Abstract
A novel biosensor for determination of L-glutamine in pharmaceutical glutamine powder was developed via immobilizing our produced glutaminase enzyme from Hypocria jecorina onto our prepared zinc oxide (ZnO) nanorod and chitosan. ZnO nanorods were prepared as surface-dependent and surface-independent and both were used. The biosensor is specific for L-glutamine and the peculiar analytical properties (linearity range, reproducibility, and accuracy) of it were experimentally determined. The optimum operating conditions of the biosensor such as buffer concentration, buffer pH, and medium temperature effect on the response of biosensor were studied. Km and Vmax values for the our-producing glutaminase enzyme from Hypocria jecorina immobilized on the biosensor were also determined as 0.29 mM and 208.33 mV/min., respectively, from Lineweaver–Burk plot. The biosensor was then used for the determination of glutamine contained in pharmaceutical formulations.
Introduction
The demand for fast, reliable, and continuous measurements of chemical species in medicine, biotechnology and environmental sciences has evolved the need for small, easy to handle, and inexpensive analytic devices (CitationMoser 2002).
Recently, nanomaterials have attracted tremendous interest due to their important performance. Metal oxide semiconductors have attracted much interest because of its broad range of applications in electronics, sensors, transducers, and biomedical sciences. These nanostructures also show high electron transfer kinetics and strong adsorption capability, providing suitable microenvironments for the immobilization of biomolecules and improved biosensing characteristics (CitationLiu et al. 2010, CitationGreene et al. 2005). Among them, zinc oxide (ZnO) nanoparticle is one of the most important nanomaterials for nanotechnology because it is biocompatible, biodegradable, and biosafe for biotechnological and medical applications in today's research (CitationZhang et al. 2012). ZnO nanoparticles can be prepared on a large scale at low cost. There are several methods for preparation of the ZnO nanoparticles such as chemical precipitation (CitationLupan et al. 2007a, Citation2007b), sol-gel synthesis (CitationKim and Kim 2003), and solvothermal/hydrothermal reaction (CitationOgata et al. 2003, CitationWan et al. 2003, CitationGrabowska et al. 2005, CitationAneesh et al. 2007). Because of low processing temperature and very easy to control the particle size, hydrothermal technique has more advances than the other methods. The method have also several advantage such as use of simple equipment, catalyst-free growth, low cost, large area uniform production, environmental friendliness, and less hazardous. The particle properties such as morphology and size can be controlled via the hydrothermal process by adjusting the reaction temperature, time and concentration of precursors. (CitationPolsongkrama et al. 2008).
Glutamine is an important energy and nitrogen source used in the culturing of eukaryotic cells (CitationDattelbaum and Lakowicz 2001). It creates 60% of amino acid pool in muscles, 20% of the total amino acid cycle in the body. Glutamine metabolism is closely related to the diseases involving ammonia imbalance such as animonia intoxication, hyperammonemias, and protein intolerance (CitationScriver et al. 1977). Glutamine concentrations in body fluids are in the millimolar range and its level is closely related to many disease states (CitationAltman and Dittmer 1961). For example, it has been observed that glutamine levels increased in the cerebrospinal fluid during hepatic coma and Reye's syndrome in children. There is a 300% increase in glutamine level in rat brain during seizure, and a 50% decrease in muscle and plasma glutamine of patients with acute pancreatitis after a major injury and during sepsis (CitationScriver et al. 1977, CitationRoth et al. 1985).
Glutamine also takes place in amino acid supplements as capsule or powder forms are available. Glutamine has an important role in protein metabolism and it is the most important amino acid for the developers of the body (CitationDattelbaum and Lakowicz 2001). Despite its importance and ubiquitous presence in the body, glutamine is not routinely determined in clinical laboratories. One reason for omission may be the absence of a standard method for the measurement of glutamine (CitationVillarta et al. 1992).
