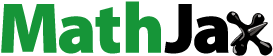
Abstract
Permeability plays an important role to achieve the desired therapeutic outcomes. Present study was designed to investigate the permeability of ciprofloxacin from ultrathin polyvinyl alcohol nanofiber through different biological membranes. Results of the permeability studies indicated that nanofibers exhibit higher drug permeability than plane drug. Intestinal tissue shows maximum permeability followed by eye, trachea, sublingual, rectal, and skin. Permeability studies also inferred a steady-state release of drug of the nanofiber, whereas a high degree of fluctuations was observed in plane drug. Nanofiber being 2D nanoscale material provides numerous advantages to be used as an encapsulated carrier system to improve the therapeutic effectiveness.
Introduction
In the recent years, the Biopharmaceutical Classification System (BCS) is one of the most significant prognostic tools shaped to stimulate product development (CitationGarg et al. 2012). The BCS serves as a scientific basis for categorizing drug substances on the basis of their aqueous solubility and intestinal permeability characteristics. The scientific basis will facilitate drug product selection and approval process for a large group of drug candidates. The BCS serves as a functional tool for developing in vitro dissolution specifications for drug products that are extrapolative of their in-vivo performance. BCS acclaims the methods for cataloguing according to dosage form dissolution along with solubility–permeability characteristics of the drug product (CitationAmidon et al. 1995, CitationReddy and Karunakar 2011). The absorption of drug is considered as a serial process in which there is dissolution of drug from the dosage form. After the dissolution, drug's solubility serves as a function of its physicochemical characteristics. After that drug's effective permeability through the intestinal mucosa and later pre-systemic metabolism of drug comes into play. Thus, the rate and extend of absorption of BCS class 4 drug is largely influenced by drugs solubility, or in other words, solubility is the rate-limiting step in determining the bioavailability of BCS class 4 drugs (CitationGarg et al. 2014).
In addition to the above, the viscoelastic layer of mucosa, pH at the site of the absorption, local microbial flora and enzymes pose a major barrier in the permeability of the drugs across the mucosal lining (CitationKaur et al. 2014a). Currently, there are several approaches studied by formulation scientists to improve the permeability of BCS class 4 drugs. The conventional approaches such as use of surfactant (CitationBakatselou et al. 1991), lipid-based systems (CitationO’Driscoll 2002, CitationCharman 2000, CitationPorter et al. 2007), solubilizer (CitationAhuja et al. 2007), prodrugs (CitationHussain and Rytting 1974), and permeation enhancers (CitationBabu et al. 2010) has been exploited as a probable possibility to improve the permeability of BCS class 4 drugs. Despite the considerable stride, only some have reached the place where they can provide safe and effective approach for the clinical application. Further conventional approach offers limited opportunity to alter the structural and functional modification of the carrier system, thus limits their performance, whereas electrospun nanofiber due to its unique properties enables a better permeability of the delivery system.
Electrospinning, a broadly used technology for electrostatic fiber formation which utilizes electrical forces to produce polymer fibers with the diameter ranging from 2 nm to several micrometers using polymer solutions of both natural and synthetic polymers, has seen a tremendous growth in research (CitationGopal et al. 2006, CitationSill and von Recum 2008). This process offers unique capabilities of producing novel natural nanofibers with controllable pore structure. Nanofibers have special characteristic features such as very large surface area-to-volume ratio, flexibility in surface functionalities, and superior mechanical performance compared with any other known form of material. These outstanding properties make the polymeric nanofiber optimal for many important applications (CitationHuang et al. 2003, CitationAgarwal et al. 2008, CitationLiang et al. 2007).
In the present study, polyvinyl alcohol (PVA) was selected as a polymeric component for its bioadhesive nature (CitationDing et al. 2012), solubilizing property (CitationOrienti et al. 2001), and penetration-enhancing property (CitationAn et al. 2003). The objective of this study is to formulate and characterize ciprofloxacin-loaded polymeric nanofiber system (PVA) and to investigate the efficacy of PVA nanofiber in the permeability model drug (ciprofloxacin) through different mucosal membranes. The objective of the study was further extended to investigate the effect of local anatomical and physiological conditions on the permeability of drugs from the proposed carrier system.
