Abstract
Microscopic, fiber-shaped cell aggregates, have been used as building blocks for fabricating macroscopic three-dimensional tissue architectures, in the field of tissue engineering. In this study, we examined the occurrence of necrotic regions in the most widely used, fiber-shaped cell aggregates, approximately 100 μm in diameter. Alginate hydrogel hollow microfibers were used as templates for the cell aggregates. We demonstrated negligible necrotic region formation occurred in the cell aggregates formed in the hollow microfibers. Furthermore, we improved on previously-reported methods for preparing the hollow microfibers to avoid common microfiber tangling during the fiber preparation process.
Introduction
Microscopic cell aggregates, formed during in vitro cell culture, have been used for tissue engineering, regenerative medicine and drug screening. Cells in the cell aggregates appear to mimic the morphology and physiology of those in native tissues, compared to conventional monolayer cell culture systems (CitationSutherland 1988, CitationKoide et al. 1989). Cell aggregates with varying shapes, including spherical (spheroid), fiber-shaped and ring-shaped, have been previously generated (CitationLin and Chang 2008, CitationMasuda et al. 2012, CitationOnoe et al. 2013). It is important during preparation of cell aggregates to avoid the generation of central necrotic regions, which results in decreased functions of the constructs (CitationIjima et al. 1998). Necrotic regions result from an insufficient supply of oxygen and nutrients to the cells. During in vitro cell culture, delivery of oxygen and nutrients to cells in cell aggregates, is based solely on diffusion from the surrounding environment (cell culture media). Therefore, to avoid generation of necrotic cells, reduction and control of the cell aggregate size is essential. In the case of spheroids, the maximum diameter, to avoid formation of necrotic cells, is reported to be less than 150–200 μm (CitationLin and Chang 2008).
Recently, fiber-shaped cell aggregates have been utilized as building blocks for fabricating macroscopic three-dimensional (3D) tissue architecture, in the field of tissue engineering (CitationMatsunaga et al. 2011). The cell aggregates were prepared using flexible alginate hydrogel hollow microfibers (CitationSugiura et al. 2008, CitationSakai et al. 2009, Citation2013, CitationRaof et al. 2011). In the previous reports, cells were first encapsulated in the hollow core of the fibers and proliferated to form fiber-shaped cell aggregates. It appears obvious, that the maximum diameter for the cell aggregates to avoid formation of necrotic regions, is smaller than that (150–200 μm as described above) of spheroids, because their surface area per unit volume is smaller. Therefore, fiber-shaped cell aggregates, approximately 100 μm in diameter, have been most widely prepared in previous reports (CitationSugiura et al. 2008, CitationRaof et al. 2011, CitationOnoe et al. 2013, CitationSakai et al. 2013). However, to our knowledge, no reports demonstrated that there were no necrotic regions in the cell aggregates.
In this study, our primary aim was to investigate occurrence of necrotic regions in fiber-shaped cell aggregates, approximately 100 μm in diameter. Our findings will impact on the advance of research when applying cell aggregates for tissue engineering, regenerative medicine and drug screening. In addition to our primary aim, we wanted to improve on previous methods for preparing alginate hydrogel hollow microfibers, to avoid common microfiber tangling during the fiber preparation process, which would hinder application of the microfibers for tissue engineering and regenerative medicine (see Results and discussion for details).
Materials and methods
Materials and cells
Sodium alginate (Na-Alg), with a molecular weight of 70,000 (61% guluronic acid residues, Kimica I-1G), was kindly donated by Kimica Co. (Tokyo, Japan). Poly(vinyl alcohol) (PVA, degree of saponification: ≥ 96%, degree of polymerization: 400–600) was purchased from Wako Pure Chemical Industries (Osaka, Japan). A human hepatoblastoma cell line (HepG2, RCB1648) was obtained from Riken Cell Bank (Tsukuba, Japan) and was maintained in Dulbecco's Modified Eagle's Medium (DMEM) containing 10% (v/v) fetal bovine serum (FBS), 100 U/ml penicillin and 100 μg/ml streptomycin.