L-Glutaminase is widely distributed in animal tissues, plants, and microorganisms including bacteria, fungi, and yeast (CitationKatikala et al. 2009). L-glutaminase production via various bacterial genera under submerged culture conditions was extensively studied (CitationWade et al. 1971, CitationImada et al. 1973, CitationSaxena and Sinha 1981, CitationYano et al. 1988, CitationShindia et al. 2007). Furthermore, submerged cultures of Verticillium malthousei (CitationImada et al. 1973), Aspergillus sojae (CitationYamamoto and Hirooka 1974), Aspergillus nidulans (CitationSaxena and Sinha 1981), Tilachlidium humicola (CitationYano et al. 1988), and Penicillium notatum (CitationShindia et al. 2007) had been used for L-glutaminases production. Although Hypocrea jecorina (anamorph Trichoderma reesei) is an industrially important producer of cellulase for an applications portfolio that includes pulp and paper processing, food and feed processing, textile manufacturing and modification, and detergents/cleaners formulation, it is potentially used of all areas of enzyme production (Bülbül and Karakuş 2013).
This paper presents a novel glutamine biosensor to determine glutamine in GNC glutamine powder. The biosensor was prepared via immobilizing our-producing glutaminase from Hypocria jecorina on our-prepared ZnO nanorod and chitosan. The bioanalytical characterization of the proposed biosensor was also carried out.
Experimental
Reagents and instruments
L-glutamine, chitosane, L-ascorbic acid, uric acid, glucose, potato dextrose agar (PDA), ethanol, KH2PO4, MgSO4.7H2O, KCl, NaCl, NaOH, NaH2PO4, Na2HPO4, KI, and HgCl2 were purchased from Sigma Chemical (St. Louis, Mo, USA). Hexamethylenetetramine (HMT) and zinc nitrate tetrahydrate were purchased from Merck (Darmstadt, Germany). ORION 4-Star benchtop pH-ion meter was used for potential measurements. Sartorius PB-11 pH meter was used to measure the pH of all solution. The potential values were given against the Ag/AgCl double junction reference electrode (ORION 90-02). All measurements were made with a 25-ml glass cell. A magnetic stirrer was used throughout the experiments.
All other chemicals used were of analytical reagent grade. Standard solutions and buffer solutions were prepared with deionized water. The absorbance measurements were carried out with Agilent UV-Vis spectrophotometer. Sartorius-PB11 model pH-meter was used for pH measurements. The incubation, sterilization, heating, cooling, and storing procedures were made with VWR-incubating mini shaker, Clifton brand water bath, Certoclav brand autoclave, Memmert brand oven, Arçelik brand refrigerator, respectively. Deionized water was obtained from Millipore MilliQ Gradient System. Our composed ZnO nanorods were imaged using Zeiss EVO LS-10 branded scanning electron microscope (SEM). The GNC-brand glutamine powder was used for glutamine assay.
Microorganism
In this study, Hypocrea jecorina microorganism obtained from Institute of Biochemical Technology and Microbiology, Vienna Technical University was used.
Cultivation
In order to sustain the growth and vitality of Hypocrea jecorina microorganism, PDA was prepared. For this purpose, 39 g of PDA was dissolved in water and the final solution volume was completed to 1 l with distilled water. This PDA solution was sterilized through autoclaving for 20 minutes at 121°C. After sterilization, it was kept at 45°C in water bath and the solution was apportion into petri dishes and allowed to cool. The solidified media was stored in a refrigerator at + 4°C until used. For preparing PDA slant agar medium, 15 mL of the solution prepared was apportion into each tube and closed lids of the tubes and sterilized through autoclaving for 20 minutes at 121°C. After sterilization process, the tubes were allowed to cool positioning of flat. The solidified media were stored at + 4°C until used.
H. jecorina was planted into each petri dish containing solidified culture media with sterile loop and incubated for 7 days in an incubator set at 30°C. After incubation period of 7 days the petri dishes contained produced H. jecorina were covered with parafilm and stored at 4°C until used. This procedure was repeated when needed fresh H. jecorina. To maintain vitality of the mold strains were stored at 4°C, it was transferred to fresh mediums once a month.