Experimentation
Materials
Ciprofloxacin was obtained from Alembic Pharmaceuticals, Baddi. Biodegradable PVA as a hydrophilic polymer soluble in water was procured from CDH, New Delhi. All the other chemicals used were of analytical grade.
Preparation of nanofiber
First, 10%w/v PVA was prepared by dissolving 1gm of PVA in 10ml of distilled water with constant stirring for 10 h. To the prepared PVA polymer solution, ciprofloxacin drug was added with constant stirring till the PVA solution becomes saturated with the drug. The drug-loaded PVA polymer solution was subjected to constant stirring again for the next 10 h. The polymer solutions were placed into a 2-mL syringe fitted to a needle with a tip diameter of 25 gauges (inner diameter, 0.25 mm) and were set up in the electrospinning apparatus. A syringe pump was used for delivering the polymer solution at a rate of 0.1 ml/h for PVA solution. Electrospinning was carried out at a voltage of 13 kV and with a needle-to-collector gap distance of 15 cm. The collector was covered with a thick aluminum foil on which the nanofibers were collected. The speed of the collector was fixed at 2000rpm.
Morphology
The morphology of the electrospun fibers and fiber diameter was examined using scanning electron microscopy EVO M-10 (Zeiss). At least 10 different positions on the fiber mat were tested to measure the diameter of the electrospun fibers. A small section of the fiber mat was placed on the SEM sample holder and sputter coated with platinum. Accelerating voltage of 15 kV was employed to take the SEM images. The SEM images were then used for the determination of average diameter of the fibers (CitationParnami et al. 2013).
Differential scanning calorimetery
The phase transition of plain drug and drug-loaded nanofiber was monitored to evaluate the thermodynamic behavior of the drug in the developed formulation. In brief, differential scanning calorimetery measurement was done with a sample weight of 3–5 mg under a nitrogen atmosphere with a scanning speed of 10°C/min. The samples were heated from 0 to 400°C at a rate of 10°C/min (CitationGarg and Goyal 2014).
Drug entrapment efficiency
The drug entrapment efficiency of nanofibers was calculated by drying the drug-loaded nanofibers at 40°C inside a hot air oven, and then, a nanofiber mat of known area (1 × 1cm) was removed and dissolved in water. The amount of drug in the solution was determined using UV Spectroscopy at 272 nm (CitationGagandeep et al. 2014). The percentage entrapment was calculated using the following formula:
Degree of swelling
The degree of swelling of the developed nanofiber mats was calculated by Eq. (1). The test was carried out in the release medium (phosphate-buffered saline [PBS], pH 7.4) at 37°C for 4, 8, and 12h.
where M is the weight of swollen nanofibers sample which is wiped dry with filter paper, and Md is the dried mass of immersed sample in buffer medium, measured by drying the swollen nanofiber mats in an oven at 40°C until constant weight was reached (CitationKataria et al. 2014).
Solubility study
The solubility study of the plain drug and drug-loaded nanofiber was carried out in water using dynamic equilibrium technique. A minimum amount of solvent was selected, and an incremental quantity of drug was added into the solvent until the solution was saturated with the drug. After complete saturation, the solution was filtered and the concentration of the drug in the solution was estimated by measuring the UV absorbance, and the solubilities of the plain drug and the drug loaded in the nanofiber were compared. The procedure used was repeated in triplicate, and the values are mean of three experiments (CitationSingh et al. 2014).
In vitro drug release studies
Drug release from electrospun nanofiber mats was measured by placing a known area of 1 × 1 cm of drug-loaded nanofiber inside the dialysis membrane bag containing PBS, and the bag was immersed into 100 of mL PBS, the release studies were carried out at 37°C at 100 rpm in a thermostatic shaking incubator. Samples were withdrawn at predetermined time intervals for analyzing the released drug. After each sampling, the volume was replaced with fresh medium. The amount of drug present in the released buffer was determined using a UV spectroscopy at a λmax of 272 nm. The release experiments of each sample were performed in triplicate, and the average values are reported. The percent drug release was calculated using the calibration curve of the drug in PBS 7.4 (CitationKaur et al. 2014b).