Preparation of microfibers
A coaxial double cylinder was constructed from two stainless steel needles cut at 90° to their axial direction (). Tip inner (id) and outer (od) diameters of the inner cylinder were 470 and 730 μm, respectively. Three outer cylinders with different id at their tips (240, 610 and 910 μm) were employed. The double cylinder was set at the bottom of a bath containing CaCl2 solution. Aqueous solutions of 10% (w/v) PVA (core solution) and 1% (w/v) Na-Alg solutions (sheath solution) were extruded simultaneously into CaCl2 solution from the inner and outer cylinders, respectively, using syringe pumps. The sheath solution gelled through uptake of Ca2+ in the CaCl2 solution (the core solution did not form a hydrogel with Ca2+). The resultant alginate hydrogel hollow microfibers were collected by a roller. The microfibers were then incubated in a large volume of 100 mM CaCl2 solution for 24 h to induce further gelation. Mean microfiber id and od were determined from measurements of approximately 100 randomly selected samples, using an optical microscope (DM IL LED, Leica, Tokyo, Japan). PVA was used to increase viscosity of the core solution, which was necessary to enable homogeneous distribution of cells in the solution during fiber preparation process.
HepG2 cell-enclosed microfibers were prepared using 10% (w/v) PVA in calcium-free Krebs-Ringer HEPES-buffered solution (CF-KRH, pH 7.4) suspending the cells (1.0 × 106 cells/ml), 1% (w/v) sodium alginate in CF-KRH and 100 mM CaCl2/10 mM HEPES solution (pH 7.4) under otherwise identical conditions. The microfibers were incubated in DMEM containing 10% (v/v) FBS at 37°C and 5% CO2 to proliferate the cells in the hollow core. The microfibers containing cell aggregates were embedded in paraffin, sliced and stained with hematoxylin and eosin. Thickness of living cell layers in the cell aggregates was evaluated by measuring the thickness in more than 30 randomly selected parts in cross-sections of the cell aggregates. The ratio of necrotic area in cell aggregates ( = necrotic area in cross-sections of cell aggregates × 100/cross-sectional area of the cell aggregates) was also calculated.
Statistical analysis
Data are presented as mean ± standard deviation. Statistical differences between two groups were analyzed using two-tailed Student's unpaired t-test.
Results and discussion
Some researchers fabricated flexible, alginate hydrogel hollow microfibers, and applied them to preparing fiber-shaped cell aggregates (CitationSugiura et al. 2008, CitationRaof et al. 2011, CitationOnoe et al. 2013, CitationSakai et al. 2013). We also previously developed methods to produce cell-enclosed alginate hydrogel microfibers in both solid and hollow structures, using coaxial double and triple cylinders, respectively (CitationTakei et al. 2006, Citation2007a, Citation2007b, CitationSakai et al. 2008, CitationTakei et al. 2010). A common issue of our and other researcher's methods for fabricating the cell-enclosed microfibers is unavoidable tangle of the fibers immersed in aqueous solutions due to their flexibility and length (> 1 m). Tangle leads to failure of high-order assembly (folding, bundling and weaving) of the cell aggregates formed in the microfibers, required for creating 3D tissue architectures (CitationOnoe et al. 2013). In this study therefore, we first modified on the previous methods to prevent tangle. In our present system, microfibers formed in a bath containing CaCl2 solution were pulled and continuously reeled by a roller (). This procedure was able to prevent tangle during the fiber preparation process.
