, Patankar S, Madhusudhan B, Souto EB. 2010. Curcuminoids-loaded lipid nanoparticles: novel approach towards malaria treatment. Colloids Surf B Biointerfaces. 81: 263–273.
- Pardeike J, Müller RH. 2007. Coenzyme Q10 loaded NLCs: preparation, occlusion properties and penetration enhancement. Pharm Technol Eur. 19:46–49.
- Pardeike J, Weber S, Haber T, Wagner J, Zarfl HP, Plank H, Zimmer A. 2011. Development of an itraconazole-loaded nanostructured lipid carrier (NLC) formulation for pulmonary application. Int J Pharm. 419:329–338.
- Patel RP, Singhal GB, Prajapati BG, Patel NA. 2011. Solid lipid nanoparticles and nano lipid carriers: As novel solid lipid based drug carrier. Int Res J Pharm. 2:40–52.
- Pathak P, Nagarsenker M. 2009. Formulation and evaluation of lidocaine lipid nanosystems for dermal delivery. AAPS PharmSciTech. 10:985–992.
- Patlolla RR, Chougule M, Patel AR, Jackson T, Tata PN, Singh M. 2010. Formulation, characterization and pulmonary deposition of nebulized celecoxib encapsulated nanostructured lipid carriers. J Control Release. 144:233–241.
- Petersen RD, Hommoss A, Peter M, Muller RH. 2006. Nanostructured lipid carrier—a delivery system with protective functions. SOFW J. 132:64–69.
- Puglia C, Blasi P, Rizza L, Schoubben A, Bonina F, Rossi C, Ricci M. 2008. Lipid nanoparticles for prolonged topical delivery: an in vitro and in vivo investigation. Int J Pharm. 357:295–304.
- Radtke M, Müller RH. 2001. Nanostructured lipid carriers: the new generation of lipid drug carriers. New Drugs. 2:48–52.
- Reithmeier H, Hermann J, Göpferich A. 2001. Lipid microparticles as a parenteral controlled release device for peptides. J Control Release. 73:339–350.
- Salunkhe SS, Bhatia NM, Pokharkar VB, Throat JD, Bhatia MS. 2013. Topical delivery of Idebenone using nanostructured lipid carriers: evaluations of sun-protection and anti-oxidant effects. J Pharm Investigation. 43:287–303.
- Sanad RA, Abdelmalak NS, Elbayoomy TS, Badawi AA. 2010. Formulation of novel oxybenzone-loaded nanostructured lipid carriers (NLCs). AAPS PharmSciTech. 11:1684–94.
- Schäfer-Korting M, Mehnert W, Korting HC. 2007. Lipid nanoparticles for improved topical application of drugs for skin diseases. Adv Drug Deliv Rev. 59:427–443.
- Schubert MA, Müller-Goymann CC. 2003. Solvent injection as a new approach for manufacturing lipid nanoparticles—evaluation of the method and process parameters. Eur J Pharm Biopharm. 55:125–131.
- Schwarz C, Mehnert W, Lucks JS, Müller RH. 1994. Solid lipid nanoparticles (SLN) for controlled drug delivery. I. Production, characterization and sterilization. J Control Release. 30:83–96.
- Shahgaldian P, Da Silva E, Coleman AW, Rather B, Zaworotko MJ. 2003. Para-acyl-calix-arene based solid lipid nanoparticles (SLNs): a detailed study of preparation and stability parameters. Int J Pharm. 253:23–38.
- Shen J, Deng Y, Jin X, Ping Q, Su Z, Li L. 2010. Thiolated nanostructured lipid carriers as a potential ocular drug delivery system for cyclosporine A: improving in vivo ocular distribution. Int J Pharm. 402:248–253.
- Souto EB, Müller RH. 2005. SLN and NLC for topical delivery of ketoconazole. J Microencapsul. 22:501–510.