Ex vivo permeability study
For intestine and trachea
Drug release from electrospun nanofiber mats was measured by placing a known area of (1 × 1 cm) of drug-loaded nanofiber inside the intestine. The ends of the intestinal duct were then tied off with silk thread. Intestinal tube containing nanofibers was immersed into 100 mL of PBS, and the studies on drug release were carried out at 37°C at 100 rpm in a thermostatic shaking incubator. Samples were withdrawn after 0, 30, 60, 90, 120, 150, 180, 210, 240, 270, 300, 330, and 360 min. After each sampling, the volume was replaced with fresh medium. The amount of drug present in the release buffer was determined using a UV–visible spectrophotometer at a λmax of 272 nm. The experiments on drug release of each sample were performed in triplicate, and average values were reported. The percent drug release was calculated using the calibration curve of the drug in PBS 7.4. The drug permeability from trachea was determined by using the procedure as described above (CitationGoyal et al. 2013).
For eye, rectal and sublingual
Drug permeability studies were carried out using freshly excised goat tissue. The receptor compartment of Franz diffusion cell was filled with freshly prepared PBS solutions. Freshly excised tissue was fixed between clamped donor and the receptor compartment in such a way that its epithelial surface was oriented toward the donor compartment. The release studies were carried out at 37°C. Samples were withdrawn after 0, 30, 60, 90, 120, 150, 180,210, 240, 270, 300, 330, and 360 min. After each sampling, the volume was replaced with fresh medium. The amount of drug present in the release medium was determined using a UV spectroscopy at a λmax of 272 nm. The release experiments of each sample were performed in triplicate, and average values are reported. The percent drug release was calculated using the calibration curve of the drug in PBS 7.4.
Results and discussion
Morphology
The results obtained from SEM studies were investigated, and the average diameter of polymeric nanofibers was predicted. The average diameter of PVA nanofibers was ranging from 200 to 400nm. SEM images of unloaded nanofibers () were also further compared with SEM images of drug-loaded nanofibers (). There was no evidence for any significant changes in the average fiber diameter values. SEM images of plane polymeric nanofibers and drug-loaded nanofiber further revealed a non-woven mat structure and smooth surface of the nanofibers.
Drug entrapment efficiency
Since electrospinning leads to in situ solidification of the polymer solution in nanoscopic scale ensuring 100% drug entrapment efficiency provided the drug is non-volatile in nature. The drug entrapment efficiency as calculated using UV analysis was found to be 95%, and therefore in the case of nanofibers, it depends on the solubility of drug in the polymer solutions. Slight decrease in entrapment efficiency could be related to fiber yield which tends to be with running time. This could be due to decreasing potential drainage capacity of the collector as the fiber get deposited on the surface of the collector leading to distraction of fiber from the collector site.
Solubility study
Solubility studies indicated that there is marked increase in drugs solubility in nanofiber (28mg/ml) compared with that of plain drug (15.8 mg/ml). This could be due to the formation of molecular dispersion of drug in the polymeric base. Molecular dispersion improves drug dissolution by decreasing the particle size and improving wettability. This finding may be correlated to the phase transition temperatures, where the drugs in nanofibers exhibit low-phase transition point (317°C) compared with plain drug (329°C). The amorphous dispersion of the drug in the polymeric nanofiber is further associated with the antiplasticizer effect of PVA and rapid in situ solidification of electrospinning jet, resulting in lower molecular mobility that could inhibit the drug molecules to form a stable crystals. Also, the exceptionally large-surface-area-to-volume ratio and microporous network with tunable pore size of nanofiber contribute significantly in improving drug solubility. Moreover, the PVA being a surfactant helps to stabilize the drug molecule with minimum free surface energy in the form of loose aggregates.
Degree of swelling
The degree of swelling of a nanofiber mat plays a major role in the release of drug from the nanofiber mat (). The swelling capacity of a polymer is determined by the amount of liquid material that can be absorbed. The degree of swelling is an important parameter of this study because the water which penetrates the nanofiber system was dissolving the encapsulated drug and thus converts the drug into the liquid form, thus making it easier to penetrate into the biological membrane. The degree of swelling of developed mats in PBS 7.4 was found to be 135% and 145% for blank and drug-loaded nanofiber, repectively, after 4 h which increased to 177%, 190% after 8 h, and in later 12 h, it was found to be 145% and 155%. It can be correlated to the average diameter of these fibers. However, it was observed that after 8 h the swelling index tends to decrease which could be due to the fact that the polymer is soluble in the dissolution medium, and hence, it diminishes its ability to swell.