The primary purpose of this study was to examine whether necrotic regions occurred in fiber-shaped cell aggregates, approximately 100 μm in diameter. To prepare these structures, precise control of the alginate hydrogel hollow microfiber size is essential. Here, we investigated the effect of preparation parameters on microfiber size, because there were no reports in which the size was controlled using an identical system to our present system. We first determined microfiber size by varying the tip id of the outer cylinders and flow rates of the core and sheath solutions at the tip (structure of the double cylinder ensured that the flow rates of the two solutions were identical at the tip). This was achieved by ensuring that core volume ratio ( = flow volume of core solution/total flow volume of core and sheath solutions) and pulling rate of the microfibers ( = rotation speed of the roller) were fixed at 0.1 and 28.8 cm/s, respectively. At a tip id of 240 μm and flow rate of 11.8 cm/s, the minimum microfiber od was 107 ± 10 μm (). The microfiber od increased with increasing flow rate. Tip id also influenced microfiber od. The od increased with increased tip id, and the maximum value was 565 ± 43 μm, with a tip id of 910 μm and flow rate of 21.8 cm/s.
![Figure 2. Effects of outer cylinder tip id [910 μm (circles), 610 μm (triangles) and 240 μm (squares)] and flow rates of core and sheath solutions at the tip on od (a) and i/o ratio (b) of the microfibers.](/cms/asset/0ee5368c-d876-4824-a1d7-26f13907ba67/ianb_a_909824_f0002_b.gif)
shows i/o ratio ( = mean id/mean od of microfibers) for each condition shown in . Theoretically, the i/o ratio depends only on the core volume ratio. Actually, the i/o ratio hardly changed at fixed core volume ratio of 0.1, showing precision of our system. We subsequently examined the effect of core volume ratio on microfiber id and od in more detail under the condition that flow rates of core and sheath solutions and pulling rate of microfibers were fixed at 17.9 and 28.8 cm/s, respectively. Core volume ratio hardly influenced microfiber od when the outer cylinder tip id was fixed. Conversely, microfiber id varied by changing core volume ratio () and an id of approximately 100 μm, adequate to prepare fiber-shaped cell aggregates of approximately 100 μm in diameter, was achieved.
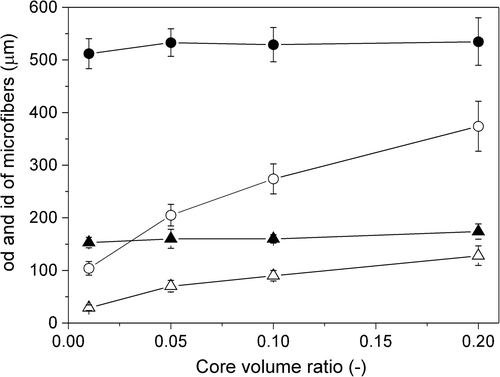
Finally, we attempted to prepare fiber-shaped cell aggregates, approximately 100 μm in diameter, using thin microfibers, 96 ± 11 μm in id prepared at a core volume ratio of 0.10 and outer cylinder tip id of 240 μm (see ). For the control group, cells were encapsulated in thick microfibers, 351 ± 25 μm in id prepared at a core volume ratio of 0.20 and outer cylinder tip id of 910 μm. HepG2 cells were used as a model cell. As shown in , HepG2 cells encapsulated in thin microfibers, proliferated and eventually formed fiber-shaped cell aggregates with identical diameter to microfiber id. Cells in the thick microfibers also formed cell aggregates. Hematoxylin and eosin-stained sections of the cell aggregates formed in thick microfibers showed existence of necrotic regions in the center of the aggregates (). The ratio of necrotic area and thickness of living cell layers in the thick cell aggregates were 28 ± 9% and 73 ± 22 μm, respectively. On the other hand, no necrotic regions were observed in the cell aggregates formed in the thin microfibers (, ratio of necrotic area = 0%, p < 0.001 vs. thick cell aggregates). These results show that the most widely used fiber-shaped cell aggregates, approximately 100 μm in diameter, are useful tools for tissue engineering, regenerative medicine and drug screening, because of the absence of necrotic regions.
Conclusions
In this study, we improved on previous methods for preparing alginate hydrogel hollow microfibers to prevent tangle of the microfibers. Furthermore, we demonstrated that necrotic regions are absent in the most widely used, fiber-shaped cell aggregates, approximately 100 μm in diameter.
Declaration of interest
The authors report no declarations of interest. The authors alone are responsible for the content and writing of the paper.