To produce crude L-glutaminase enzyme extract from H. jecorina, we used the our-modified fermenting liquid media method proposed by El-Sayed's method (CitationEl-Sayed 2009). The medium contains these components (in grams per liter): glucose as carbon source 1.0, KH2PO4 0.1, MgSO4.7H2O 0.05, KCl 0.05, NaCl 5.0, and L-glutamine as nitrogen source 4.0. After sterilization of 100 mL of the solution containing these liquid media components at 121°C for 20 minutes, the solution was incubated in water bath at 30°C for 30 minutes. 2 mL of H. jecorina pure culture was added into this sterilized solution medium and incubated at 30°C and 150 rpm in shaking incubator for 30 minutes. After incubation of 30 minutes, we measured L-glutaminase activity. Experiments and enzymatic assay were performed in triplicates for each sample.
To maximize the yield of L-glutaminase from Hypocrea jecorina, several parameters such as effect of substrate and salt concentrations were investigated.
To determine the optimum substrate concentration for maximum L-glutaminase activity, it was prepared culture medium contained L-glutamine concentration ranging from 1% to 5% w/v separately. The effect of different doses of NaCl (0–15% w/v) to the production medium to maximize enzyme activity was also studied. The effects of substrate and salt concentration on the enzyme production were evaluated by measuring L-glutaminase enzyme activity. The optimized parameter was incorporated at its optimized level in the all experiments. All the experiments were conducted in triplicate. After incubation of each fermentation sample, the crude enzyme was prepared. All of these experimental studies were presented in our previous study (CitationBülbül and Karakuş 2013).
Preparation of surface-dependent and surface-independent ZnO nanorods
ZnO nanorod was prepared as both surface-dependent (silver, gold wire or sheet, etc.) and surface-independent. In this study, both ZnO nanorods were produced using hydrothermal method (CitationÇoğal 2009).
In order to prepare the surface-dependent ZnO nanorod, the silver wire washed with acetone, ethanol, and bidistilled water was immersed in the solution composed of 25 ml of Zn (NO3)2 (0.025 M) and 0.025 M 25 ml of HMT (0.025 M) at 95°C and incubated for 2–4 hours to evaporate the solution. White-colored layer on the surface of silver wire indicates the formation of ZnO nanorod. The ZnO-coated wire was washed with bidistilled water and allowed to dry at room temperature for 5 min. The resulting SEM image of the ZnO nanorod was obtained ().
In order to prepare the surface-independent ZnO nanorod, the silver wire washed with acetone, ethanol, and bidistilled water was immersed in the solution composed of 0.025 M 25 ml Zn (NO3)2 and 0.025 M 25 ml HMT at 95°C and incubated for 2–4 hours to completely evaporate the solution. The surface-independent ZnO nanorod was obtained by scrapping the white layer at the bottom of the beaker.
Preparation of glutamine biosensor
Glutamine biosensor was prepared using both surface-dependent and surface-independent ZnO nanorod. After surface-dependent ZnO nanorod was incubated into 0.15 M phosphate buffer (pH 7.4) for 5 min to occur on the hydrophilic surface on nanorod surface, it was dipped into the solution containing 1 ml of glutaminase enzyme solution from H. jecorina (pH 7.4) and 0.5 ml chitosan (5%) for 30 minutes to provide electrostatically and chemically immobilization of the enzyme onto nanorod and chitosan, respectively. The prepared sensor was allowed to dry at + 4°C for 5 minutes and dipped into the solution containing 1 ml of 5% of chitosan and 2 mg of surface-independent ZnO nanorod for 15 min + 4°C and dried for 15 min at same temperature. As a result of this, it was provided to be hold surface-independent ZnO nanorod particles and cover chitosan layer onto surface-dependent ZnO nanorod surface. After drying, the biosensor was incubated in the solution of 1 ml of glutaminase enzyme solution from H. jecorina (pH 7.4) for 1 h and allowed to dry for 15 minutes at + 4°C and our glutamine biosensor was obtained. The scheme of the our produced-glutamine biosensor is shown in . When not in use, biosensors were stored in a refrigerator at 4°C.
Measurement of the biosensor response to glutamine
Potential measurements for the glutamine biosensor were carried out by varying glutamine concentration in steady-state condition. 0.1 M of phosphate buffer was used as a working buffer solution at optimum pH for each biosensor. Measurements were made with the proposed glutamine biosensor and Ag/AgCl reference electrode. Biosensor was immersed to a depth of 1.5 cm in glutamine solution that was stirred using a magnetic stirrer. All the experimental works were carried out at room temperature (20–25°C). The calibration curves were obtained by plotting the potential values of a series of standard glutamine solution against the logarithm of glutamine concentration.