In-vitro drug permeability study
The data obtained from the in vitro release study of the plain drug and the polymeric nanofiber from the dialysis membrane is shown in and , respectively.
The release study was carried out in Franz diffusion cell using dialysis membrane. Results indicated an initial burst release of 25% in 1 h followed by a steady-state release profile comprising of a cumulative release of 97.8% after 8 h. The initial burst release most likely be due to the rapid release of surface-associated drug molecules followed by a controlled release, where the release is governed by both diffusion and erosion mechanisms from the matrix. The reference data are also supported by the studies on swelling where the degree of swelling was found to be maximum after 4 h, indicating an extensive diffusion process supported by low swelling indices due to polymer erosion which demonstrates both diffusion and erosion mechanisms to be operative during drug release from developed nanofiber.
Ex vivo drug permeability study
The data obtained from Ex-vivo permeability study through the various mucosal sites are shown in and .
Ex vivo drug permeability study indicates that drug-loaded nanofiber shows enhanced drug permeability compared to the plain drug. Higher drug solubility and ability of maintaining the concentrations gradient over a prolonged period of time could be the driving forces behind the increased drug permeability of nanofiber. Further PVA because of its solubilizing and wetting property reduces the interfacial tension, thereby facilitating the paracellular permeability of drug across the biological membranes. Moreover, the bio-adhesive nature of PVA ensured the retention of carrier system at the absorption site which acts as a key driving force for diffusion. Moreover, the high surface area of PVA nanofiber results a higher degree of cross-linking with the mucosal tissue that allows greater control of drug release, and PVA modulates transport pathways by opening epithelial tight junction to promote the drug diffusion.
Furthermore, the results of permeability studies indicated that drug permeability is highest in intestinal mucosa followed by ophthalmic > pulmonary > sublingual > rectal tissue. Maximum drug permeability across intestinal mucosa could be attributed to the large surface area assisting greater exchange of drugs. Comparative low drug absorption from ocular tissue may be related to limited surface area and tight junction of apical corneal epithelium. Moreover, comparative poor drug permeability in trachea may be related to high collagen content in tracheal membrane. However, a higher tracheal permeability of drug over rectal tissue could be associated with surface area of tracheal tissue with cilia. The least rectal permeability is associated with low surface area of rectal tissue. Overall, the results of permeability studies inferred that nanofiber exhibits higher drug permeability compared to the plain drug.
Conclusions
Solubility is a key issue to improve the permeability of class 4 drugs. Nanofiber due to its multifunctional properties offers enormous opportunity and potential to improve drug solubility of poorly aqueous soluble drugs. Further, the advent in polymer science and simple electrospinning technique enables us to fabricate and modulate the properties of nanofiber to achieve the desired pharmaceutics and pharmacological properties of encapsulated therapeutics.
Declaration of interest
The authors report no declarations of interest. The authors alone are responsible for the content and writing of the paper.
References
- Agarwal S, Wendorff JH, Greiner A. 2008. Use of electrospinning technique for biomedical applications. Polymer. 49: 5603–5621.
- Ahuja N, Katare OP, Singh B. 2007. Studies on dissolution enhancement and mathematical modeling of drug release of a poorly water-soluble drug using water-soluble carriers. Eur J Pharm Biopharm. 65: 26–38.
- Amidon GL, Lennernäs H, Shah VP, Crison JR. 1995. A theoretical basis for a biopharmaceutic drug classification: the correlation of in vitro drug product dissolution and in vivo bioavailability. Pharm Res. 12: 413–420.
- An NM, Kim DD, Shin YH, Lee CH. 2003. Development of a novel soft hydrogel for the transdermal delivery of testosterone. Drug Dev Ind Pharm. 29: 99–105.
- Babu VR, Areefulla S, Mallikarjun V. 2010. Solubility and dissolution enhancement: an overview. J Pharm Res. 3: 141–145.
- Bakatselou V, Oppenheim RC, Dressman JB. 1991. Solubilization and wetting effects of bile salts on the dissolution of steroids. Pharm Res. 8: 1461–1469.