This work was supported by a Grant-in-Aid for Young Scientists (B), 25820383, 2013, from Japan Society for the Promotion of Science (JSPS).
References
- Ijima H, Matsushita T, Nakazawa K, Fujii Y, Funatsu K. 1998. Hepatocyte spheroids in polyurethane foams: functional analysis and application for a hybrid artificial liver. Tissue Eng. 4:213–226.
- Koide N, Shinji T, Tanabe T, Asano K, Kawaguchi M, Sakaguchi K, et al. 1989. Continued high albumin production by multicellular spheroids of adult-rat hepatocytes formed in the presence of liver-derived proteoglycans. Biochem Biophys Res Commun. 161:385–391.
- Lin RZ, Chang HY. 2008. Recent advances in three-dimensional multicellular spheroid culture for biomedical research. Biotechnol J. 3:1172–1184.
- Masuda T, Takei N, Nakano T, Anada T, Suzuki O, Arai F. 2012. A microfabricated platform to form three-dimensional toroidal multicellular aggregate. Biomed Microdevices. 14:1085–1093.
- Matsunaga YT, Morimoto Y, Takeuchi S. 2011. Molding cell beads for rapid construction of macroscopic 3D tissue architecture. Adv Mater. 23:H90–H94.
- Onoe H, Okitsu T, Itou A, Kato-Negishi M, Gojo R, Kiriya D, et al. 2013. Metre-long cell-laden microfibres exhibit tissue morphologies and functions. Nat Mater. 12:584–590.
- Raof NA, Padgen MR, Gracias AR, Bergkvist M, Xie YB. 2011. One- dimensional self-assembly of mouse embryonic stem cells using an array of hydrogel microstrands. Biomaterials. 32:4498–4505.
- Sakai S, Yamaguchi S, Takei T, Kawakami K. 2008. Oxidized alginate-crosslinked alginate/gelatin hydrogel fibers for fabricating tubular constructs with layered smooth muscle cells and endothelial cells in collagen gel. Biomacromolecules. 9:2036–2041.
- Sakai S, Ito S, Ogushi Y, Hashimoto I, Hosoda N, Sawae Y, Kawakami K. 2009. Enzymatically fabricated and degradable microcapsules for production of multicellular spheroids with well-defined diameters of less than 150 μm. Biomaterials. 30:5937–5942.
- Sakai S, Liu Y, Mah EJ, Taya M. 2013. Horseradish peroxidase/catalase- mediated cell-laden alginate-based hydrogel tube production in two-phase coaxial flow of aqueous solutions for filament-like tissues fabrication. Biofabrication. 5:015012.
- Sugiura S, Oda T, Aoyagi Y, Satake M, Ohkohchi N, Nakajima M. 2008. Tubular gel fabrication and cell encapsulation in laminar flow stream formed by microfabricated nozzle array. Lab Chip. 8:1255–1257.
- Sutherland RM. 1988. Cell and environment interactions in tumor microregions - the multicell spheroid model. Science. 240:177–184.
- Takei T, Sakai S, Ijima H, Kawakami K. 2006. Development of mammalian cell-enclosing calcium-alginate hydrogel fibers in a co-flowing stream. Biotechnol J. 1:1014–1017.
- Takei T, Sakai S, Ijima H, Kawakami K. 2007a. Expression of a liver- specific function by a hepatoblastoma cell line cocultured with three-dimensional endothelialized tubes in collagen gels. J Biosci Bioeng. 103:200–202.
- Takei T, Sakai S, Yokonuma T, Ijima H, Kawakami K. 2007b. Fabrication of artificial endothelialized tubes with predetermined three-dimensional configuration from flexible cell-enclosing alginate fibers. Biotechnol Prog. 23:182–186.
- Takei T, Kishihara N, Sakai S, Kawakami K. 2010. Novel technique to control inner and outer diameter of calcium-alginate hydrogel hollow microfibers, and immobilization of mammalian cells. Biochem Eng J. 49:143–147.