- Souza LG, Silva EJ, Martins ALL, Mota MF, Braga RC, Lima EM, et al. 2011. Development of topotecan loaded lipid nanoparticles for chemical stabilization and prolonged release. Eur J Pharm Biopharm. 79:189–196.
- Tan SW, Billa N, Roberts CR, Burley JC. 2010. Surfactant effects on the physical characteristics of Amphotericin B-containing nanostructured lipid carriers. Colloids Surf A Physicochem Eng Aspects. 372:73–79.
- Taratula O, Kuzmov A, Shah M, Garbuzenko OB, Minko T. 2013. Nanostructured lipid carriers as multifunctional nanomedicine platform for pulmonary co-delivery of anticancer drugs and siRNA. J Control Release. 171:349–357.
- Tiwari R, Pathak K. 2011. Nanostructured lipid carrier versus solid lipid nanoparticles of simvastatin: comparative analysis of characteristics, pharmacokinetics and tissue uptake. Int J Pharm. 415:232–243.
- Trotta M, Debernardi F, Caputo O. 2003. Preparation of solid lipid nanoparticles by a solvent emulsification-diffusion technique. Int J Pharm. 257:153–160.
- Melike U, Gulgun Y. 2007. Importance of solid lipid nanoparticles (SLN) in various administration routes and future perspectives. Int J Nanomedecine. 2:289–300.
- Varshosaz J, Hassanzadeh F, Sadeghi H, Andalib S. 2012. Synthesis of octadecylamine-retinoic acid conjugate for enhanced cytotoxic effects of 5-FU using LDL targeted nanostructured lipid carriers. Euro J Med Chem. 54:429–438.
- Westesen K, Bunjes H, Koch MHJ. 1997. Physicochemical characterisation of lipid nanoparticles and evaluation of their drug loading capacity and sustained release potential. J Control Release. 48:223–236.
- Wretlind A. 1981. Development of fat emulsions. JPEN J Parenter Enteral Nutr. 5:230–235.
- Yang XY, Li YX, Li M, Zhang L, Feng LX, Zhang N. 2013. Hyaluronic acid-coated nanostructured lipid carriers for targeting paclitaxel to cancer. Cancer Lett. 334:338–345.
- Zhang T, Chen J, Zhang Y, Shen Q, Pan W. 2011. Characterization and evaluation of nanostructured lipid carrier as a vehicle for oral delivery of etoposide. Eur J Pharm Sci. 43:174–179.
- Zhang X, Liu J, Qiao H, Liu H, Ni J, Zhang W, Shi Y. 2010. Formulation optimization of dihydroartemisinin nanostructured lipid carrier using response surface methodology. Powder Technol. 197:120–128.
- Zhang Z, Sha X, Shen A, Wang Y, Sun Z, Gu Z, Fang X. 2008. Polycation nanostructured lipid carrier, a novel nonviral vector constructed with triolein for efficient gene delivery. Biochem Biophys Res Commun. 370:478–48.
- Zhao C, Liu Y, Fan T, Zhou D, Yang Y, Jin Y, Zhang Z, Huang Y. 2012. A novel strategy for encapsulating poorly soluble drug into nanostructured lipid carriers for intravenous administration. Pharm Dev Technol. 17:443–456.
- Zhu Q, Feng C, Liao W, Zhang Y, Tang S. 2013. Target delivery of MYCN siRNA by folate-nanoliposomes delivery system in a metastatic neuroblastoma model. Cancer Cell Int. 13:65–70.
- Zhuang CY, Li N, Wang M, Zhang XN, Pan WS, Peng JJ, et al. 2010. Preparation and characterization of Vinpocetine loaded nanostructured lipid carriers (NLC) for improved oral bioavailability. Int J Pharm. 394:179–185.
- zur Mühlen A, Schwarz C, Mehnert W. 1998. Solid lipid nanoparticles (SLN) for controlled drug delivery–drug release and release mechanism. Eur J Pharm Biopharm. 45:149–155.