Procedure for determination of glutamine in commercial glutamine powder
It was used the standard addition method to determine the glutamine amount in commercial glutamine powder using our prepared glutamine biosensor. Firstly, the biosensor was immersed in 0.15 M of phosphate buffer containing certain amount of serum samples at pH 7.4. Then, stock glutamine solution (1.0 × 10− 2 M pH 7.4) was added to this solution step-by-step. After each step, potential values against added amount (mL) of glutamine solution were plotted and glutamine amount was determined using our prepared glutamine biosensor. It was checked with our results obtained from the proposed biosensor by comparing this values with reported values.
Results and discussion
The calibration curve of glutamine biosensor for glutamine is shown at .
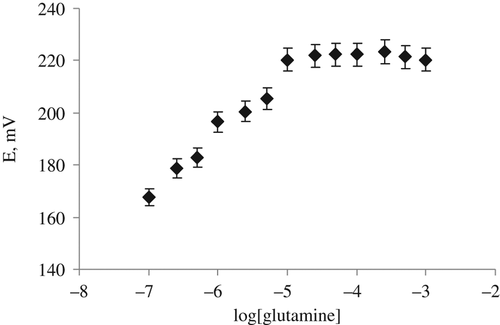
As it can be seen from the calibration graph of the biosensor in , the slope of the glutamine biosensor is 37.2 mV/log[glutamine], which is closed to Nernstian slope value of 59 mV/substrate concentration. The working concentration range of the biosensor is 1.0 × 10− 5–1.0 × 10− 7 M. It will be possible to be carried out in small glutamine concentration. It is an advantages for a biosensor to determine the concentration of the analyte in real samples. Because the glutamine selectivity of our prepared glutamine biosensor— based on glutaminase from H. jecorina—ZnO nanorod and chitosane are good enough, we have decided that the our prepared glutamine biosensor can be used for glutamine determination in real samples.
Effect of buffer concentration
The slope values of the biosensor were determined at six different buffer concentrations varying from 0.05 M to 0.2 M. Maximum performance was obtained at 0.15 M phosphate buffer to determine the buffer concentration in response of the glutamine biosensor ().
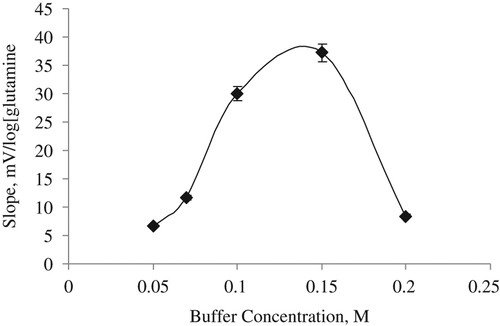
In this study, the highest slope is obtained for the glutamine biosensor that was found to be 0.15 M (). It can be said that the biosensor response was lower at the lower buffer concentrations than 0.15 M because of the lower buffering capacity. Phosphate buffer was generally selected for glutamine biosensor as working buffer as that of us (CitationMoser et al. 1995, CitationVillarta et al. 1992).
Effect of pH
The pH effect on the analytical signal of the glutamine biosensor based on ZnO nanorod, chitosan, and glutaminase from H. jecorina was investigated by measuring phosphate buffer at six different pH in range of 6.5–7.6. The results obtained are presented in .