- Charman WN. 2000. Lipids, lipophilic drugs, and oral drug delivery-some emerging concepts. J Pharm Sci. 89: 967–978.
- Ding J, He R, Zhou G, Tang C, Yin C. 2012. Multilayered mucoadhesive hydrogel films based on thiolated hyaluronic acid and polyvinylalcohol for insulin delivery. Acta Biomater. 8: 3643–3651.
- Gagandeep GARG, T., Malik B, Rath G, Goyal AK. 2014. Development and characterization of nano-fiber patch for the treatment of glaucoma. Eur J Pharm Sci. 53: 10–6.
- Garg T, Goyal AK. 2014. Biomaterial-based scaffolds – current status and future directions. Expert Opin Drug Deliv. 11: 767–789.
- Garg T, Rath G, Goyal AK. 2014. Comprehensive review on additives of topical dosage forms for drug delivery. Drug Deliv.(Epub ahead of Print).
- Garg T, Singh O, Arora S, Murthy R. 2012. Scaffold: a novel carrier for cell and drug delivery. Crit Rev Ther Drug Carrier Syst. 29: 1–63.
- Gopal R, Kaur S, Ma Z, Chan C, Ramakrishna S, Matsuura T. 2006. Electrospun nanofibrous filtration membrane. J Memb Sci. 281: 581–586.
- Goyal G, Garg T, Malik B, Chauhan G, Rath G, Goyal AK. 2013. Development and characterization of niosomal gel for topical delivery of benzoyl peroxide. Drug Deliv. (Epub ahead of Print).
- Huang Z-M, Zhang Y-Z, Kotaki M, Ramakrishna S. 2003. A review on polymer nanofibers by electrospinning and their applications in nanocomposites. Compos Sci Technol. 63: 2223–2253.
- Hussain A, Rytting JH. 1974. Prodrug approach to enhancement of rate of dissolution of allopurinol. J Pharm Sci. 63: 798–799.
- Kataria K, Garg T, Rath G, Goyal AK. 2014. Novel technology to improve drug loading in polymeric nanofibers. Drug Deliv Lett. 4: 79–86.
- Kaur M, Garg T, Rath G, Goyal AK. 2014a. Current nanotechnological strategies for effective delivery of bioactive drug molecules in the treatment of tuberculosis. Crit Rev Ther Drug Carrier Syst. 31: 49–88.
- Kaur M, Malik B, Garg T, Rath G, Goyal AK. 2014b. Development and characterization of guar gum nanoparticles for oral immunization against tuberculosis. Drug Deliv. DOI.:https://doi.org/10.3109/10717544.2014.894594.
- Liang D, Hsiao BS, Chu B. 2007. Functional electrospun nanofibrous scaffolds for biomedical applications. Adv Drug Deliv Revi. 59: 1392–1412.
- O’Driscoll CM. 2002. Lipid-based formulations for intestinal lymphatic delivery. Eur J Pharm Sci. 15: 405–415.
- Orienti I, Bigucci F, Gentilomi G, Zecchi V. 2001. Self-assembling poly(vinyl alcohol) derivatives, interactions with drugs and control of release. J Pharm Sci. 90: 1435–1444.
- Parnami N, Garg T, Rath G, Goyal AK. 2013. Development and characterization of nanocarriers for topical treatment of psoriasis by using combination therapy. Artif Cells Nanomed Biotechnol. DOI:https://doi.org/10.3109/21691401.2013.837474.
- Porter CJ, Trevaskis NL, Charman WN. 2007. Lipids and lipid-based formulations: optimizing the oral delivery of lipophilic drugs. Nat Rev Drug Discov. 6: 231–248.
- Reddy BBK, Karunakar A. 2011. Biopharmaceutics classification system: a regulatory approach. Dissolution Technol. 18: 31–37.
- Sill TJ, von Recum HA. 2008. Electrospinning: applications in drug delivery and tissue engineering. Biomaterials. 29: 1989–2006.
- Singh H, Sharma R, Joshi M, Garg T, Goyal AK, Rath G. 2014. Transmucosal delivery of Docetaxel by mucoadhesive polymeric nanofibers. Artif Cells Nanomed Biotechnol. (Epub ahead of Print).