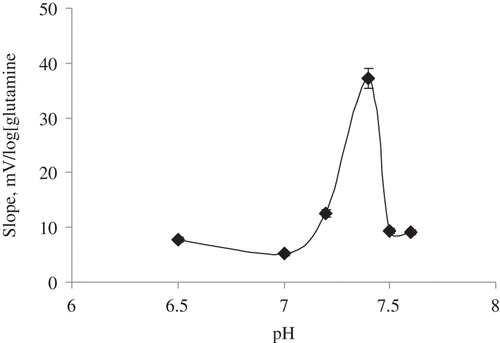
It is shown in that the slopes and sensitivity of glutamine biosensor prepared with glutaminase from H. jecorina are sharply decreasing after pH 7.4 because the glutaminase enzyme immobilized onto the biosensor is denaturated (CitationKarakuş et al. 2005). The optimum pH of the biosensor was determined as pH 7.4 (). Although CitationWakayama et al. (2005) identified glutaminase enzyme was more stable at pH 8.0 (CitationWakayama et al. 2005), it was generally selected neutral pH to study with glutamine biosensors (CitationMoser et al. 1995, CitationVillarta et al. 1992). Other measurements of the biosensor were made at the optimum pH.
Effect of temperature
After glutamine calibration solutions were prepared at optimum conditions of the our prepared glutamine biosensor, we separately studied its response at seven different temperatures varying from 5°C to 40°C. The slope values of the biosensor were determined by plotting calibration graphs for each temperature. Because the maximum slope value was obtained at 25°C, its optimum temperature was determined as 25°C (). This is good result for the biosensor because we can study at room temperature.
Interference effects on the glutamine biosensor
In order to determine the interference effects of L-ascorbic acid and uric acid on the biosensor, the solutions of glutamine, L-ascorbic acid and uric acid were prepared at different concentrations between 10 − 7 M and 10 − 5 M at the optimum working conditions of the proposed biosensor.
The potential measurements were carried out for L-ascorbic acid, uric acid and glutamine without any other substance adding and determined the interference effect of both substance as a percentage. L-ascorbic acid and uric acid didn't show any important interference effect for the proposed biosensor.
Response time, lifetime and reproducibility of the glutamine biosensor
An ideal biosensor should response in a short time. The response time of the glutamine biosensor was determined by recording the time elapsed to reach a stable potential value after the biosensor and the reference electrode were immersed in calibration solutions. It was found that the response time of the biosensor was less than 1 min.
After our prepared glutamine biosensor was stored at + 4°C to determine the lifetime of it, the calibration graph of the biosensor was plotted 2 days a week. Although the analytical performances of the biosensor are very good, it was shown that the biosensor's life-time is about 1 month. At the end of 1 month, it couldn't be observed any response for the biosensor.
In order to determine the reproducibility of the glutamine biosensors, mV measurements were made in four serial glutamine calibration solutions at optimum pH of the biosensor in the same day and the slope was determined after each measurement from calibration graph. Numerically greatest slope value was considered as 100% the other slope values was calculated accordingly that. The results obtained that the reproducibility of our glutamine biosensor is very good.
Storage stability of the lysine biosensors
To determine the lifetime of the glutamine biosensor for a period of 45 days, and the linear working range and slopes were determined by drawing calibration graphs at each day during 45 days. The relative activity values were determined and plotted against days ().
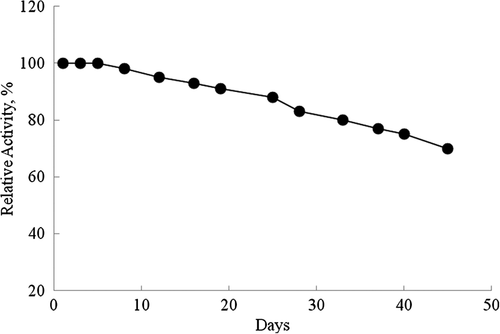
It is shown in , 10% and 20% of activity losses of the biosensor after 19 and 33 days, respectively. It can be said that our prepared glutamine biosensor based on glutaminase from H. jecorina and ZnO nanorod can be easily used in glutamine assay because of its long lifetime and wide linear working range.
Determination of kinetic constants of glutaminase from H. jecorina immobilized on the proposed glutamine biosensor
Maximum speed (Vmax) is highest speed that enzymatic catalysis reach maximum at this speed value. Michaelis Menten constant (Km) is the amount of substrate which it corresponds to half of the maximum speed. If an enzyme has lower Km value, it will be high-affinity against its substrate (CitationSegel 1993).
In order to determine Vmax and Km of the glutaminase enzyme from H. jecorina immobilized in the proposed biosensor, the initial reaction rates were separately measured by using glutamine calibration solutions at different concentrations between 1 and 10 mM at optimal pH and temperature values. Km and Vmax values were calculated by means of the Lineweaver–Burk plots (CitationLineweaver & Burk,1934; ). Km and Vmax values of the glutamine biosensor are found as 0.29 mM and 208.33 mV/min, respectively.
Determination of glutamine in GNC glutamine powder and recovery studies
After we optimized our prepared ZnO nanorod, chitosan and glutaminase from H. jecorina-based glutamine biosensor, we investigated whether it can be used in glutamine powder sample for glutamine determination using the standard addition method. After each addition of sample, the glutamine concentration was determined using the proposed biosensor and potential values were recorded. The original glutamine amount in GNC glutamine powder is presented in .
Table I. The results of glutamine content obtained from the proposed glutamine biosensor and the original value in GNC glutamine powder.
As it is seen in , the result obtained with our prepared glutamine biosensor is in good agreement with the original value. Therefore it can be said that glutamine assay was successfully made with our-produced biosensor without any interfering effect. As a result of this, it can be said that the presented ZnO nanorod, chitosan, and glutaminase from H. jecorina-based glutamine enzyme biosensor allowed the determination of glutamine with comparable accuracy to original value.
Conclusion
The paper presents the glutamine biosensor composed off glutaminase enzyme from H. jecorina, our produced-ZnO nanorod and chitosan. We obtained very good analytical results such as quick response time, high sensitivity, cheap, and simple biosensor construction with the biosensor. L-ascorbic acid and uric acid that can present in biological medium have not shown important interference effect on the response of the proposed biosensor. The determination of glutamine assay in GNC glutamine powder with the biosensor was also carried out.
Declaration of interest
The authors report no declarations of interest. The authors alone are responsible for the content and writing of the paper.
We gratefully acknowledge for the financial support of Yildiz Technical University Science Research Project Foundation (Project No: 2012-01-02-KAP10).
References
- Altman PL, Dittmer DS. eds. 1961. Blood and other boc and fluids. Federation of American Societies for Experimental Biology, Washington D.C.
- Aneesh PM, Vanaja KA, Jayaraj MK. 2007. Synthesis of ZnO nanoparticles by hydrothermal method. Nanophotonic Materials IV. 6639:66390J1–66390J9.
- Bülbül D, Karakuş E. 2013. Production and optimization of L-glutaminase enzyme from Hypocrea jecorina pure culture. Prep Biochem Biotechnol. 43:385–397.
- Çoğal S. 2009. The synthesis and optical-electronics characterization of zinc oxide nanoparticles based on microwave and autoclave at different crystal structure. MsD Thesis, Ege University, Science Institute.
- Dattelbaum JD, Lakowicz JR. 2001. Optical determination of glutamine using a genetically engineered protein. Anal. Biochem. 291:89–95.
- El-Sayed ASA. 2009. L-Glutaminase Production by Trichoderma koningii under solid state fermentation. Indian J Microbiol. 49: 243–250.
- Grabowska J, Nanda KK, McGlynn K, Mosnier JP, Henry MO, Beaucamp A, Meaney A. 2005. J Mater Sci: Mater Electron. 16:397.
- Greene LE, Law M, Tan DH, Montano M, Goldberger J, Somorjai G, Yang P. 2005. General route to vertical ZnO nanowire arrays using textured ZnO seeds. Nano Lett. 5:1231–1236.
- Imada A, Igarasi S, Nakahama K, Isono M. 1973. Asparaginase and glutaminase activities of microorganisms. J Gen Microbiol. 76:85–99.
- Karakus E, Pekyardımci S, Kılıc E. 2005. Urea biosensor based on PVC membrane containing palmitic acid. Artif Cells Blood Substit Immobil Biotechnol. 33:329.
- Katikala PK, Bobbarala V, Tadimalla P, Guntuku GS. 2009. Screening of L-Glutaminase producing marine bacterial cultures for extracellular production of L-Glutaminase. International Journal of Pharm Tech Research. 1:1232–1235.
- Kim KS, Kim HW. 2003. Synthesis of ZnO nanorod on bare Si substrate using metal organic chemical vapor deposition. Phys B: Condens Matter. 328:368.
- Lineweaver H, Burk D. 1934. The determination of enzyme dissociation constant. J Am Chem Soc. 56:658.
- Liu W, Greytak AB, Lee J, Wong CR, Park J, Marshall LF, et al. 2010. Compact biocompatible quantum dots via RAFT-mediated synthesis of imidazolebased random copolymer ligand. J Am Cheml Soc. 132:472–483.
- Lupan O, Chai G , Chow L. 2007a. Fabrication of ZnO nanorod-based hydrogen gas. nano sensor. Microelectr J. 38:1211–1216.
- Lupan O, Chow L, Chai G, Roldan B, Naitabdi A, Schulte A, Heinrich H. 2007b. Nanofabrication and characterization of ZnO nanorod arrays and branched microrods by aqueous solution route and rapid thermal processing. Mater Sci Eng B. 145:57.
- Moser I, Jobst G, Aschauer E, Svasek P, Varahram M, Urban G, et al. 1995. Miniaturized thin film glutamate and glutamine biosensors. Biosens Bioelectron. 10:527–532.
- Moser I, Jobst G, Urban GA. 2002. Biosensor arrays for simultaneous measurement of glucose, lactate, glutamate, and glutamine. Biosens Bioelectron. 17:297–302.
- Ogata K, Maejima K, Fujita S, Fujita S. 2003. Growth mode control of ZnO toward nanorod structures or high-quality layered structures by metal-organic vapor phase epitaxy. J Cryst Growth. 248:25.
- Polsongkrama D, Chamninok P, Pukird S, Chowb L, Lupan O, Chai G, et al. 2008. Effect of synthesis conditions on the growth of ZnO nanorods via hydrothermal method. Physica B. 403:3713–3717.
- Roth E, Zoch G, Schulz F, Karner J, Muhlbacher F, Hamilton G, et al. 1985. Amino acid concentrations in plasma and skeletal muscle of patients with acute hemorrhagic necrotizing pancreatitis. Clin Chem. 31:1305–1309.
- Saxena RK, Sinha U. 1981. L-asparaginase and glutaminase activities in the culture fi lterates of Aspergillus nidulans. Curr Sci. 50:218–219.
- Scriver CR, Altman P, Katz DD. 1977. Human Health and Dfiease. Federation of American Societies for Experimental Biology, Maryland. p.100.
- Segel IH. 1993. Enzyme Kinetics: Behavior and analysis of rapid equilibrium and. steady-state enzyme systems. Canada: John Wiley and Sons Inc.
- Shindia AA, Kkalaf SA, El-Sayed ASA. 2007. L-glutaminase production by some filamentous fungi. Bull Faculty of Science, Zagazig Univ, Egypt. 29:99–111.
- Villarta RL, Palleschi G, Suleiman A, Guilbault GG. 1992. Determination of glutamine in serum using an amperometric enzyme electrode. Electrochem. 4:27–31.
- Wade HE, Robinson HK, Philipsi BW. 1971. Asparaginase and glutaminase activities of bacteria. J Gen Microbiol. 69:299–312.
- Wakayama M, Yamagata T, Kamemura A, Bootim A, Yano S, Tachiki T, et al. 2005. Characterization of salt-tolerant glutaminase fromStenotrophomonas maltophilia NYW-81 and its application in Japanese soy sauce fermentation. J Ind Microbiol Biotechnol. 32:383–390.
- Wan Q, Yu K, Wang TH. 2003. Low-field electron emission from tetrapod-like ZnO nanostructures synthesized by rapid evaporation. Appl Phys Lett. 83:2253.
- Yamamoto S, Hirooka H. 1974. Production of glutaminase by Aspergillus sojae. J Ferment Technol. 52:564–569
- Yano T, Ito M, Tomita K, Kumagai H, Tochikura T. 1988. Purification and properties of glutaminase from Aspergillus oryzae. J Ferment Technol. 66:137–143.
- Zhang Y, Ram MK, Stefanakos EK, Goswami DY. 2012. Synthesis, Characterization, and Applications of ZnO Nanowires. J Nano mat. 2012:22 pages.